Abstract
Soil macroarthropods are organisms considered as key factors to sustain soil quality and sustainability due to their services to soil ecosystem. The community structure and seasonal variation of soil macroarthropods in 5-year cultivation of green manure plant species in the Brazilian semi-arid, Areia, Paraiba, Brazil were investigated between June and December 2018. Soil samples and macroarthropod specimens were collected using soil cores and Provid-type traps, respectively, during rainy and dry seasons. We characterized both soil chemical properties and macroarthropod community under ten green manure plant species: Brachiaria decumbens Stapf. cv. Basilisk, Canavalia ensiformis (L.) DC, Crotalaria juncea L., Crotalaria ochroleuca G. Don, Crotalaria spectabilis Roth, Lablab purpureus (L.) Sweet, Mucuna pruriens (L.) DC., Neonotonia wightii (Wight & Arn.) J.A. Lackey, Pennisetum glaucum L., and Stilozobium aterrimum Piper & Tracy. The highest values of richness, diversity and dominance in the macroarthropod community during the rainy season were found on the plots where C. spectabilis and M. pruriens were cultivated, whereas during the dry season the highest values were found in the plots where C. ochroleuca, C. spectabilis, and S. aterrimum were cultivated. In the plots where B. decumbens and P. glaucum where cultivated, we found lower values of richness, diversity, and dominance in the macroarthropod community compared to the leguminous plant species during rainy and dry seasons. Our findings suggest that: 1) green manure practice and seasonality changed soil macroarthropod community, trophic structure, and ecological processes in semi-arid conditions; and 2) abundance of predators, ecosystem engineers, decomposers and herbivores was promoted by plant species from Fabaceae family. The results highlight the importance to consider plant species from Fabaceae family as habitat and food resources for soil fauna community.
Similar content being viewed by others
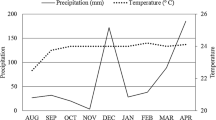
Explore related subjects
Discover the latest articles, news and stories from top researchers in related subjects.Avoid common mistakes on your manuscript.
Introduction
The Brazilian semi-arid is an ecoregion that covers 1,128,697 km2 of Brazil’s territory and comprises the states of Alagoas, Bahia, Ceara, Minas Gerais, Paraiba, Pernambuco, Piaui, Rio Grande do Norte and Sergipe (Silva et al. 2018). This ecoregion is characterized by frequent dry periods, annual rainfall lower than 800 mm, aridity index lower than 0.40 and a unique soil macroarthropod community (Souza et al. 2016). However, both the diversity and the dominance of this soil organism community are often restricted by seasonal variation and the quality of soil organic matter (Souza et al. 2016; Coyle et al. 2017; Roy et al. 2018; Melo et al. 2019).
In this context, the cultivation of plant species (e.g., that supports high biomass production) during the rainy season in the Brazilian semi-arid might play a significant role in the macroarthropod community composition and their services to the ecosystem in a subsequent dry season, where there is a significant reduction of resources and habitats (Solen et al. 2018). According to Amazonas et al. (2018) and Souza and Freitas (2018), plant species of the Fabaceae family with fast growth and high rate of biomass production influence positively the species richness, Shannon’s diversity index and Simpson’s dominance index of the native macroarthropod community in Brazilian semi-arid conditions. Leguminous species, such as Crotalaria spectabilis Roth and Mucuna pruriens (L.) DC., can increase macroarthropod richness (e.g., on average 200% higher species richness and 140% higher Shannon’s diversity index than in non-legume species) (Melo et al. 2019). Understanding the effect of the addition of these legumes which may regulate the macroarthropod diversity in a semi-arid ecoregion is essential to explain why soil fauna groups (e.g., litter transformers, herbivores and predators) become less frequent during the dry season (Manwaring et al. 2018; Roy et al. 2018; Nunes et al. 2019).
In this work, we hypothesized that leguminous plant species can promote soil macroarthropod abundance during both rainy and dry seasons. In the first one, leguminous plant species promote the soil organisms group due to increases in the fauna’s habitat structure, while in the second one (e.g., when plant biomass was incorporated into the soil profile), we promote soil macroarthropod abundance and diversity due to increases in both food and habitat of soil fauna community. Based on the enemies and resource concentration hypotheses, we expected to find higher macroarthropod abundance during the dry period in plots where Fabaceae plant species were cultivated, due to their high biomass production which acts following soil incorporation as both habitat and food for a diverse soil macroarthropod community (Silva et al. 2013; Souza et al. 2016; Melo et al. 2019). Our aim was to determine whether the long-term green manure practice and seasonality alters soil macroarthropod community structure in an agroecosystem of the Brazilian semi-arid. Based on the studies developed by Vasconcellos et al. (2010), Shakir and Ahmed (2015), and Bento et al. (2016), evaluating seasonal variation of soil macroarthropods in semi-arid conditions, we expected to find variation in abundance and diversity of macroarthropod group in our study conditions. To accomplish this, we performed a field experiment and sampled soil macroarthropods in the rainy and dry seasons of an agroecosystem with long-term cultivation of plant species used as green manure in the Brazilian semiarid.
Materials and methods
Study system, climatic conditions, and soil type
Sampling was carried out in a long-term field experiment using green manure plants at the “Chã-de-Jardim” Experimental Station, Agrarian Sciences Centre, Federal University of Paraiba (CCA-UFPB), located in Areia, Paraiba, Brazil (06°58′12” S, 35°42′15” W, altitude 619 m above sea level). The climate in the experimental area is tropical with dry-summer characteristics (e.g., As-type following Köppen climate classification), with average annual precipitation and air temperature of 1500 mm and +21 °C, respectively (Alvares et al. 2013). Climate data, monthly rainfall and mean air temperature for Areia, Paraiba, Brazil (June to December 2018) (Fig. 1) were obtained online: http://www.inmet.gov.br. Green manure farming system started in 2014 using the same treatments in each studied year (for more details about the studied treatments see Souza et al. 2018 and Melo et al. 2019). The plants used as green manure were representatives of two families: Fabaceae and Poaceae. The soil type of the experimental area was classified as Regosol (WRB 2006).
Monthly rainfall (grey bars) and air temperature (black line) data from the experimental area in Areia, Paraiba, Brazil (June to December 2018); data were obtained online: http://www.inmet.gov.br
Experimental design
The results of this study were obtained in 2018. We analysed the effects of different plant species cultivated in a green manure farming system for five consecutive years. The field experiment was allocated in a randomized block design consisting of ten treatments of one plant species (Table 1). For more details about soil prepare, fertilizers, liming, fertilization doses and its application mode see Souza et al. (2018). Each treatment plot (6 × 4 m) was replicated five times (blocks). All plant species were sown at a seeding rate of 400 seeds m−2 at a 2-cm depth and the plots were spaced 0.5 m apart.
Soil macroarthropod sampling
To sample soil macroarthropod individuals, we used the Tropical Soil Biology and Fertility method (Anderson and Ingram 1993). We did not find any nest (i.e., ants and termites nests) in the plots or nearby our field experiment. During the whole rainy and dry seasons, we placed one Provid-type trap (each trap had four windows with 4 × 4 cm opening) in the center of each plot and at a soil depth of 20 cm following a 2-days schedule without any disruption to collect soil macroarthropod specimens (e.g., We placed the traps 31 times during the rainy season, whereas during the dry season we placed the traps 41 times), but we present the mean of each season in our results. Into each Provid-type trap, we added 200 ml of a distilled water and neutral detergent solution at a concentration of 25% plus 30 ml of 70% alcohol. Only the soil macroarthropod specimens longer than 0.2 cm were preserved in 70% alcohol and considered for our analyses. All individuals were then sorted, counted and classified into the following taxonomic units (Order – Family): Araneae – Filistatidae, Blattodea – Blattidae, Blattodea – Termitidae, Coleoptera – Carabidae, Dermaptera – Forficulidae, Diptera – Muscoidea, Hemiptera – Cercopidae, Homoptera – Membracidae, Hymenoptera – Formicidae, larvae of Coleoptera - Passalidae, larvae of Lepidoptera – Pyralidae, Neuroptera – Ithonidae, Orthoptera – Grylloidea, Scorpiones – Scorpionidae and Thysanoptera – Thripidae. The community structure of soil macroarthropod in each studied treatment was characterized by the following parameters: mean abundance (ind. m−2) of soil macroarthropod taxonomic groups (where we divided the mean number of individuals obtained into each trap by 24 – the area in m2 of each plot), richness - S, Shannon diversity index – H′ (Shannon and Weaver 1949) and Simpson dominance index – C (Simpson 1949). In addition, we classified the functional group of each taxonomic unit following criteria described by Souza and Freitas (2018).
Plant dry biomass production and soil chemical properties
Plant dry biomass, soil reaction (i.e., soil pH) and total organic carbon were measured at each studied plot. We selected ten plants per plot, that were harvested at 8–10 cm above the ground level. We used the plant dry biomass of these ten plants to estimated plant biomass production in t ha−1. We used two equations to accomplish it. First, we estimated the number of plants per hectare: NP = density of plants per plot × 10,000 m2 /24 m2, where the density of plants per plot was provided in Table 1, 10,000 m2 is the area of one hectare, and 24 m2 is the area of each plot. Next, we estimated the plant dry biomass using the formula: PDB (t ha−1) = NP × BP10 × 100, where the NP is the number of plants per ha, BP10 is the dry biomass of the 10 sampled plants per plot, and 100 is a factor to convert the results from kg ha−1 to t ha−1.
To characterize soil reaction and total organic carbon, the soil samples were collected at the beginning of each studied month. Samples were collected at a depth of 0–20 cm, air-dried and passed through a 2-mm sieve. We determined soil reaction in a suspension of distilled water and soil (Black 1965). Total organic carbon was determined by rapid dichromate oxidation method following the protocol described in Okalebo et al. (1993).
Index of soil quality
An index of soil quality was calculated using the PCA-LSF-SQIw approach, described by Mishra et al. (2017), which combines soil physical and chemical characteristics, plant biomass production, and soil macroarthropod community measured at all studied plots. Based on this approach we developed a model (Eq. 1) to determine the index of soil quality (ISQ). High values of ISQ indicated a high-class soil that provides habitat and food to a diverse and abundant soil biota community and plant biomass production without negative effects to soil ecosystem (Online Resource 1).
where: NH-F = Normalized values of Hymenoptera-Formicidae abundance (ind. m−2), NLc-P = Normalized values of larvae of Coleoptera - Passalidae abundance (ind. m−2), NPDB = Normalized values of plant dry biomass (t ha−1), NB-T = Normalized values of Blattodea - Termitidae abundance (ind m−2), NC-C = Normalized values of Coleoptera-Carabidae abundance (ind m−2), and Nsoil pH = Normalized values of soil pH in water. All normalized values were obtained dividing the mean of each component by their scores obtained in a PCA analysis.
Statistical analyses
Soil macroarthropods presence was converted into abundance, expressed as number of individuals per square meter (ind. m−2) for each studied plot. Since the Shapiro-Wilk test indicated normality of the data, we used the F-test in two-way ANOVA to compare soil macroarthropods abundance, richness, diversity, dominance and soil quality index among all studied treatments (i.e., plant species vs. seasons). We used the Bonferroni test to compare soil macroarthropod community structure at plots with plant species used as green manure during dry and rainy seasons. We performed PCA to outline the relationship between the soil macroarthropod community structure and soil chemical properties. All statistical analyses were performed using the packages ade4 and vegan within R software (R Core Team 2018).
Results
Seasonal variation of soil macroarthropod abundance, diversity, and dominance among green manure species
The mean abundances of soil macroarthropods varied significantly among plant species and studied seasons (p < 0.001). The most abundant taxonomic group was Hymenoptera – Formicidae. This taxonomic group had abundances varying from 14.3 ± 0.8 (S. aterrimum plots) to 34.0 ± 1.4 (M. pruriens plots) during the rainy season, while in the dry season its abundance varied from 28.0 ± 2.4 (C. spectabilis plots) to 197.3 ± 15.0 (B. decumbens plots) (Table 2).
Effect of green manure farming system on soil macroarthropod community
The two-way ANOVA results showed significant differences among plant species and studied seasons on species richness (F9,77 = 10.70, p < 0.01), Shannon’s diversity index (F9,77 = 108.02, p < 0.001), and Simpson’s dominance index (F9,77 = 61.163, p < 0.001). The highest values of species richness, Shannon’s diversity index, and Simpson’s dominance index in the rainy season were found on plots where C. spectabilis was cultivated (6.00 ± 0.17; 1.32 ± 0.02; and 0.65 ± 0.09, respectively). During the dry season, we found the highest values of species richness, Shannon’s diversity index and Simpson’s dominance index on plots where C. ochroleuca, S. aterrimum and C. spectabilis were cultivated, respectively (Table 3).
Plant dry biomass production and soil chemical properties
The two-way ANOVA results showed significant differences among plant species and studied seasons on plant dry biomass (F9,77 = 23.67, p < 0.01). The highest plant dry biomass was found on the plots where L. purpureus (14.0 ± 0.1 T ha−1) was cultivated during dry season. We also found that there was an increase in plant dry biomass production from rainy to dry season on plots where C. ensiformis, C. spectabilis, C. ochroleuca, L. purpureus and N. wightii were cultivated, whereas there was a decrease on plots where B. decumbens, C. juncea, M. pruriens, P. glaucum and S. aterrimum were cultivated (Fig. 2).
The one-way ANOVA results showed significant differences among the studied plant species used as green manure on total organic carbon (F9,3483 = 26.63, p < 0.001) and soil pH (F9,3483 = 11.19, p < 0.001). The S. aterrimum plants were useful to promote total organic carbon, whereas Poaceae as a group (i.e., B. decumbens and P. glaucum) promoted both soil chemical properties (Table 4).
Multivariate analyses
Principal component analyses (PCA) showed that Hymenoptera, larvae of Coleoptera, plant dry biomass, Blattodea, Coleoptera and soil pH were the principal components contributing to the variance of the data. The PCA also showed: i) high difference between wet and dry season (along PC1) with lower variability in dry season (along PC2); ii) positive relation between plant dry biomass, larvae of Coleoptera and Araneae; iii) positive relation between soil pH and Hymenoptera; iv) negative relation between total organic carbon and Hymenoptera; v) positive relation between Homoptera, Blattodea-Termitidae and Coleoptera; and vi) negative relation between soil pH and Hemiptera (Fig. 3).
PCA score plot of soil chemical properties, plant dry biomass production and soil macroarthropod groups for two Family groups (Poaceae and Fabaceae) during two studied seasons (rainy and dry). Circles and squares represent samples from each plot for Fabaceae and Poaceae groups, respectively. The two axes explained 80.55% (PC1 = 59.36% and PC2 = 21.19%) of the total variance
Soil macroarthropod diversity and soil biological quality index
Soil biological quality index was reduced when P. glaucum plants were cultivated in our experiment. We found the highest values of soil biological quality index in both rainy and dry seasons on the plots where M. pruriens was cultivated (13.72 ± 0.98 and 48.55 ± 2.33, respectively). Overall, the soil biological quality index was affected by the studied plant species and seasonality (Table 5).
Discussion
Our results emphasize the influence of leguminous species and seasonality on soil macroarthropod community composition (e.g., abundance, richness, diversity, and dominance) and soil biological quality index in a semi-arid environment. Essentially, we wanted to understand how both the biomass production (e.g., during rainy season) and biomass incorporation into soil profile (e.g., during dry season) of plant species used as green manure changes the macroarthropod communities during the rainy (e.g., when the plant biomass acts as fauna’s habitat) and dry seasons (e.g., when the plant biomass was incorporated into soil profile and acts as both fauna’s habitat and food resource). The results of this study revealed that there were significant differences among the studied plant species and seasons on macroarthropod abundance and occurrence. According to Melo et al. (2019), plant species from Fabaceae family, such as C. spectabilis and M. pruriens, showed high macroarthropod richness, Shannon’s diversity index and Simpson’s dominance index on a Regosol in field conditions. These authors also report that these two plant species present the following characteristics: i) high quality of their residues; ii) high root activity; iii) fast growth; and iv) high water use efficiency.
These results agree with previous works (Moura et al. 2015; Roy et al. 2018) that reported high soil rootability in the rhizosphere of plant species used as green manure. By altering soil rootability in their rhizosphere, plant species may alter soil reaction by the extrusion of H+ and some organic compounds (e.g., citric acid) and thus may be responsible for the abundance and occurrence of macroarthropod community composition in plots where plant species of Fabaceae family were cultivated (Delgado-Baquerizo et al. 2018; Silva et al. 2018; Vukicevich et al. 2016). Our hypothesis that leguminous plant species promote soil macroarthropod abundance independently of the studied season was supported, except for C. juncea, which presented similar values of richness, diversity, and dominance for both B. decumbens and P. glaucum. Overall, the macroarthropod community and plant status in C. juncea, B. decumbens and P. glaucum plots were characterized by a low abundance or even absence of some predators (e.g.,. Araneae - Filistatidae, Coleoptera - Carabidae, Dermaptera - Forficulidae, Scorpiones - Scorpionidae), absence of herbivores (e.g., Thysanoptera - Thripidae, Hemiptera - Cercopidae, Orthoptera - Grylloidea, Homoptera - Membracidae), and high abundance of decomposers (e.g., Diptera - Muscoidea) and ecosystem engineers (e.g., Hymenoptera - Formicidae) which created a negative effect in the trophic structure by disrupting some important ecological process such as biological control, mutualism, parasitism and nutrient cycling (Souza and Freitas 2017, 2018; Zhang et al. 2018; Mishra et al. 2017).
For plant dry biomass production, soil organic carbon and pH, L. purpureus, S. aterrimum and P. glaucum showed higher values of these variables among the other studied plant species. However, these results do not support our hypothesis that high biomass production may improve the diversity of soil macroarthropod community. In fact, high biomass production only acts improving soil macroarthropod abundance as described by Moura et al. (2015). It can be supported by our results for soil macroarthropod abundance during rainy season. Although our experiment was not designed to directly test whether root activity affects macroarthropod diversity, we must consider that the highest diversity and soil biological quality index was found on plots where C. spectabilis and C. ochroleuca were cultivated. According to Melo et al. (2019), the high diversity of soil macroarthropod community presented on the plots cultivated with plant species of Crotalaria genus may be related to their high N content in plant tissue, fast growth, and soil cover rates. We cannot exclude the habitat quality and nutrient hypotheses described by Moura et al. (2015) and Melo et al. (2019), in which macroarthropod diversity in agroecosystems is driven by litter quality (e.g., nutrient content hypothesis), which can be supported by results on the plots where C. spectabilis and C. ochroleuca based on Shannon’s diversity index. These results agree with previous studies done by Marichal et al. (2014); Kamau et al. (2019); and Guo and Wang (2019), which reported that the constant amendment with high quality soil residues on soil ecosystems directly influence functional groups of soil macroarthropod community [e.g., litter transformers (Diplopoda – Spirobolida and Coleoptera - Scarabaeidae), ecosystem engineers (Blattodea – Termitidae), predators (Araneae – Filistatidae, and Dermaptera - Forficulidae), Decomposers (Diptera – Muscoidea) and herbivores (Orthoptera – Gryllotalpidae)].
Plant biomass production during rainy season and the quality of the plant biomass incorporated into the soil profile during dry season are two important drivers to soil macroarthropod community structure and functional groups (Law et al. 2019). Functional groups, especially the detritivores (larvae of Coleoptera – Passalidae) were found more abundantly during the dry season, due to their necessity of soil habitat quality (e.g., improved by plant biomass incorporation into the soil profile) that acts as refuge against predators, such as Dermaptera -Forficulidae or even Coleoptera - Carabidae group (Costa et al. 2009; Souza and Freitas 2018; Silva et al. 2013; Souza et al. 2016; Melo et al. 2019). Considering some relationships among the functional groups that we observed in our study, we found positive effects on trophic structure during dry season on plots where plant species of Fabaceae family were cultivated. In these plots, we found a significant abundance of both predators (e.g., Araneae - Filistatidae, Dermaptera – Forficulidae, and Scorpiones - Scorpionidae) and herbivores (e.g., Thysanoptera - Thripidae, Hemiptera - Cercopidae, Orthoptera - Grylloidea, Homoptera - Membracidae), which may be related to active biological control processes. These results agree with Yang et al. (2018) and Balkenhol et al. (2018), who reported high abundance of predators (e.g., both spider and beetle communities) in soil ecosystems with aboveground plant species characterized by fast growth and high biomass production. In both studied conditions, these authors reported that the biomass production acts promoting herbivory community and activity by improving food resource. Once herbivores abundance is improved, plant biomass production directly and indirectly promotes predator community abundance by acting as habitat and improving their food resource (e.g. herbivore specimens), respectively (Ng et al. 2018). Ecosystem engineers (e.g., Hymenoptera -Formicidae) were the most abundant functional group during both rainy and dry season. This result agrees with Yang et al. (2018) who reported ecosystem engineers as the largest functional groups in different land uses in eastern coast of China. Antoniazzi et al. (2019); Melo et al. (2019) and Wink et al. (2005) also described ecosystem engineers as bioindicators of soil disturbance (e.g., by changing their abundance and diversity), soil physical quality (e.g., by creating biogenic structures), or even high soil organic matter content (e.g., by their activity incorporating litter and other organic materials into the soil profile) (Lima et al. 2011; Marques et al. 2014; Brito et al. 2016).
Although our experiment was not designed to directly test whether soil pH and soil organic carbon content affect soil macroarthropod community through changes in rhizosphere microbiome that in turns affects trophic structure and ecological processes, the results of the PCA analyses showed that herbivores (e.g., Hemiptera - Cercopidae) and ecosystem engineers (e.g., Hymenoptera - Formicidae) could be affected by changes in soil pH and soil organic carbon content. Our results agree with the findings of Amazonas et al. (2018); Machado et al. (2015) and Jinxia et al. (2010), who reported high tolerance of Hymenoptera - Formicidae of chemical inputs and changes into soil profile and positive correlation between Hemiptera and soil organic matter.
Conclusions
The green manure practice and seasonality determined soil macroarthropod community, trophic structure, and ecological processes in a sandy soil of the Brazilian semi-arid. The use of plant species of Fabaceae family (e.g., C. spectabilis and M. pruriens) showed high macroarthropod richness, Shannon’s diversity index and Simpson’s dominance index on a Regosol under field conditions. Our findings suggest that these plant species have positive effects on soil macroarthropod functional groups (e.g., predators, ecosystem engineers, decomposers, herbivores), their trophic structure and ecological processes (e.g., nutrient cycling, predation, herbivory, parasitism, and biological control). The results of our study highlight the importance of considering plant species as habitat and food resource for soil macroarthropod community, based on a sustainable way to improve soil biodiversity, ecological processes, soil quality, soil productivity and human health. Thus, long-term experiments considering green manure practice and seasonality may exploit a positive or negative feedback among plant species, soil biodiversity and soil chemical properties.
References
Alvares CA, Stape JL, Sentelhas PC, Moraes G, Leonardo J, Sparovek G (2013) Köppen’s climate classification map for Brazil. Meteorol Z 22:711–728. https://doi.org/10.1127/0941-2948/2013/0507
Amazonas NT, Viani RAG, Rego MGA, Camargo FF, Fujihara RT, Valsechi OA (2018) Soil macrofauna density and diversity across a chronosequence of tropical forest restoration in southeastern Brazil. Braz J Biol 78:449–456. https://doi.org/10.1590/15196984.169014
Anderson JN, Ingram JSI (1993) Tropical soil biology and fertility: a handbook of methods. CAB International, Wallingford
Antoniazzi R, Garro RNSL, Dáttilo W, Ribeiro SP, Neves FS (2019) Ant species richness and interactions in canopies of two distinct successional stages in a tropical dry forest. Sci Nat 106:20. https://doi.org/10.1007/s00114-019-1614-0
Balkenhol B, Haase H, Gebauer P, Lehmitz R (2018) Stteplebushes conquer the contryside: influence of invasive plant species on spider communities (Araneae) in former wet meadows. Biodivers Conserv 27:2257–2274. https://doi.org/10.1007/s10531-018-1536-8
Bento DDM, Ferreira RL, Prous X, Souza-Silva M, Bellini BC, Vasconcellos A (2016) Seasonal variations in cave invertebrate communities in the semiarid Caatinga, Brazil. J Cave Karst Stud 78:61–71. https://doi.org/10.4311/2015LSC0111
Black CA (1965) Methods of soil analysis, part 2. In: Black CA (ed) Agronomy monograph no. 9. American Society of Agronomy, Madison, pp 771–1572
Brito MF, Tsujigushi BP, Otsubo AA, Silva RF, Mercante FM (2016) Diversidade da fauna edáfica e epigeica de invertebrados em consórcio de mandioca com adubos verdes. Pesqui Agropecuária Bras 51:253–260. https://doi.org/10.1590/S0100-204X2016000300007
Costa CMQ, Silva FAB, Farias AI, Moura RC (2009) Diversidade de Scarabaeidae (Coleoptera, Scarabaeidae) coletados com armadilha de intercepção de vôo no refúgio ecológico Charles Darwin, Igarassu-PE-Brasil. Rev Bras Entomol 53:88–94. https://doi.org/10.1590/S0085-56262009000100021
Coyle DR, Nagendra UJ, Taylor MK, Campbell JH, Cunard CE, Joslin AH, Mundepi A, Phillips CA, Callaham Junior MA (2017) Soil fauna responses to natural disturbances, invasive species, and global climate change: current state of the science and a call to action. Soil Biol Biochem 110:116–133. https://doi.org/10.1016/j.soilbio.2017.03.008
Delgado-Baquerizo M, Eldridge DJ, Maestre FT, Ochoa V, Gozalo B, Reich PB, Singh BK (2018) Aridity decouples C:N:P stoichiometry across multiple trophic levels in terrestrial ecosystems. Ecosystem 21:459–468. https://doi.org/10.1007/s10021-017-0161-9
Guo H, Wang C-Z (2019) The ethological significance and olfactory detection of herbivore induced plant volatiles in interactions of plants, herbivorous insects, and parasitoids. Arthropod Plant Interac 13:161–179. https://doi.org/10.1007/s11829-019-09672-5
Jinxia L, Mei H, Xiuqin YIN, Jiliang LIU (2010) Effects of the accumulation of the rare earth elements on soil macrofauna community. J Rare Earths 28:957–964. https://doi.org/10.1016/S1002-0721(09)60233-7
Kamau S, Karanja N, Ayuke FO, Lehmann J (2019) Short-term influence of biochar and fertilizer-biochar blends on soil nutrients, fauna and maize growth. Biol Fert Soils 55:661–673. https://doi.org/10.1007/s00374-019-01381-8
Law S, Eggleton P, Griffiths H, Ashton L, Parr C (2019) Suspended dead wood decomposes slowly in the tropics, with microbial decay greater than termite decay. Ecosystems 22:1176–1188. https://doi.org/10.1007/s10021-018-0331-4
Lima SS, Aquino AM, Leite LFC, Velásquez E, Lavelle P (2011) Relação entre macrofauna edáfica e atributos químicos do solo em diferentes agroecossistemas. Pesqui Agropecuária Bras 45:322–331
Machado DL, Pereira MG, Correia MEF, Diniz AR, Menezes CEB (2015) Fauna edáfica na dinâmica sucessional da mata atlântica em floresta estacional semidecidual na bacia do rio Paraíba do Sul – RJ. Cienc Florest 25:91–106. https://doi.org/10.5902/1980509817466
Manwaring M, Wallace HM, Weaver HJ (2018) Effects of a mulch layer on the assemblage and abundance of mesostigmatan mites and other arthropods in the soil of a sugarcane agro-ecosystem in Australia. Exp Appl Acarol 74:291–300. https://doi.org/10.1007/s10493-0180227-1
Marichal R, Grimaldi M, Feijoo A, Oszwald J, Praxedes C, Cobo DHR, Hurtado MP, Desjardins T, Silva Junior ML, Costa LGS, Miranda IS, Oliveira MND, Brown GG, Tsélouiko S, Martins MB, Decaëns T, Velasquez E, Lavelle P (2014) Soil macroinvertebrate communities and ecosystem services in deforested landscapes of Amazonia. Appl Soil Ecol 83:177–185. https://doi.org/10.1016/j.apsoil.2014.05.0060929-1393
Marques DM, Silva AB, Silva LM, Moreira EA, Pinto GS (2014) Macrofauna edáfica em diferentes coberturas vegetais. Biosci J 30:1588–1597
Melo LN, Souza TAF, Santos D (2019) Cover crop farming system affect macroarthropods community diversity of Caatinga. Brazil Biologia. https://doi.org/10.2478/s11756-019-00272-5
Mishra G, Marzaioli R, Giri K, Borah R, Dutta A, Jayaraj RSC (2017) Soil quality assessment under shifting cultivation and forest in Northeasatern Himalaya of India. Arch Agron Soil Sci 63:1355–1368. https://doi.org/10.1080/03650340.2017.1281390
Moura EG, Aguiar ACF, Piedade AR, Rousseau GX (2015) Contribution of legume tree residues and macrofauna to the improvement of abiotic soil properties in the eastern Amazon. Appl Soil Ecol 86:91–99. https://doi.org/10.1016/j.apsoil.2014.10.008
Ng K, McIntyre S, Macfadyen S, Barton PS, Driscoll DA, Lindenmayer DB (2018) Dynamic effects of ground-layer plant communities on beetles in a fragmented farming landscape. Biodivers Conserv 27:2131–2153. https://doi.org/10.1007/s10531-018-1526-x
Nunes LAPL, Araújo ASF, Pessoa MMC, Sousa RS, Silva JDC, Matos-Filho CHA (2019) Edaphic fauna in a vegetation gradient in the Sete Cidades National Park. Braz J Biol 79:45–51. https://doi.org/10.1590/1519-6984.174135
Okalebo JR, Gathia KW, Woomer PL (1993) Laboratory methods of plant and soil analysis: a working manual. Technical Bulletin No 1, Soil Science Society East Africa, Nairobi
R Core Team (2018) R: a language and environment for statistical computing. R Foundation for Statistical Computing, Vienna, Austria https://www.R-project.org/
Roy S, Roy MM, Jaiswal AK, Baitha A (2018) Soil arthropods in maintaining soil health: thrust areas for sugarcane production systems. Sugar Tech 20:376–391. https://doi.org/10.1007/s12355-018-0591-5
Shakir MM, Ahmed S (2015) Seasonal abundance of soil arthropods in relation to meteorological and edaphic factors in the agroecosystems of Faisalabad, Punjab, Pakistan. Int J Biometeorol 59:605–616. https://doi.org/10.1007/s00484-014-0874-9
Shannon CE, Weaver W (1949) The mathematical theory of communication. University of Illinois Press, Urbana
Silva CF, Pereira GHA, Pereira MG, Silva AN (2013) Soil fauna in a periodically flooded Restinga salt marsh area in Marambaia, RJ. Rev Bras de Cienc Solo 37:587–595. https://doi.org/10.1590/S0100-06832013000300004
Silva RA, Siqueira GM, Costa MKL, Guedes Filho O, Silva EFF (2018) Spatial variability of soil fauna under different land use and managements. Rev Bras Cienc Solo 42:e0170121. https://doi.org/10.1590/18069657rbcs20170121
Simpson EH (1949) Measurement of diversity. Nature 163:688
Solen LC, Nicolas J, Xavier AS, Thibaud D, Simon D, Michel G, Johan O (2018) Impacts of agricultural practices and individual life characteristics on ecosystem services: a case study on family farmers in the context of an Amazonian Pioneer front. Environ Manag 61:772–785
Souza TAF, Freitas H (2017) Arbuscular mycorrhizal fungal community assembly in the Brazilian tropical seasonal dry forest. Ecol Processes 6:1–10. https://doi.org/10.1186/s13717-017-0072-x
Souza TAF, Freitas H (2018) Long-term effects of fertilization on soil organism diversity. In: Gaba S, Smith B, Lichtfouse E (eds) Sustainable agriculture reviews. Springer, Cham, pp 211–247. https://doi.org/10.1007/978-3-319-90309-5_7
Souza TAF, Rodrígues AF, Marques LF (2016) Long-term effects of alternative and conventional fertilization on macroarthropod community composition: a field study with wheat (Triticum aestivum L) cultivated on a Ferralsol. Org Agric 6:323–330. https://doi.org/10.1007/s13165-015-0138-y
Souza AVSS, Souza TAF, Santos D, Rios ES, Souza GJL (2018) Agronomic evaluation of legume cover crops for sustainable agriculture. Russ Agric Sci 44:31–38. https://doi.org/10.3103/S1068367418010093
Vasconcellos A, Andreazze R, Almeida AM, Araujo HF, Oliveira ES, Oliveira U (2010) Seasonality of insects in the semi-arid Caatinga of northeastern Brazil. Rev Bras Entomol 54:471–476. https://doi.org/10.1590/S0085-56262010000300019
Vukicevich E, Lowery T, Bower P, Úrbez-Torres JR, Hart M (2016) Cover crops to increase soil microbial diversity and mitigate decline in perennial agriculture: a review. Agron Sustain Dev 36:48. https://doi.org/10.1007/s13593-016-0385-7
Wink C, Guedes JVC, Fagundes CK, Rovedder AP (2005) Insetos edáficos como indicadores da qualidade ambiental / soil borne insects as indicators of environmental quality. Rev Cienc Agrovet 4:60–71
WRB - IUSS Working Group (2006) World Reference Base for soil. World Soil Resources Reports. Rome, FAO
Yang B, Zhang W, Xu H, Wang S, Xu X, Fan H, Chen HYH, Ruan H (2018) Effects of soil fauna on leaf litter decomposition under different land used in eastern coast of China. J For Res 29:973–982. https://doi.org/10.1007/s11676-017-0521-5
Zhang P, Neher DA, Li B, Wu J (2018) The impacts of above- and belowground plant input on soil microbiota: invasive Spartina alterniflora versus native Phragmites australis. Ecosystem 21:469–481
Acknowledgments
The authors thank the Federal University of Paraíba for the availability of area, equipment, staff, and facilities for the study. To the “Tecnológico Nacional de México” (TecNM) as well as the “Consejo Nacional de Ciencia y Tecnología” (CONACYT) for sponsoring and financing the postgraduate scholarship to the first author.
Author information
Authors and Affiliations
Contributions
We declare that all the authors made substantial contributions to the conception, design, acquisition, analysis, and interpretation of the data. All the authors participate in drafting the article, revising it critically for important intellectual content; and finally, the authors gave final approval of the version to be submitted to Biologia.
Corresponding author
Ethics declarations
Conflict of interest
The authors declare that they have no conflict of interest.
Additional information
Publisher’s note
Springer Nature remains neutral with regard to jurisdictional claims in published maps and institutional affiliations.
Electronic supplementary material
ESM 1
(DOCX 581 kb)
Rights and permissions
About this article
Cite this article
Forstall-Sosa, K.S., de Souza, T.A.F., de Oliveira Lucena, E. et al. Soil macroarthropod community and soil biological quality index in a green manure farming system of the Brazilian semi-arid. Biologia 76, 907–917 (2021). https://doi.org/10.2478/s11756-020-00602-y
Received:
Accepted:
Published:
Issue Date:
DOI: https://doi.org/10.2478/s11756-020-00602-y