Abstract
Chlor-alkali plants using mercury (Hg) cell technology are acute point sources of Hg pollution in the aquatic environment. While there have been recent efforts to reduce the use of Hg cells, some of the emitted Hg can be transformed to neurotoxic methylmercury (MeHg). Here, we aimed (i) to study the dispersion of Hg in four reservoirs located downstream of a chlor-alkali plant along the Olt River (Romania) and (ii) to track the activity of bacterial functional genes involved in Hg methylation. Total Hg (THg) concentrations in water and sediments decreased successively from the initial reservoir to downstream reservoirs. Suspended fine size particles and seston appeared to be responsible for the transport of THg into downstream reservoirs, while macrophytes reflected the local bioavailability of Hg. The concentration and proportion of MeHg were correlated with THg, but were not correlated with bacterial activity in sediments, while the abundance of hgcA transcript correlated with organic matter and Cl− concentration, indicating the importance of Hg bioavailability in sediments for Hg methylation. Our data clearly highlights the importance of considering Hg contamination as a legacy pollutant since there is a high risk of continued Hg accumulation in food webs long after Hg-cell phase out.
Similar content being viewed by others
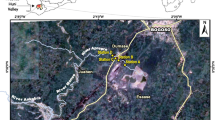
Explore related subjects
Discover the latest articles, news and stories from top researchers in related subjects.Avoid common mistakes on your manuscript.
Introduction
Mercury (Hg) is an important environmental contaminant. Although atmospheric emissions dominate Hg inputs on a global scale, the direct release of Hg from industrial processes has impacted numerous sites (Stewart et al. 2011; Bravo et al. 2014). Hg release from chlor-alkali plants is a well-recognized point source of Hg pollution in the aquatic environment. Over the last decade, the number of the chlor-alkali plants and the Hg-cell-based production were reduced. However, Hg-cell technology is still being used in 45 active plants corresponding to the half of worldwide chlorine production capacity (UNEP 2013a) and 15 % of the total global Hg consumption (UNEP 2011). According to UNEP, there are 30 chlor-alkali units in Europe alone, which represents 72 % of Hg cells worldwide and 31 % of the European chlorine production capacity (UNEP 2013a). Despite the fact that chlorine producers across Europe are progressively moving towards Hg-free technologies and have voluntary pledged to phase out all Hg-cell units by 2020 (UNEP 2013a). the ongoing use of Hg cells and the legacy effect remaining from past releases remain a major concern for affected areas. In Europe, 96 % of the Hg emissions from chlor-alkali plants are released into the environment as liquid waste with the consequent risk of altering water ecosystems (UNEP 2013a).
Emissions of Hg to the water bodies are of special concern due to the possibility of methylmercury (MeHg) formation and subsequent biomagnification in food chains, potentially affecting wildlife (Scheuhammer et al. 2007) and human health (UNEP 2013b). Over the past 10 years, we have intensively studied the impact of an active chlor-alkali plant using both Hg-cell and Hg-free units in Romania (Bravo et al. 2009, 2010, 2014; Regier et al. 2013). The Babeni Reservoir directly receiving wastewater discharges of the chlor-alkali plant in 2009 showed extremely high MeHg levels in sediments (~30 ng g−1), water (5 ng L−1), and biota (up to 10 g μg−1 dry weight in fish) with plankton and macrophytes identified as the main entry of Hg into the food chain (Table 1; Bravo et al. 2014). Particularly, a high MeHg production was observed deep in the water column and at the sediment–water interface of the Babeni Reservoir. However, the impact on downstream reservoirs (Fig. 1) and the whole river system remains unclear.
The formation of MeHg from the released inorganic Hg (IHg) in freshwater systems is influenced by a variety of environmental factors, including temperature, pH, redox potential, presence of inorganic and organic complexing agents, bioavailable Hg, and total microbial activity (Jonsson et al. 2012). The highest MeHg production occurs mostly in sediments at the oxic/anoxic transition zones during the anaerobic degradation of natural organic matter (Compeau and Bartha 1985; Gray et al. 2004; Bravo et al. 2014). Several guilds of bacteria are known to methylate IHg, notably iron-reducing bacteria (FeRB), sulfate-reducing bacteria (SRB), and methanogens (Fleming et al. 2006; Kerin et al. 2006; Gilmour et al. 2011, 2013; Hamelin et al. 2011; Yu et al. 2013). The specific mechanisms and metabolic pathways involved in Hg methylation remains undefined, but two genes in particular (cluster hgcA and hgcB) have been shown to be required for Hg methylation (Gilmour et al. 2013; Parks et al. 2013). The diversity of these genes was recently investigated in sites with distinct methylation potentials, namely the everglades, wetlands, and rice paddies (Bae et al. 2014; Liu et al. 2014; Schaefer et al. 2014). Nonetheless, our global understanding of the biotically mediated Hg methylation, and the quantitative importance of such processes, notably hgcAB cluster activity at the ecosystem level is only emerging and remains insufficient.
The aims of this study were as follows: (i) to assess the dispersion of Hg emitted by the chlor-alkali plant in downstream reservoirs of the impacted Babeni Reservoir along the Olt River, (ii) to determine the amount of Hg which is methylated to MeHg in sediments of these reservoirs, and (iii) to elucidate whether bacterial activity estimated through transcript abundance was related to the MeHg concentration and/or formation in environmental samples. We measured total Hg (THg) concentrations in the water, sediments, and biota of 4 reservoirs located downstream of the discharge channel of the chlor-alkali plant and in one upstream reservoir. Bacterial activity in sediments was assessed using quantitative PCR (qPCR) targeting transcripts known to be involved in carbon, sulfate, iron, and Hg biogeochemical cycling. In addition, we measured MeHg concentrations in sediments along with a suite of physico-chemical characteristics of the water and sediments to predict the influence of abiotic factors on microbial Hg methylation.
Materials and methods
Description of the studied area
The Olt River is a tributary of the Danube River. It takes its source in the northeast of Romania and flows to the South. The river is affected by Hg released from a chlor-alkali plant located in the industrial platform of Ramnicu Valcea (Bravo et al. 2009, 2010, 2014). The wastewater treatment plant of the industrial platform pours its effluents in the Govora River that shortly flows into the Olt River upstream of Babeni Reservoir. In the present study, Babeni Reservoir as well as the three subsequent downstream reservoirs (Ionesti, Zavideni, and Dragasani) were studied (Fig. 1). One upstream reservoir (Valcea) was used as a non-contaminated reference reservoir. Samples of water, sediments, and biota were taken during the field campaign in September 2014.
Labware and reagents
All materials were soaked twice in 10 % v/v HNO3 (pro analysis, Merck, Nyon, Switzerland) followed by 10 % v/v hydrochloric acid (HCl) (pro analysis, Merck, Nyon, Switzerland) acid baths for 1 week, thoroughly rinsed with ultrapure water (<18.2 MΩ, MilliQ Direct system, Merck Millipore, Darmstadt, Germany), and dried under a laminar flow hood.
Water, sediments, and biota sampling
Surface water was collected at 0.5-m depth with a submersed Teflon bottle, filtered immediately through a 0.45-μm membrane filter (Sterivex-HV, Millipore, Darmstadt, Germany) and acidified with HCl (Suprapur, Merck, Nyon, Switzerland) to 0.5 % v/v final concentration. Sediment cores were collected with a homemade Jenkin–Mortimer-type gravity corer and immediately brought to the laboratory. The cores were kept vertically with undisturbed overlying water from the sampling site until they were opened (within 4 h) in a glove box under N2 atmosphere to avoid oxidation of reduced species and sectioned in 0–1-, 1–3-, 3–5-cm-deep slices. Sediment aliquots were immediately flash frozen in liquid nitrogen and freeze-dried for Hg analysis or kept frozen at −80 °C for RNA extraction, granulometry, and organic matter measurements until analyzed.
All biota were collected as close as possible to coring sites. Elodea nuttallii was chosen as a representative species of macrophytes, based on its abundance in the Olt River and Hg bioaccumulation capacities (Regier et al. 2012, 2013; Cosio et al. 2014). Shoots of macrophytes were collected with a grapnel. Seston samples, consisting of all organisms bigger than the mesh size of 65 μm including plytoplankton and zooplankton, were collected using a homemade tow net. All biological samples collected in the field were kept in an icebox until they were processed in the laboratory within 4 h. Biota was separated into two subsamples; the first was rinsed in milliQ water only to determine total accumulated Hg, while the second was additionally rinsed in 1 mM cysteine (97 %, Sigma-Aldrich, Buchs, Switzerland) for 10 min and again in milliQ water to quantify tightly bound Hg concentration (e.g., intracellular).
Water sample analyses
Depth profiles of ancillary parameters (depth, temperature, specific conductivity, pH, and dissolved oxygen (DO) content) were measured in situ using a multiparameter water quality probe 600R (YSI, Yellow Springs, OH, USA). Calibration of pH and DO were performed prior to each deployment using pH 7.0 and 9.0 buffers, and water-saturated air medium, respectively.
For major cation and anion analysis, water was filtered to 0.45 μm with Sterivex-HV filters and stored at 4 °C until analyzed (less than 2 weeks) by ion chromatography (Dionex ICS-3000 Thermo Fisher Scientific Inc., Waltham, MA, USA). A certified reference material (CRM) Ontario-99 (National Water Research Institute, Environment Canada, Burlington, Ontario, Canada) was analyzed to verify the accuracy of ion measurements.
Water samples were analyzed for dissolved organic carbon (DOC) concentration using 550 °C oven-muffled glass bottles after filtration with pre-pyrolyzed 0.7-μm GF/F filters (Whatman, Zweigniederlassung, Scwitzerland) and were acidified with 2 M HCl (pro analysis, Merck, Nyon, Switzerland). DOC measurements were performed with a TOC-5000A analyzer (Shimatzu, Kyoto, Japan).
Sediment organic matter and grain size distribution
Organic matter content (OM) in sediments was estimated by loss of ignition (LOI) (Dean 1974). For total carbon and nitrogen measurements, between 7 and 10 mg of sample were packed tightly in pressed tin capsules (6 · 4 mm, Elemental Microanalysis, Devon, UK) and analyzed by high temperature catalytic oxidation using a ECS 4010 elemental analyzer (Costech Analytical Technologies, Valencia, CA, USA) and calibrated with a sulfanilamide standard (C 41.84 %, N 16.27 %, H 4.68 %, O 18.58 %, S 18.62 %).
Grain size distribution was measured using a Coulter LS-100 particle size analyzer (Beckman Coulter, Fullerton, CA, USA). The median grain size and the proportion of three major size classes (clay < 2 μm; silt 2–63 μm; and sand > 63 μm) were determined.
Mercury analysis
Total Hg (THg = IHg + MeHg) in sediments and biota was quantified by atomic absorption spectrometry (Advanced Mercury Analyzer; AMA 254, Altec, Dvůr Králové nad Labem, Czech republic). The accuracy of the measurements was checked by analyzing the CRM Mess-3 (National Research Council Canada, Ottawa, Ontario, Canada). The extraction of MeHg species from sediments was achieved with a focused microwave method (4 min, 80 °C) from 200 mg of sediment in 5 ml of 6 N HCl (Suprapur, Merck, Nyon, Switzerland) (Rodriguez Martín-Doimeadios et al. 2003). MeHg in sediment extracts was measured by capillary gas chromatography-inductively coupled plasma mass spectrometry (GC-ICPMS, Agilent Technologies 6890-7500a, Santa Clara, CA, USA) and species-specific isotope dilution (Rodriguez Martín-Doimeadios et al. 2003; Monperrus et al. 2008).
THg in water was measured by cold vapor fluorescence spectrometry with the MERX Automated Analytical System (Brooks Rand Instruments, Seattle, WA, USA) following manufacturer’s instructions and standardized protocols (USEPA 2001).
RNA extraction and reverse transcriptase quantitative polymerase chain reaction (RT-qPCR)
RNA was extracted in triplicate from homogenized sediments (between 1.5 and 2 g) with the RNA Power Soil Extraction kit (Mo-Bio, Carlsbad, CA, USA) under sterile conditions according to instructions from the manufacturer. RNA was quantified with a quBIT fluorometer (Invitrogen, Thermo Fisher Scientific, Waltham, MA USA). Its integrity (Rin between 7.0 and 10.0) was determined using an Agilent 2100 BioAnalyser (Agilent Technologies, Santa Clara, CA, USA), and its purity (A260/A280 between 1.8 and 2.0) was quantified using a spectrophotometer HeλIOS γ (Thermo Scientific, Reinach, Switzerland). Recovered RNA was treated with RNase-free DNase I (Fermentas, Thermo Fisher Scientific, Reinach, Switzerland) for 30 min at 37 ° C to remove any residual DNA. The absence of DNA was subsequently verified by performing PCR with primers targeting the 16S ribosomal RNA (rRNA) gene on all RNA samples with visualization of PCR products on a 2 % agarose gel stained with SybrSafe (Invitrogen, Thermo Fisher Scientific, Waltham, MA, USA). Then 1 μg of total RNA was used to synthetize complementary DNA (cDNA) with the M-MLV Reverse Transcriptase (Invitrogen, Thermo Fisher Scientific, Reinach, Switzerland) using oligo (dT)15 primers (Promega, Madison, WI, USA) in 20 μL total volume. RNA and cDNA were stored at −80 °C and kept on ice when in use. Primers for qPCR were chosen based on the literature, notably for their capacity to amplify a large section of the biodiversity of targeted genes (Table 2, Table S1). Nevertheless, primers for hgcA are known to amplify better the hgcA sequences from the δ-Proteobacteria over more diverse families such as firmicutes (Schaefer et al. 2014). This was done in order to target reliably previously characterized Hg methylators, e.g., Desulfovibrio and Geobacter species.
For the standard curve, cDNAs were amplified with each pair of primers and correct lengths of PCR products were verified on 2 % agarose gel. The resulting amplicons were purified, quantified, and prepared for serial dilution and stored at −80 °C until used as calibration standards for the RT-qPCR quantification. The reproducibility of qPCR was tested by performing all analysis in triplicates. qPCR for quantification of transcripts was performed, using an Eco cycler (Illumina, San Diego, CA, USA) and the Kapa SYBR Fast qPCR kit (Kapa Biosystems, Wilmington, MA, USA). All reactions were performed with 1 μL of 1/10 diluted cDNA in 20 μL final volume according to the supplier’s protocol (200 nM of each primer, enzyme activation 3 min at 95 °C, denaturation 3 s at 95 °C, annealing at 55 °C 20 s) with 40 amplification cycles and quantitative data acquired during the annealing step of each cycle. Finally, one cycle of denaturation at 95 °C for 15 s, annealing at 55 °C for 15 s, and a ramp up to 95 °C for 15 s was applied to generate the melting curve. Positive (standard samples) and no-template controls were run with each qPCR reaction and the numbers of targets per sample were calculated. The quality of standard curve and melting curves were tested with qpcR package (www.dr-spiess.de/qpcR.html; Ritz and Spiess 2008; Tichopad et al. 2003) in the freely available R software (http://www.r-project.org/). Standard curves had an amplification efficiency ranging between 1.38 and 1.89, a R2 > 0.980, and a minimum sensitivity of 103 target molecules per reaction (Table S1). In addition the absence of primer dimers or other nonspecific amplicons in these reactions were further confirmed by visualizing the PCR samples on an agarose gel.
Statistical analysis
Principal component analysis (PCA) was performed to identify patterns in response variables and individuals, as well as highlight similarities and differences between data sets. Redundancy analysis (RDA) was also used to extract and summarize the variation in the set of variables by another set of explanatory variables. Both PCA and RDA were conducted with the packages FactoMineR (http://factominer.free.fr/) and vegan (http://vegan.r-forge.r-project.org/), respectively, in freely available R software (http://www.r-project.org). Pearson’s correlation coefficients (α = 0.05) to measure the linear dependence (correlation) between variables two by two were calculated in Excel (Microsoft, Redmond, WA, USA). A Student’s t test was also used in Excel to determine if two sets of data were significantly different.
Results
Water chemistry characterization
Water temperature and pH remained close to 20 °C and 8.0, respectively, in the five studied reservoirs of the Olt River (Fig. 1). In the Govora River, a small tributary of the Olt River upstream of the Babeni Reservoir that receives Hg discharges of the chlor-alkali plant, temperature was also close to 20 °C, but pH (10.8) and conductivity (close to 12,000 μS cm−1) were much higher than in the downstream reservoirs. More in detail, conductivity in the reference reservoir of Valcea, located upstream of the wastewater outlet, was 225 μS cm−1. The impact of the discharged water was obvious in the Babeni Reservoir where conductivity was 842 μS cm−1. In further downstream reservoirs, the conductivity slowly decreased to 655 μS cm−1 in the Dragasani Reservoir (last of downstream). Throughout the water column conductivity, pH and temperature were stable with depth in all reservoirs. The concentration of dissolved oxygen (DO) was around 10 mg L−1 in the surface water of all reservoirs (Fig. 1). DO depletion was observed in Babeni’s water column, with a value of 1 mg L−1 at 10-m depth and anoxic conditions at the bottom of the reservoir. A decrease of DO with the water depth was also found in other reservoirs (Fig. 1), but the water column remained oxic until the surface of the sediments. DOC concentration was very high in the Govora River (58.4 ± 0.02 mg L−1) compared to values measured in the upstream and downstream reservoirs (2.7 to 3.8 mg L−1; Table 3). DOC was comparable in the 5 reservoirs of the Olt River studied here (Table 3). The concentrations of Na+ and Cl− were also much higher in the Govora River compare to the Valcea Reservoir with an increase of 13- and 288-fold, respectively, and were observed to also impact downstream reservoirs. SO4 2− showed a fourfold increase in the Govora River vs Valcea Reservoir, but SO4 2− concentrations were not significantly different between the studied reservoirs. However, THg concentration in water of the Govora River was ten-fold higher than that of the Valcea Reservoir, showing significant discharge of Hg-laden waste waters (Table 3). The concentration of THg in surface water of Babeni Reservoir was higher than in Valcea Reservoir. THg in water of the further downstream reservoir, Ionesti, was similar to Valcea Reservoir, while THg in water of Zavideni and Dragasani Reservoirs was lower than in the Valcea Reservoir. The above observations clearly revealed a gradual decrease of the THg concentration in downstream reservoirs. The water quality of the Govora River and in general the Olt River was thus strongly impacted by the release of the chemical platform despite the presence of a wastewater treatment plant.
Sediment characterization
The principal physico-chemical parameters of sediments collected in the deepest part of the different reservoirs are given in Table 4. LOI was similar in the different layers and reservoirs, but C/N tended to decrease downstream. Sediments of the Valcea Reservoir were composed of more sand and less clay than downstream reservoirs, but silt was the most abundant size class in all reservoirs. THg contents in sediments were higher in downstream reservoirs than in the Valcea Reservoir and increased with depth, except in the Dragasani Reservoir where the highest concentration was found at the surface of the core. MeHg concentrations in sediments were low and around 5 ng g−1 in all reservoirs except in the surface sediments of the Babeni Reservoir that reached 18 ng g−1. In this layer, MeHg proportion represented 1 % of THg, while MeHg represented 0.5 ± 0.1 % of THg in all other samples. In Valcea, Babeni, and Ionesti Reservoirs, the MeHg proportion was slightly higher in the first-cm layer, while in Zavideni and Dragasani Reservoirs, the highest MeHg proportion was found in the 1–3-cm layer. However, no obvious trend with distance from the source could be identified for MeHg in sediments. On the other hand, correlation based on Pearson’s product–moment correlation coefficient also showed significant positive correlations between MeHg concentration and proportion in surface sediments and THg in water (0.89 and 0.97 respectively), as well as negative correlations with SO4 2− concentrations in water (−0.87 and −0.86, respectively; Table S2) further supporting that Hg found in sediments originated from the water.
PCA analysis of parameters measured in sediments revealed a similar behavior of MeHg concentration with THg concentration and clay proportion (Fig. 2a), suggesting that (i) THg concentration was bound to fine grains and hence supports that THg reaches sediments through transport of suspended particles, and that (ii) in sediments, MeHg concentration was linked to THg concentration. The first two dimensions explained 71.9 % of the variability. The first dimension was driven by granulometry and Hg species concentrations, while the second one by OM content (LOI) and characteristics (C/N) (Fig. 2b). Samples from Valcea and Babeni Reservoirs were placed distantly in the PCA, indicating a significant difference in the sediment chemistry between these two reservoirs. Samples from the Ionesti Reservoir were placed close to the Babeni Reservoir, highlighting the impact of the chlor-alkali plant also in this reservoir. However, Zavideni and Dragasani were located in the PCA closer to Valcea, suggesting a lower impact on their sediments quality (Fig. 2b). Individual factor map of PCA thus showed a distribution of samples congruent with distance from the Govora River between the extremes represented by Valcea and Babeni Reservoirs and pointed to a gradual improvement of quality of sediments in downstream reservoirs (Fig. 2b).
Transcripts related to carbon, sulfate, iron, and Hg biogeochemical cycles
The expression level of representative genes measured by qPCR was used to characterize microbial activity in the sediments of different reservoirs. The global microbial activity assessed with the rRNA 16S gene showed similar bacterial activities in the sediments (Table 5). The transcript of merA, involved in Hg resistance was the most abundant functional transcript among the measured ones. The same order of dsrA (used to identify SRB) and GCS (used to identify Geobacteraceae, the genus of FeRB able to methylate Hg) activity were found, while mcrA (used to identify methanogens) was less abundant appearing close to or below detection limit in several samples (Table 5). Overall, higher gene activity was found in the surface layer of the sediments in all reservoirs. None of the studied transcripts revealed any obvious difference or trend in abundance between the reservoirs.
The first two dimensions of the PCA (Fig. 3) explained 82.43 % of the variability. Samples from different depths in the same reservoir were distributed along the second axis in the PCA and no obvious trend of position of samples from different reservoirs and distance from the source could be found, confirming the main differences between samples to be linked with depth rather than distance from the source (Fig. 3a). Microbial activity in the different reservoirs seemed to be quite homogenous for the measured transcripts. Indeed, merA, GCS and even mcrA showed a significant Pearson’s coefficient (0.80; 0.86; 0.55, Table S3) with global bacterial activity, while hgcA showed a different pattern as shown by Pearson’s coefficient (0.06; Fig. 3b, Table S3). Pearson’s correlation analysis also showed a strong correlation coefficient (0.91) between GCS and merA suggesting that, somehow, Geobacteraceae was linked to Hg resistance (Table S3).
Global analysis of parameters measured in sediments was further conducted with RDA analysis (Fig. 4), constraining environmental parameters with the transcript abundance in sediments. The first dimension was dominated by mcrA, and dsrA, while the second dimension was dominated by merA. In the RDA, sites were distributed between Babeni Reservoir and Valcea Reservoir: as in the PCA, repartition is thus congruent with distance from the pollution source. Finally, we note that the activity of hgcA appears to be correlated with dsrA in the RDA analysis, pointing to SRB as main methylators. Nevertheless, gene activity poorly explained the dimensions of the RDA, representing only 30 % of the observed variations. However, the Pearson correlation coefficient showed strong positive correlation (0.79) between rRNA 16S expression level and ratio of MeHg to THg in sediments, supporting a link between bacterial activity and Hg net methylation (Table S3). Other sediment characteristics and transcript abundance were not significantly correlated (Table S3), except hgcA abundance that was inversely correlated to LOI (−0.85) in surface sediments (0–1 cm) and to Cl− concentration in water (−0.76) (Table S2).
Hg accumulation in seston and macrophytes
The THg contents in biota representative for the base of the food chain were measured in the studied reservoirs (Table 6). THg content in the seston was significantly lower (p < 0.05) in the Valcea Reservoir as compared with Babeni, and Zavideni Reservoirs. THg concentration in seston collected in the Ionesti Reservoir was in the same order of magnitude as Valcea Reservoir but more variable. After washing with cysteine (used to remove loosely bound Hg), the seston showed a statistically significant increase of the intracellular THg contents for all downstream reservoirs vs seston sampled in the Valcea Reservoir. In general, THg concentration in seston seemed to be higher in all downstream reservoirs than in the Valcea Reservoir.
THg contents in the macrophyte Elodea nuttallii were higher in Babeni and Zavideni Reservoirs, and lower in Ionesti, and Dragasani Reservoirs as compared with the Valcea Reservoir (Table 6). Intracellular THg content measured in cysteine washed organisms was statistically higher in the macrophyte of the Babeni Reservoir, while plants of the further downstream reservoir showed similar (Zavideni Reservoir) or lower (Ionesti, Dragasani Reservoirs) THg contents.
Pearson’s coefficients showed significant correlation of THg and intracellular THg concentrations in E. nuttalii with THg concentration in water (0.80; 0.88), and MeHg concentration in sediments (0.82; 0.89), while seston did not correlate (0.33; 0.28) with those factors (Table S2). THg and intracellular THg in E. nuttalii was inversely correlated (−0.80; −0.80) to SRB activity in sediments, and inversely correlated (−0.80; −0.88) to SO4 2− concentration in water (Table S2), suggesting a link with Hg bioavailability. Moreover, THg in E. nuttallii and seston was inversely correlated (−0.78) to the sediments grain size, notably to sand (−0.83), while it was correlated with clay (0.71) suggesting that this parameter could control THg bioavailability (Table S2).
Discussion
Dispersion and fate of Hg in downstream reservoirs
The effect of the industry on Hg concentrations in water and sediments was measurable in 2014 in all downstream reservoirs (Tables 3, 4 and 6). Sediments and seston showed the most distant impact with significantly higher THg concentrations still found in the Dragasani Reservoir (last of downstream) than in the Valcea Reservoir (upstream). MeHg concentrations in sediments also decreased progressively to reach comparable values in Valcea and Dragasani Reservoirs (Table 4, Fig. 2b). THg content in water and macrophytes from Babeni Reservoir were higher than that from Valcea, while the water and macrophyte samples from the other reservoir exhibited lower or comparable THg contents, respectively. THg contents in seston and macrophytes of Babeni Reservoir were similar, but the decrease of THg content in downstream reservoirs was more pronounced for macrophytes than for seston. The different bioaccumulation trends in downstream reservoirs observed for seston and macrophytes was probably related to their ecology: seston is transported with water to downstream reservoirs, while macrophytes as sessile organisms reflected Hg concentration in water and sediments where they grow. Indeed the THg content in macrophytes correlated with THg in water and MeHg in sediments for the respective reservoirs. These results agree with previous studies showing that E. nuttallii accumulated IHg from the water column and remobilized MeHg from the sediments in shoots (Regier et al. 2012, 2013; Larras et al. 2013). The high THg in E. nuttallii sampled in the Zavideni Reservoir could thus reflect local MeHg production in sediments; for example, rhizospheric sediments are known as a hotspot for Hg methylation (Regier et al. 2012; Cosio et al. 2014). Moreover, the high proportion of intracellular Hg contents in both seston and macrophyte is of concern, since intracellular Hg is considered to be available for dietary transfer to higher trophic levels (Mason et al. 1996). Taken together, these results suggest that seston and, in some reservoirs, macrophytes might be an important source of Hg for higher trophic levels and fish. From a societal perspective, this finding is of major concern for local anglers who typically consume fish around 6 times a week (Bravo et al. 2010). Moreover, observations made with seston together with finding a correlation between increased THg and MeHg in sediments with increase in clay (PCA; Fig. 2a), further support that seston and fine suspended particles, both transported by water flow, contributed to the dispersion of THg (and certainly MeHg) from the Govora River through the Babeni Reservoir and downstream reservoirs. Nonetheless, the sequential decrease in THg contents in the water, sediments, and biota in downstream reservoirs indicated an improvement of river environmental quality with distance from the source of pollution.
Homogenous distribution of the hgcA gene expression in Olt River reservoirs
Despite the reduced impact of chlor-alkali Hg discharge on water and sediment quality with distance from the point source observed in 2014, no clear pattern in the abundance of Hg-related transcripts in sediments could be detected. Specifically, qPCR measurements of the expression level of genes revealed that global bacterial activity measured by 16S rRNA activity was similar between samples, although this observation has to be taken cautiously as 16S rRNA is known to be present as multiple copies in different organisms and its activity appears to be growth-phase dependent (Case et al. 2007). Morever, SRB and Geobacteraceae showed similar activities based on respective transcript abundance, while methanogens were less active. This finding is in agreement with previous data based on culture and the most probable number which identified SRB and FeRB in the Babeni Reservoir (Bravo et al. 2014) as well as with another study showing SRB and FeRB coexistence in sediments (Yu et al. 2012). The greatest methylation activity is often found just below the oxic/anoxic transition zone in sediments underlying oxygenated water (Watras et al. 1995; Ullrich et al. 2001; Bravo et al. 2014). where both sulfate and iron reduction rates can be high. Effectively, the hgcA transcript abundance was correlated to SRB activity as evidenced by RDA, supporting a role of SRB for Hg methylation in these sediments. The pair of primers used in the present study targeting drsA transcript do not necessarily hit all known Hg methylators in SRBs, however, support the existence of a link between sulfate reducing conditions and the activity of the Hg methylation- related gene as previously shown for periphyton (Acha et al. 2011). No correlation between the activity of the hgcA gene and MeHg content in sediments (or the ratio between MeHg and THg) was found. The bacterial ability to methylate Hg has been shown to depend on the presence of the hgcAB gene cluster in the laboratory (Gilmour et al. 2013; Parks et al. 2013), but the fact that the concentration of MeHg in environmental samples is the net result of (i) formation (Hg methylation), (ii) degradation (MeHg demethylation), and (iii) Hg2+ reduction to Hg0 could explain the lack of obvious correlation between the gene expression level and the MeHg concentration. However, this observation was also in agreement with a recent laboratory study showing a poor correlation of hgcAB expression level with net Hg methylation (Goni-Urriza et al. 2015). Furthermore, in the present study, the abundance of the hgcA transcript correlated with the OM in the surface sediments (0–1 cm), suggesting that the expression of this gene depends rather on OM available for the activity of the heterotrophic Hg-methylating bacteria. Moreover, hgcA was inversely correlated with Cl− concentration in water. As Cl− concentration is expected to affect Hg speciation and thus bioavailability in water (Morel et al. 1998; Ullrich et al. 2001) this could suggest a link between the expression level of the methylation gene hgcA and Hg bioavailability to bacteria, as also supported by the correlation found here between THg and MeHg in sediments (Fig. 2a). In addition, we observed a correlation between the GCS gene (from the FeRB Hg methylator Geobacter spp.) and the merA gene (involved in Hg reduction) indicating the coexistence of these two processes in sediments of the Olt River and emphasizes the complexity of microbial communities involved in Hg biogeochemical cycling. Overall, these observations highlight the difficulty of linking MeHg concentration or proportion to bacterial activities in the field, pointing the limits of the current understanding of the Hg methylation mechanisms and the existing research gaps such as Hg bioavailability to bacteria in anoxic sediments, and biochemical pathway of MeHg production and degradation, particularly in the field settings (Hsu-Kim et al. 2013).
Environmental implications of phasing out Hg technology in chlor-alkalis
The present study demonstrated a decrease of THg concentrations in the Govora River and Babeni Reservoir in water sampled in September 2014 (Table 3) as compared with those measured during a field campaign in 2009 (Table 1; Bravo et al. 2014). The decrease of the Hg release by the chlor-alkali plant was also evidenced by THg contents measured in the sediments (Table 4), the seston, and the macrophytes sampled in 2014 (Table 6), which were 0.7-fold and fivefold lower than those measured in 2009, respectively (Table 1). These observations showed that the reduced production of the chlor-alkali plant due to financial crisis lead to a significant reduction of Hg contamination level in the Olt River. Nonetheless, the particularly high MeHg concentration found in the Babeni Reservoir in 2014 (Table 4) is comparable and in good agreement with that measured during the 2009 summer campaign (Table 1, Bravo et al. 2014) and highlights the long-term legacy impact of the chlor-alkali plant discharge. Moreover, THg concentration in the upstream Valcea Reservoir in water and sediments increased six- and seven-fold, respectively, as compared with 2009 (Tables 1 and 4), suggesting a possible novel contamination source of Hg such as contaminated material dump (http://www.oltchim.ro/).
The results of the present study show persistent Hg contamination of sediments in the Babeni Reservoir receiving chlor-alkali discharge via Govora River and continued Hg dispersion in four downstream reservoirs. More specifically, the decrease of chlorine production and/or the phase out of Hg technology in the studied chlor-alkali plant and associated reservoirs resulted in a reduction of THg concentrations in the water, sediments, and primary producers. We can expect that with the complete termination of Hg release, Hg concentration in water would eventually approach to background levels, and Hg accumulated in surface sediments would be quickly buried, due to the quite high sedimentation rate in the Olt River (6 cm y−1 in the Babeni Reservoir; Bravo et al. 2009). Nevertheless, the burial of Hg released from chlor-alkali plant in the other impacted reservoirs having low sedimentation rates might be a longer process than here and does not necessarily eliminate the risk of food chain contamination by Hg. For example, in the infamous Minamata Bay (Japan), in which no river flows in, the highest THg concentrations in sediments as well as highest Hg methylation concentration were reported at 10- and 35-cm depth, although Hg discharges have been discontinued for over 30 years (Tomiyasu et al. 2006, 2014). Moreover, Hg concentrations in mussels were also reported to be higher than background concentration, which demonstrate that sediments remain a source of MeHg for several decades (Tomiyasu et al. 2006). However, in such a context of the Olt River, it is of the utmost importance to avoid dredging in the future and prevent the possible releases of Hg from the sediments. Moreover discharge channels of the chlor-alkali plants themselves, as well as erosion of contaminated soils around the chlor-alkali plant, might still be an important source of Hg to the Olt River and should continue to be monitored, along with Hg levels in biota in the coming years and decades.
Conclusions
Total Hg concentrations in the water, sediments, seston, and macrophytes decreased notably since the phase out of Hg cells and/or reduced production of the studied chlor-alkali plant. Moreover, distance from the source resulted in decreased impact in the water, sediments, and biota in downstream reservoirs, although the risk of MeHg biomagnification in food remains a realistic threat to human health for local anglers in particular. In summary, this study clearly demonstrates the importance of continuing to assess Hg cycling in all compartments of aquatic ecosystems that have historically been exposed to point source inputs of Hg from industrial sources. In particular, dredging and remobilization of Hg should be avoided in the future.
References
Acha D, Hintelmann H, Yee J (2011) Importance of sulfate reducing bacteria in mercury methylation and demethylation in periphyton from Bolivian Amazon region. Chemosphere 82:911–916
Bae H-S, Dierberg FE, Ogram A (2014) Syntrophs dominate sequences associated with the mercury-methylating gene hgcA in the water conservation areas of the Florida Everglades. Appl Environ Microbiol 80:6517–6526
Ben-Dov E, Brenner A, Kushmaro A (2007) Quantification of sulfate-reducing bacteria in industrial wastewater, by real-time polymerase chain reaction (PCR) using dsrA and apsA genes. Microb Ecol 54:439–451
Bond DR, Mester T, Nesbo CL, Izquierdo-Lopez AV, Collart FL, Lovley DR (2005) Characterization of citrate synthase from Geobacter sulfurreducens and evidence for a family of citrate synthases similar to those of eukaryotes throughout the Geobacteraceae. Appl Environ Microbiol 71:3858–3865
Bravo AG, Loizeau J-L, Ancey L, Ungureanu VG, Dominik J (2009) Historical record of mercury contamination in sediments from the Babeni Reservoir in the Olt River, Romania. Environ Sci Pollut Res 16:66–75
Bravo AG, Loizeau JL, Bouchet S, Richard A, Rubin JF, Ungureanu VG, Amouroux D, Dominik J (2010) Mercury human exposure through fish consumption in a reservoir contaminated by a chlor-alkali plant: Babeni Reservoir (Romania). Environ Sci Pollut Res 17:1422–1432
Bravo AG, Cosio C, Amouroux D, Zopfi J, Chevalley P-A, Spangenberg JE, Ungureanu V-G, Dominik J (2014) Extremely elevated methyl mercury levels in water, sediment and organisms in a Romanian reservoir affected by release of mercury from a chlor-alkali plant. Water Res 49:391–405
Case RJ, Boucher Y, Dahllof I, Holmstrom C, Doolittle WF, Kjelleberg S (2007) Use of 16S rRNA and rpoB genes as molecular markers for microbial ecology studies. Appl Environ Microbiol 73:278–288
Compeau GC, Bartha R (1985) Sulfate-reducing bacteria : principal methylators of mercury in anoxic estuarine sediment. Appl Environ Microbiol 50:498–502
Cosio C, Fluck R, Regier N, Slaveykova VI (2014) Effects of macrophytes on the fate of mercury in aquatic systems. Environ Toxicol Chem 24:1225–1237
Dean WE (1974) Determination of carbonate and organic matter in calcareous sediments and sedimentary rocks by loss on ignition; comparison with other methods. J Sediment Res 44:242–248
el Fantroussi S, Verschuere L, Verstraete W, Top EM (1999) Effect of phenylurea herbicides on soil microbial communities estimated by analysis of 16S rRNA gene fingerprints and community-level physiological profiles. Appl Environ Microbiol 65:982–988
Fleming EJ, Mack EE, Green PG, Nelson DC (2006) Mercury methylation from unexpected sources: molybdate-inhibited freshwater sediments and an iron-reducing bacterium. Appl Environ Microbiol 72:457–464
Gilmour CC, Elias DA, Kucken AM, Brown SD, Palumbo AV, Schadt CW, Wall JD (2011) Sulfate-reducing bacterium Desulfovibrio desulfuricans ND132 as a model for understanding bacterial mercury methylation. Appl Environ Microbiol 77:3938–3951
Gilmour CC, Podar M, Bullock AL, Graham AM, Brown SD, Somenahally AC, Johs A, Hurt RA Jr, Bailey KL, Elias DA (2013) Mercury methylation by novel microorganisms from new environments. Environ Sci Technol 47:11810–11820
Goni-Urriza M, Corsellis Y, Lanceleur L, Tessier E, Gury J, Monperrus M, Guyoneaud R (2015) Relationships between bacterial energetic metabolism, mercury methylation potential, and hgcA/hgcB gene expression in Desulfovibrio dechloroacetivorans BerOc1. Environ Sci Pollut Res Int (in press)
Gray JE, Hines ME, Higueras PL, Adatto I, Lasorsa BK (2004) Mercury speciation and microbial transformations in mine wastes, stream sediments, and surface waters at the Almadén Mining District, Spain. Environ Sci Technol 38:4285–4292
Hamelin S, Amyot M, Barkay T, Wang YP, Planas D (2011) Methanogens: principal methylators of mercury in lake periphyton. Environ Sci Technol 45:7693–7700
Hsu-Kim H, Kucharzyk KH, Zhang T, Deshusses MA (2013) Mechanisms regulating mercury bioavailability for methylating microorganisms in the aquatic environment: a critical review. Environ Sci Technol 47:2441–2456
Jonsson S, Skyllberg U, Nilsson MB, Westlund P-O, Shchukarev A, Lundberg E, Bjo E (2012) Mercury methylation rates for geochemically relevant HgII species in sediments. Environ Sci Technol 21:11653–11659
Kerin EJ, Gilmour CC, Roden E, Suzuki MT, Coates JD, Mason RP (2006) Mercury methylation by dissimilatory iron-reducing bacteria. Appl Environ Microbiol 72:7919–7921
Larras F, Regier N, Planchon S, Poté J, Renaut J, Cosio C (2013) Physiological and proteomic changes suggest an important role of cell walls in the high tolerance to metals of Elodea nuttallii. J Hazard Mater 263:575–583
Liu Y-R, Yu R-Q, Zheng Y-M, He J-Z (2014) Analysis of the microbial community structure by monitoring an Hg methylation gene (hgcA) in paddy soils along an Hg gradient. Appl Environ Microbiol 80:2874–2879
Mason RP, Reinfelder JR, Morel FMM (1996) Uptake, toxicity, and trophic transfer of mercury in a coastal diatom. Environ Sci Technol 30:1835–1845
Monperrus M, Gonzalez PR, Amouroux D, Alonso JIG, Donard OFX (2008) Evaluating the potential and limitations of double-spiking species-specific isotope dilution analysis for the accurate quantification of mercury species in different environmental matrices. Anal Bioanal Chem 390:655–666
Morel FMM, Kraepiel AML, Amyot M (1998) The chemical cycle and bioaccumulation of mercury. Annu Rev Ecol Syst 29:543–566
Parks JM, Johs A, Podar M, Bridou R, Hurt RA, Smith SD, Tomanicek SJ, Qian Y, Brown SD, Brandt CC, Palumbo AV, Smith JC, Wall JD, Elias DA, Liang L (2013) The genetic basis for bacterial mercury methylation. Science 339:1332–1335
Regier N, Frey B, Converse B, Roden E, Grosse-Honebrick A, Bravo AG, Cosio C (2012) Elodea nuttallii roots effect on bacterial communities and MMHg proportion in a Hg polluted sediment. PLoS ONE 7:e45565
Regier N, Larras F, Bravo AG, Ungereanu VG, Cosio C (2013) Hg bioaccumulation in the macrophyte Elodea nuttallii in the field and in microcosm: Hg in shoots accumulated from the water might involve Cu transporters. Chemosphere 90:595–602
Ritz C, Spiess A-N (2008) qpcR: an R package for sigmoidal model selection in quantitative real-time polymerase chain reaction analysis. Bioinformatics 24:1549–1551
Rodriguez Martín-Doimeadios RC, Monperrus M, Krupp E, Amouroux D, Donard OFX (2003) Using speciated isotope dilution with GC-inductively coupled plasma MS to determine and unravel the artificial formation of monomethylmercury in certified reference sediments. Anal Chem 75:3202–3211
Schaefer JK, Yagi J, Reinfelder JR, Cardona T, Ellickson KM, Tel-Or S, Barkay T (2004) Role of the bacterial organomercury lyase (MerB) in controlling methylmercury accumulation in mercury-contaminated natural waters. Environ Sci Technol 38:4304–4311
Schaefer JK, Kronberg R-M, Morel FMM, Skyllberg U (2014) Detection of a key Hg methylation gene, hgcA, in wetland soils. Environ Microbiol Rep 6:441–447
Scheuhammer AM, Meyer MW, Sandheinrich MB, Murray MW (2007) Effects of environmental methylmercury on the health of wild birds, mammals, and fish. Ambio 36:12–18
Steinberg LM, Regan JM (2008) Phylogenetic comparison of the methanogenic communities from an acidic, oligotrophic fen and an anaerobic digester treating municipal wastewater sludge. Appl Environ Microbiol 74:6663–6671
Stewart AJ, Smith JG, Loar JM (2011) Long-term water-quality changes in east fork poplar creek, Tennessee: background, trends, and potential biological consequences. Environ Manage 47:1021–1032
Tichopad A, Dilger M, Schwarz G, Pfaffl MW (2003) Standardized determination of real-time PCR efficiency from a single reaction set-up. Nucleic Acids Res 31:e122
Tomiyasu T, Matsuyama A, Eguchi T, Fuchigami Y, Oki K, Horvat M, Rajar R, Akagi H (2006) Spatial variations of mercury in sediment of Minamata Bay, Japan. Sci Total Environ 368:283–290
Tomiyasu T, Takenaka S, Noguchi Y, Kodamatani H, Matsuyamab A, Oki K, Kono Y, Kanzaki R, Akagi H (2014) Estimation of the residual total mercury in marine sediments of Minamata Bay after a pollution prevention project. Mar Chem 159:19–24
Ullrich SM, Tanton TW, Abdrashitova SA (2001) Mercury in the aquatic environment: a review of factors affecting methylation. Crit Rev Environ Sci Technol 31:241–293
UNEP (2011) INC2 briefing paper series. Mercury in chlor-alkali plants. http://www.unep.org/chemicalsandwaste/Portals/9/Mercury/Documents/INC2/ZMWG6_Chlor_20alkali_FS0111_04.pdf. Accessed 09.06.2015.
UNEP (2013a) Global estimate of global mercury cell chlorine capacity, world chlorine report to UNEP on chlor-alkali partnership data 2011 and 2012. http://www.unep.org/chemicalsandwaste/Metals/GlobalMercuryPartnership/Chlor-alkaliSector/Reports/tabid/4495/Default.aspx. Accessed 09.06.2015.
UNEP (2013b Global mercury assessment: sources, emissions, releases and environmental transport. http://www.unep.org/PDF/PressReleases/GlobalMercuryAssessment2013.pdf. Accessed 09.06.2015.
USEPA (2001) Method 1630: methyl mercury in water by distillation, aqueous ethlylation, purge and trap, and CVAFS. US Environmental Protection Agency, Office of Water, draft
Watras CJ, Morrison KA, Host JS, Bloom NS (1995) Concentration of mercury species in relationship to other site-specific factors in the surface waters of northern Wisconsin lakes. Limnol Oceanogr 40:556–565
Yu RQ, Flanders JR, Mack EE, Turner R, Mirza MB, Barkay T (2012) Contribution of coexisting sulfate and iron reducing bacteria to methylmercury production in freshwater river sediments. Environ Sci Technol 46:2684–2691
Yu RQ, Reinfelder JR, Hines ME, Barkay T (2013) Mercury methylation by the methanogen Methanospirillum hungatei. Appl Environ Microbiol 79:6325–6330
Acknowledgments
This work is part of the Romanian–Swiss Research Program with the project number IZERZO-142228, funded by the Swiss National Science Foundation (SNF) and Romanian Executive Agency for Higher Education, Research, Development and Innovation Funding (UEFISCDI). The work was also partially supported by the SNF (project 205321_138254) and Swedish Research Council (project 623-2011-7191). We thank the National Administration Apele Romane Olt for permission to work in their laboratory, GeoEcoMar for providing the boat, Aline Freiburghaus and Dany Dumitru Grosu for support and help during the sampling, Dolly Kothawala for English editing and improvement.
Author information
Authors and Affiliations
Corresponding author
Additional information
Responsible editor: Philippe Garrigues
Electronic supplementary material
Below is the link to the electronic supplementary material.
Table S1
Sequences and characteristics of primers used to analyze the microbial activity by RT-qPCR. (DOCX 29 kb)
Table S2
Pearson correlation coefficients (α = 0.05) between water surface sediments, transcripts’ abundance, and biota sampled in several reservoirs along the Olt River (Romania) during field campaign in September 2014. Coefficients higher than 0.75 are shown in bold. (XLSX 18 kb)
Table S3
Pearson correlation coefficient (α = 0.05) between sediments and transcripts’ abundance in sediments sampled in several reservoirs along the Olt River (Romania) during field campaign in September 2014. Coefficients higher than 0.75 are shown in bold. (DOCX 19 kb)
Rights and permissions
About this article
Cite this article
Bravo, A.G., Loizeau, JL., Dranguet, P. et al. Persistent Hg contamination and occurrence of Hg-methylating transcript (hgcA) downstream of a chlor-alkali plant in the Olt River (Romania). Environ Sci Pollut Res 23, 10529–10541 (2016). https://doi.org/10.1007/s11356-015-5906-4
Received:
Accepted:
Published:
Issue Date:
DOI: https://doi.org/10.1007/s11356-015-5906-4