Abstract
A simple, rapid response time and ultrahigh sensitive chemiluminescence (CL) DNA assay based on Fe3O4@SiO2@Au-functionalized magnetic nanoparticles (Au-MNPs) was developed for detection of p53 tumor suppressor gene. In this study, 2′,6′-dimethylcarbonylphenyl-10-sulfopropyl acridinium-9-carboxylate 4′-NHS ester (NSP-DMAE-NHS), as a new kind of highly efficient luminescence reagent, was immobilized on the complementary sequence of the wild-type p53 (ssDNA) to improve the detection sensitivity. The optimal concentration of ssDNA-(NSP-DMAE-NHS) conjugates mixed with the wild-type p53 (wtp53) samples respectively. Then, the wtp53-Au-MNPs conjugates were added to continue the competitive reaction in the above solution. Subsequently, the Au-MNPs separated under magnetic field, measured by a homemade luminescent measurement system. Under optimal conditions, the method exhibited ultrasensitive sensitivity with a detection limit of 0.001 ng mL−1 (0.16 pM), a wide range of liner response from 0.001 ng mL−1~6.6 μg mL−1. Therefore, the immunomagnetic nanocomposites-based detection strategy was rapid, low-cost, and highly sensitive that can be easily extended to the early diagnosis of cancer development and monitoring of patient therapy.
Similar content being viewed by others
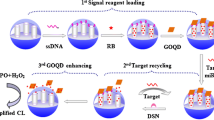
Avoid common mistakes on your manuscript.
Introduction
The p53 tumor suppressor gene, well known as “the guardian of the genome,” plays important roles in maintenance of genome integrity [1]. While, it is the most commonly mutated gene during cell stress (e.g., DNA damage induced by UV rays) in human tumors and has considerable impact on the “success” of the carcinogenic process (increasing the chances of a tumor cell surviving) [2, 3]. It is estimated that approximately 50% of all human cancers owing to DNA damage by dysfunction of genes involved in controlling the cell cycle, DNA repair, and apoptosis [4, 5]. Therefore, it is essential to understand the molecular basis of p53 inactivation in cancer; a sensitive and rapid detection of p53 gene is required for the early diagnosis and prompt operation.
Thus far, various analytical methods were employed for the determination of p53 gene including reverse transcription polymerase chain reaction (RT-PCR) [6, 7], the denaturing high-performance liquid chromatography (DHPLC) [8], the single-strand conformation polymorphism (SSCP) [9], the denaturing gradient gel electrophoresis (DGGE) [10], traditional nucleic acid probe [11], immunostaining [12], and immunohistochemistry [13, 14]. However, these traditional methods are time consuming for long incubation periods, less sensitive, and multiple steps. As an alternative, other techniques have been exhibited including matrix of polydimethylsiloxane (PDMS)-assisted bead [15], surface plasmon resonance (SPR) [16, 17], field-effect transistor biosensor [18, 19], and surface-enhanced Raman scattering [20]. Nevertheless, these methods typically require sample pre-treatment, skilled operators, and expensive instruments for high sensitivity. Two common methods, the electrochemical biosensor technology based on platforms [21, 22] and p53 antibody-based enzyme-linked immunosorbent assay (ELISA) [23,24,25], were employed later. The former is simple but still requires the sophisticated electrode materials, whereas the latter is sensitive but requires the use of p53 antibody and measures only the total p53.
Currently, chemiluminescence (CL) is one of the fast-growing analytical methods that have attracted the attention owing to enhancing emission intensity and improving selectivity for quantitative analysis [26]. Generally, the luminol or ABEI [N-(4-aminobutyl)-N-(ethylisoluminol)] as a luminescence reagent has been exploited to catalyze redox in the current CL reactions [26,27,28,29]. As an alternative, 2′,6′-dimethylcarbonylphenyl-10-sulfopropyl acridinium-9-carboxylate 4′-NHS ester (NSP-DMAE-NHS) demonstrates much more effective than the traditional chemiluminescence reagent such as luminol [30] and exhibits the high performance in the dilute alkaline hydrogen peroxide [31]. Meanwhile, the ability of coupling biomolecules by immobilization can improve the performance of biosensors and amplifying signal with functionalized magnetic nanoparticles (MNPs) as a carrier material [32]. MNPs have excellent abilities for biomolecule (proteins, DNA) immobilization due to their non-toxic nature, large surface-to-volume ratio, and biocompatibility. Moreover, they can also be easily separated from the sample matrix without damaging the biochemical activity of biomolecules.
To the best of our knowledge, 2′,6′-dimethylcarbonylphenyl-10-sulfopropyl acridinium-9-carboxylate 4′-NHS ester (NSP-DMAE-NHS) as an efficient luminescence reagent, this is the first report sensitive detection of the p53 gene based on the Fe3O4@SiO2@Au magnetic nanoparticles. In this paper, a simple and ultrasensitive competitive chemiluminescence DNA assay based on Au-MNPs was developed for detection of the p53 gene. The wild-type p53 (wtp53) was immobilized on the surface of Au-MNPs through a series of chemical reaction. NSP-DMAE-NHS was immobilized on the complementary sequence of the wild-type p53 (ssDNA). Then, the optimal concentration of ssDNA-NSP-DMAE-NHS conjugates mixed with the wild-type p53 (wtp53) samples respectively. Subsequently, the wtp53-Au-MNPs conjugates were added to continue the competitive reaction in the above solution. Finally, Au-MNPs were washed and separated under the magnetic field, and measured by a luminescent measurement system. The principle of chemiluminescence DNA assay detection based on Au-MNPs is schematically shown in Fig. 1.
Experimental
Materials and Reagents
The DNA oligonucleotides were obtained from Sangon Biotechology Inc. (China). Wild-type p53 (wtp53): 5′-NH2-(CH2)6-GGCACAAACACGCACCTCAA-3′, mutant-type p53 (mtp53): 5′-GGCACAAACATGCACCTCAA-3′, complementary sequence of target ssDNA: 5′-NH2-(CH2)6-TT GAG GTG CGT GTT TGT GCC-3′, three-base mismatched sequence of p53 DNA: 5′-GGCTCAAAGACGCACCACAA-3′. l-lysine and Sephadex G-25 were purchased from Seebio (Shanghai, China). Bovine serum albumin (BSA), 11-mercaptoundecanoic acid (MUA), 2-(N-morpholino)-ethanesulfonic acid (MES), and 3-aminopropyltriethoxysilane (APTES) were purchased from J&K Scientific Ltd. N-hydroxysuccinimide (NHS), 1-ethyl-3-(3-dimethylamino-propyl)-carbodiimidehydrochloride (EDC), and N,N-dimethylformamide (DMF) were purchased from Sigma Company (China). 2′,6′-Dimethylcarbonylphenyl-10-sulfopropyl acridinium-9-carboxylate 4′-NHS ester (NSP-DMAE-NHS) was purchased from MaterWin New Materials Co., Ltd. (Shanghai, China). FeCl3·6H2O, tetraethoxysilane (TEOS), sodium acetate, sodium chloride, sodium phosphate monobasic (NaH2PO4), sodium phosphate dibasic (Na2HPO4), methanol, ethanol, ethylene glycol, HCl, NaOH, and aqueous ammonia (28 wt%) were of analytical grade and purchased from Tianjin Tianli Chemical Regents Ltd. All the chemicals were used without further treatment. Deionized water was used for all the experiments.
Synthesis of Fe3O4@SiO2@ Au Magnetic Nanoparticles
Magnetic nanoparticles (MNPs) were prepared by solvothermal synthesis reaction [33] with some modifications. Briefly, FeCl3·6H2O (1.35 g) was dissolved in ethylene glycol (40 mL) to form a clear solution, followed by polyethylene glycol (1.0 g) and sodium acetate (3.6 g) as a stabilizing agent. The mixture was stirred vigorously for 30 min and then sealed in an autoclave (100-mL capacity). The autoclave was heated to 180 °C for 12 h and allowed to cool to room temperature (RT). The black products were washed three times with deionized water and three times with ethanol, and dried at 80 °C in vacuum for 2 h.
The above-prepared MNPs were stabilized by coating a layer of silica on the surface according to the Stober process [34]. Namely, dried MNPs (110 mg) were dispersed in 120 mL ethanol, followed by 30 mL deionized water, 2.4 mL ammonia, and 1 mL TEOS. The mixture solution was stirred at room temperature for 12 h. The silica-coated MNPs were washed and dried in the same way with the prepared MNPs.
Gold colloids with 20-nm size were prepared by reduction of HAuCl4 with sodium citrate. Then, 30 mL of the abovementioned treated Fe3O4@SiO2 nanoparticles was added dropwise to 100 mL of the citrate-stabilized gold colloids. The products were formed after 1 h, which were separated under the magnetic field and washed with deionized water (Fig. 2).
Preparation of NSP-DMAE-NHS-Labeled Complementary Sequence of Target
The ssDNA-NSP-DMAE-NHS conjugates were prepared according to related reference with some modifications [35]. Purified complementary sequence of target (ssDNA) (100 μM dissolved in 200 μL of PBS, 0.1 M, pH 8.0) was labeled with NSP-DMAE-NHS (1 mg NSP-DMAE-NHS dissolved in 316 μL of dry DMF) (Fig. S1). The reaction mixture was shaken for 1 h at RT and quenched by 100 μL of l-lysine (10 mg mL−1 of sterile distilled water) for 15 min. The labeled conjugates were separated from unbound NSP-DMAE-NHS by gel filtration on a Sephadex G-25 column (1 × 25 cm) equilibrated with PBS (0.1 M, pH 7.4). The peak nearby 8 mL demonstrated that the complementary sequence of target (ssDNA) has been labeled by NSP-DMAE-NHS. The conjugates have a strong absorption peak at 260 nm, and also a strong chemiluminescence peak. The results are shown in Fig. 3. It can be seen that the NSP-DMAE-NHS-labeled ssDNA eluted nearby volume 8 mL and collected in single-dose vials, enriched, and stored at 4 °C.
The above ssDNA-NSP-DMAE-NHS conjugates solution was serially diluted from 1:10 to 1:10−5 in the purification buffer to determine the sensitivity. Then, the dilute ssDNA-NSP-DMAE-NHS conjugates were measured with a homemade luminescent measurement system. The trigger solution was injected into each well tube through a peristaltic pump and well at 2 s. The number of photons detected in unit time by the detector and its intensity were counted. The signals were monitored and handled in real time by a computer. Figure 4a showed the moles of complementary sequence of target (ssDNA) were plotted against the emitted relative light unit (RLU). Figure 4b showed the change of chemiluminescent intensity over time. The sensitivity was determined to be at least 5 × 10−17 mol.
Immobilization of Wild-Type p53 via BSA on Au-MNPs
Fe3O4@SiO2@Au magnetite nanoparticles were dispersed in 5 mL MUA (5 mM) ethanol solution for 16 h at room temperature. The carboxyl-Au-MNPs were formed and washed twice with 2-(N-morpholino)-ethanesulfonic acid (MES) buffer solution. The carboxyl-Au-MNPs were activated by incubation with 100 μL of a 1:1 ratio mixture of EDC (200 mM) and NHS (50 mM) in MES buffer at 37 °C for 1 h. Then, the activated carboxyl-Au-MNPs were washed three times with PBS (0.01 M, pH 7.4) in a magnetic field and mixed with 100 μL BSA (0.1 mg mL−1 in deionized water) with gentle stirring for 2 h. The obtained Au-MNPs-BSA conjugates were separated under the magnetic field [36, 37].
The Au-MNPs-BSA-wtp53 conjugates were prepared as follows: the above obtained Au-MNPs-BSA were dissolved in 2 mL PBS (0.01 M, pH 7.4) with adding 4.6 mg of N-hydroxysuccinimide (NHS) and 7.7 mg of 1-(3-dimethylamino-propyl)-3-ethylcarbodiimidehydrochloride (EDC). The solution was stirred for 30 min at 37 °C. Then, wild-type p53 (wtp53) were added and stirred for another 2 h. Subsequently, the remaining carboxyl group was blocked with ethanolamine (1 M, pH 8.5) and stirred for 30 min. Finally, the Au-MNPs-BSA-wtp53 conjugates were separated with magnet.
Establishment of CLIA Method Based on Au-MNPs for Wild-Type p53 Detection
The process of reaction is shown in Fig. 1b. Wild-type p53 was dissolved in buffer solution to prepare the standard samples in which the concentrations of the wild-type p53 were 0.001, 0.0066, 0.066, 0.66, 6.6, 66, 660, and 6600 ng mL−1 respectively. The above solutions were mixed with the optimized amount of ssDNA-NSP-DMAE-NHS conjugates, which incubated at 37 °C for 1 h. Then, the mixture reacted with 100 μL of wild-type p53 immobilized Au-MNPs-BSA at 37 °C for 1 h with gentle shaking. Finally, the particles were washed by PBS in a magnetic field. Here, HNO3 + H2O2 and NaOH acted as the pre-trigger and trigger respectively. A volume of 100 μL of the pre-trigger was previously added to the tube containing Au-MNPs and 100 μL of trigger was injected into the tube by a peristaltic pump. At the same time, the chemiluminescence emission intensity was measured versus time by the luminescent measurement system (Fig. S2).
Results and Discussion
Determination of the Optimized Amount of NSP-DMAE-NHS-Labeled ssDNA
Before construction of standard curves for different concentrations of wild-type p53 (wtp53), the amount of NSP-DMAE-NHS-labeled ssDNA should be optimized. Different amounts (1, 5, 10, 20, 35, 40, 50, 60 μL) of ssDNA-NSP-DMAE-NHS conjugates were bound on Au-MNPs-BSA-wtp53 conjugates. Figure 5 showed the chemiluminescence intensity corresponding to different amounts of ssDNA-NSP-DMAE-NHS conjugates. The concentration of ssDNA-NSP-DMAE-NHS was 0.01 mg mL−1. It is clear that the chemiluminescence intensity was proportional to the amount of ssDNA-NSP-DMAE-NHS in the range of 1–50 μL and reached saturation value at 50 μL. However, it is interesting to note that signals showed a drop when the ssDNA-NSP-DMAE-NHS conjugates were beyond 50 μL. This extraordinary result may be explained by steric effect. Simply, the steric effect could influence the reaction between the ssDNA-NSP-DMAE-NHS and Au-MNPs-BSA-wtp53 conjugates. Once the amount of ssDNA-NSP-DMAE-NHS conjugates was beyond 50 μL, the mass of them affected each other to generate conformational changes, which was unfavorable to make the antibodies recognize antigens. To ensure higher sensitivity, 25-μL ssDNA-NSP-DMAE-NHS conjugates were chosen as the optimal volume for the competitive reaction, which is the 50% inhibition concentration (IC50).
Calibration Curve for Wild-Type p53 Analytes
Different concentrations of wild-type p53 (0.001, 0.0066, 0.066, 0.66, 6.6, 66, 660, 6600 ng mL−1) were mixed with 25-μL ssDNA-NSP-DMAE-NHS solution and the mixture were incubated at 37 °C for 1 h. Then, 100 μL of the Au-MNPs-BSA-wtp53 conjugates were added and stirred for another 30 min at 37 °C. After the reaction was complete, the particles were washed three times with PBS buffer (containing 0.1% Tween 20). In Fig. 6a, it can be seen that chemiluminescence intensities were linearly decreased according to the increases of wild-type p53 concentrations. That is due to the competitive immune binding of the analyte and tracer to the immobilized antibodies. Figure 6b showed the calibration curve of wild-type p53 detection. The peak of photon count was found to be linear with the logarithm of wtp53 concentration in the range from 0.001 to 100 ng mL−1. The equation for the resulting calibration plot was y = 85,445.6–21,831.5x (x was the concentration of wtp53, y was the photon count); correlation coefficient r2 was 0.990. The limit of detection (LOD) was 0.001 ng mL−1 (0.16 pM), which was estimated using 3σ (where σ is the relative standard deviation of a blank solution, n = 6) [38]. The IC50 values (the competitor concentration that causes 50% growth inhibition) were about 0.68 ng mL−1. Table 1 shows the comparison between this method and other typical detection methods. The result is comparable or better than that of previously reported p53 assays [39, 40, 43] and is more sensitive and advantageous in wider linear range than the former [41, 44]. That might be greatly contributed by the increased binding ratio of the ssDNA-NSP-DMAE-NHS conjugates coupling Au-MNPs-BSA-wtp53 conjugates and the high emitting efficiency of NSP-DMAE-NHS.
Selectivity of CLIA Detection Wild-Type p53
The selectivity was investigated by hybridize with ssDNA-NSP-DMAE-NHS sequences related to p53 tumor suppressor gene using Au-MNPs as a carrier. Three the same concentrations (0.66 ng mL−1) of wild-type p53 were prepared. Then, one-base mismatched wtp53 (6.6 ng mL−1) and three-base mismatched wtp53 (6.6 ng mL−1) were introduced into the 1 and 2 detection system, respectively, while the three-detection system was replaced by a blank buffer solution [45]. Further, 25-μL ssDNA-NSP-DMAE-NHS solution was mixtured with the above-incubated solution for 1 h, and then repeated the previous steps. The experiment results showed that the peaks of photon count did not decrease with the addition of mutated gene interference respectively (Fig. S3). Those signals in the presence of wtp53 were basically consistent. The results indicated that ssDNA-NSP-DMAE-NHS conjugates could clearly distinguish wtp53 from one-base mismatched wtp53 and three-base mismatched wtp53. That confirmed the high selectivity of this assay, importantly providing a novel sensing platform for the early detection diagnosis of cancer through mutated genes [42, 46].
Conclusions
In this study, a simple and ultrahigh sensitive chemiluminescence (CL) DNA assay based on Fe3O4@SiO2@Au-functionalized magnetic nanoparticles (Au-MNPs) has been established for the detection of p53 tumor suppressor gene. In this proposed strategy, application of integration of the advantages of NSP-DMAE-NHS and Au-MNPs in immunoassay was first adopted in p53 tumor suppressor gene. The calibration curve detection has been obtained for wild-type p53 with a wider linear measurement range through competition experiments. Furthermore, this proposed method exhibited high sensitivity for detection of wild-type p53 with a detection limit of 0.001 ng mL−1 (0.16 pM), and the results demonstrated that the chemiluminescence (CL) DNA assay has ultrahigh sensitivity and good selectivity. We also anticipate that the novel biosensor platform could be expanded for the cancer diagnosis and early detection of cancer and to make this commercialized for detection of other biomarkers in the future.
References
Junttila, M. R., & Evan, G. I. (2009). p53— a Jack of all trades but master of none. Nature Reviews Cancer, 9(11), 821–829.
Evan, G. I., & Vousden, K. H. (2001). Proliferation, cell cycle and apoptosis in cancer. Nature, 411(6835), 342–348.
Duffy, M. J., Synnott, N. C., McGowan, P. M., Crown, J., O’Connor, D., & Gallagher, W. M. (2014). p53 as a target for the treatment of cancer. Cancer Treatment Reviews, 40(10), 1153–1160.
Gaspar, V. M., Correia, I. J., Sousa, A., Silva, F., Paquete, C. M., Queiroz, J. A., & Sousa, F. (2011). Nanoparticle mediated delivery of pure P53 supercoiled plasmid DNA for gene therapy. Journal of Controlled Release, 156(2), 212–222.
Paleček, E., Ostatná, V., Černocká, H., Joerger, A. C., & Fersht, A. R. (2011). Electrocatalytic monitoring of metal binding and mutation-induced conformational changes in p53 at picomole level. Journal of the American Chemical Society, 133(18), 7190–7196.
Will, K., Warnecke, G., Bergmann, S., & Deppert, W. (1995). Species- and tissue-specific expression of the C-terminal alternatively spliced form of the tumor suppressor p53. Nucleic Acids Research, 23(20), 4023–4028.
Miyajima, K., Tamiya, S., Oda, Y., Adachi, T., Konomoto, T., Toyoshiba, H., Masuda, K., & Tsuneyoshi, M. (2001). Relative quantitation of p53 and MDM2 gene expression in leiomyosarcoma; real-time semi-quantitative reverse transcription-polymerase chain reaction. Cancer Letters, 164(2), 177–188.
Narayanaswami, G., & Taylor, P. D. (2002). Site-directed mutagenesis of exon 5 of p53: purification, analysis, and validation of amplicons for DHPLC. Genetic Testing, 6(3), 177–184.
Behn, M., & Schuermann, M. (1998). Sensitive detection of p53 gene mutations by a ‘mutant enriched’ PCR-SSCP technique. Nucleic Acids Research, 26(5), 1356–1358.
Van Orsouw, N. J., Dhanda, R. K., Rines, R. D., Smith, W. M., Sigalas, I., Eng, C., et al. (1998). Rapid design of denaturing gradient-based two-dimensional electrophoretic gene mutational scanning tests. Nucleic Acids Research, 26(10), 2398–2406.
Wang, J., Rivas, G., Cai, X., Chicharro, M., Parrado, C., Dontha, N., Begleiter, A., Mowat, M., Palecek, E., & Nielsen, P. E. (1997). Detection of point mutation in the p53 gene using peptide nucleic acid biosensor. Analytica Chimica Acta, 344(1–2), 111–118.
Noguchi, S., Koyama, H., Kasugai, T., Tsuji, N., Tsuda, H., Akiyama, F., Motomura, K., & Inaji, H. (1998). The possible prognostic significance of p53 immunostaining status of the primary tumor in patients developing local recurrence after breast-conserving surgery. Oncology, 55(5), 450–455.
Barnes, D. M., Dublin, E. A., Fisher, C. J., Levison, D. A., & Millis, R. R. (1993). Immunohistochemical detection of p53 protein in mammary carcinoma: an important new independent indicator of prognosis? Human Pathology, 24(5), 469–476.
Henke, R. P., Kruger, E., Ayhan, N., Hubner, D., Hammerer, P., & Huland, H. (1994). Immunohistochemical detection of p53 protein in human prostatic cancer. Journal of Urology, 152(4), 1297–1301.
Marquette, C. A., Degiuli, A., Imbert-Laurenceau, E., Mallet, F., Chaix, C., Mandrand, B., et al. (2005). Latex bead immobilisation in PDMS matrix for the detection of p53 gene point mutation and anti-HIV-1 capsid protein antibodies. Analytical and Bioanalytical Chemistry, 381(5), 1019–1024.
Xia, N., Liu, L., Yi, X., & Wang, J. (2009). Studies of interaction of tumor suppressor p53 with apo-MT using surface plasmon resonance. Analytical and Bioanalytical Chemistry, 395(8), 2569–2575.
Jiang, T., Minunni, M., Wilson, P., Zhang, J., Turner, A. P., & Mascini, M. (2005). Detection of TP53 mutation using a portable surface plasmon resonance DNA-based biosensor. Biosensors and Bioelectronics, 20(10), 1939–1945.
Han, S. H., Kim, S. K., Park, K., Yi, S. Y., Park, H. J., Lyu, H. K., Kim, M., & Chung, B. H. (2010). Detection of mutant p53 using field-effect transistor biosensor. Analytica Chimica Acta, 665(1), 79–83.
Chen, C. P., Ganguly, A., Lu, C. Y., Chen, T. Y., Kuo, C. C., Chen, R. S., Tu, W. H., Fischer, W. B., Chen, K. H., & Chen, L. C. (2011). Ultrasensitive in situ label-free DNA detection using a GaN nanowire-based extended-gate field-effect-transistor sensor. Analytical Chemistry, 83(6), 1938–1943.
Domenici, F., Bizzarri, A. R., & Cannistraro, S. (2012). Surface-enhanced Raman scattering detection of wild-type and mutant p53 proteins at very low concentration in human serum. Analytical Biochemistry, 421(1), 9–15.
Marquette, C. A., Lawrence, M. F., & Blum, L. J. (2006). DNA covalent immobilization onto screen-printed electrode networks for direct label-free hybridization detection of p53 sequences. Analytical Chemistry, 78(3), 959–964.
Wang, J., Zhu, X., Tu, Q., Guo, Q., Zarui, C. S., Momand, J., Sun, X. Z., & Zhou, F. (2008). Capture of p53 by electrodes modified with consensus DNA duplexes and amplified voltammetric detection using ferrocene-capped gold nanoparticle/streptavidin conjugates. Analytical Chemistry, 80(3), 769–774.
Rippin, T. M., Freund, S. M. V., Veprintsev, D. B., & Fersht, A. R. (2002). Recognition of DNA by p53 core domain and location of intermolecular contacts of cooperative binding. Journal of Molecular Biology, 319(2), 351–358.
Portefaix, J. M., Fanutti, C., Granier, C., Crapez, E., Perham, R., Grenier, J., Pau, B., & del Rio, M. (2002). Detection of anti-p53 antibodies by ELISA using p53 synthetic or phage-displayed peptides. Journal of Immunological Methods, 259(1–2), 65–75.
Xue, P., Zhang, K., Zhang, Z., Li, Y., Liu, F., Sun, Y., Zhang, X., Song, C., Fu, A., Jin, B., & Yang, K. (2012). Highly sensitive chemiluminescent analysis of residual bovine serum albumin (BSA) based on a pair of specific monoclonal antibodies and peroxyoxalate-glyoxaline-PHPPA dimer chemiluminescent system in vaccines. Applied Biochemistry and Biotechnology, 166(6), 1604–1614.
Kugimiya, A., & Fukada, R. (2015). Chemiluminescence detection of serine, proline, glycine, asparagine, leucine, and histidine by using corresponding aminoacyl-tRNA synthetases as recognition elements. Applied Biochemistry and Biotechnology, 176(4), 1195–1202.
Chuanlai, X., Cifang, P., Kai, H., Zhengyu, J., & Wukang, W. (2006). Chemiluminescence enzyme immunoassay (CLEIA) for the determination of chloramphenicol residues in aquatic tissues. Luminescence, 21(2), 126–128.
Lin, S., Han, S. Q., Liu, Y. B., Xu, W. G., & Guan, G. Y. (2005). Chemiluminescence immunoassay for chloramphenicol. Analytical and Bioanalytical Chemistry, 382(5), 1250–1255.
Xin, T. B., Wang, X., Jin, H., Liang, S. X., Lin, J. M., & Li, Z. J. (2009). Development of magnetic particle-based chemiluminescence enzyme immunoassay for the detection of 17beta-estradiol in environmental water. Applied Biochemistry and Biotechnology, 158(3), 582–594.
Yang, X. Y., Guo, Y. S., Bi, S., & Zhang, S. S. (2009). Ultrasensitive enhanced chemiluminescence enzyme immunoassay for the determination of alpha-fetoprotein amplified by double-codified gold nanoparticles labels. Biosensors and Bioelectronics, 24(8), 2707–2711.
Natrajan, A., & Wen, D. (2011). Facile N-alkylation of acridine esters with 1,3-propane sultone in ionic liquids. Green Chemistry, 13(4), 913.
Pingarrón, J. M., Yáñez-Sedeño, P., & González-Cortés, A. (2008). Gold nanoparticle-based electrochemical biosensors. Electrochimica Acta, 53(19), 5848–5866.
Xu, X., Deng, C., Gao, M., Yu, W., Yang, P., & Zhang, X. (2006). Synthesis of magnetic microspheres with immobilized metal ions for enrichment and direct determination of phosphopeptides by matrix-assisted laser desorption ionization mass spectrometry. Advanced Materials, 18(24), 3289–3293.
Deng, Y., Qi, D., Deng, C., Zhang, X., & Zhao, D. (2008). Superparamagnetic high-magnetization microspheres with an Fe3O4@SiO2 core and perpendicularly aligned mesoporous SiO2 shell for removal of microcystins. Journal of the American Chemical Society, 130(1), 28–29.
Schlaeppi, J.-M. A., Kessler, A., & Foery, W. (1994). Development of a magnetic particle-based automated chemiluminescent immunoassay for triasulfuron. Journal of Agricultural and Food Chemistry, 42(9), 1914–1919.
Malar, C. G., Seenuvasan, M., & Kumar, K. S. (2018). Prominent study on surface properties and diffusion coefficient of urease-conjugated magnetite nanoparticles. Applied Biochemistry and Biotechnology.
Pudlarz, A. M., Czechowska, E., Ranoszek-Soliwoda, K., Tomaszewska, E., Celichowski, G., Grobelny, J., & Szemraj, J. (2018). Immobilization of recombinant human catalase on gold and silver nanoparticles. Applied Biochemistry and Biotechnology.
Wang, X., Wang, X., Wang, X., Chen, F., Zhu, K., Xu, Q., & Tang, M. (2013). Novel electrochemical biosensor based on functional composite nanofibers for sensitive detection of p53 tumor suppressor gene. Analytica Chimica Acta, 765, 63–69.
Raoof, J. B., Ojani, R., Golabi, S. M., Hamidi-Asl, E., & Hejazi, M. S. (2011). Preparation of an electrochemical PNA biosensor for detection of target DNA sequence and single nucleotide mutation on p53 tumor suppressor gene corresponding oligonucleotide. Sensors and Actuators B: Chemical, 157(1), 195–201.
Chen, X., He, C., Zhang, Z., & Wang, J. (2013). Sensitive chemiluminescence detection of wild-type p53 protein captured by surface-confined consensus DNA duplexes. Biosensors and Bioelectronics, 47, 335–339.
Luo, X.-W., Du, F.-J., Wu, Y., Gao, L.-J., & Li, X.-X. (2013). Electrochemical DNA sensor for determination of p53 tumor suppressor gene incorporating gold nanoparticles modification. Chinese Journal of Analytical Chemistry, 41(11), 1664–1668.
Afsharan, H., Navaeipour, F., Khalilzadeh, B., Tajalli, H., Mollabashi, M., Ahar, M. J., & Rashidi, M. R. (2016). Highly sensitive electrochemiluminescence detection of p53 protein using functionalized Ru-silica nanoporous@gold nanocomposite. Biosensors and Bioelectronics, 80, 146–153.
Tiwari, A., Deshpande, S. R., Kobayashi, H., & Turner, A. P. F. (2012). Detection of p53 gene point mutation using sequence-specific molecularly imprinted PoPD electrode. Biosensors and Bioelectronics, 35(1), 224–229.
Qi, Y., Xiu, F. R., Zheng, M., & Li, B. (2016). A simple and rapid chemiluminescence aptasensor for acetamiprid in contaminated samples: sensitivity, selectivity and mechanism. Biosensors and Bioelectronics, 83, 243–249.
Esteban-Fernandez de Avila, B., Araque, E., Campuzano, S., Pedrero, M., Dalkiran, B., Barderas, R., et al. (2015). Dual functional graphene derivative-based electrochemical platforms for detection of the TP53 gene with single nucleotide polymorphism selectivity in biological samples. Analytical Chemistry, 87(4), 2290–2298.
Altintas, Z., & Tothill, I. E. (2012). DNA-based biosensor platforms for the detection of TP53 mutation. Sensors and Actuators, B: Chemical, 169, 188–194.
Funding
This work was supported by the National Natural Science Foundation of China (NSFC, Grant No. 81371642) and the 111 Project (B14040).
Author information
Authors and Affiliations
Corresponding author
Ethics declarations
Conflict of Interest
The authors declare that they have no competing interests.
Electronic Supplementary Material
ESM 1
(DOCX 5558 kb)
Rights and permissions
About this article
Cite this article
Wang, L., Yao, M., Fang, X. et al. Novel Competitive Chemiluminescence DNA Assay Based on Fe3O4@SiO2@Au-Functionalized Magnetic Nanoparticles for Sensitive Detection of p53 Tumor Suppressor Gene. Appl Biochem Biotechnol 187, 152–162 (2019). https://doi.org/10.1007/s12010-018-2808-1
Received:
Accepted:
Published:
Issue Date:
DOI: https://doi.org/10.1007/s12010-018-2808-1