Abstract
A Sphingobium sp. strain isolated from radioactive solid waste management site (RSMS) completely degraded 7.98 g/L of tributyl phosphate (TBP) from TBP containing suspensions in 3 days. It also completely degraded 20 mM dibutyl phosphate (DBP) within 2 days. The strain tolerated high levels of TBP and showed excellent stability with respect to TBP degradation over several repeated subcultures. On solid minimal media or Luria Bertani media supplemented with TBP, the RSMS strain showed a clear zone of TBP degradation around the colony. Gas chromatography and spectrophotometry analyses identified DBP as the intermediate and butanol and phosphate as the products of TBP biodegradation. The RSMS strain utilized both TBP and DBP as the sole source of carbon and phosphorous for its growth. The butanol released was completely utilized by the strain as a carbon source thereby leaving no toxic residue in the medium. Degradation of TBP or DBP was found to be suppressed by high concentration of glucose which also inhibited TBP or DBP dependent growth. The results highlight the potential of Sphingobium sp. strain RSMS for bioremediation of TBP and for further molecular investigation.
Similar content being viewed by others
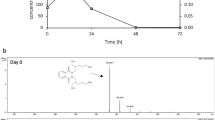
Explore related subjects
Discover the latest articles, news and stories from top researchers in related subjects.Avoid common mistakes on your manuscript.
Introduction
Tributyl phosphate (TBP) is a phosphotriester used in large volumes (∼3,000–5,000 tons/annum) in nuclear industry for extraction of uranium and plutonium (Hernandez 2001). TBP is also used as a plasticizer in the plastic industry, as a component of aircraft hydraulic fluid, and as an antifoaming agent (Nakamura 1991). Chemical destruction or incineration is generally used for the treatment of TBP waste (Anon 1992). Alkali treatment of TBP at high temperatures results in the formation of dibutyl phosphate and butanol (Healy 1976; Schulz and Navratil 1984), while incineration leads to formation of P2O5, a corrosive product (Manohar et al. 1999). Management of the byproducts formed by both these methods is rather difficult.
Bacterial strains have been reported to degrade TBP, either as mixed cultures or as single isolates (Ahire et al. 2011; Berne et al. 2005, 2004; Jeong et al. 1994; Owen et al. 1992; Rosenberg and Alexander 1979; Thomas and Macaskie 1998, 1996 Thomas et al. 1997). For example, Serratia odorifera or Rhodopseudomonas palustris, respectively, degraded 0.6 mM TBP within 8 h or 1.6 mM of TBP in 3 weeks. However, these strains could not mineralize or utilize TBP as carbon or phosphorous source for growth (Berne et al. 2005, 2004). In contrast, mixed cultures containing Pseudomonas have been shown to degrade 2 mM TBP in ∼3 days and utilize TBP both as carbon and phosphorous source (Thomas et al. 1997). But, the corresponding Pseudomonas strain irreversibly lost the ability to degrade or utilize TBP after eight serial subcultures (Thomas et al. 1997). Recently, 15 bacterial strains were reported for their ability to degrade and utilize TBP as the sole source of carbon and phosphorous (Ahire et al. 2011) but showed rather poor rates of TBP degradation (1–3.05 mM TBP degradation in 4 days). No sphingomonad has been reported to degrade TBP so far. The intermediates or products of TBP degradation formed by any of the strains reported so far have not been identified.
This paper reports isolation of a Sphingobium sp. strain RSMS (hereafter referred to as RSMS strain), which efficiently mineralized high level of TBP in media to butanol and phosphate and utilized them as carbon and phosphorous source for its growth. The strain exhibited excellent stability in terms of degradation and utilization of TBP over repeated subcultures. The biochemical pathway of TBP degradation by RSMS strain has been elucidated for the first time with dibutyl phosphate (DBP) as an intermediate of the process, which results in the formation of butanol, which is fully utilized, and excess inorganic phosphate as the end products.
Materials and methods
Isolation of the strain
A TBP degrading bacterial strain was isolated from the TBP waste storage tank located at the RSMS in Bhabha Atomic Research Centre, Mumbai, and denoted as RSMS strain. Swabs were taken from the side walls of the TBP waste storage tank with sterile cotton and immersed in 10 ml of sterile normal saline (0.85 % NaCl), shaken well and added to 250 ml conical flask containing 50 ml of Lurea Bertani medium supplemented with 2 mM TBP. The flasks were incubated under shaking (150 rpm) at 30 °C for 3 days. The cells were harvested, washed with saline, and directly plated on to mineral medium (hereafter referred as MM) agar (Thomas et al. 1997) plates supplemented with 2 mM TBP, as the sole source of carbon and phosphorous. The plates were incubated at 30 °C for 3 days. The colonies which grew and showed clearance of TBP around them were considered as putative TBP degraders. Such colonies were isolated and purified. The purified colonies were inoculated in to MM supplemented with 2 mM TBP, as the sole source of carbon and phosphorous and grown under shaking (180 rpm) at 30 °C. Cells from isolated colonies were washed with sterile saline and stored in 15 % glycerol at −70 °C. Luria Bertani agar (LBA) or MM agar plates supplemented with TBP were prepared by suspending 2.66 g/L TBP (equivalent to 10 mM TBP when dissolved) of medium and shaking them well. Due to insolubility of TBP, such plates were opaque in nature (Fig. 1). The TBP degradation by the strain was visualized by spotting aliquots of 20 μl of 20 OD600nm cells on such plates and incubating them at 30 °C for 7 days or until a clearly visible zone of clearance of TBP appeared.
Identification of the TBP degrading strain
Identification of the strain was carried out by 16S rRNA gene sequencing. The 16S rRNA gene amplification was carried out by using 8F (5'-AGAGTTTGATCCTGGCTCAG-3') (Eden et al. 1991) and 1387R (5'-GGGCGGA/TGTGTACAAGGC-3') (Marchesi et al. 1998) primers, and the purified DNA product was sequenced. Similarity searches for the obtained 16S rRNA gene sequence were carried out using the BLAST algorithm available at http://www.ncbi.nlm.nlh.gov (Altschul et al. 1990). The nucleotide sequence (1,248 nt) was submitted to Genbank (accession no. EU629211.2). Nitrate reductase test was performed as described earlier (Skerman 1967). The strain was deposited with Microbial Type Culture Collection and Gen Bank, Chandigarh, India (http://mtcc.imtech.res.in) under the accession number 11630.
Media and growth conditions
A mineral medium described earlier (Thomas et al. 1997) was used as basal medium for the growth of the strain. The mineral medium was buffered with 100 mM 3-(N-morpholino) propane sulfonic acid at pH 7.0 (hereafter referred as modified mineral medium or MMM). Glucose and 5 mM KH2PO4 or 20 mM DBP or the required amounts of TBP were added to MMM as the sole source of carbon and phosphorous. Due to its low solubility, TBP addition to the media did not yield a clear solution but resulted in opaque whitish TBP containing suspensions, which were used directly for experiments. The strain was grown either in liquid MMM at 30 °C under shaking (180 rpm) or on solid media supplemented with 1.5 % Difco Bacto agar (MMMA) at 30 °C. All the glassware were soaked in 30 % (v/v) of nitric acid for 12 h and then washed two times with distilled water to remove inorganic phosphate.
Preinoculum preparation
A single colony of RSMS strain was inoculated in MMM supplemented with 0.5 % glucose and 5 mM KH2PO4. Exponentially grown cells were harvested by centrifugation at 10,000 rpm for 5 min. The cell pellet was washed three times with equal volumes of MMM to remove residual glucose and phosphate and resuspended in fresh MMM. This cell suspension was used as preinoculum for all the growth and TBP degradation related experiments.
Growth and TBP degradation
All growth related experiments in liquid MMM were started with an initial inoculum density of 0.1 OD600nm (∼5 × 107 CFU/ml). The ability of the RSMS strain to utilize TBP or DBP was assessed by inoculating the strain into MMM containing desired amounts of TBP or DBP (British Drug Houses, UK) as the sole source of carbon and phosphorous. Cell density required for the optimal rate of DBP or TBP degradation was assessed by inoculating RSMS culture at various cell densities (OD600nm) into MMM supplemented with 20 mM DBP or 7.98 g/L of TBP (equivalent to 30 mM TBP when dissolved). Suppression of DBP or TBP degradation by glucose was studied by inoculating the strain in to MMM containing 10 mM DBP or 2.66 g /L TBP (equivalent to 10 mM TBP when dissolved) supplemented with different concentrations of glucose. Appropriate controls were used in all experiments.
Growth was generally measured as colony forming units (CFUs) at 30 °C after 48 h growth on MMMA plates supplemented either with 0.5 % glucose and 5 mM KH2PO4 or with TBP/DBP (added as the sole source of carbon and phosphorous). Growth was also assessed spectrophotometrically as increase in the cell density (OD at 600 nm). TBP and DBP degradation were spectrophotometrically assessed as phosphate released in the medium by phosphomolybdic acid method (Ames 1966).
Analysis of TBP degradation products
A gas chromatograph (Schimadzu-GC-2014) equipped with a 10 % XE-60 column (1.5 m × 0.32 cm) was used for the analysis of TBP, DBP, and butanol. Helium was used as carrier gas at a flow rate of 40 ml/min. Column temperature was maintained at 120 °C (1 min) to 230 °C (10 min), with an increment of 10 °C/min. The injection port and detector temperatures were maintained at 240 °C and 260 °C, respectively. Thermal conductivity detector was used for the quantitation of organophosphates (TBP, DBP) while butanol was estimated using a flame ionization detector.
Predetermined amounts of TBP (0.532 to 7.93 g/L) or DBP (1–30 mM) were added to MMM and individually acidified with 1/100 volumes of 10 N H2SO4. To the acidified samples, 1/4 volume of 10 % benzyl alcohol (in benzene, v/v) [hereafter referred as benzene benzyl alcohol( BBA)] was added, shaken well for 15 min, and allowed to stand for clear separation of layers. To the top organic layer, four volumes of diazomethane were added for esterification and the esterified samples were injected in to gas chromatograph in appropriate volumes (1–2 μl). Quantification of butanol was carried out by direct injection of MMM containing predetermined amount of butanol. Standard curves for all the analytes were prepared by plotting peak areas against the amount of the analyte injected in to GC.
For the analysis of TBP degradation products by RSMS strain, freshly prepared preinoculum was inoculated at a cell density of 3 OD600nm in to 5 ml of MMM supplemented with 7.98 g/L TBP (equivalent to 30 mM TBP) or 20 mM DBP. The cultures were incubated at 30 °C under shaking (180 rpm). The following controls were used: MMM containing equivalent of 30 mM TBP (7.98 g/L) or 20 mM DBP without cells and MMM containing 3 OD600nm cells without TBP or DBP. Samples were removed each day and subjected to centrifugation at 10,000 rpm for 5 min. A small volume (500 μl) of the supernatant was aliquoted for the analysis of butanol and phosphate. The remaining ∼4.5 ml of supernatant was collected, along with traces of TBP present on the walls of the conical flask by rinsing with 1 ml of BBA. These samples were processed for the analysis of TBP, DBP, and butanol by gas chromatography. The concentration of each compound was calculated by using the calibration curve.
Results
Isolation of the TBP degrading strain
A single monoxenic bacterial isolate capable of degrading and utilizing TBP as sole source of carbon and phosphorous was isolated and named as RSMS strain. On account of its very low solubility, the TBP containing LBA and MMMA solid media were opaque in nature. A clear transparent distinct zone around the culture spot revealed degradation of TBP. The isolated strain showed a clearly visible phenotype for TBP degradation around the growing colonies (Fig. 1a, b).
Identification of the strain
Similarity search for the 16S rRNA gene sequence of the isolated strain by BLAST analysis showed that the strain had the highest homology (1,245 identities out of 1,248 nucleotides) with Sphingobium sp. strain KK22 (GenBank acc. no. HQ830159.1) (Kunihiro et al. 2013). Analysis of the DNA sequence for the signature nucleotides showed nucleotides T, G, A, T, A, U, G, and T at positions 52, 134, 359, 593, 987, 990, 1,215, and 1,218, respectively, characteristics of the genus Sphingobium. The strain tested negative for nitrate reductase test. A phylogenetic tree was constructed by taking 16s rRNA gene sequences of 55 sphingomonads (Fig. S1). The analysis corroborated identification of RSMS strain as a Sphingobium species with three other Sphingobium species, including Sphingobium sp. strain KK22, in the same clad.
Growth and TBP degradation
RSMS strain could not grow in MMM containing glucose or KH2PO4 alone. However, MMM supplemented with TBP alone supported active growth of the strain, suggesting that the strain utilized TBP as sole source of carbon and phosphorous (Fig. 2a). Growth and TBP degradation by the strain increased with increasing amounts of TBP up to 7.98 g/L (equivalent to 30 mM TBP when dissolved) (Fig. 2a, b). The number of CFUs in MMM suspensions containing TBP increased from 5 × 107/ml at inoculation to 1.3 × 109/ml after 48 h, which is equivalent to >25 times increase in cell number or 4.5 doublings (Fig. 2a). The deduced doubling time for the strain at this TBP concentration was ∼11 h. The strain continued to show TBP degradation from TBP suspensions even after attaining stationary phase. Stability of the RSMS strain with respect to TBP degradation and utilization for growth was assessed in 20 repeated consecutive subcultures of 24 h duration each in MMM supplemented with 2.66 g/L of TBP (equivalent to 10 mM TBP when dissolved). In 20 repeated subcultures, the strain exhibited complete stability with respect to the TBP degradation and growth.
Growth and TBP degradation kinetics of the RSMS strain. a Growth and b TBP degradation were studied in MMM supplemented with 0.532 g/L (black-filled square), 1.33 g/L (black-filled circle), 2.66 g/L (black-filled up-pointing triangle), 7.98 g/L (white circle), or 13.3 g/L (black-filled down-pointing triangle) of TBP, as the sole source of carbon and phosphorous. Initial inoculum density was set at an OD600nm of 0.1 (∼5 × 107 CFU/ml). Following controls were also included: TBP supplemented MMM without cells (black left-pointing triangle), glucose supplemented culture in MMM without added TBP (white square) or KH2PO4 supplemented culture in MMM without added TBP (white diamond). Growth was assessed as colony forming units (CFU) and TBP degradation was spectrophotometrically estimated as phosphate released upon TBP degradation. c Degradation of TBP by RSMS strain inoculated at different cell densities (OD600nm) in MMM supplemented with 7.98 g/L TBP as the sole source of carbon and phosphorous. TBP degradation was spectrophotometrically assessed in terms of phosphate released 24 after inoculation
The initial inoculum density determined the rate of TBP degradation by RSMS strain (Fig. 2c). Culture incubated in 7.98 g/L TBP suspension at a cell density of 3 OD600nm showed complete degradation of 10 mM TBP in 24 h (Fig. 2c) yielding a degradation rate of ∼ 0.42 μmol TBP ml−1 h−1. The rate of TBP degradation at 2 mM TBP (concentration used in previously reported studies) concentration was observed to be 0.25 ± 0.02 μmol ml−1 h−1 (Table 1). No further increase in the rate of TBP degradation was observed at higher TBP concentration (>7.98 g/L) (data not shown). The RSMS strain degraded 2 mM TBP in 8 h and 30 mM TBP in 3 days (Table 1). Attempts were also made to see the TBP and DBP degradation in cell free extracts of RSMS strain. While no TBP degradation was seen in cell free extracts, DBP degradation and release of inorganic phosphate could be seen in cell free extracts of both TBP and DBP grown cells (Table. S1). The reasons for the requirement of the intact cells for TBP to DBP conversion are unknown, but may have to do with localization of corresponding triesterase in periplasm/membrane.
Suppression of TBP and DBP degradation in the presence of glucose
Possible catabolic repression by glucose was evaluated in MMM suspensions containing 2.66 g/L TBP (equivalent to 10 mM TBP when dissolved) (Fig. 3), wherein the growth was less than that in 7.98 g/L TBP suspension (Fig. 2a). Cultures grown in 2.66 g/L TBP suspension alone showed threefold less growth compared to the cultures grown in such amounts of TBP along with 0.2 or 0.5 % glucose, but the TBP degradation was observed to be similar in all the cases (Fig. 3a, b). Decreased TBP degradation and corresponding growth inhibition (due to unavailability of phosphorous source) were observed with increasing concentrations of glucose above 0.5 % (Fig. 3a, b). No degradation of TBP and correspondingly no growth was observed in cultures supplemented with 5 or 10 % glucose (Fig. 3c). DBP degradation was found to be similarly inhibited by 10 % glucose (Fig. 3c). However, such repression of TBP/DBP degradation by glucose was reversible. TBP degradation and dependent growth was induced upon removal of glucose albeit after a 6 h lag (Fig. S2). DBP degradation in cell free extracts similarly revived after a lag upon removal of glucose (Table. S1).
Inhibition of a TBP dependent growth and b TBP degradation by glucose. MMM suspension containing 2.66 g/L TBP (black-filled up-pointing triangle) was supplemented with glucose either at 0.2 % (white circle), 0.5 % (white square), 1 % (black-filled down-pointing triangle), 2 % (black-filled square), 5 % (white diamond), or 10 % (black left-pointing triangle) concentration. Following controls were also included: cells in MMM (black right-pointing triangle), cells in MMM supplemented with 10 % glucose (black-filled diamond), or cells in MMM supplemented with 10 % glucose and 5 mM KH2PO4 (black-filled circle). Other details were as described in legend to Fig. 2. c Suppression of TBP or DBP dependent growth by glucose. Growth was assessed as CFUs on MMMA plates containing 2.66 g/L TBP or 10 mM DBP. The plates were supplemented with or without 10 % glucose
Analysis of TBP degradation products
Gas chromatography procedures were standardized to detect TBP, DBP, and butanol, as well resolved peaks. The sensitivity of detection was 0.1, 0.5, and 0.67 nmol for TBP, DBP, and butanol, respectively. The methods showed linearity up to 30 nmol for all the analytes (data not included).
MMM suspension containing either 7.98 g/L TBP (equivalent to 30 mM TBP when dissolved) or 20 mM DBP alone (without cells) did not show any of their respective intermediates or products of the degradation (data not shown). The RSMS strain completely degraded 30 mM TBP in 3 days when inoculated at a cell density of 3 OD600nm. Both DBP and butanol were detected as products of TBP degradation by gas chromatography (Fig. 4a). The concentration of butanol remained at peak levels in the first 2 days, whereas the DBP reached peak levels at 48 h. Independent measurements showed that the phosphate increased linearly between 0 and 72 h before reaching a peak value. After 3 days, neither TBP, DBP nor butanol was detected in the culture medium suggesting their complete degradation/utilization. Concomitantly, growth of the strain saturated by day 3. The RSMS strain also degraded 20 mM DBP in 2 days with 50 % degradation occurring in the first 24 h (Fig. 4b). Butanol and phosphate were detected as the end products. While butanol was completely used up as carbon source by day 3, phosphate released was in excess of growth requirement and accumulated in the medium. Appearance of butanol (an alcohol) and phosphate among the TBP and DBP degradation products suggests sequential cleavage of all ester bonds, resulting in the conversion of TBP to DBP and then (possibly through MBP) to butanol and phosphate.
Analysis of TBP degradation products. The degradation of added analytes by RSMS strain and appearance of degradation products in MMM supplemented with a 7.98 g/L TBP or b 20 mM DBP. Various symbols indicate levels of TBP (black-filled circle), DBP (white square), butanol (black-filled square), or inorganic phosphate (black-filled up-pointing triangle). Growth (black-filled down-pointing triangle) of the strain using added compounds as the sole source of carbon and phosphorous is shown for each analyte
Discussion
TBP storage tanks were chosen for the isolation of TBP degrading strain, wherein the TBP concentrations are very high and no other carbon or phosphorous source is available. Such a choice might have facilitated exclusion of organisms incapable of utilizing TBP or unable to survive at such high TBP concentration (1.1 M) (Schultz and Navratil 1984). The RSMS strain showed a clear zone of TBP degradation around the colony (Fig. 1). Such visible phenotype has not been reported earlier for any of the strains reported to degrade TBP. The RSMS strain was identified to belong to the genus Sphingobium based on signature nucleotides of 16S rRNA gene sequence and the negative nitrate reductase test, which are characteristic features of the genus Sphingobium (Takeuchi et al. 2001). Sphingomonads have been known to metabolize several xenobiotics, but this is the first strain of a sphingomonad reported to biodegrade TBP.
The solubility of TBP in water is 400 mg/L (∼1.5 mM) (Hernandez, 2001). Most of the previous studies therefore have been restricted to 2 mM TBP concentrations. However, TBP concentration at storage sites is ∼1.1 M and any real biodegrading strain must function at much higher concentration of TBP than what the solubility permits. Addition of >2 mM TBP to the solutions results in opaque liquid or solid media wherein the effective concentration of TBP cannot be estimated due to the immiscible nature of TBP. However, active culture of an efficient TBP degrading strain under strong agitation should quickly degrade the sparingly dissolved TBP forcing fresh solubilization and further degradation. The process can continue until all the added TBP is completely degraded.
We attempted such degradation of TBP from nonhomogeneous liquid suspensions and solid media containing equivalent of up to 30 mM TBP when dissolved. Even from such immiscible media, TBP was rapidly and completely degraded and utilized by RSMS strain. This was evident from (1) formation of a clear, transparent zone of clearance around cells on TBP containing agar plates (Fig. 1), (2) TBP concentration dependent increase in phosphate release (TBP degradation) as well as growth of the RSMS strain (∼25 times increase in CFUs) in TBP supplemented suspensions (Fig. 2), and (3) release of ∼29 mM inorganic phosphate after complete degradation of 30 mM TBP. The strain continued to show TBP degradation even in stationary phase (Fig. 2b) and also utilized the released butanol (∼90 mM) for its growth (Fig. 4). This type of efficient utilization can prevent the accumulation of toxic byproducts such as butanol formed due to TBP degradation in the medium.
TBP degradation results in inorganic phosphate release which can inhibit further TBP degradation and TBP dependent growth as reported earlier (Thomas and Macaskie 1998). However, no adverse effect on TBP dependent growth was seen in the presence of 0–20 mM phosphate (supplied in the form of KH2PO4) and TBP degradation was not effected significantly even in the presence of 100 mM phosphate, as adjudged from diameter of the zone of clearance on MMM plates supplemented with 10 mM TBP + 0–100 mM KH2PO4 (data not included).
TBP degradation rates of various bacterial strains reported earlier range from 1.6 mM in 3 weeks (R. palustris) and 2 mM in 3 days (mixed cultures of Pseudomonas) (Berne et al. 2005; Thomas et al. 1997) at 2 mM TBP concentration. An earlier study reported 3.05 mM TBP degradation from media suspensions containing equivalent of 5 mM dissolved TBP in 4 days (Ahire et al. 2011). This was claimed to be the highest amount of TBP degradation reported so far. In contrast, the RSMS strain was found to be far superior. TBP degradation by RSMS strain ranged from 0.25–0.45 μmol ml−1 h−1 and resulted in complete degradation of 2 or 30 mM TBP in 8 or 73 h, respectively.
TBP at 10 to 400 μM concentration inhibits cell division in most bacteria (Nakamura 1991). Few strains have been reported to tolerate 5 mM TBP concentration, which is the highest tolerance to TBP reported so far (Ahire et al. 2011). In contrast, the RSMS strain was found to tolerate equivalent of even 30 mM TBP in suspension without loss of viability in MMM which is truly remarkable. Such high tolerance is needed when dealing with the actual waste where the concentrations of TBP are very high (Schultz and Navratil 1984).
TBP and DBP degradation were strongly inhibited by glucose concentrations above 5 %, indicating possible catabolite repression (Fig. 3b, c). The consequent unavailability of phosphorous (from TBP/DBP degradation) completely inhibited growth in TBP or DBP supplemented cultures containing 5 or 10 % of glucose, indicating complete repression of TBP degradation (Fig. 3a). Such efficient regulation of TBP degradation has also not been reported earlier.
Over the last three decades, mineralization of TBP has been proposed to be mediated by phosphoesterases, wherein the tri-, di-, and monoesterases would sequentially act on TBP to release DBP and monobutyl phosphate (MBP) as intermediates and butanol and phosphate as final products (Macaskie 1991; Owen et al. 1992; Rosenberg and Alexander 1979; Thomas et al. 1997), but no such intermediates or butanol were detected or reported earlier. The present study clearly showed DBP as the most immediate intermediate in TBP degradation and butanol and phosphate as the products of TBP degradation by RSMS strain. Butanol and phosphate were also found to be the products of DBP degradation (Fig. 4b). The scheme of TBP degradation revealed by the present investigation is delineated in Fig. 5. It is possible that DBP degradation to butanol and phosphate proceeds through MBP. However, unavailability of purified MBP precluded experiments which could establish/rule out this possibility.
More than 20 bacterial isolates have been reported so far to degrade TBP. Many of the earlier reported strains were relatively inefficient or unstable (Thomas et al. 1997). For molecular investigation of the TBP degradation, it is necessary to have a stable, single pure isolate which exhibits a visual or easy to score phenotype and survives on high concentrations of TBP, utilizing it as the sole source of carbon and phosphorous. The Sphingobium sp. strain RSMS fulfills all these requirements and qualifies as an appropriate strain for further molecular dissection of mechanisms (genes/proteins) underlying TBP biodegradation.
References
Ahire KC, Kapadnis BP, Kulkarni GJ, Shouche YS, Deopurkar RL (2011) Biodegradation of tributyl phosphate by novel bacteria isolated from enrichment cultures. Biodegradation 23:165–176
Altschul SF, Gish W, Miller W, Myers EW, Lipman DJ (1990) Basic local alignment search tool. J Mol Biol 215:403–410
Ames BN (1966) Assay of inorganic phosphate, total phosphate and phosphatases. In: Elizabeth F. Neufeld VG (ed) Methods in Enzymology Vol 8. Academic, p 115–118
Anon (1992) Treatment and conditioning of radioactive organic liquids. In: IAEA (ed). IAEA, Vienaa. http://www-pub.iaea.org/MTCD/publications/pdf/te_656_web.pdf. Accessed 16 Jan 2013
Berne C, Allainmat B, Garcia D (2005) Tributyl phosphate degradation by Rhodopseudomonas palustris and other photosynthetic bacteria. Biotechnol Lett 27:561–566
Berne C, Montjarret B, Guountti Y, Garcia D (2004) Tributyl phosphate degradation by Serratia odorifera. Biotechnol Lett 26:681–686
Eden PA, Schmidt TM, Blakemore RP, Pace NR (1991) Phylogenetic analysis of Aquaspirillum magnetotacticum using polymerase chain reaction-amplified 16S rRNA-specific DNA. Int J Syst Bacteriol 41:324–325
Healy TV (1976) Fuel reprocessing solvent tributyl phosphate: its degradation, clean-up, and disposal. Management of radioactive waste from the nuclear fuel cycle. In: IAEA, Vienna, vol 1
Hernandez O (2001) Tributyl phosphate-SIDS initial assessment report for 12th SIAM. UNEP publications. http://www.chem.unep.ch/irptc/sids/OECDSIDS/126-73-8.pdf. Accessed 17 Jan 2013
Jeong B, Klm H-W, Owen S, Dick RE, Macaskie L (1994) Phosphoesterase activity and phosphate release from tributyl phosphate by a Citrobacter sp. Appl Biochem Biotechnol 47:21–32
Kunihiro M, Ozeki Y, Nogi Y, Hamamura N, Kanaly RA (2013) Benz[a]anthracene biotransformation and production of ring fission products by Sphingobium sp. strain kk22. Appl Environ Microbiol 79:4410–4420
Macaskie LE (1991) The application of biotechnology to the treatment of wastes produced from the nuclear fuel cycle: biodegradation and bioaccumulation as a means of treating radionuclide-containing streams. Crit Rev Biotechnol 11:41–112
Manohar S, Srinivas C, Vincent T, Wattal PK (1999) Management of spent solvents by alkaline hydrolysis process. Waste Manage 19:509–517
Marchesi JR, Sato T, Weightman AJ, Martin TA, Fry JC, Hiom SJ, Dymock D, Wade WG (1998) Design and evaluation of useful bacterium-specific PCR primers that amplify genes coding for bacterial 16S rRNA. Appl Environ Microbiol 64:795–799
Nakamura A (1991) International programme on chemical safety—environmental health criteria, 112-tri-n-butyl phosphate. World Health Organisation. Geneva. http://whqlibdoc.who.int/ehc/WHO_EHC_112_eng.pdf. Accessed 3 Apr 2012
Owen S, Jeong BC, Poole PS, Macaskie LE (1992) Tributyl phosphate degradation by immobilized cells of a Citrobacter sp. Appl Biochem Biotechnol 34–35:693–707
Rosenberg A, Alexander M (1979) Microbial cleavage of various organophosphorus insecticides. Appl Environ Microbiol 37:886–891
Schulz WW, Navratil JD (1984) Science and technology of tributyl phosphate, vol 1. CRC, Boca Raton
Skerman VBD (1967) A guide to the identification of the genera of bacteria. The Williams & Wilkins Co, Baltimore
Takeuchi M, Hamana K, Hiraishi A (2001) Proposal of the genus Sphingomonas sensu stricto and three new genera, Sphingobium, Novosphingobium and Sphingopyxis, on the basis of phylogenetic and chemotaxonomic analyses. Int J Syst Evol Microbiol 51:1405–1417
Thomas RA, Macaskie LE (1998) The effect of growth conditions on the biodegradation of tributyl phosphate and potential for the remediation of acid mine drainage waters by a naturally occurring mixed microbial culture. Appl Microbiol Biotechnol 49:202–209
Thomas RA, Morby AP, Macaskie LE (1997) The biodegradation of tributyl phosphate by naturally occurring microbial isolates. FEMS Microbiol Lett 155:155–159
Thomas RAP, Macaskie LE (1996) Biodegradation of tributyl phosphate by naturally occurring microbial isolates and coupling to the removal of uranium from aqueous solution. Environ Sci Technol 30:2371–2375
Acknowledgments
The authors thank Anubrata Das and A.S Rao for the isolation of the RSMS strain, Chitra Seetharam Misra for the valuable suggestions during manuscript preparation, Dr. Kayzad Nilgiriwala for the help in 16S rRNA gene sequencing, and Mrs. Saraswathi Perumal for the technical help.
Author information
Authors and Affiliations
Corresponding author
Electronic supplementary material
Below is the link to the electronic supplementary material.
ESM 1
(PDF 914 kb)
Rights and permissions
About this article
Cite this article
Rangu, S.S., Muralidharan, B., Tripathi, S.C. et al. Tributyl phosphate biodegradation to butanol and phosphate and utilization by a novel bacterial isolate, Sphingobium sp. strain RSMS. Appl Microbiol Biotechnol 98, 2289–2296 (2014). https://doi.org/10.1007/s00253-013-5158-5
Received:
Revised:
Accepted:
Published:
Issue Date:
DOI: https://doi.org/10.1007/s00253-013-5158-5