Abstract
In the present study, a new method based on microwave-assisted extraction and dispersive liquid–liquid microextraction (MAE–DLLME) followed by high-performance liquid chromatography (HPLC) was proposed for the separation and determination of oleuropein (Ole) and hydroxytyrosol (HyT) from olive pomace samples. The effective factors in the MAE–DLLME process such as microwave power, extraction time, the type and volume of extraction, and dispersive solvents were studied and optimized with the aid of response surface methodology (RSM) based on a central composite design (CCD) to obtain the best condition for Ole and HyT extraction. At the optimized conditions, parameter values were 220 W microwave power, 12 min extraction time, 60 μL extracting solvent, and 500 μL dispersive solvent. The calibration graphs of the proposed method were linear in the range of 10–500,000 μg L−1, with the coefficient of determination (R2) higher than 0.99 for Ole and HyT. Repeatability of the method, described as the relative standard deviation (RSD), was 4.12–5.63% (n = 6). The limits of detection were 35 and 20 μg L−1 for Ole and HyT, respectively. The recoveries of these compounds in the spiked olive pomace sample were from 93 to 98%. The proposed method, MAE–DLLME–HPLC–UV, was an accurate, rapid, and reliable method when compared with previous methods.
Similar content being viewed by others
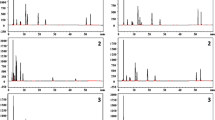
Explore related subjects
Discover the latest articles, news and stories from top researchers in related subjects.Avoid common mistakes on your manuscript.
Introduction
By-products and wastes from plant food processing, which are principal problems for the food industry, are promising sources of bioactive compounds. A vast amount of different by-products is generated during the olive processing industry, which includes the production of olive oil and table olives, such as olive pomace, wastewater, and leaves (Romero-García et al. 2014). The olive leaves and pomace contain a wide variety of phenolic compounds such as oleuropein (Ole), hydroxytyrosol (HyT), tyrosol, apigenin, and luteolin that are potent antioxidants (Rodrigues et al. 2015). Olive leaf extracts have been shown to possess antioxidant activities upper than those of BHT and vitamin E with regard to the protective effect applied on methyl linoleate (Le Tutour and Guedon 1992). The chemical composition of olive leaves and pomace is changeable due to the origin of the olives, olive variety, culture conditions, and extraction procedure (Dermeche et al. 2013).
Ole is the most abundant phenolic compound in unprocessed olive fruit and leaves and is responsible for the bitter taste of olive fruit (Malik and Bradford 2006). HyT, an Ole derivative, is present in large amounts in the processed olive fruit and olive oil. Health benefits of these compounds have been extensively evaluated. It has been demonstrated that Ole and HyT act as antioxidants and reduce the risk of coronary diseases (Manna et al. 2002; Vilaplana-Pérez et al. 2014), they protect enzyme activity and cell death in cancer patients (Tripoli et al. 2005; Vilaplana-Pérez et al. 2014), and they exhibit antimicrobial and antiviral properties (Kubo et al. 1995; Malik and Bradford 2006), and HyT also protects against atherosclerosis (Tripoli et al. 2005) and prevents diabetic neuropathies (Japón-Luján et al. 2006b).
Numerous methods were used for extraction of antioxidant components from natural products such as conventional heat-assisted extraction, soxhlet extraction, maceration, and percolation, among others. These conventional methods require large quantities of solvents and are often time-consuming (Heleno et al. 2016; Yang et al. 2017). Recently, emerging extraction technologies such as ultrasound-assisted extraction, supercritical fluid extraction, pressurized solvent extraction, and microwave-assisted extraction have been applied by the researchers with the aim of more efficient extraction of minor and major antioxidant agents in the natural sources. These emerging methods have several benefits like saving energy and reagents, being fast and efficient, avoiding losses, and optimizing the extraction procedure (Heleno et al. 2016).
Recently, the health benefits of Ole and HyT have stimulated the development of analytical methods for their separation and quantification. Ultrasound-assisted extraction coupled with capillary electrophoresis–diode array detection was used in the extraction and determination of Ole and HyT in alperujo (Priego-Capote et al. 2004). Ultrasound-assisted extraction followed by liquid chromatography–photodiode array detection was applied to determine Ole in the olive leaves (Japón-Luján et al. 2006a). In other studies, the isolation and determination of Ole in the olive leaves were done by microwave-assisted extraction coupled with liquid chromatography–photodiode array detection (Japón-Luján et al. 2006b), and also, microwave-assisted extraction followed by liquid chromatography–tandem mass spectrometry was applied in the extraction and determination of Ole and HyT in the alperujo (Pérez-Serradilla et al. 2007). Godoy-Caballero et al. (2013) employed the reversed-phase dispersive liquid–liquid microextraction for the determination of Ole and HyT in virgin olive oil coupled with rapid resolution liquid chromatography–diode array detection and liquid chromatography–mass spectrometry. In another study, ultrasound-assisted emulsification–microextraction followed by liquid chromatography–photodiode array detection was developed to identify and quantify Ole and HyT from extra-virgin olive oil (Reboredo-Rodríguez et al. 2014). These methods have been evaluated as reliable alternatives to the classic liquid–liquid extraction (LLE) technique.
In recent years, much attention has been considered on the application of microwave-assisted extraction (MAE) in analytical and biological chemistry (Bener et al. 2016). MAE is the process by which microwave energy is applied to heat extraction solvents in contact with solid samples. This phenomenon leads to migration of target compounds from the sample into the extraction solvent while the extraction solvent diffuses into the samples and extracts the target components. It is noticeable that MAE has several advantages when compared with traditional methods. It can be done in a short time and at a high rate with good product quality and the solvent consumption can be reduced; polar or non-polar extraction solvents can be used for extraction of target compounds; all parameters in the MAE method can be controlled by a software-based system (Bener et al. 2016; Khajeh and Ghanbari 2011); better reproducibility, higher recoveries, and extraction efficiency can be achieved; and the thermal degradation effects can be avoided (Bener et al. 2016). Besides, in terms of used energy, sample preparation, and extraction solvents, MAE is a green extraction and green chemistry (Rombaut et al. 2014).
Dispersive liquid–liquid microextraction (DLLME) is an alternative sample preparation method which was introduced in 2006 (Rezaee et al. 2006) and is based on a ternary component solvent. The emulsion phenomena are performed by injecting a mixture of the extraction and disperser solvents in the aqueous sample containing the target analyte and a cloudy emulsion form. After a very short time, equilibrium is achieved and the separation process is done by centrifugation (Habibi et al. 2017; Sereshti et al. 2014). This technique is simple, rapid, and inexpensive and provides high recovery and enrichment factors (Farajzadeh et al. 2016). However, the chlorinated solvents as extraction solvents such as chlorobenzene, chloroform, or carbon tetrachloride that were used in most applications of DLLME procedure were typically highly toxic (Habibi et al. 2017; Madani-Tonekaboni et al. 2015).
MAE–DLLME has been used for extraction of PAHs from grilled meat (Kamankesh et al. 2015), polyamines from turkey breast meat (Bashiry et al. 2016), and nitrosamines from sausage and salami (Ramezani et al. 2015). Nevertheless, MAE–DLLME–HPLC has not been used to facilitate the extraction and determination of Ole and HyT in olive pomace or the other food samples.
Response surface methodology (RSM) is an empirical model method useful to improve, develop, and optimize processes. RSM is a multivariate statistic technique for designing the experiments, describing the interactive effects and the relationship between the factors and the response, making models, and looking for the optimal condition (Habibi et al. 2013; Sereshti et al. 2014). RSM minimizes the number of experimental runs and is capable of finding the interactive effect of the independent variables on the response and leads to estimate the best response and optimal conditions (Habibi et al. 2017).
Therefore, in this study, we propose for the first time to the best of our knowledge a new and reliable MAE–DLLME–HPLC-UV method to extract and determine Ole and HyT from olive pomace samples. Important parameters that may affect extraction efficiency in the MAE-DLLME procedure, such as microwave power, extraction time, type and volume of extraction, and dispersive solvents, were optimized with the aid of RSM based on a CCD to introduce the best conditions for the separation and determination of Ole and HyT in olive pomace samples.
Materials and Methods
Reagent and Chemicals
Ole and HyT with purity higher than 98% were purchased from Sigma-Aldrich (Hohenbrunn, Germany). 1-Octanol, ethanol, methanol, glacial acetic acid, and acetonitrile were prepared from Merck (Darmstadt, Germany). The single stock solution of Ole and HyT was prepared at a concentration of 1000 μg mL−1 in methanol. For preparation of standard solution with a concentration of 500 μg mL−1, the stock standard solution was diluted with methanol. Working standard solutions from 10 to 500,000 μg L−1 were prepared by diluting stock standard solution with methanol. All solutions were refrigerated at 4 °C.
Sample and Sample Preparation
Olive pomace samples, as olive oil industry by-products, were prepared from the olive oil industry in November 2016. Olive pomace samples were dried and milled in the laboratory. 0.5 g of dried olive pomace samples was accurately weighed and transferred to a quartz reaction vessel, and 5 mL of water:ethanol solution (40:60) was added to extract Ole and HyT from olive pomace. The vessel was closed and placed in a Multiwave 3000 microwave system (Anton Paar, Austria) for microwave-assisted extraction. The extraction was carried out at a power of 220 W for 12 min. After the extraction, the solution was transferred into a 10-mL conical flask and centrifuged for 5 min at 4000 rpm. The supernatant was separated and filtered with a syringe filter (0.45 μm) and transferred to another conical flask containing 5 mL deionized water where the DLLME procedure was performed. All experiments were done in triplicate.
DLLME Technique
A solution consisting of 60 μL 1-octanol (extraction solvent) and 500 μL ethanol (disperser solvent) was rapidly injected into the conical flask containing sample solution, and then the mixture was thoroughly agitated using a shaker for 2 min. In this step, Ole and HyT were inserted into fine droplets of 1-octanol. Thereafter, the cloudy solution was centrifuged for 10 min at 4000 rpm to separate the organic phase. After this process, a tiny droplet of 1-octanol was floated on the aqueous sample and 20 μL of the floated phase was injected directly into the HPLC using a Hamilton microsyringe.
Instrumentation
An Agilent 1260 infinity liquid chromatographer consisting of a vacuum degasser, an autosampler, and a quaternary pump equipped with a C18 ODS (250 × 4.6 mm, 5 μm) column from Agilent Technologies (Santa Clara, CA, USA) was applied for quantitative determination of Ole and HyT. The mobile phases used were water with glacial acetic acid (0.5%) (eluent A) and pure acetonitrile (eluent B), and the elution gradient program for the HPLC was as follows: 0–30 min, 5–20% B; 30–40 min, 20–30% B; and 40–45 min, 30–35% B. The flow rate was set at 1 mL min−1 throughout the gradient. The column temperature of 25 °C was used and the injection volume was 20 μL. The effluent was monitored at 280 nm for Ole and HyT.
Experimental Design and Statistical Analysis
In the present study, RSM based on a CCD was performed to determine the most effective independent factors in the MAE-DLLME method such as microwave power, extraction time, extraction solvent volume, and dispersive solvent volume. A four-factorial CCD was used to reach the best value of these factors on the response variable, namely, the sum of the peak area of Ole and HyT. The experimental levels and codes of variables in this study are shown in Table 1. The total experimental points (N) needed for the development of CCD are determined as N = 2f + 2f + n, where f is the number of factors that should be optimized and n is the number of central points (Habibi et al. 2013). Therefore, the employed design included 30 randomly experimental runs. The design was complete with six replicates of the central point to estimate the experimental error. Three replicate extractions and quantitative determinations were performed for each experiment. Three-dimensional surface plots were employed to demonstrate the effects of the independent variables on the response variable. The adequacy of the MAE-DLLME method was evaluated by testing for lack of fit. Significant lack of fit (p < 0.05) displays that the proposed model is inadequate and requires improving. The experimental data were analyzed with Design-Expert version 8.0.5 (Minneapolis, USA).
Analytical Figures of Merit
The calibration curves of Ole and HyT were investigated by the peak area of Ole and HyT versus its concentrations for ten points, and a coefficient of determination (R2) was represented. The limit of detection (LOD) and the limit of quantitation (LOQ) were calculated based on a signal-to-noise ratio of 3 and 10, respectively. Precision, which is a measure of repeatability, is expressed as percent relative standard deviation (RSD %) which was calculated from six analyses of the olive pomace sample. The recovery for Ole and HyT was also studied for the proposed method by comparing the amount of Ole and HyT added to the olive pomace sample with the concentration found after the procedure.
Results and Discussion
Selection of the Best Ratio of Water:Ethanol
Water:methanol and water:ethanol mixtures as extraction solvents with different ratios depending on the target analytes and sample matrix were usually applied for extraction of phenolic compounds (Pérez-Serradilla et al. 2007). The use of organic solvents such as methanol, ethanol, and acetone strongly affects the extraction efficiency and improves the recovery of target analytes from the solid or semi-solid samples, and it is noticeable that the MAE technique reduces extraction time and helps rapidly transfer the target analytes into the liquid phase when compared with routine methods (Aeenehvand et al. 2016). As shown in Fig. 1, water:ethanol (W:E) mixtures as cheap and non-toxic extraction solvents were used for extraction of Ole and HyT at different ratios. A mixture of water and ethanol as an extraction solvent has a higher extraction efficiency than water and ethanol alone in the extraction of Ole and HyT that it is in agreement with other studies (Aeenehvand et al. 2016; Pérez-Serradilla et al. 2007). It is clear that the mixture of water and ethanol had better solubility features and enhanced the release of Ole and HyT from the olive pomace sample. The highest extraction efficiency for HyT was observed at a ratio of 50W:50E, and the highest extraction efficiency for Ole was found at a ratio of 20W:80E; this is related to the difference in the chemical structure of Ole and HyT, and also, it may be because HyT is more soluble in water and polar organic solvents in comparison with Ole (Vilaplana-Pérez et al. 2014). No significant difference was observed between 50W:50E and 40W:60E for HyT and between 20W:80E and 40W:60E for Ole (Fig. 1). Based on this result, the ratio of 40W:60E was selected as the best ratio for simultaneous extraction of Ole and HyT.
Selection of Extraction and Dispersive Solvents
The selection of a suitable organic solvent is very important for efficient DLLME. In DLLME technique, a high partitioning coefficient of the target analytes in the solvent, high efficiency, low density of water, good chromatographic behavior, immiscibility with water, and high solubility in dispersive solvent are important properties in selecting an appropriate extracting solvent (Habibi et al. 2017; Kamankesh et al. 2015). Moreover, solvents with a higher density than water, the chlorinated solvents, that are used for extraction of target analytes require additional steps such as evaporation and dissolution of the residue analyte into a polar solvent while they have a non-polar nature and therefore cannot be injected into the reverse HPLC which increases the time of extraction and the risk of analyte losses (Madani-Tonekaboni et al. 2015). Based on the above considerations, 2-ethyl-1-hexanol and 1-octanol were tested as extraction solvents (Fig. 2). After the evaluation, 1-octanol was selected as the extracting solvent because it has lower density in comparison with water, high extraction capability of Ole and HyT, and good HPLC behavior. The miscibility of the dispersive solvent, both in the aqueous phase (water) and organic phase (extracting solvent), is the important factor in the selection of dispersive solvent (Madani-Tonekaboni et al. 2015; Rezaee et al. 2006). Therefore, in this study, four organic solvents including methanol, ethanol, acetonitrile, and acetone were tested to select the best dispersive solvent for the DLLME in the model sample under the same conditions. The results indicated that ethanol gave the maximum recovery (Fig. 2). Therefore, ethanol was chosen as a proper dispersive solvent. Finally, 1-octanol-ethanol as the most suitable solvent pair was selected for extraction of Ole and HyT.
RSM for the Selection of Optimum Conditions
Optimal separation and detection of Ole and HyT was obtained by optimizing four important variables such as microwave power, extraction time, volume of extraction solvent, and volume of disperser solvent in the DLLME method. RSM based on CCD was applied to optimize the DLLME process and increase the extraction efficiency. It also provides acceptable response at low analyte concentration. The results obtained from CCD were fitted to a second-order polynomial model. Equation 1 shows the RSM model in terms of coded factors:
where R is the sum of the peak areas of Ole and HyT (response) as a function of A (microwave power), B (extraction time), C (volume of extraction solvent), and D (volume of dispersive solvent).
There was a good correlation between predicted and actual responses under the studied condition which suggests good fitness of the second-order polynomial model. To evaluate the significance of each factor and interaction terms, analysis of variance was used (Table 2). Regarding the estimated p value from the ANOVA results, the effects of extraction conditions on the response were considered to be significant (p < 0.05). According to the ANOVA summary, all of the variables on the DLLME process had a significant effect on the response except D (p value < 0.05). Significant interactions have been viewed between microwave power and extraction time, microwave power and volume of extraction solvent, extraction time and volume of extraction solvent, and extraction time and volume of dispersive solvent. Other interactions were not significant (p value > 0.05).
The “Model F-value” of 23.97 implied that the model was significant with a p value less than 0.0001. The “Lack of Fit P-value” of 0.2857 implies the LOF is not significantly associated with the pure error. The regression equation obtained from the ANOVA showed that the coefficient of determination (R2) was 0.9572. This is an estimate of the fraction of the overall variation in the data accounted by the model, and thus, the model is capable of explaining 95.72% of the variation in response. The “adjusted-R2” was found to be 0.9173 that showed a good relationship between the experimental data and the fitted model, and the “predicted-R2” of 0.8149 indicated that the model had a high potential to predict the response.
Three-dimensional model graphs were applied to evaluate the interactive effect of two variables on the extraction response. Investigation of the interactive effect of microwave power vs. extraction time, volume of extraction, and dispersive solvents (Fig. 3a–c) showed that an increase of microwave power resulted in a higher response in the middle range of extraction time, volume of extraction, and dispersive solvents while the other variables were fixed in their center points. It can be explained that microwave energy leads to the breakdown of the plant cell walls and consequently results in a higher release of phenolic compounds such Ole and HyT from the plant matrices (Gögüs and Maskan 2001). The combination effect of microwave power and extraction time has shown that shorter extraction time at high microwave power seems to release and maintain Ole and HyT better than long extraction time in the middle range of microwave power (Ahmad-Qasem et al. 2013). In addition, the short time required for extraction by the microwave device can keep the phenolic content such as Ole and HyT against oxidation. High microwave power may inactivate and reduce the enzymatic oxidation caused by polyphenol oxidases, which are widely distributed among the different plant tissues (Ortega-García et al. 2008). A slight decrease in total response can be observed in the higher range of microwave power and long extraction time (Fig. 3a, d), and it may be due to the increase in the thermal degradation of phenolic compounds like Ole and HyT during the extraction procedure (Gögüs and Maskan 2001). According to Fig. 3d–f, the extraction efficiency decreased when the volumes of extraction solvent and dispersive solvent were increased to higher values, and it could be due to the decrease in concentration of Ole and HyT in 1-octanol. On the other hand, the solubility of Ole and HyT in aqueous phase may be increased by adding more than 500 μL of ethanol that eventually causes a decrease in response. Also, as shown in Fig. 3e, f, the volume of ethanol lower and higher than 500 μL reduces total response because the droplet of 1-octanol was not shaped well. It is remarkable that the volume of extraction solvent, lower than 60 μL, was difficult to handle for collecting the extraction phase. To compare the effect of variables on extraction efficiency, there is no data about the determination of Ole and HyT in olive pomace based on the MAE–DLLME–HPLC/UV method, but it was found in a study that 14 min of microwave irradiation at 200 W was sufficient for extraction of Ole and HyT from alperujo by microwave-assisted extraction coupled with liquid chromatography–tandem mass spectrometry method (Pérez-Serradilla et al. 2007).
Response surfaces using the central composite design obtained by plotting: a microwave power vs. extraction time; b microwave power vs. volume of extraction solvent; c microwave power vs. volume of dispersive solvent; d extraction time vs. volume of extraction solvent; e extraction time vs. volume of dispersive solvent; f volume of extraction solvent vs. volume of dispersive solvent (In all response surfaces, other variables are in their central points)
According to the results of the present study, the optimal experimental conditions for extraction of Ole and HyT from olive pomace samples were microwave power, 220 W; extraction time, 12 min; volume of extraction solvent, 60 μL; and volume of dispersive solvent, 500 μL.
Figures of Merit of the Proposed MAE–DLLME–HPLC/UV Methodology
To evaluate the proposed method, linearity, recovery, repeatability, limit of detection (LOD), and limit of quantification (LOQ) were studied under optimized conditions. The figures of merit for this method are comparable to other methods (Table 3). The calibration curves of Ole and HyT (standard solution) were linear over the range of 10–500,000 μg L−1 for ten points with a coefficient of determination (R2) more than 0.99 for Ole and HyT. The peak area, calculated from six replicate extractions from the olive pomace sample, was used to estimate the repeatability and was represented as relative standard deviation percentage (RSD%). As Table 3 shows, the RSD% was 5.63 for Ole and 4.12 for HyT that were more than the results reported by Pérez-Serradilla et al. (2007) with the aid of the MAE–HPLC–MS–MS method in the alperujo sample. The RSD% of HyT in virgin olive oil evaluated by USLLE–HPLC–DAD was the lowest value reported in Table 3 (Pizarro et al. 2013). The values of recovery using the proposed method were 93.4 and 98.7 for Ole and HyT, respectively, which were better than the values evaluated by SPE, LLME, and USLLE-HPLC-DAD method in the virgin olive oil (Pizarro et al. 2013). The LOD and LOQ for Ole and HyT at the optimized conditions were 35 and 100 μg L−1 for Ole and 20 and 60 μg L−1 for HyT, respectively, and these values were lower than those of the other methods (reported in Table 3), except the amounts of LOD and LOQ reported by the MAE–HPLC–MS–MS method in the alperujo sample (Pérez-Serradilla et al. 2007). These differences can be due to the type of samples, the extraction methods, and the type and conditions of the apparatus used for quantitative determination.
Application of the MAE–DLLME–HPLC/UV Methodology to Olive Pomace Samples
To evaluate the applicability of the proposed method for quantitative determination of Ole and HyT in olive pomace samples, three samples were evaluated under optimized conditions and the concentrations of the Ole and HyT in the olive pomace samples were determined by the standard addition method (Table 4). As seen in Table 4, the amount of Ole and HyT in this study is more than the Ole and HyT levels found in the previous studies which were a value of 21.30 ± 2.95 to 644 ± 35 mg kg−1 for Ole and a value of 149 ± 5 to 890.93 ± 1.75 mg kg−1 for HyT in alperujo samples (Pérez-Serradilla et al. 2007; Priego-Capote et al. 2004), but the HyT content of olive pomace sample 2 (859.52 ± 3.53 mg kg−1) is in agreement with the results reported by Pérez-Serradilla et al. (2007), who achieved a value 890.93 ± 1.75 mg kg−1 for HyT in the alperujo sample. The diversity in the amount of Ole and HyT among the olive pomace samples of this study and the other studies can be related to differences in the olive variety, origin of the olives, culture conditions, and olive oil extraction method. The average value of Ole in the olive pomace samples (from 1763.67 ± 5.03 to 3539.66 ± 4.50 mg kg−1) was lower than the levels reported in the olive leaves (23,200 ± 0.85 mg kg−1) which confirms the olive leaves as a better source of Ole in comparison with olive pomace, and conversely, the HyT content of olive pomace is higher than that of the olive leaves (Japón-Luján et al. 2006b). The mean concentrations of Ole and HyT in the olive pomace samples are higher than those reported in the olive oil by some researchers (Godoy-Caballero et al. 2013; Reboredo-Rodríguez et al. 2014), who obtained values of non-detectable to < 0.14 mg kg−1 for Ole and values of 0.31 to 29.7 mg kg−1 for HyT. These findings confirm that olive pomace (as a by-product of the olive oil industry) is a good and inexpensive source of both Ole and HyT. Figure 4 depicts the chromatograms obtained by MAE–DLLME–HPLC/UV for non-spiked (a) and spiked (b) olive pomace sample (sample 1). The concentration of Ole and HyT in the spiked sample was 100 ng g−1. The analytical results showed that MAE–DLLME–HPLC/UV is a powerful method for monitoring Ole and HyT in olive pomace samples.
Conclusion
In the present study, a new, simple, efficient, and reliable microextraction method was applied and validated to extract Ole and HyT in olive pomace samples. RSM based on CCD design was used to optimize and determine the interaction and quadratic effects of important parameters on the performance of the microextraction process. In comparison to other methods, the proposed method has significant advantages, including low consumption of solvent, good precision, low cost, repeatability, high recovery, short extraction time, and good merit figures. Finally, the proposed method is capable of extracting and determining low levels of Ole and HyT from olive pomace samples.
References
Aeenehvand S, Toudehrousta Z, Kamankesh M, Mashayekh M, Tavakoli HR, Mohammadi A (2016) Evaluation and application of microwave-assisted extraction and dispersive liquid–liquid microextraction followed by high-performance liquid chromatography for the determination of polar heterocyclic aromatic amines in hamburger patties. Food Chem 190:429–435
Ahmad-Qasem MH, Barrajón-Catalán E, Micol V, Mulet A, García-Pérez JV (2013) Influence of freezing and dehydration of olive leaves (var. Serrana) on extract composition and antioxidant potential. Food Res Int 50:189–196
Bashiry M, Mohammadi A, Hosseini H, Kamankesh M, Aeenehvand S, Mohammadi Z (2016) Application and optimization of microwave-assisted extraction and dispersive liquid–liquid microextraction followed by high-performance liquid chromatography for sensitive determination of polyamines in Turkey breast meat samples. Food Chem 190:1168–1173
Bener M, Özyürek M, Güçlü K, Apak R (2016) Optimization of microwave-assisted extraction of curcumin from Curcuma longa L. (Turmeric) and evaluation of antioxidant activity in multi-test systems. Rec Nat Prod 10:542
Dermeche S, Nadour M, Larroche C, Moulti-Mati F, Michaud P (2013) Olive mill wastes: biochemical characterizations and valorization strategies. Process Biochem 48:1532–1552
Farajzadeh MA, Asghari A, Feriduni B (2016) Development of a dispersive liquid–liquid microextraction method with a new sequence of steps and its comparison with a conventional method. J Sep Sci 39:3178–3185
Godoy-Caballero M, Acedo-Valenzuela M, Galeano-Diaz T (2013) New reversed phase dispersive liquid–liquid microextraction method for the determination of phenolic compounds in virgin olive oil by rapid resolution liquid chromathography with ultraviolet–visible and mass spectrometry detection. J Chromatogr A 1313:291–301
Gögüs F, Maskan M (2001) Drying of olive pomace by a combined microwave-fan assisted convection oven. Mol Nutr Food Res 45:129–132
Habibi H, Mohammadi A, Hoseini H, Mohammadi M, Azadniya E (2013) Headspace liquid-phase microextraction followed by gas chromatography–mass spectrometry for determination of furanic compounds in baby foods and method optimization using response surface methodology. Food Anal Method 6:1056–1064
Habibi H, Mohammadi A, Kamankesh M (2017) Hydroxymethylfurfural in fruit puree and juice: preconcentration and determination using microextraction method coupled with high-performance liquid chromatography and optimization by Box–Behnken design. J Food Meas Charact:1–9
Heleno SA, Diz P, Prieto M, Barros L, Rodrigues A, Barreiro MF, Ferreira IC (2016) Optimization of ultrasound-assisted extraction to obtain mycosterols from Agaricus bisporus L. by response surface methodology and comparison with conventional Soxhlet extraction. Food Chem 197:1054–1063
Japón-Luján R, Luque-Rodríguez J, De Castro ML (2006a) Dynamic ultrasound-assisted extraction of oleuropein and related biophenols from olive leaves. J Chromatogr A 1108:76–82
Japón-Luján R, Luque-Rodríguez J, De Castro ML (2006b) Multivariate optimisation of the microwave-assisted extraction of oleuropein and related biophenols from olive leaves. Anal Bioanal Chem 385:753–759
Kamankesh M, Mohammadi A, Hosseini H, Tehrani ZM (2015) Rapid determination of polycyclic aromatic hydrocarbons in grilled meat using microwave-assisted extraction and dispersive liquid–liquid microextraction coupled to gas chromatography–mass spectrometry. Meat Sci 103:61–67
Khajeh M, Ghanbari M (2011) Optimization of microwave-assisted extraction procedure to determine metal in fish muscles using Box–Behnken design. Food Anal Method 4:431–436
Kubo A, Lunde CS, Kubo I (1995) Antimicrobial activity of the olive oil flavor compounds. J Agr Food Chem 43:1629–1633
Le Tutour B, Guedon D (1992) Antioxidative activities of Olea europaea leaves and related phenolic compounds. Phytochemistry 31:1173–1178
Madani-Tonekaboni M, Kamankesh M, Mohammadi A (2015) Determination of furfural and hydroxymethyl furfural from baby formula using dispersive liquid–liquid microextraction coupled with high performance liquid chromatography and method optimization by response surface methodology. J Food Compos Anal 40:1–7
Malik NS, Bradford JM (2006) Changes in oleuropein levels during differentiation and development of floral buds in ‘Arbequina’ olives. Sci Hortic 110:274–278
Manna C, D'Angelo S, Migliardi V, Loffredi E, Mazzoni O, Morrica P, Galletti P, Zappia V (2002) Protective effect of the phenolic fraction from virgin olive oils against oxidative stress in human cells. J Agr Food Chem 50:6521–6526
Ortega-García F, Blanco S, Peinado MÁ, Peragón J (2008) Polyphenol oxidase and its relationship with oleuropein concentration in fruits and leaves of olive (Olea europaea) cv. ‘Picual’ trees during fruit ripening. Tree Physiol 28:45–54
Pérez-Serradilla J, Japon-Lujan R, de Castro ML (2007) Simultaneous microwave-assisted solid–liquid extraction of polar and nonpolar compounds from alperujo. Anal Chim Acta 602:82–88
Pizarro ML, Becerra M, Sayago A, Beltrán M, Beltrán R (2013) Comparison of different extraction methods to determine phenolic compounds in virgin olive oil. Food Anal Method 6:123–132
Priego-Capote F, Ruiz-Jiménez J, de Castro ML (2004) Fast separation and determination of phenolic compounds by capillary electrophoresis–diode array detection: application to the characterisation of alperujo after ultrasound-assisted extraction. J Chromatogr A 1045:239–246
Ramezani H, Hosseini H, Kamankesh M, Ghasemzadeh-Mohammadi V, Mohammadi A (2015) Rapid determination of nitrosamines in sausage and salami using microwave-assisted extraction and dispersive liquid–liquid microextraction followed by gas chromatography–mass spectrometry. Eur Food Res Technol 240:441–450
Reboredo-Rodríguez P, Rey-Salgueiro L, Regueiro J, González-Barreiro C, Cancho-Grande B, Simal-Gándara J (2014) Ultrasound-assisted emulsification–microextraction for the determination of phenolic compounds in olive oils. Food Chem 150:128–136
Rezaee M, Assadi Y, Hosseini M-RM, Aghaee E, Ahmadi F, Berijani S (2006) Determination of organic compounds in water using dispersive liquid–liquid microextraction. J Chromatogr A 1116:1–9
Rodrigues F, Pimentel FB, Oliveira MBP (2015) Olive by-products: challenge application in cosmetic industry. Ind Crop Prod 70:116–124
Rombaut N, Tixier AS, Bily A, Chemat F (2014) Green extraction processes of natural products as tools for biorefinery. Biofuels Bioprod Biorefin 8:530–544
Romero-García J, Niño L, Martínez-Patiño C, Álvarez C, Castro E, Negro M (2014) Biorefinery based on olive biomass. State of the art and future trends. Bioresour Technol 159:421–432
Sereshti H, Ahmadvand M, Asgari S (2014) A rapid quantification of β-carotene in fruits and vegetables by dispersive liquid–liquid microextraction coupled with UV–vis spectrophotometry: optimized by response surface methodology. Food Anal Method 7:1481–1488
Tripoli E, Giammanco M, Tabacchi G, Di Majo D, Giammanco S, La Guardia M (2005) The phenolic compounds of olive oil: structure, biological activity and beneficial effects on human health. Nutr Res Rev 18:98–112
Vilaplana-Pérez C, Auñón D, García-Flores LA, Gil-Izquierdo A (2014) Hydroxytyrosol and potential uses in cardiovascular diseases, cancer, and AIDS. Front Nutr 1:1–11
Yang P et al (2017) Dispersive liquid-liquid microextraction method for HPLC determination of phenolic compounds in wine. Food Anal Method:1–15
Acknowledgements
This study has been supported by the Faculty of Nutrition Sciences and Food Technology, Shahid Beheshti University of Medical Sciences, Tehran, Iran. We gratefully appreciate their assistance. We also appreciate the “Student Research Committee” and “Research & Technology Chancellor” of Shahid Beheshti University of Medical Sciences for their support of project NO 1395/78295 from the Student Research Committee, Shahid Beheshti University of Medical Sciences, Tehran, Iran.
Author information
Authors and Affiliations
Corresponding author
Ethics declarations
Conflict of Interest
Hossein Habibi declares that he has no conflict of interest. Abdorreza Mohammadi declares that he has no conflict of interest. Mehdi Farhoodi declares that he has no conflict of interest. Sahar Jazaeri declares that she has no conflict of interest.
Ethical Approval
This article does not contain any studies with human participants or animal performed by any of the authors.
Rights and permissions
About this article
Cite this article
Habibi, H., Mohammadi, A., Farhoodi, M. et al. Application and Optimization of Microwave-Assisted Extraction and Dispersive Liquid–Liquid Microextraction Followed by High-Performance Liquid Chromatography for the Determination of Oleuropein and Hydroxytyrosol in Olive Pomace. Food Anal. Methods 11, 3078–3088 (2018). https://doi.org/10.1007/s12161-018-1279-x
Received:
Accepted:
Published:
Issue Date:
DOI: https://doi.org/10.1007/s12161-018-1279-x