Abstract
Soil degradation by salinity and accumulation of trace elements such as cadmium (Cd) in the soils are expected to become one of the most critical issues hindering sustainable production and feeding the increasing population. Biochar (BC) has been known to protect the plants against soil salinity and heavy metal stress. A soil culture study was performed to evaluate the effect of BC on wheat (Triticum aestivum L.) growth, biomass, and reducing Cd and sodium (Na) uptake grown in Cd-contaminated saline soil under ambient conditions. Soil salinity decreased the plant growth, biomass, grain yield, chlorophyll contents, and gas exchange parameters and caused oxidative stress in plants compared with Cd stress alone. Salt stress increased Cd and Na uptake and reduced the potassium (K) and zinc (Zn) uptake by plants. AB-DTPA-extractable Cd and soil electrical conductivity (ECe) increased under salt stress compared to the soil without NaCl stress. Biochar application improved the plant growth and reduced the Cd and Na uptake except in plants treated with higher BC and salt stress (5.0% BC + 50 mM NaCl). Biochar application reduced the oxidative stress in plants and modified the antioxidant enzyme activities, and reduced the bioavailable Cd under salt stress. The positive effects of BC under lower salt stress while the negative effects of BC under higher BC and salt levels indicated that BC doses should be used with great care in higher soil salinity levels simultaneously contaminated with Cd to avoid the negative effects of BC on growth and metal uptake.
Similar content being viewed by others
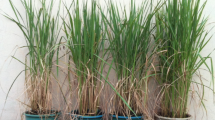
Explore related subjects
Discover the latest articles, news and stories from top researchers in related subjects.Avoid common mistakes on your manuscript.
Introduction
Deterioration of the environment has become an emerging constraint to crop production worldwide (Nagajyoti et al. 2010; Murtaza et al. 2015). Crop productivity is seriously affected by abiotic stresses such as soil salinity and heavy metal contamination (Abbasi et al. 2016; Rizwan et al. 2016a). Cadmium (Cd) is among the most toxic environmental pollutants for living things which enters in agricultural soils mainly through anthropogenic activities such as use of phosphate fertilizers, industrial activities, and application of sewage sludge (Nagajyoti et al. 2010; Murtaza et al. 2015). Cadmium could easily transfer to the food chain via plants due to its high mobility in the environment which can cause serious disorders in living things (Gallego et al. 2012). Previous studies have revealed that Cd is highly toxic to plants and inhibits the plant growth and even plant death occurs (Rizwan et al. 2012, 2016a). Cadmium toxicity has been shown to reduce the photosynthesis and mineral nutrition in plants (Rizwan et al. 2016b). In addition, Cd induces oxidative stress in plants by enhancing the production of reactive oxygen species (ROS) including malondialdehyde (MDA), electrolyte leakage (EL), and hydrogen peroxide (H2O2) ((Nagajyoti et al. 2010; Gallego et al. 2012). The overproduction of ROS may damage the biological macromolecules and disorder the antioxidant system in plants.
On the other hand, about 800 million hectares of land are affected by salinity worldwide (Munns and Tester 2008). Agricultural soils are degraded by salinity mainly due to irrigation with wastewater, sewage sludge, phosphate fertilizers, and biosolids (Abbasi et al. 2016; Rehman et al. 2016). Salt stress has been shown to decrease plant growth, biomass, and mineral nutrient uptake (Hussain et al. 2015). Similarly, salt stress decreased potassium (K) and calcium (Ca) concentrations in wheat seedlings (Azeem et al. 2015). Salinity caused oxidative stress in wheat and reduced the antioxidant enzyme activities (Khaliq et al. 2015; Mohamed et al. 2017).
Wheat (Triticum aestivum L.) is an important part of human diet and is the staple food for most of the world population. Wheat production was reported about 318 and 713 MT in Asia and the world, respectively, in 2013. In Pakistan, wheat production was about 24.23 MT from an area of 8.69 million hectares in 2013, which ranked Pakistan among the top ten wheat-producing countries (FAO 2014). A large number of reports highlighted that Cd may accumulate in wheat and could transfer to the food chain via the consumption of wheat-derived food products (Naeem et al. 2015; Rehman et al. 2015). In addition, wheat may also grow in marginal soils to feed the increasing population and is irrigated with sewage water due to the shortage of good-quality irrigation (Rehman et al. 2015). Under real field conditions, soils mainly receiving wastewater or sewage sludge are often subjected to multiple stresses such as salinity and heavy metals (Bauddh and Singh 2012). Saline soils have low fertility and have the high bioavailability of heavy metals (Rady et al. 2016). The presence of heavy metal and salinity in the soil simultaneously has negative effects on the crops compared to these stresses alone (Shafi et al. 2009; Zhang et al. 2016). For example, the application of NaCl and Cd individually as well as in combination decreased the growth and chlorophyll and relative water contents in plants (Garg and Chandel 2012). Both stresses, NaCl and Cd, decreased the plant height, root length, MDA content, and activities of antioxidant enzymes in wheat than either NaCl or Cd treatments alone (Shafi et al. 2009). Application of NaCl on the leaves increased the Cd accumulation in durum wheat compared to the control (Ozkutlu et al. 2007). Similarly, halophyte species Carpobrotus rossii accumulated higher Cd concentration in shoots in the presence of salt stress compared to either salt or Cd stress alone (Zhang et al. 2016). The combined stress of NaCl and Cd increased the MDA contents in maize seedlings compared to the relative Cd or Na stress alone (Abbasi et al. 2016).
Different strategies have been developed to reduce the toxic effects of heavy metals and salt stresses in plants (Adrees et al. 2015; Rizwan et al. 2015, 2016a). The application of biochar (BC), a pyrolysed organic material under limited supply of oxygen, as a soil amendment is currently gaining considerable interest worldwide (Ok et al. 2015; Rizwan et al. 2016c). A number of studies have reported the positive effects of BC under either heavy metal (Younis et al. 2016; Abbas et al. 2017) or salt stress (Akhtar et al. 2015a). The addition of BC in the soil increased the soil pH and decreased the bioavailability and uptake by plants (Zhang et al. 2016). It has been reported that BC was more effective in reducing Cd uptake by wheat plants compared to other organic amendments (Yousaf et al. 2016). Similarly, BC application increased potato growth, photosynthesis, and yield under salt stress while it decreased the Na+ and increased the K+ content in the xylem (Akhtar et al. 2015a). The BC in combination with plant growth-promoting bacteria increased maize growth and biomass and decreased the Na+ and increased the K+ content in xylem sap of maize (Akhtar et al. 2015b). Biochar application reduced the oxidative stress and increased the bean growth under salt stress (Farhangi-Abriz and Torabian 2017). Subsequently, little information is available in the literature regarding the effect of the BC amendment on Cd uptake in wheat grown in saline soil despite the fact that wheat is facing both Cd and salt stresses simultaneously. We hypothesized that BC may alleviate Cd stress in wheat by reducing Cd bioavailability in the saline soil and its uptake by plants. Thus, the aim of the present study was to understand the effect of BC on wheat growth, biomass, Cd, and other mineral (Na, K, and Zn) uptake under the combined Cd and salt stress and determine the suitability of BC for reducing Cd and salt stress in wheat.
Materials and methods
Material collection and characterization
Soil used in the present study was sampled from an agricultural field in Multan, Pakistan (30° 12′ N, 71° 28′ E, and 215 m above sea level). The soil used is under crop cultivation especially wheat, and farmers use mainly raw city effluent due to shortage of canal water for irrigation. As a result, soil has been polluted with toxic heavy metals mainly Cd because the soil has been receiving raw city effluent since more than 20 years. Soil is a sandy loam, alkaline in nature, and free of carbonates. Soil samples were collected from the surface (0–20 cm) with the help of a stainless steel spade, air-dried under shade, ground to a small size, and sieved through a 2-mm sieve. Detailed information on the characterization methods and soil properties is available in Rehman et al. (2015). In brief, total metal contents in the soil were measured by using 1.0 g of air-dried soil in 10 ml of concentrated NHO3 and the flasks were kept overnight. After this, the solution was heated to 200 °C and the samples cooled, and then again, 1.0 ml of HNO3 and 4 ml of HClO4 were added to the mixture and heated to 200 °C. Samples were removed from the hot plate when the fumes of HClO4 appeared and the samples were cooled, and 1:10 HCl was added and the samples heated to 70 °C for 1 h and again allowed to cool; the final volume of 50 ml was made with 1.0% HCl, and samples were filtered with paper (Whatman filter paper No. 42) (Amacher 1996). The Bouyoucos (1962) method was used to measure the soil particle size, and pH of the soil-saturated paste was measured by using a pH meter (Jenway, Model 671P). The electrical conductivity of the soil-saturated paste extract (ECe) and soluble ions and sodium adsorption ratio (SAR) were measured by standard methods (US Salinity Lab. Staff 1954; Page et al. 1982). For determination of bioavailable metal concentrations in the soil, 10 g air-dried soil was extracted with ammonium bicarbonate diethylenetriaminepentaacetic acid (AB-DTPA, pH 7.6) solution (Soltanpour 1985). The selected soil physicochemical properties are reported in Table 1.
The BC used in the experiment was prepared from rice straw as feedstock. Rice straw was collected from an agricultural field located in the suburb of Multan, Pakistan, air-dried, and then ground to pass a 10-mesh sieve. The BC was prepared by the method described in Qayyum et al. (2015). In brief, laboratory designed scale stainless-steel vertical silo type furnace (10 kg capacity) equipped with a gas burner was used for the pyrolysis of the material. The fire was not in direct contact with the material, and both the time and temperature were noted during the preparation (450 °C for 2 h), and then the material was allowed to cool in the furnace. The BC was characterized for different physicochemical properties; details of BC characterization can be found in Qayyum et al. (2015). In brief, the volatile content and total ash of the BC were determined by burning the BC in the muffle furnace at 450 and 550 °C, respectively. The pH and EC of BC (1:20, w/v, weight to distilled water ratio) were measured using pH and EC meters. An elemental analyzer (Elementar, Germany) was used to measure total hydrogen, carbon, and nitrogen. Phosphorus (P), potassium (K), and sodium (Na) concentrations were measured using a spectrophotometer and flame photometer, respectively, after digesting the BC samples in di-acid (HNO3/HClO4) mixture. The BC has ash content, volatile matter, EC, and pH values of 22.5%, 24%, 2.4 dS m−1, and 10.0, respectively. The values of carbon, nitrogen, P, K, and Na in BC were 42.3, 1.5, 0.3, 2.54, and 1.1%, respectively. The BET surface area of the BC was 2.51 m2/g.
Experimental design
A pot culture experiment was conducted in the wirehouse of the Government College University, Faisalabad, Pakistan, during Rabi season from November 2015 to April 2016 under ambient conditions. Each plastic pot (height 20 cm, top and bottom diameter of 18 and 15 cm, respectively) was filled with 3 kg of air-dried soil. Biochar was added at doses of 0, 3.0, and 5.0% w/w in the soil and thoroughly mixed and then incubated for 2 weeks at 40% of soil water holding capacity. Before sowing, wheat (Lasani-2008) seeds were sterilized with H2O2 (10%, v/v) solution for 15 min and then thoroughly washed with distilled water. The selected wheat variety has the ability to accumulate low Cd concentration in shoots and has the higher metal tolerance ability (Naeem et al. 2016). Initially, 15 seeds per pot were sown, replicated three times by following a completely randomized design. After 7 days of germination, ten plants in each pot were maintained. After 45 days of sowing, the seedlings were subjected to three (0, 25, and 50 mM NaCl) levels of saline irrigation treatments. The salinity levels were developed by adding NaCl in water having EC values of 0.3 dS m−1. Total volume of water used was 2.4 l in each pot which was obtained by adding 400 ml of saline or non-saline water two times in a week with a total of six saline irrigations during 3 weeks. Saline irrigations were stopped as there was stunted plant growth in pots treated with 5.0% BC + 50 mM NaCl stress. In total, there were nine treatments in the completely randomized design with three replicates of each treatment. Treatment without application of BC and salinity was considered as control (0 mM NaCl + 0% BC).
Each pot was fertilized with NPK fertilizers at 120–50–25 kg ha−1 as urea, DAP, and K2SO4. Total doses of PK and half of N were applied by mixing in 500 ml of water for each pot before wheat sowing while the remaining half of N was added after 35 days of sowing. Soil moisture content in pots was maintained at about 70% of soil water holding capacity, and weeds were removed regularly.
Plant harvesting and biomass determination
The plant was harvested at physiological maturity (after 130 days of germination) and separated into roots, shoots, and spikes. Both shoot and spike lengths were measured with a ruler. Then, plant parts were washed thoroughly with tap water following washing with distilled water. The samples of roots were washed with 1% HCl and then thoroughly washed with distilled water. All the samples were separated into different parts such as grains, husks, shoots, and roots; oven was used to dry the samples at 70 °C till constant weight, and dry biomass was determined for each part separately, ground, and stored for further analysis.
Photosynthetic pigments and gas exchange parameters
Chlorophyll contents and gas exchange parameters were measured after 65 days of sowing by uprooting one plant from each treatment and replicate. Chlorophyll contents were measured using a spectrophotometer (Halo DB-20/DB-20S, Dynamica Company, London, UK) at 470, 647, and 664.5 nm after extracting the samples with 85% (v/v, Sigma) acetone in the dark at 4 °C for 24 h and then centrifugation at 4000×g for 10 min (Lichtenthaler 1987). Gas exchange parameters including stomatal conductance (Gs), photosynthetic rate (Pn), transpiration rate (Tr), and water use efficiency (WUE) were determined using a portable infrared gas analyzer (IRGA) (Analytical Development Company, Hoddesdon, England). These parameters were measured between 10:00 a.m. to 11:00 a.m. when the plants were fully functional.
Determination of EL, MDA, and H2O2 contents
After 65 days of sowing, EL in shoots was determined by uprooting one plant from each treatment and replication. The samples were cut into pieces of small size and placed vertically in the tubes with 8 ml of deionized water, and the initial EC of the solution was determined after incubating the tubes for 2 h at 32 °C. The samples were again autoclaved for 20 min at 121 °C, final EC of the solution was determined after cooling the sample to 25 °C, and then EL was determined using the following equation (Dionisio-Sese and Tobita 1998).
Malondialdehyde contents were estimated according to the method described by Heath and Packer (1968) with little changes done in the latter (Dhindsa et al. 1981; Zhang and Kirkham 1994). Briefly, in the 0.25 g fresh sample, 5.0 ml of 0.1% thiobarbituric acid (TBA) was added. The supernatant was collected after centrifuging the aliquot for 10 min at 12,000×g under 4 °C. Then, 4 ml of 20% TCA (containing 0.5% thiobarbituric acid) was added in 1 ml of the supernatant and put in boiling water (95 °C) for 30 min, and then the solution was rapidly cooled down in an ice bath and centrifuged at 10,000×g for 10 min. The absorbance of the samples was taken at 532 nm, and the value for non-specific absorbance at 600 nm was subtracted, then MDA contents were calculated by using an extinction coefficient of 155 mM−1 cm−1.
For the measurement of H2O2, phosphate buffer (50 mM, pH 6.5) solution (3 ml) was added in 50 mg leaf samples to make a homogeneous mixture, and then the mixture was centrifuged for 30 min at 6000×g under 4 °C. Then, 1 ml of 0.1% titanium sulfate in 20% (v/v) H2SO4 was mixed in the extracted solution and the mixture was again centrifuged at 6000×g for 20 min in a controlled temperature of 4 °C. The absorption of the supernatant was measured at 410 nm. The extinction coefficient of 0.28 μmol−1 cm−1 was used to calculate H2O2 contents.
Determination of antioxidant enzymes
Activities of superoxide dismutase (SOD), peroxidase (POD), catalase (CAT), and ascorbate peroxidase (APX) were determined spectrophotometrically. For this, mortar and pestle was used to ground the shoot samples in liquid nitrogen. Phosphate buffer (0.05 M, pH 7.8) was used to standardize the samples and filtered and then the solution was centrifuged for 10 min at 12,000×g under 4 °C, and the supernatant was collected and used for further analyses. The activities of SOD and POD were determined following the method of Zhang (1992).
The CAT activity was assessed following the method of Aebi (1984). Briefly, 100 μl enzyme extract, 100 μl H2O2 (300 mM), and 2.8 ml 50 mM phosphate buffer with 2 mM CA (pH 7.0) were added in 3 ml of assay mixture. The decrease in absorbance at 240 nm was measured to calculate the CAT activity. For the determination of APX activity, 100 μl enzyme extract, 100 μl ascorbate (7.5 mM), 100 μl H2O2 (300 mM), and 2.7 ml 25 mM potassium phosphate buffer with 2 mM CA (pH 7.0) were added in the reaction mixture. The ascorbate oxidation activity was measured by determining at 290 nm (ε = 2.8 mM−1 cm−1) (Nakano and Asada 1981).
Metal content analysis in plants
The plant samples (1 g each) were digested in 10 ml of HNO3·HClO4 (3:1, v/v) in a conical flask and kept overnight and then placed on a hot plate after adding 5 ml more HNO3 until a clear solution was obtained (Ryan et al. 2001). An atomic absorption spectrophotometer (Thermo Electron S series) was used for the determination of metal contents in the plant digests. Shoots and roots and Na and K concentrations were determined by using a flame photometer after dissolving the ashed samples in HNO3.
Soil sampling and analyses
Soil samples were taken at the end of the experiment and oven-dried at 40 °C until constant weight and sieved through a 2-mm sieve. AB-DTPA-extractable metals in the soil, soil EC, and soil pH were measured as described in Material collection and characterization.
Statistical analyses
One-way ANOVA was used to statistically analyze the data at 5% probability level using IBM SPSS Statistics for Windows, Version 21.0. (IBM Corp, Armonk, NY). Where significant, the multiple comparisons of means were made using Tukey’s HSD post hoc test. Two-way ANOVA was used to analyze the combined effect of BC and salinity. Pearson correlation coefficients between some of the variables were done using the above said statistical program.
Results
Plant growth and grain yield
Data related to plant growth, biomass, and grain yield are presented in Figs. 1 and 2. Shoot and spike lengths of wheat (Fig. 1) as well as dry biomass of roots, shoots, spikes, and grains decreased with increasing doses of NaCl in the soil (Fig. 2). Shoot and spike lengths of 50 mM NaCl treatment decreased respectively by 19.5 and 28% compared to the control (0 mM NaCl + 0% BC). Biochar soil amendment increased the shoot and spike lengths in a dose-additive manner, except in the treatment with 5.0% BC + 50 mM NaCl stress, compared to the same NaCl treatment without BC supply. The maximum significant increase in shoot and spike length (approximately 20 and 23% in shoots and spike, respectively) was observed in 5.0% BC treatment compared to the control (Fig. 2a, b). Dry weights of roots, shoots, spikes, and grains significantly (p < 0.05) decreased with increasing salinity levels in the soil (Fig. 2). At 50 mM NaCl treatment, the decrease in roots, shoots, spikes, and grain weights was about 18, 22, 25, and 25.5% compared to the control, respectively. Biochar soil usage significantly increased the dry weights of different parts of wheat plants in a dose-additive manner compared to the same NaCl treatment without BC application. The maximum significant increase in root, shoot, spike, and grain biomass was also observed in 5.0% BC supply, which was approximately 42, 12, 31, and 28% compared to the control, respectively. However, there was not a significant increase (root biomass) or decrease (shoot and grain biomass) in dry weights at 5.0% BC + 50 mM NaCl compared to the same salt stress alone.
Shoot length (a), spike length (b), root biomass (c), shoot biomass (d), spike biomass (e), and grain biomass of wheat (f) grown in a historically Cd-contaminated soil and treated with increasing doses of biochar and salinity. Values are means ± SD (n = 4). Different lowercase letters indicate a significant difference among biochar treatments at p < 0.05
Chlorophyll contents and gas exchange parameters
Chlorophyll a and chlorophyll b concentrations measured in leaves significantly decreased with increasing salinity levels in the soil (Fig. 3a, b). The maximum decrease in both chlorophyll a and b was about 25 and 38% in 50 mM NaCl compared to the control, respectively. Biochar application significantly (p < 0.05), except at 5.0% BC + 50 mM NaCl, increased the chlorophyll contents compared to the control and NaCl treatments without BC application. The maximum chlorophyll a and b contents were observed in 5.0% BC treatment, which was about 76 and 67% compared to the control. Salt stress decreased Pn, Gs, Tr, and WUE compared to the control (Fig. 3c–f). Biochar application significantly affected these gas exchange parameters compared to the respective treatments without BC supply. The maximum increase in Pn, Gs, Tr, and WUE was observed in 5.0% BC which was about 56, 51, 94, and 54% compared to the control, respectively.
Chlorophyll contents (a, b) and gas exchange parameters (c–f) of wheat grown in a historically Cd-contaminated soil and treated with increasing doses of biochar and salinity. Values are means ± SD (n = 4). Different lowercase letters indicate a significant difference among biochar treatments at p < 0.05
Oxidative stress and antioxidant enzymes
Data for oxidative stress (MDA, EL, and H2O2) and antioxidant enzymes (SOD, POD, and CAT) in leaves are illustrated in Fig. 4. NaCl treatments caused an increase in MDA, EL, and H2O2 contents in leaves compared to the control (0% BC + 0 mM NaCl) treatment. The maximum increase in MDA, EL, and H2O2 (approximately 32, 20, and 23.4% compared to the control, respectively) contents was observed in 50 mM NaCl treatment. Biochar usage significantly decreased MDA, EL, and H2O2 contents compared to the same NaCl treatment without BC application (Fig. 4). The lowest concentration of MDA, EL, and H2O2 was observed in 5.0% BC supply. Salt stress decreased SOD and CAT activities and enhanced POD activity compared to the control. Biochar application reduced POD activity and enhanced the SOD and CAT activities compared to the same NaCl treatments without BC usage (Fig. 4d–f).
Concentrations of malondialdehyde (a), electrolyte leakage (b), and hydrogen peroxide (c) and antioxidant enzyme activities (superoxide dismutase (SOD), peroxidase (POD), and catalase (CAT)) (d–f) in leaves of wheat grown in a historically Cd-contaminated soil and treated with increasing doses of biochar and salinity. Values are means ± SD (n = 4). Different lowercase letters indicate a significant difference among biochar treatments at p < 0.05
Metal distribution in plants
The Cd concentration increased in shoots, roots, and grains with increasing salinity levels in the soil (Fig. 5a–c). The maximum increase in Cd concentration was observed in these plant parts treated with 50 mM NaCl, which was about 29, 36, and 44% in shoots, roots, and grains compared to the control, respectively. Exogenous application of BC significantly (p < 0.05) decreased Cd concentration in these plant parts compared to the respective treatments without BC application. The lowest Cd concentration in shoots, roots, and grains was observed in 5.0% BC treatment, which was about 50, 48, and 40% compared to the control, respectively. Salt stress caused a significant reduction in Zn concentration in shoots, roots, and grains of wheat in a dose-additive manner (Fig. 5d–f). At 50 mM NaCl stress, the reduction in shoot, root, and grain Zn concentration was about 26, 30, and 44% compared to the control, respectively. Biochar soil amendment increased Zn concentration in different plant parts with a significant (p < 0.01) increase in 5.0% BC application under different salinity levels compared to the respective salinity treatments without BC supply. Cadmium concentration in grains was positively correlated to Cd concentrations in shoots and roots while it was negatively correlated to Zn concentrations in shoots and roots (Table S1). Salt stress significantly (p < 0.05) increased Na concentrations and decreased K and K/Na ratio in shoots and roots compared to the control (Table 2). Biochar soil application significantly increased K and the K/Na ratio while it decreased Na concentrations in shoots and roots in a dose-additive manner. The maximum K and minimum Na concentrations were observed in 5.0% BC treatment while the opposite trend was observed in 50 mM NaCl stress.
Cadmium (Cd) and zinc (Zn) concentrations in shoot, roots, and grains of wheat grown in a historically Cd-contaminated soil and treated with increasing doses of biochar and salinity. Values are means ± SD (n = 4). Different lowercase letters indicate a significant difference among biochar treatments at p < 0.05
Post-harvest soil properties and available Cd contents
Results related to post-harvest soil pH, EC, and AB-DTPA-extractable Cd are presented in Table 3. Salinity increased the soil pH compared to the control, and BC application further increased the soil pH compared to the respective treatments without BC application. Salinity significantly (p < 0.05) increased EC of soil saturated paste. As compared to the control, BC application increased EC of soil without salt stress. However, BC decreased EC of soil under 25 mM salt stress compared to the respective treatment without BC supply. Compared to 50 mM salt stress, 3.0% BC + 50 mM NaCl application decreased and 5.0% BC + 50 mM NaCl increased the EC of the soil. Salinity increased AB-DTPA-extractable Cd in the soil with a maximum bioavailable Cd at 50 mM salt stress. Biochar soil application significantly decreased extractable Cd in the soil compared to the respective treatments without BC application. The minimum Cd concentration was observed in 5.0% BC treatment (Table 3), and Cd concentration in the soil was negatively correlated to the soil pH (Table S1).
Discussion
In the present study, wheat growth and biomass were lower in the control (0% BC + 0 mM NaCl) and salt stress further decreased the plant growth and biomass (Figs. 1 and 2). The combined effect of NaCl and Cd on plant height and root length was larger than both NaCl and Cd treatments alone (Shafi et al. 2009). Abbasi et al. (2015) reported that Cd + NaCl decreased or increased maize growth and biomass, depending upon studied cultivars, compared to Na and Cd treatments alone. Decrease in wheat growth and biomass under Cd and salt stress might be due to the severe structural disorganization of different plant parts such as nucleus, chloroplast, and mitochondrial damage (Abbasi et al. 2015). The decrease in root biomass with salinity stress might be due to the accumulation of Na+ ions in the salinized soil (Akhtar et al. 2015a). Biochar soil usage increased the plant growth and biomass, except at 5.0% BC + 50 mM NaCl, compared to the respective treatments without BC (Fig. 2). Under salt stress, increasing trends of plant growth and biomass with BC application rates have been reported in wheat (Lashari et al. 2013; Akhtar et al. 2015c), maize (Akhtar et al. 2015b), and potato (Akhtar et al. 2015a). Similarly, several studies showed the growth and biomass improvement with BC under Cd stress (Younis et al. 2016; Yousaf et al. 2016). The increase in plant biomass and yield with BC under stressful conditions might be due to the improvement in soil physical and chemical properties under salt stress (Lashari et al. 2013) or Cd stress (Yousaf et al. 2016). However, no studies reported the effects of BC under combined Cd and salt stress. The increase in wheat growth and biomass under combined Cd + salt stress might be due to the reduction of Na and Cd while it increased K and Zn concentrations in different plant parts (Fig. 5, Table 2) which reduced the oxidative stress (Fig. 4) and improved the photosynthesis (Fig. 3). However, reduction in plant biomass and yield at 5.0% BC + 50 mM salt stress might be due to the higher EC values (Table 3).
Combined salinity and Cd stress decreased the chlorophyll contents and gas exchange attributes, and BC application increased these plant parameters either Cd stress alone or combined BC + salt stress compared to the respective treatments without BC supply (Fig. 3). The increase in chlorophyll contents, Pn, and Gs in leaves with BC application under salt stress has been reported in many plant species such as potato (Akhtar et al. 2015a), maize (Akhtar et al. 2015b), and wheat (Akhtar et al. 2015c). Similarly, BC application increased photosynthesis in plants under Cd stress (Younis et al. 2016; Yousaf et al. 2016). The increase in photosynthesis with the BC application under salt and Cd stress might be due to the ultra-structural improvement of wheat seedlings as Cd and salt stress caused severe disorganization of the chloroplast (Abbasi et al. 2015).
Oxidative stress affects many cellular functions in plants by causing lipid peroxidation and damaging nucleic acids (Schutzendubel and Polle 2002). In contrast, this ROS production in plants is scavenged by key antioxidant enzymes present in plants such as SOD, POD, and CAT. For example, SOD converts O2•− to H2O2, CAT directly converts H2O2 to H2O and O2, and POD plays a role in catalyzing H2O2. Thus, in this way, ROS detoxification occurs in plants and overall a balance is required between production and degradation of ROS for normal plant functions (Schutzendubel and Polle 2002). However, under stressful conditions, plants fail to detoxify the ROS and as a result, oxidative stress occurs in plants (Abbasi et al. 2016). In the present study, salinity has increased EL, MDA, and H2O2 contents and POD activity while it decreased SOD and CAT activities in leaves of wheat seedlings compared to the control (Fig. 4). This increase of oxidative stress and reduced SOD and CAT activities under salinity might indicate that salt stress severely damaged the plant antioxidative system. Mandhania et al. (2006) reported that salt stress increased the activities of CAT, POD, and ascorbate peroxidase (APX) and declined the SOD activity in two cultivars of wheat. Published literature reported that salt stress increased MDA contents and CAT and POD activities in maize leaves in combined NaCl and Cd stress compared to the control and respective treatments alone (Abbasi et al. 2016). Salt stress increased MDA contents in Ricinus communis and Brassica Juncea, and the response depends upon the species and the duration of the growth period (Bauddh and Singh 2012). Furthermore, the combined effect of NaCl and Cd on MDA and activities of antioxidant enzymes in wheat plants was larger than both NaCl and Cd treatments alone (Shafi et al. 2009). Salt stress increased the MDA, H2O2, O2•−, SOD, POD, and CAT activities in shoots and roots of bean in a dose-additive manner compared to the control (Farhangi-Abriz and Torabian 2017). Overall, the increase or decrease in antioxidant enzyme activities in plants might be due to variation in plant species and/or cultivars within the same species. The combined BC + NaCl usage increased SOD and CAT activities in leaves and decreased the POD activity as well as EL, MDA, and H2O2 contents compared to the respective salinity treatments alone (Fig. 4). Previous study showed that BC + salt treatment decreased the MDA and H2O2 contents and antioxidant enzyme activities compared to the plants under salt stress alone (Farhangi-Abriz and Torabian 2017). Biochar application decreased MDA contents in spinach under Cd stress (Younis et al. 2016). Overall, in the present study, the reduction in oxidative stress might be due to decrease in Cd and Na concentrations in different plant parts (Fig. 5, Table 3).
Salinity increased Cd and Na and decreased Zn and K concentrations in different plant parts (Fig. 5, Table 2). Salinity stress caused a significant increase in Cd concentrations in two cultivars of edible amaranth (Xu et al. 2017). Salinity enhanced the Cd and Na uptake by soybean and their accumulation to grains (Ashrafi et al. 2014). Salinity increased Cd accumulation in B. juncea and R. communis compared to the Cd treatment alone (Bauddh and Singh 2012). The increase in Cd uptake under salt stress might be due to salinity-induced osmoregulation which triggered the biogeochemical processes involved in Cd mobilization in the rhizosphere soil as well as Cd absorption and translocation by plants (Xu et al. 2017). Salt stress decreased K and increased Na concentrations in wheat leaves depending upon salt-tolerant and salt-sensitive cultivars (Cheng et al. 2015). Salt stress increased Na and K concentrations in R. communis and B. juncea while the response varied with the species and the duration of the growth period (Bauddh and Singh 2012). Salt stress increased Zn concentration in Spartina densiflora, a hylophyte species, grown in hydroponic conditions (Redondo-Gómez et al. 2011). Salt stress decreased Zn concentration in wheat seedlings in a dose-additive manner while Zn application increased the Zn concentration in wheat seedlings (Saeidnejad et al. 2016). Increasing NaCl stress caused a significant reduction in dissolved organic carbon and significantly increased the concentrations of Cu, Zn, and Cd both in the rhizosphere and fruit/leaf of radish (Ondrasek et al. 2012). The increased Cd accumulation in C. rossii might be due to increased ionic strength in the soil, which may increase Cd solubility in the soil (Zhang et al. 2016). The salt-induced higher Cd accumulation might be due to the higher accumulation of Cl by plants, which may mobilize the shoot Cd and increase its transfer to grains (Ozkutlu et al. 2007). In the present study, higher Cd concentrations in the plants correlated with Cd bioavailable concentrations in the soil (Table S1), which indicated that higher Cd under salt stress might be due to higher bioavailable Cd concentrations in the soil (Table 3). The decrease in Zn uptake by plants under salt stress (Fig. 5d–f) might be due to increase in bioavailable Cd in the soil (Table 3) which may decrease Zn uptake by plants due to competition between Cd and Zn at the root surface during uptake by the roots (Rizwan et al. 2016a).
Biochar soil amendments decreased Cd and Na and increased Zn and K concentrations in different plant parts compared to the respective treatments without BC supply (Fig. 5, Table 2). Biochar usage decreased Na and increased K contents in wheat leaves under salt stress (Akhtar et al. 2015c). The BC application increased P, K, and S concentrations in leaves of Acacia mearnsii grown in saline sodic soil (Drake et al. 2016). The increase in K concentration with BC might enhance salt tolerance in wheat plants (Cheng et al. 2015). Reduced Na uptake in BC-treated plants might be due to higher Na adsorption by BC which may reduce the osmotic stress on plants (Akhtar et al. 2015a). In addition, increased availability of mineral nutrients in the soil as well as improvement in soil physical and chemical properties in the presence of BC might also play an important role in reduced Na uptake by plants (Lashari et al. 2013). At 5.0% BC either alone or under salt stress (25 mM), the Cd concentrations in wheat grains were well below the maximum permitted Cd levels (0.2 mg kg−1 dry weight; FAO 2014) in cereal grains. This showed that BC might be used to reduce Cd concentrations in cereals grown on Cd-contaminated saline soils.
Salinity increased the soil pH, EC, and AB-DTPA-extractable Cd concentrations in the post-harvest soil while BC application decreased Cd and increased soil pH and EC values compared to the treatments without BC supply (Table 3). The reduction in AB-DTPA-extractable Cd contents in the soil might be due to sorption of Cd to BC, and the sorption process is often irreversible with low desorption of Cd into the aqueous solution (Rizwan et al. 2016c). Application of Cd + NaCl increased the soil pH, EC (1:5 water), and Cd (water-extractable) concentration in the rhizospheric soil taken after C. rossii harvest compared to the control and respective Cd and NaCl treatments alone (Zhang et al. 2016). Biochar prepared from poultry manure decreased the soil pH when applied in the saline soil compared to the soil with only salinity (Lashari et al. 2013). Biochar application increased EC of the soil compared to the soil without BC, and the increasing trend was higher with increasing BC doses in the soil (Akhtar et al. 2015b). However, in the present study, there were lower EC values with BC treatments under 25 mM salt stress (Table 2). This reduction in EC values with BC amendments might be due to sorption of Na on the BC sites (Akhtar et al. 2015a). Lower Na concentration was found in leachates from columns treated with BC compared to the non-biochar-treated columns (Akhtar et al. 2015c).
Conclusion
The results of the present study showed that BC soil usage was an effective strategy with regard to stimulating wheat growth and biomass and alleviating salt and Cd stress in plants especially under mild salt stress (25 mM NaCl). The BC usage decreased the oxidative stress and enhanced the activities of antioxidant enzymes under combined Cd and salt stress. BC + NaCl increased the chlorophyll concentrations and gas exchange traits compared to the same NaCl treatments alone. The enhanced Cd and salt tolerance in wheat with the BC application was at least partly due to the reduction in NaCl-induced Cd and Na uptake by roots. However, higher BC and NaCl treatments negatively affected the plant growth, which indicated that BC doses should be used with great care in saline soils simultaneously contaminated with Cd to avoid the negative effects of BC on growth and metal uptake especially at higher BC and salinity treatments. Thus, more experiments are needed by different BC rates under combined salt and metal stress especially under field conditions.
References
Abbas T, Rizwan M, Ali S, Rehman MZ, Qayyum MF, Abbas F, Hannan F, Rinklebe J, Ok YS (2017) Effect of biochar on cadmium bioavailability and uptake in wheat (Triticum aestivum L.) grown in a soil with aged contamination. Ecotoxicol Environ Saf 140:37–47
Abbasi GH, Akhtar J, Anwar-ul-Haq M, Malik W, Ali S, Chen ZH, Zhang G (2015) Morpho-physiological and micrographic characterization of maize hybrids under NaCl and Cd stress. Plant Growth Regul 75:115–122
Abbasi GH, Ijaz M, Akhtar J, Anwar-Ul-Haq M, Jamil M, AliS AR, Khan HN (2016) Profiling of anti-oxidative enzymes and lipid peroxidation in leaves of salt tolerant and salt sensitive maize hybrids under NaCl and cd stress. Sains Malay 45:177–184
Adrees M, Ali S, Rizwan M, Rehman MZ, Ibrahim M, Abbas F, Farid M, Qayyum MF, Irshad MK (2015) Mechanisms of silicon-mediated alleviation of heavy metal toxicity in plants: a review. Ecotoxicol Environ Saf 119:186–197
Aebi H (1984) Catalase in vitro. Methods Enzymol 105:121–126
Akhtar SS, Andersen MN, Liu F (2015a) Biochar mitigates salinity stress in potato. J Agron Crop Sci 201:368–378
Akhtar SS, Andersen MN, Liu F (2015b) Residual effects of biochar on improving growth, physiology and yield of wheat under salt stress. Agri Water Manag 158:61–68
Akhtar SS, Andersen MN, Naveed M, Zahir ZA, Liu F (2015c) Interactive effect of biochar and plant growth-promoting bacterial endophytes on ameliorating salinity stress in maize. Funct Plant Biol 42:770–781
Amacher MC (1996) Nickel, cadmium and lead. p. 739–768. In: Sparks DL (ed) Methods of soil analysis. Part 3. Chemical methods, 3rd edn. SSSA/ASA, Madison
Ashrafi A, Zahedi M, Fahmi K, Nadi R (2014) Neighbour effects of purslane (Portulaca oleracea L.) on Cd bioaccumulation by soybean in saline soil. Plant Soil Environ 60:439–445
Azeem M, Iqbal N, Kausar S, Javed MT, Akram MS, Sajid MA (2015) Efficacy of silicon priming and fertigation to modulate seedling’s vigor and ion homeostasis of wheat (Triticum aestivum L.) under saline environment. Environ Sci Pollut Res 22:14367–14371
Bauddh K, Singh RP (2012) Growth, tolerance efficiency and phytoremediation potential of Ricinus communis (L.) and Brassica juncea (L.) in salinity and drought affected cadmium contaminated soil. Ecotoxicol Environ Saf 85:13–22
Bouyoucos GJ (1962) Hydrometer method improved for making particle size analyses of soils. Agron J 54:464–465
Cheng D, Wu G, Zheng Y (2015) Positive correlation between potassium uptake and salt tolerance in wheat. Photosyn 53:447–454
Dhindsa RS, Dhindsa PP, Thorpe TA (1981) Leaf senescence: correlated with increased levels of membrane permeability and lipid peroxidation, and decreased levels of superoxide dismutase and catalase. J Exp Bot 32:93–101
Dionisio-Sese ML, Tobita S (1998) Antioxidant responses of rice seedlings to salinity stress. Plant Sci 135:1–9
Drake JA, Cavagnaro TR, Cunningham SC, Jackson WR, Patti AF (2016) Does biochar improve establishment of tree seedlings in saline sodic soils? Land Degrad Develop 27:52–59
FAO (2014) ProdStat. Core production data base, Electronic resource under http://faostat.fao.org/. Accessed 30 June 2015
Farhangi-Abriz S, Torabian S (2017) Antioxidant enzyme and osmotic adjustment changes in bean seedlings as affected by biochar under salt stress. Ecotoxicol Environ Saf 137:64–70
Gallego SM, Pena LB, Barcia RA, Azpilicueta CE, Iannone MF, Rosales EP, Benavides MP (2012) Unravelling cadmium toxicity and tolerance in plants: insight into regulatory mechanisms. Environ Exp Bot 83:33–46
Garg N, Chandel S (2012) Role of arbuscular mycorrhizal (AM) fungi on growth, cadmium uptake, osmolyte, and phytochelatin synthesis in Cajanus cajan (L.) Millsp. under NaCl and Cd stresses. J Plant Growth Regul 31:292–308
Heath RL, Packer L (1968) Photoperoxidation in isolated chloroplasts: I. Kinetics and stoichiometry of fatty acid peroxidation. Arch Biochem Biophys 125:189–198
Hussain RA, Ahmad R, Waraich EA, Nawaz F (2015) Nutrient uptake, water relations, and yield performance lf different wheat cultivars (Triticum aestivum L.) under salinity stress. J Plant Nutr 38:2139–2149
Khaliq A, Zia-ul-HaqM AF, Aslam F, Matloob A, Navab A, Hussain S (2015) Salinity tolerance in wheat cultivars is related to enhanced activities of enzymatic antioxidants and reduced lipid peroxidation. CLEAN–Soil Air Water 43:1248–1258
Lashari MS, Liu Y, Li L, Pan W, Fu J, Pan G, Zheng J, Zheng J, Zhang X, Yu X (2013) Effects of amendment of biochar-manure compost in conjunction with pyroligneous solution on soil quality and wheat yield of a salt-stressed cropland from Central China Great Plain. Field Crops Res 144:113–118
Lichtenthaler HK (1987) Chlorophylls and carotenoids—pigments of photosynthetic biomembranes. In: Colowick SP, Kaplan NO (eds) Methods in enzymology, vol 148. Academic Press, San Diego, pp 350–382
Mandhania S, Madan S, Sawhney V (2006) Antioxidant defense mechanism under salt stress in wheat seedlings. Biol Plant 50:227–231
Mohamed AKSH, Qayyum MF, Abdel-Hadi AM, Rehman RA, Ali S, Rizwan M (2017) Interactive effect of salinity and silver nanoparticles on photosynthetic and biochemical parameters of wheat. Arch Agron Soil Sci. doi:10.1080/03650340.2017.1300256
Munns R, Tester M (2008) Mechanisms of salinity tolerance. Annu Rev Plant Biol 59:651–681
Murtaza G, Javed W, Hussain A, Wahid A, Murtaza B, Owens G (2015) Metal uptake via phosphate fertilizer and city sewage in cereal and legume crops in Zea mays Pakistan. Environ Sci Pollut Res 22:9136–9147
Naeem A, Ghafoor A, Farooq M (2015) Suppression of cadmium concentration in wheat grains by silicon is related to its application rate and cadmium accumulating abilities of cultivars. J Sci Food Agri 95:2467–2472
Naeem A, Saifullah RMZ, Akhtar T, Ok YS, Rengel Z (2016) Genetic variation in cadmium accumulation and tolerance among wheat cultivars at the seedling stage. Commun Soil Sci Plant Anal 47:554–562
Nagajyoti PC, Lee KD, Sreekanth TVM (2010) Heavy metals, occurrence and toxicity for plants: a review. Environ Chem Lett 8:199–216
Nakano Y, Asada K (1981) Hydrogen peroxide scavenged by ascorbate specific peroxidase in spinach chloroplasts. Plant Cell Physiol 22:867–880
Ok YS, Chang SX, Gao B, Chung HJ (2015) SMART biochar technology—a shifting paradigm towards advanced materials and healthcare research. Environ Technol Innov 4:206–209
Ondrasek G, Rengel Z, Romic D, Savic R (2012) Salinity decreases dissolved organic carbon in the rhizosphere and increases trace element phyto-accumulation. Eur J Soil Sci 63:685–693
Ozkutlu F, Ozturk L, Erdem H, McLaughlin M, Cakmak I (2007) Leaf-applied sodium chloride promotes cadmium accumulation in durum wheat grain. Plant soil 290:323–31
Page AL, Miller RH, Keeny DR (1982) Methods of soil analysis (Part 2). Chemical andmicrobiological properties. Agron. 9. SSSA, Madison
Qayyum MF, Abid M, Danish S, Saeed MK, Ali MA (2015) Effects of various biochars on seed germination and carbon mineralization in an alkaline soil. Pak J Agri Sci 51:977–982
Rady MM, Mounzer O, Alarcón J, Abdelhamid M, Howladar S (2016) Growth, heavy metal status and yield of salt-stressed wheat (Triticum aestivum L.) plants as affected by the integrated application of bio-, organic and inorganic nitrogen-fertilizers. J Appl Bot Food Qual 89:21–28
Redondo-Gómez S, Andrades-Moreno L, Mateos-Naranjo E, Parra R, Valera-Burgos J, Aroca R (2011) Synergic effect of salinity and zinc stress on growth and photosynthetic responses of the cordgrass, Spartina densiflora. J Exp Bot 62:5521–5530
Rehman MZ, Rizwan M, Ghafoor A, Naeem A, Ali S, Sabir M, Qayyum MF (2015) Effect of inorganic amendments for in situ stabilization of cadmium in contaminated soils and its phyto-availability to wheat and rice under rotation. Environ Sci Pollut Res 22:16897–16906
Rehman MZ, Rizwan M, Sabir M, Shahjahan AS, Ahmed HR (2016) Comparative effects of different soil conditioners on wheat growth and yield grown in saline-sodic soils. Sains Malay 45:339–346
Rizwan M, Meunier JD, Hélène M, Keller C (2012) Effect of silicon on reducing cadmium toxicity in durum wheat (Triticum turgidum L. cv. Claudio W.) grown in a soil with aged contamination. J Hazard Mater 209-210:326–334
Rizwan M, Ali S, Ibrahim M, Farid M, Adrees M, Bharwana SA, Rehman MZ, Qayyum MF, Abbas F (2015) Mechanisms of silicon-mediated alleviation of drought and salt stress in plants: a review. Environ Sci Pollut Res 22:15416–15431
Rizwan M, Ali S, Abbas T, Zia-ur-Rehman M, Hannan F, Keller C, Al-Wabel MI, Ok YS (2016a) Cadmium minimization in wheat: a critical review. Ecotoxicol Environ Saf 130:43–53
Rizwan M, Ali S, Adrees M, Rizvi H, Rehman MZ, Hannan F, Qayyum MF, Hafeez F, Ok YS (2016b) Cadmium stress in rice: toxic effects, tolerance mechanisms and management: a critical review. Environ Sci Pollut Res 23:17859–17879
Rizwan M, Ali S, Qayyum MF, Ibrahim M, Rehman MZ, Abbas T, Ok YS (2016c) Mechanisms of biochar-mediated alleviation of toxicity of trace elements in plants: a critical review. Environ Sci Pollut Res 23:2230–2248
Ryan PR, Delhaize E, Jones DJ (2001) Function and mechanism of organic anion exudation from plant roots. Ann Rev Plant Physiol Plant Mol Biol 52:527–560
Saeidnejad AH, Kafi M, Pessarakli M (2016) Interactive effects of salinity stress and Zn availability on physiological properties, antioxidants activity and micronutrients’ content of wheat (Triticum aestivum) plants. Commun Soil Sci Plant Anal 47:1048–1057
Salinity Laboratory Staff US (1954) Diagnosis and improvement of saline and alkali soils. Agriculture handbook 60. United States Salinity Laboratory, USDA, Washington DC, p 160
Schutzendubel A, Polle A (2002) Plant responses to abiotic stresses: heavy metal induced oxidative stress and protection by mycorrhization. J Exp Bot 53:1351–1365
Shafi M, Bakht J, Hassan MJ, Raziuddin M, Zhang G (2009) Effect of cadmium and salinity stresses on growth and antioxidant enzyme activities of wheat (Triticum aestivum L.) Bull Environ Contam Toxicol 82:772–776
Soltanpour PN (1985) Use of AB-DTPA soil test to evaluate elemental availability and toxicity. Commun Soil Sci Plant Anal 16:323–338
Xu ZM, Li QS, Yang P, Ye HJ, Chen ZS, Guo SH, Wang LL, He BY, Zeng EY (2017) Impact of osmoregulation on the differences in Cd accumulation between two contrasting edible amaranth cultivars grown on Cd-polluted saline soils. Environ Pollut. doi:10.1016/j.envpol.2016.12.067
Younis U, Malik SA, Rizwan M, Qayyum MF, Ok YS, Shah MHR, Rehman RA, Ahmad N (2016) Biochar enhances the cadmium tolerance in spinach (Spinacia oleracea) through modification of Cd uptake and physiological and biochemical attributes. Environ Sci Pollut Res 23:21385–21394
Yousaf B, Liu G, Wang R, Rehman MZ, Rizwan MS, Imtiaz M, Murtaza G, Shakoor A (2016) Investigating the potential influence of biochar and traditional organic amendments on the bioavailability and transfer of Cd in the soil–plant system. Environ Earth Sci 75:1–10
Zhang XZ (1992) The measurement and mechanism of lipid peroxidation and SOD POD and CAT activities in biological system. In: Zhang XZ (ed) Research methodology of crop physiology. Beijing Agriculture Press, Beijing, pp 208–211
Zhang J, Kirkham MB (1994) Drought-stress-induced changes in activities of superoxide dismutase, catalase, and peroxidase in wheat species. Plant Cell Physiol 35:785–791
Zhang C, Sale PW, Tang C (2016) Cadmium uptake by Carpobrotus rossii (Haw.) Schwantes under different saline conditions. Environ Sci Pollut Res 23:13480–13488
Acknowledgements
The financial support from Government College University, Faisalabad, and Higher Education Commission of Pakistan is gratefully acknowledged.
Author information
Authors and Affiliations
Corresponding author
Additional information
Responsible editor: Elena Maestri
Electronic supplementary material
ESM 1
(DOCX 15 kb)
Rights and permissions
About this article
Cite this article
Abbas, T., Rizwan, M., Ali, S. et al. Effect of biochar on alleviation of cadmium toxicity in wheat (Triticum aestivum L.) grown on Cd-contaminated saline soil. Environ Sci Pollut Res 25, 25668–25680 (2018). https://doi.org/10.1007/s11356-017-8987-4
Received:
Accepted:
Published:
Issue Date:
DOI: https://doi.org/10.1007/s11356-017-8987-4