Abstract
The resinous portion of the Aquilaria tree is called agarwood, a valuable non-timber product being used as medicine and incenses in Asia, Middle East, and Europe. Driven by high demand, the wild resources of agarwood-producing trees have been greatly threatened. This fragrant product contains many aromatic substances and is obtained from the pathological conditions of the wood of living trees. The knowledge regarding the technology for inducing agarwood and its continuous formation in the tree is still limited. To conserve the wild Aquilaria spp. and to supply sustainable amount of agarwood, cultivation of Aquilaria trees in combination with induction through artificial technique is seen as the best approach. In this chapter we will discuss the fundamentals of agarwood formation in the producing trees, the molecular pathway in its synthesis, current methods applied for agarwood induction in cultivated trees, and finally the factors influencing agarwood yield and quality.
Access provided by Autonomous University of Puebla. Download chapter PDF
Similar content being viewed by others
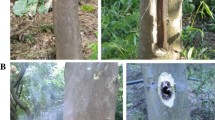
Keywords
These keywords were added by machine and not by the authors. This process is experimental and the keywords may be updated as the learning algorithm improves.
3.1 Introduction
Agarwood is a precious non-timber product of tropical tree origin. Due to its aromatic fragrance, it is used as a raw material in making incenses and perfumes. Agarwood is held with high regard in many different cultures of the world, thus the various names: agar (Hindi), agaru (Tibetans), akil (Tamil), chenxiang (Chinese), eaglewood (Papua New Guinea), gaharu (Malay), jinkoh (Japanese), oud (Arabic), mai ketsana (Laos), mai kritsana (Thai), sasi or sashi (Assamese), and tramhuong (Vietnamese). The Japanese jinkoh literally means “sinking incense,” and the highest grade is known as kyara (Kiyoko 1992). In Europe, agarwood is historically known as Lignum aquila (eaglewood)/agilawood, Lignum aloes, or aloeswood (Henry and Burnell 1903).
Agarwood has high commercial value but the tree source is severely endangered due to indiscriminate felling. Over the years, demand for this scarce material, which is often obtained from natural population, has increased tremendously. This has led to its exploitation in sourcing countries such as India, China, and Southeast Asian countries, together with West Papua and Papua New Guinea (PNG) (Barden et al. 2000; TRAFFIC Oceania, 2002; TRAFFIC East Asia-Taipei and TRAFFIC Southeast Asia, 2005; Wyn and Anak 2010). Agarwood is exported in the form of blocks, wood chips, powder, dust, and essential oil. All these come in a wide range of grades and thus prices. To overcome demand and conserve the sources, much effort has been drawn toward cultivating the tree species in sourcing countries, such as Cambodia, China, Indonesia, Malaysia, Thailand, and Vietnam.
3.2 Agarwood-Producing Tree Genera
The Thymelaeaceae family consists of many important incense-producing tree species, including those from the genera Aquilaria, Gyrinops, Gonystylus, and Aetoxylon. While all have the ability to produce incense wood, Aquilaria and Gyrinops are the two most important genera when it comes to producing agarwood. Gonystylus is better known for its hardwood timber from the peat swamp, whereas Aetoxylon produces fragrant wood of less importance, by comparison.
The genus Aquilaria has a wide distribution in the Indomalesian region. This is reflected from the wealth of names given to this highly valuable wood in different cultures. For a long period of time, Aquilaria has been harvested severely from the forests forcing their numbers to decline. The species A. malaccensis, in particular, was so heavily poached that in 1995, it became the first species subjected to the trading regulation of the Convention of the International Trade of Endangered Species (CITES). Worldwide, there are 21 Aquilaria species and nine Gyrinops; these have been reviewed and accepted (The Plant List, version 1.1). Many of them have significant profitable value. Among the Aquilaria genus that have been exploited are A. beccariana, A. crassna, A. filaria, A. malaccensis, A. microcarpa, A. sinensis and A. subintegra, while from the Gyrinops are G. ledermannii, G. versteegii, and G. walla. Because of their close resemblance, it was difficult to ascertain species identity. This effectively placed all Aquilaria spp. and Gyrinops spp. under CITES listing in 2005. Gonystylus was not spared as well but for reasons associated to timber logging. The listing under Appendix II of CITES is perceived as an international driving force toward a legal and sustainable manner of agarwood trading between importing and exporting countries (Compton 2004). CITES is one of the world’s commanding agreements on species conservation. Its method of controlling is by employing trade permit and by imposing a quota on the export quantity by country members. The rationale of doing this is to protect agarwood from overharvesting, regardless of species.
Aquilaria has been studied in more detail when compared to Gyrinops; thus it can be considered as the model tree for incense-producing species. In this chapter, special attention is given to Aquilaria due to the current mass of scientific knowledge gathered on this genus.
3.3 The Beginning of Agarwood in the Wood Tissue
Agarwood is an oleoresin; it has a solid or semisolid form (viscous) and it is insoluble in water. Like many softwood tree species, the agarwood resin is secreted in the stem tissues of Aquilaria. However, in Aquilaria, bundles of phloem cells are produced throughout the xylem as well as in a layer external to the xylem. This is in contrast to angiosperms that produce phloem cells growing out from the circumference of the cambium. Indeed, wood anatomy of Aquilaria is exceptional because the xylem region, in addition to vessels, fibers, and parenchyma cells, also consists of groups of phloem cells called included phloem or interxylary phloem. Living parenchyma cells are the most important elements in agarwood formation because they are able to biosynthesize resinous substances of agarwood (Nobuchi and Mohd Hamami 2008). Brownish droplets containing the resinous substances have been detected in the included phloems, parenchyma cells, and vessels, in the xylem region of agarwood-containing stem (Fig. 3.1) (Mohamed et al. 2013). This network of anatomical structures appears to be responsible in producing, storing, and distributing agarwood constituents to affected areas as the tree defends itself against various enemies and damages. Previously, it was thought only old trees produce resinous agarwood. New evidence has emerged that even juvenile Aquilaria trees own these structures and are capable to produce agarwood when induced (Mohamed et al. 2014b).
A transverse section of an agarwood-containing stem. Brownish substances are seen deposited in the included phloems (IP) and ray parenchyma (RP) cells in this unstained section (Scale bar = 100 μm). V vessel, F fiber (Source: Mohamed et al. 2013)
To understand agarwood formation in Aquilaria, it is valuable to look into another tree family that produces resin, the Pinaceae. In pine and spruce trees, interconnecting vertical and horizontal resin canals are regular features of the wood. The canals are tubular structures composed of long-lived epithelial resin-secreting cells, parenchyma support cells, and strand tracheids. Resin is formed in the epithelial cells and is transported through the matrix of vertical and horizontal ducts (reviewed in Cown et al. 2011). Heartwood formation (resin impregnation) commences when the stems progresses outward from the pith, and this is most apparent in old pine stems (25–30 years) where the resin may account for 25–35 % of the weight of the heartwood (reviewed in Cown et al. 2011). In relation to Aquilaria, the oleoresin filled up the various compartments, became hard, and got impregnated in the wood. The extensive deposits of resin over time increase the wood density of agarwood to unusually high levels.
3.4 Causes to Agarwood Formation
General observations established that the fragrant resin is never produced in sound trees. This suggests an association exists between damaged tissues and infestation by biotic agents, which leads to the resulting agarwood (Oldfield et al. 1998). Indeed, wounding has been recognized as the first cause to agarwood formation (Pojanagaroon and Kaewrak 2005; Nobuchi and Siripatanadilok 2008). Physical damage causes the tree to weaken and become vulnerable to fungal infection. Soon after, in a matter of days, a slight discoloration would become visible surrounding the wound. It darkens with time and is easily detected against the otherwise whitewood. The darkened zone eventually gets bigger and signifies agarwood presence. This proves that agarwood formation may involve prolonged microbial infection, which continuously elicits synthesis of agarwood constituents in the area. Damage by boring insects, for example, involves wounding followed by infection. Fungi, in particular, are believed to be the major infecting agent that enters the host through the wounds. The host’s defense system reacts by producing agarwood compounds to fight off the pathogens. Since the 1900s, many workers have associated the agarwood zones to fungal infection (reviewed in Ng et al. 1997). Direct fungal isolation, microscopy, and more recently rDNA cloning and sequencing have revealed that the area in and surrounding agarwood zones do harbor a variety of fungi. Among them are members of the genera Aspergillus, Cunninghamella, Curvularia, Fusarium, Lasiodiplodia, Penicillium, Pythium, Trichoderma (Mohamed et al. 2010; Premalatha and Kalra 2013), and many others.
In its natural surroundings, Aquilaria is highly exposed to a diverse group of microorganisms, whether in the rhizosphere in the soil (Nimnoi et al. 2011) or in the stems itself (Zhang et al. 2014b). There are limited studies regarding Aquilaria’s interaction with its diverse fungal community. In one study, three fungal species in two wounded wild A. malaccensis were tracked over time using quantitative real-time PCR (qPCR), in wounds that represent different time points, i.e., 0–18 h, 2–13 days, 2–18 weeks, and 6–12 months (Mohamed et al. 2014a). The three species were common fungi often associated with agarwood formation: Cunninghamella bainieri, Fusarium solani, and Lasiodiplodia theobromae. The qPCR data revealed that the abundance of the three species decreased over time. The fungi were detected in high numbers during the first few hours and days after wounding and in low numbers many months later. On the contrary, the darkened zone where agarwood is formed continued to enlarge over time (Fig. 3.2). The accumulation of agarwood compounds at the wounding site could have caused the decline in fungal abundance, which is consistent with its role in defense response.
The progression of the darkened zones observed on wounded stem surfaces at different times after wounding. (a) 2 days, light yellow; (b) 5 days, light brown; (c) 2 weeks, brown; (d) 4 weeks, dark brown; (e) 6 months, blackish brown; and (f) 9 months, black. The diameter of the hole is 16 mm (red arrow) (Source: Mohamed et al. 2014a)
3.4.1 Customary Induction
Agarwood induction, as perceived by local people, on trees growing in the natural environment, happened in old trees that had been stroked by lightning or attacked by animals, insects, or microbes. The formation is usually in proximity to wounded or decaying parts of the trunk. Initially it was thought that formation of agarwood takes place only in the stem or the main branches. New observations however revealed that it happens in roots and twigs as well. The production of agarwood was thought to happen very slowly in old trees (Gianno 1986; Chakrabarty et al. 1994), and the slow process due to fungal involvement in infecting the wood. New studies revealed that even at the age of 3 years, cultivated trees can produce agarwood when artificially treated (Heuveling van Beek and Phillips 1999; Mohamed et al. 2014b).
Various conventional methods have been developed. For example, the Chinese farmers have been using the burning-chisel-drilling and partial-trunk-pruning methods. Other methods of wounding by using ax, machete, bark removal, and nailing have also been useful in other parts of the world such as in Indonesia, Malaysia, and Thailand. These methods are hand-down traditions and have been practiced sustainably on trees growing on individual and ancestral lands and in natural forests.
3.4.2 Non-customary Induction
Over decades, people pondered on how agarwood forms in nature. From the information gathered, nonconventional methods had emerged to mimic the natural event. Many researchers began to employ artificial induction by inoculating inducing agents directly into the tree stem. Early works have been documented by Ng et al. (1997), beginning with Tunstall in 1929, who first inoculated fungi experimentally into Aquilaria agallocha followed by others such as Bose (1934), Sadgopal and Varma (1952), Gibson (1977), Rahman and Khisa (1984), and Santoso (2013). All applied open wounds as the mode of inoculation with the only difference being the fungal species. The outcomes of their inoculation tests were of mixed results. At that point, it was concluded that agarwood formation was initiated by wounding followed by nonspecific fungal infection (Rahman and Basak 1980).
3.4.3 Modern Artificial Induction
To meet the demand and market needs of agarwood, large plantations have been established in sourcing countries. This trend has been increasing for the last 20 years with participation from individuals, local communities, entrepreneurs, and government agencies. Although planting trees is a straightforward venture, agarwood induction is quite complicated. Without an efficient and proven induction method, return on investment made in the plantations will be minimal because the healthy wood is of little value. To develop proven methods, two directions have been considered: (1) the delivery mode and (2) superior inducing agents, whether in the form of microbes, chemicals, or both. One such method was pioneered by Blanchette and Heuveling van Beek (2009) known as Cultivated Agarwood kits (CA-Kits), where tubes are placed in the tree trunk as a mean to introduce microbes and to arouse production of the defense compounds by the tree naturally. Others such as the Taiwan and Pheerapan methods are also available in the market. Both methods use microbes for the production of agarwood (Chang et al. 2011); however, it is a slow process.
3.4.3.1 Inoculum-Based Inducer
Generally, inoculum- or microbe-based techniques require a long incubation time before harvesting to produce darker wood and subsequently better forms of agarwood. In a study to evaluate the effect of several fungal species on agarwood formation over time in A. malaccensis, changes in the length and intensity of the darkened zone were observed after 3- and 6-month periods following inoculation (Mohamed et al. 2014b). A positive relationship with time was perceived but not with the tested fungal species. Gas chromatography-mass spectrometry (GC-MS) analysis of the 6-month-old sample yielded some important agarwood compounds such as benzylacetone, anisylacetone, guaiene, and palustrol. Similar results were obtained from artificially inoculated A. sinensis with the fungus Melanotus flavolivens when samples were harvested 6 months and 1 year post inoculation (Lin et al. 2010). Major agarwood compounds – specifically benzaldehyde, benzenepropanoic acid, anisylacetone, and a chromone, 8-methoxy-2-(2-phenylethyl)-4H-1-benzopyran-4-one – were detected. Other successful inocula that have been tested include the ascomycete fungus Paraconiothyrium variabile (Cui et al. 2013) and the deuteromycete Lasiodiplodia theobromae (Zhang et al. 2014a) on A. sinensis and Fusarium oxysporum and Fusarium solani on A. microcarpa (Akhsan et al. 2015). However, less promising results for artificial fungal induction methods were also reported (Tamuli et al. 2005; Bhuiyan et al. 2009). When under attack, the first defense mechanism activated by Aquilaria is observed in the form of callus around the wounded area where agents gained penetration into the host’s cell (Blanchette and Heuveling van Beek 2009). It can be speculated that Aquilaria choose to perform callusing rather than producing resin when the threat level is weak and nonpersistent. In view of this, to develop an effective inoculum-based inducer, interaction between inoculum strain and Aquilaria genotypes needs to be explored further.
3.4.3.2 Chemical as Inducer
Chemicals appear to be potent agarwood inducers. Wei et al. (2010) showed that chemicals when coupled with an ingenious mode of delivery system can induce agarwood in the whole tree and not confined to local areas as shown in other conventional methods. This promising method, patented as “whole-tree agarwood-inducing technique” (Agar-Wit), applies simple and cheap transfusion sets through which agarwood inducers are injected into the xylem part of the tree (Zhang et al. 2012). The inducer is in liquid form and is elated to the whole body of the tree due to water transportation causing agarwood to form in every woody part. Most importantly, the induced agarwood meets the medicinal quality set by the Chinese Pharmacopoeia (Liu et al. 2013). The identities of chemicals in patents are generally not made public, or their strengths and formulations are kept discrete. They could be in the form of plant defense elicitors such as hydrogen peroxide (H2O2), methyl jasmonate (MJ), or salicylic acid (SA) (Okudera and Ito 2009; Wijitphan 2009; Wei et al. 2010) or chemicals such as sodium chloride, sulfuric acid, formic acid, and sodium methyl bisulfite (Thanh et al. 2015). Chemical-based inducer acts by causing severe injury to Aquilaria cell structure, and as a result callusing cannot be performed to cover up the wound. While some chemicals such as organic based may be safe to consume, there are some unscrupulous individuals who applied pesticides as type of inducer. The impact of this inducer is a great concern to the environment and agarwood consumers. Chemical-based inducers appeared to be very attractive due to the ease of preparation and application, inexpensive, and fast result, but more evidence is needed to demonstrate the effectiveness. More importantly, appropriate tests must be conducted to establish the chemical residual content in the resulting agarwood that does not become a health hazard.
3.5 Compounds in Agarwood
The main compounds found in the oleoresin of agarwood are a complex mixture of sesquiterpenes and 2-(2-phenylethyl) chromones (reviewed in Naef 2011). Together with some simple volatile aromatic compounds, they create this impressive pleasing odor that some described as balsamic, spicy, woody, and sweet. Analysis into the chemical constituents of agarwood had actively begun in the 1960s and is continuing into the twenty-first century. Thus far, the total number of identified compounds is over 150. This explains the richness and diverse organoleptic properties found in agarwood from various species and regions. Agarwood is rich in terpenoid contents, but as of today none of these terpenoids can be manufactured industrially, thus the reliance on natural sources. Attempts have been made to categorize Aquilaria and Gyrinops species according to their chemical profiles. However, this seemed unattainable because the composition is highly dependent on geographical sites.
Sesquiterpenes in agarwood were first characterized from A. agallocha by Indian chemists, more than 40 years ago (reviewed in Konishi et al. 2002). Some 70 sesquiterpene compounds have been identified so far and their structures elucidated (Naef 2011). Examples are agarofurans, eudesmanes, and guaienes and their oxidized forms such as jinkoh-eremol and agarospirol. Both of the latter compounds are known to have sedative and analgesic effects (Okugawa et al. 1996; Takemoto et al. 2008). Another characteristic for agarwood is chromone. The first report on oxygenated chromone derivative from agarwood was released in 1978 (Yoshii et al. 1978). Since then, many more structurally different chromones have been discovered. Close to 40 2-(2-phenylethyl) chromones have been recognized from agarwood of numerous abilities, of which 17 are agarwood specific and may be used as phytochemical markers for authentication purposes (reviewed in Naef 2011). In addition, three derivatives of diepoxy-tetrahydro-2-(2-phenylethyl) chromones may be used to indicate infected wood because they appeared only in resins isolated from wounded wood of Aquilaria and not in the healthy part (Yagura et al. 2005). Interestingly, the trio also appeared in Aquilaria calli and cell cultures in addition to guaiene-derived compounds (Okudera and Ito 2009) indicating that in vitro agarwood production is possible. Chromone compounds are described as having bigger role in producing the warm, balsamic, and enduring odor when agarwood is heated and smoke is produced. In very high-quality agarwood such as kanankoh, the chromone content is reportedly at 60 %, while the less superior type jinkoh has only 1.5 % (Ishihara et al. 1993). Kanankoh is used in the kohdoh ceremony (listening to incense) where the fragrance of agarwood is appreciated in an elaborated traditional manner.
The types and derivatives of sesquiterpenoids and chromones in agarwood are extensive. After the comprehensive review by Naef (2011), additional new compounds have been discovered from agarwood (Wu et al. 2012; Li et al. 2014, 2015; Yang et al. 2014; Wang et al. 2015), and the number no doubt will continue to grow.
3.6 Biosynthesis of Major Compounds
From general understanding, it can be concluded that the most probable role of agarwood is for the tree to defend itself against biotic and abiotic stresses. Stress induces the defense response and triggers the secondary metabolism network leading to agarwood compound formation and resin accumulation. Essentially, agarwood gets its fragrance from the presence of the aromatic terpenes, specifically the sesquiterpenes, and the chromones (Naef 2011). For that reason it is important to understand the synthetic mechanisms of these two compounds. In this section, special emphasis is given to the activation of genes in the sesquiterpenoid synthetic pathway.
3.6.1 Sesquiterpenoid Biosynthetic Pathway
Much information has been gathered on terpenoid biosynthetic pathways (Hu and Lu 2015; Singh and Sharma 2015). In plants, two pathways have been established: (1) the mevalonic acid (MVA) and (2) the 1-deoxy-d-xylulose-5-phosphate (DXP), also known as the methylerythritol phosphate (MEP) pathways. They manufacture the C5 isoprene units, isopentenyl diphosphate (IPP), and its isomers, dimethylallyl diphosphate (DMAPP). These are important terpenoid building blocks, being synthesized either in the cytoplasm or the plastid organelle, according to the respective pathway. The formation of IPP and DMAPP from acetyl-CoA or pyruvate are catalyzed by a sequence of different enzymes. Genes encoding for these enzymes have been identified and characterized from Aquilaria species via transcriptome (Xu et al. 2013) and genome sequencing (Chen et al. 2014) and are discussed in more detail in Chap. 5. In the following step, IPP or DMAPP is connected through the head-to-tail connections to form farnesyl diphosphate (FPP) (C15 unit), the precursor of sesquiterpenes, in the presence of FPP synthase (FaPS) (Cao et al. 2012). Kenmotsu et al. (2011) first cloned the Am-FaPS-1 gene from A. microcarpa, which exhibited high homology with FaPS from different plant sources. The transcript was abundant upon exposure of the cell culture to MJ, yeast extract, and Ca2+ ionophore A23187, indicating that the two former substances are triggers to induced responses in plants, while Ca2+ acts as a molecule messenger in activating the process. This clearly shows that substances such as MJ could be used to enhance biosynthetic pathways of secondary metabolites in Aquilaria.
In the final step, the FPPs are transformed into sesquiterpenes (C15) by specific enzymes; sesquiterpene synthases. Genes encoding for sesquiterpene synthases in Aquilaria are present in multiple copies (Kumeta and Ito 2010). At least five clones have been reported from A. crassna, all having high similarities in amino acid sequences. After being expressed in E. coli and the product enzymatically assayed using FPP, only three clones generated the same compounds as mined from MJ-treated cells. The product was δ-guaiene. These genes and their encoded enzymes are the first sesquiterpene synthases yielding guaiene-type sesquiterpenes as the major products. Via transcriptome sequencing, Xu et al. (2013) have identified several clones of sesquiterpene synthases (ASS1, ASS2, and ASS3) from A. sinensis, all yielding guaiene-type product as well. Despite its richness in sesquiterpenes, genes encoding for sesquiterpene synthases producing other types of sesquiterpenes have not been reported from Aquilaria.
3.6.2 Signaling Pathway
Wounding, insect boring, and fungal infection are types of abiotic and biotic stresses that can provoke agarwood production in Aquilaria. Aquilaria reacts to wounding and pathogen attack by stimulating specific genes of which some are expressed in the area of wound site, and the rest is triggered in the non-damage part of the plant, via the activation of multiple signaling events, similar to other plants’ response to stress (Mucciarelli et al. 2007; Rodriguez et al. 2009; Wang et al. 2010). One pathway that has been proposed for Aquilaria is the MAPK (mitogen-activated protein kinase) signaling pathway (Xu et al. 2013). Throughout the eukaryotic evolution, MAPK cascades have been highly conserved modules (Kusari et al. 2004; Pitzschke et al. 2009). These cascades are nominally made up of MAPK kinase kinase (MAPKKK), a MAPKK (MAPK kinase), while MAPK connects the upstream receptors to downstream targets. Using A. sinensis as the model tree, it has been proposed that at transcriptional level, the ASS1 gene expression is controlled by wound signal, which activates the MAPK cascade and phosphorylates downstream transcription factors (TFs) like MYB or WRKY (Xu et al. 2013). The initiation of ASS1 transcription must be due to triggered TFs that bind to the cis-acting elements in the promoter of ASS1. About 41 unigenes were interpreted to be correlated to the MAPK pathway and another 25 to calcium signaling that may perform roles in the wound responses of agarwood formation in A. sinensis. The treatment of MJ showed considerable upregulation of transcription factors like MYB4, WRKY4, MAPK2, MAPKKK, and the NADPH oxidase. Elicitors like jasmonic acid (JA) are known to trigger the TFs downstream through the hydrogen peroxide pathway (Kazan and Manners 2008). Some of the TFs with positive regulators of sesquiterpene synthases like the AP2, WRKY, and MYC genes have been identified from A. sinensis (Xu et al. 2013) and their functions compared to their homologues in other plant species. In Gossypium arboreum, the GaWRKY1 transcription factor shows positive expression of (+)-δ-cadinene synthase that catalyzes the biosynthesis of sesquiterpene gossypol (Xu et al. 2004). In Artemisia annua, the ADS gene regulates the biosynthesis of artemisinin in the presence of the TF, AaWRKY1 (Ma et al. 2009). The responsive AP2/ERF (ethylene-responsive factor) was also found to control the biosynthesis of artemisinin by binding to CBF2 and RAA (Yu et al. 2012). Similarly, when Oryza sativa was treated with MJ, a marked increase in the expression of the sesquiterpene synthase gene, TPS3, was observed, in addition to the release of more than ten sesquiterpenes mainly of the β-caryophyllene type (Cheng et al. 2007).
3.6.3 Chromones
Chromones are one of the major classes of naturally occurring compounds including flavonoids and possess important biological activities as antitumor, antioxidant, anti-inflammatory, antibacterial, and many more (Tawfik et al. 2014). Chemically, chromones (4H-chromen-4-ones) are heterocyclic compounds with the benzopyranone ring. Aquilaria spp. is one of the few plant species that produces the rare chromone known as 2-(2-phenylethyl) chromone. To our knowledge, there is no report on the biosynthesis of chromones in Aquilaria, although generally they are thought to be a result from convergence of multiple biosynthetic pathways such as the acetate, pentaketide, and shikimate pathways. This chromone group has been discovered only in a handful plant species (reviewed in Ibrahim and Mohamed 2015). Due to the vast range of biological functions associated with this compound scaffold, several synthetic applications have been developed to find new chemical entities as new drugs using the chromone ring system as the backbone structure. Advances in chemical processes have shown that the synthesis of 2-(2-phenylethyl) chromone is possible (Goel et al. 2006).
3.7 Factors Influencing Agarwood Yield and Quality
The yield and quality of the resinous agarwood vary considerably. Gianno (1986) suggested that a tree above 20 cm in diameter at breast height produces approximately 1 kg of agarwood. However, research in West Kalimantan, Indonesia, shows that the yield of Aquilaria resin is not correlated with tree diameter or timber volume, even when the trees have similar progression in infection (Soehartono and Mardiastuti 1997). Several factors are thought to affect the produced agarwood, both in quantity and quality (Ng et al. 1997). In our opinion, the most important factor is genetic variability between the tree species themselves. Unfortunately, no information is available regarding genetic variation or heritability for Aquilaria or Gyrinops. This is partly due to the difficulty in quantifying and ascertaining quality of the produced agarwood. Another important factor is the treatment applied onto the tree to induce agarwood. In a field trial conducted in planted A. crassna, Thanh et al. (2015) showed that agarwood oil samples from different treatments including biological (fungal mixture), chemical (sulfuric acid and sodium methyl bisulfite mixture), and mechanical (hammered nails) differ in quantity and quality. A year after, chemical treatment yielded agarwood oil with the highest sesquiterpene content compared to the other treatments and non-treated. On the other hand, biological treatment gave the highest sesquiterpene yield when the trees were left for 2 years. Time appears to be a major factor when biological agent is involved.
To grow Aquilaria species or genotype in its native climatic region is perhaps more suitable compared to foreign species. However, it has been shown that Aquilaria adapts easily to new environment, resulting in many Aquilaria plantations in sourcing countries been planted with nonnative species. For example, successful Aquilaria plantations have been established in Australia, a region that is beyond the tree natural distribution (Page and Awarau 2012). In Malaysia, where A. malaccensis is native, people have succeeded in planting A. crassna and A. subintegra species as well. One problem that could arise from this situation is the complex relationship between inoculum, tree genotype, and the environment. The type of inoculum or inducer is an important factor that influences agarwood quality. Each strain of inoculum certainly propagates better in its own environment; therefore a broad-spectrum inoculum is needed for economic benefits. Similar strains when applied on Aquilaria and Gyrinops trees yielded agarwood of differing smells (Turjaman and Santoso 2012). If the host factor is removed, and the inoculum is replaced by chemical inducer, the resulting agarwood could be of a more consistent quality (Liu et al. 2013). This proves that agarwood quality is controlled by genetic factors of the host and the inoculum, with the environment playing a compounding role.
Agarwood is in the trade history since long time ago, but its quality is very subjective and highly dependent on personal experiences. In the past, collectors harvested agarwood from wild trees, and quality determined from the age of the tree. It was thought that an older tree yielded higher agarwood quality (Barden et al. 2000; Persoon 2007). In contrast to this common belief, 7 to 8 years old trees are found capable of producing agarwood (Paoli et al. 2001; Chetpattananondh 2012). Even trees as young as 3 to 5 years old have been shown to form agarwood (Xu et al. 2013; Gao et al. 2014; Mohamed et al. 2014b). Therefore, it is not the age of the tree but the use of proper treatment that is paramount for inducing agarwood. It has now become evident that plantation trees can yield agarwood with quality similar to high-grade wild agarwood when using suitable induction methods such as the Agar-Wit (Liu et al. 2013). More on the different agarwood grades and grading methods are discussed in Chap. 10.
3.8 Valuable Agarwood Compounds from Cell Suspension Culture
Biotechnology offers an opportunity to exploit new means for the production and accumulation of many of the valuable chemical compounds found in plants such as alkaloids, terpenoids, steroids, saponins, phenolics, flavanoids, and amino acids, through plant cell cultures. Even though there are limitations in using plant cell cultures, some can produce higher amount of secondary metabolites than the intact plants (Sree et al. 2010). At present, only limited work on plant cell culture of Aquilaria has been reported. The production of valuable agarwood compounds has been shown feasible in cell suspension culture of Aquilaria when induced with a proper elicitor. Three species of sesquiterpene (á-guaiene, á-humulene, and δ-guaiene) and four of chromones (phenylethylchromones (5S,6R,7R,8S)-2-(2-phenylethyl)-5e’,6e,7a,8a’tetrahydroxy-5,6,7,8- tetrahydrochromone; 6-hydroxy-2-(2phenylethyl) chromone; 6-methoxy-2-(2-phenylethyl) chromone; 6-methoxy-2-[2-(3-methoxyphenyl)ethyl] chromone; 6,7-dimethoxy-2-(2-phenylethyl) chromone) were found to be induced by molecules in signaling transduction such as MJ and salicylic acid (Ito et al. 2005; Okudera and Ito 2009). Elicitors are signal molecules responsible for triggering the signal transduction cascade leading to the activation and expression of genes in the biosynthesis of secondary metabolites (Wang and Wu 2013). In Aquilaria, induction can be in the form of abiotic or biotic factors; as such, elicitors could be molecules that are excreted by the pathogen or the fungal propagules themselves. Cell suspension culture of A. sinensis produced four derivatives of 2-(2-phenylethyl) chromones when challenged with crude fungal extracts of M. flavolivens (Qi et al. 2005). In another study (Jayaraman and Mohamed 2014), crude mycelial extracts of Trichoderma sp. elicited several important agarwood compounds including 8-epi-.gamma.-eudesmol, á-guaiene, and alloaromadendrene oxide-1. The elicitor was added to the cell suspension culture, initiated with fresh calli originated from the leaf explants of A. malaccensis (Fig. 3.3). These are promising results on the potential use of fungal elicitor as biological inducer for valuable agarwood compound production in in vitro Aquilaria cultures.
Callus induced from Aquilaria malaccensis leaf explants after 30 days incubation in MS medium supplemented with 1.1 μM naphthaleneacetic acid (NAA) and various 6-benzylaminopurine (BAP) concentrations, (a) 0.55 μM, (b) 1.1 μM, (c) 2.2 μM, (d) 3.3 μM. Bar = 5 mm (Jayaraman et al. 2014)
3.9 Conclusion and Future Perspectives
Since the ever increasing international demand for agarwood cannot be satisfied by limited natural stocks of Aquilaria trees, there is a need for mass cultivation of the trees to relieve the pressure on its natural population. Not only planting better genotypes can help, but one has to develop techniques that are efficient at inducing agarwood and practical for application in plantation setting. To achieve this goal, background knowledge of the biology of the organism is important. Therefore, understanding agarwood induction and formation, specifically at molecular level, is essential for improving the production in living trees. By elucidating the biosynthetic pathway and regulation of induction, better ways and techniques can be developed to boost the success of agarwood formation. In the future, novel techniques created from this knowledge may be used to supply quality agarwood from plantation trees and thus satisfy not only the demand but most crucially to help to preserve wild Aquilaria trees in their already depleting natural populations.
References
Akhsan N, Mardji D, Sutisna M. Response of Aquilaria microcarpa to two species of Fusarium under two different cultivation systems. J Trop For Sci. 2015;27:447–55.
Barden A, Anak NA, Mulliken T, Song M. Heart of the matter: Agarwood use and trade and cites implementation for Aquilaria malaccensis. Cambridge, UK: TRAFFIC International; 2000.
Bhuiyan MNI, Begum J, Bhuiyan MNH. Analysis of essential oil of eaglewood tree (Aquilaria agallocha Roxb.) by gas chromatography mass spectrometry. Bangladesh. J Pharma. 2009;4:24–8.
Blanchette RA, Heuveling van Beek H. Cultivated agarwood. US Patent 7638145 B2; 2009.
Bose SR. The nature of agar formation. Sci Cult. 1934;4(2):89–91.
Cao X, Yin T, Miao Q, Li C, Ju X, Sun Y, Jiang J. Molecular characterization and expression analysis of a gene encoding for farnesyl diphosphate synthase from Euphorbia pekinensis Rupr. Mol Biol Rep. 2012;39:1487–92.
Chang YS, Nor Azah MA, Rashid AMA. Inducement of gaharu and potential of gaharu oils. In: Rashid AMA, Zuhaidi YA, editors. Tapping the wealth from karas (Aquilaria malaccensis) tree. Malayan Forest Records No. 50, Forest Research Institute Malaysia, Kepong. 2011. p. 48–63.
Chen CH, Kuo TCY, Yang MH, Chien TY, Chu MJ, Huang LC, Chen CY, Lo HF, Jeng ST, Chen LFO. Identification of cucurbitacins and assembly of a draft genome for Aquilaria agallocha. BMC Genomics. 2014;15(578):1–11.
Cheng AX, Xiang CY, Li JX, Yang CQ, Hu WL, Wang LJ, Lou YG, Chen XY. The rice (E)-beta-caryophyllene synthase (OsTPS3) accounts for the major inducible volatile sesquiterpenes. Phytochemistry. 2007;68:1632–41.
Chetpattananondh P. Overview of the agarwood oil industry. International Federation of Essential Oils and Aroma Trades (IFEAT) International Conference Singapore; 2012. p. 131–8.
Compton J. Final draft report to FAO TCP/PNG/2901, eaglewood management project. Southeast Asia: TRAFFIC; 2004.
Chakrabarty K, Kumar A, Menon V. Trade in agarwood. New Delhi: TRAFFIC India and WWF-India; 1994.
Cown DJ, Donaldson LA, Downes GM. A review of resin features in radiata pine. New Zea J Forest Sci. 2011;41:41–60.
Cui J, Wang C, Guo S, Yang L, Xiao P, Wang M. Evaluation of fungus-induced agilawood from Aquilaria sinensis in China. Symbiosis. 2013;60:37–44.
Gao ZH, Yang Y, Zhang Z, Zhao WT, Meng H, Jin Y, Huang JQ, Xu YH, Zhao LZ, Liu J, Wei JH. Profiling of microRNAs under wound treatment in Aquilaria sinensis to identify possible microRNAS involved in agarwood formation. Inter J Biol Sci. 2014;10(5):500–10.
Gibson I. The role of fungi in the origin of oleoresin deposits (Agaru) in the wood of Aquilaria agallocha. Roxb Bano Biggyan Patrika. 1977;6(1):16–26.
Gianno R. The exploitation of resinous products in a low land Malayan forest. Wallaceana. 1986;43:3–6.
Goel S, Shashi, Makrandi JK. Synthesis of 2-(2-phenylethyl) chromones. Indian J Chem 2006;45B:535–6.
Heuveling van Beek H, Phillips D. Agarwood: trade and CITES implementation in Southeast Asia. Unpublished report prepared for TRAFFIC Southeast Asia, Malaysia; 1999.
Henry Y, Burnell AC. “Eaglewood” Hobson-Jobson: a glossary of colloquial Anglo-Indian words and phrases, and of kindred terms, etymological, historical, geographical and discursive. 2nd ed. London: John Murray; 1903. p. 335.
Hu C, Lu W. Insight into yeast: a study model of lipid metabolism and terpenoid biosynthesis. Biotech Appl Biol Chem. 2015;62:323–8.
Ibrahim SRM, Mohamed GA. Natural occurring 2-(2-phenylethyl) chromones, structure elucidation and biological activities. Nat Prod Res. 2015;29:1489–520.
Ito M, Okimoto K, Yagura T, Honda G. Induction of sesquiterpenoid production by methyl jasmonate in Aquilaria sinensis cell suspension culture. J Essen Oil Res. 2005;17:175–80.
Ishihara M, Tsuneya T, Uneyama K. Components of the volatile concentrate of agarwood. J Essen Oil Res. 1993;5:283–9.
Jayaraman S, Mohamed R. Crude extract of Trichoderma elicits agarwood substances in cell suspension culture of the tropical tree, Aquilaria malaccensis Lam. Turk J Agric For. 2014;39:163–73.
Jayaraman S, Daud NH, Halis R, Mohamed R. Effects of plant growth regulators, carbon sources and pH values on callus induction in Aquilaria malaccensis leaf explants and characteristics of the resultant calli. J For Res. 2014;25:535–40.
Kazan K, Manners JM. Jasmonate signaling: toward an integrated view. Plant Physiol. 2008;146:1459–68.
Kiyoko M. The book of incense: enjoying the traditional art of Japanese scents. New York: Kodansha International Ltd.; 1992.
Kenmotsu Y, Ogita S, Katoh Y, Yamamura Y, Takao Y, Tatsuo Y, Fujino H, Kadota S, Kurosaki F. Methyl jasmonate-induced enhancement of expression activity of Am-FaPS-1, a putative farnesyl diphosphate synthase gene from Aquilaria microcarpa. J Nat Med. 2011;65:194–7.
Konishi T, Konoshima T, Shimada Y, Kiyosawa S. Six new 2-(2-phenylethyl) chromones from agarwood. Chem Pharm Bull. 2002;50(3):419–22.
Kumeta Y, Ito M. Characterization of δ-guaiene synthases from cultured cells of Aquilaria, responsible for the formation of the sesquiterpenes in agarwood. Plant Physiol. 2010;154:1998–2007.
Kusari AB, Molina DM, Sabbagh W, Lau CS, Lee B. A conserved protein interaction network involving the yeast MAP kinases Fus3 and Kss1. J Cell Biol. 2004;164(2):267–77.
Li W, Cai CH, Dong WH, Guo ZK, Wang H, Mei WL, Dai HF. 2-(2-phenylethyl) chromone derivatives from Chinese agarwood induced by artificial holing. Fitoterapia. 2014;98:117–23.
Li W, Cai CH, Guo ZK, Wang H, Zuo WJ, Dong WH, Mei WL, Dai HF. Five new eudesmane-type sesquiterpenoids from Chinese agarwood induced by artificial holing. Fitoterapia. 2015;100:44–9.
Lin F, Mei WL, Wu J, Dai HF. GC-MS analysis of volatile constituents from Chinese eaglewood produced by artificial methods. J Chinese Med Mat. 2010;33:222–5.
Liu Y, Chen H, Yang Y, Zhang Z, Wei J, Meng H, Chen W, Feng J, Gan B, Chen X, Gao Z, Huang J, Chen B, Chen H. Whole-tree agarwood-inducing technique: an efficient novel technique for producing high-quality agarwood in cultivated Aquilaria sinensis trees. Molecules. 2013;18:3086–106.
Ma DM, Pu GB, Lei CY, Ma LQ, Wang HH, Guo YW, Chen JL, Du ZG, Wang H, Li GF, Ye HC, Liu BY. Isolation and characterization of AaWRKY1, an Artemisia annua transcription factor that regulates the amorpha-4,11-diene synthase gene, a key gene of artemisinin biosynthesis. Plant Cell Physiol. 2009;50(12):2146–61.
Mohamed R, Jong PL, Zali MS. Fungal diversity in wounded stems of Aquilaria malaccensis. Fungal Divers. 2010;43:67–74.
Mohamed R, Wong MT, Halis R. Microscopic observation of ‘Gaharu’ wood from Aquilaria malaccensis. Pertanika J Trop Agric Sci. 2013;36(1):43–50.
Mohamed R, Jong PL, Ismail NI. Succession patterns of fungi associated to wound-induced agarwood in wild Aquilaria malaccensis revealed from quantitative PCR assay. World J Microbiol Biotechnol. 2014a;30:2427–36.
Mohamed R, Jong PL, Kamziah AK. Fungal inoculation induces agarwood in young Aquilaria malaccensis trees in the nursery. J For Res. 2014b;25(1):201–4.
Mucciarelli M, Camusso W, Maffei M, Panicco P, Bicchi C. Volatile terpenoids of endophyte-free and infected peppermint (Mentha piperita L.): chemical partitioning of a symbiosis. Microb Ecol. 2007;54(4):685–96.
Naef R. The volatile and semi-volatile constituents of agarwood, the infected heartwood of Aquilaria species: a review. Flavour Fragr J. 2011;26:73–89.
Ng LT, Chang YS, Kadir AA. A review on agar (gaharu) producing Aquilaria species. J Trop For Products. 1997;2:272–85.
Nimnoi P, Pongsilp N, Lumyong S. Actinobacterial community and diversity in rhizosphere soils of Aquilaria crassna Pierre ex Lec assessed by RT-PCR and PCR-DGGE. Biochem Syst Ecol. 2011;39:509–19.
Nobuchi T, Mohd Hamami S. The formation of wood in tropical trees: a challenge from the perspective of functional wood anatomy. Serdang: Penerbit Universiti Putra Malaysia; 2008.
Nobuchi T, Siripatanadilok SA. The formation of wood in tropical forest trees. In: Nobuchi T, Mohd Hamami S, editors. Cytological observations of Aquilaria crassna wood associated with the formation of aloes-wood. Serdang: UPM Press; 2008. p. 147–60.
Okugawa H, Ueda R, Matsumoto K, Kawanishi K, Kato A. Effect of jinkoh-eremol and agarospirol from agarwood on the central nervous system in mice. Planta Med. 1996;62:2–6.
Okudera Y, Ito M. Production of agarwood fragrant constituents in Aquilaria calli and cell suspension cultures. Plant Biotechnol. 2009;26:307–15.
Oldfield S, Lusty C, MacKinven A. The world list of threatened trees. Combridge, UK: World conservation press; 1998.
Page T, Awarau W. Performance of agarwood (Aquilaria crassna) seedling transplants improved by shade and fertiliser. For Ecol Manage. 2012;265:258–69.
Paoli GD, Peart DR, Leighton M, Samsoedin I. An ecological and economic assessment of the nontimber forest product gaharu wood in Gunung Palung National Park, West Kalimantan, Indonesia. Conservation Biol. 2001;15(6):1721–32.
Persoon GA. Agarwood: the life of a wounded tree. IIAS Newsletter. 2007;45:24–5.
Premalatha K, Kalra A. Molecular phylogenetic identification of endophytic fungi isolated from resinous and healthy wood of Aquilaria malaccensis. A red listed and highly exploited medicinal tree. Fungal Ecol. 2013;6:205–11.
Pitzschke A, Schikora A, Hirt H. MAPK cascade signalling networks in plant defence. Curr Opin Plant Biol. 2009;12:1–6.
Pojanagaroon S, Kaewrak C. Mechanical methods to stimulate aloes wood formation in Aquilaria crassna Pierre ex H Lec (Kritsana) trees. Acta Hort. 2005;676:161–66. doi: 10.17660/ActaHortic.2005.676.20
Qi SY, He ML, Lin DL, Zhang CH, Hu LJ, Zhang HZ. Production of 2-(2-phenylethyl) chromones in cell suspension cultures of Aquilaria sinensis. Plant Cell Tiss Org Cult. 2005;83:217–21.
Rahman MA, Khisa SK. Agar production in agar tree by artificial inoculation and wounding. II. Further evidences in favour of agar formation. Bano Biggyan Patrika. 1984;13:57–63.
Rahman MA, Basak AC. Agar production in agar tree by artificial inoculation and wounding. Bano Biggyan Patrika. 1980;9:87–93.
Rodriguez RJ, White JJF, Arnold AE, Redman RS. Fungal endophytes: diversity and functional roles. New Phytol. 2009;182(2):314–30.
Sadgopal, Varma BS. Agar oil from the wood of Aquilaria agallocha Roxb. 1952. Indian Forester. 78:26–33.
Santoso E. Agarwood formation by fungal bioinduction technology. In: Susmianto A, Turjaman M, Setio P, editors. Track record: agarwood inoculation technology by FORDA. Bogor: FORDA Press, 2013. 294 p.
Singh B, Sharma RA. Plant terpenes: defense responses, phylogenetic analysis, regulation and clinical applications. 3 Biotech. 2015;5:129–51.
Soehartono T, Mardiastuti A. The current trade in gaharu in West Kalimantan. J Ilmiah Biodiversitas Indonesia. 1997;1:1–10.
Sree N, Udayasri P, Kumar Y, Babu B, Kumar Y, Varma M. Advancements in the production of secondary metabolites. J Nat Prod. 2010;3:112–23.
Takemoto H, Ito M, Shiraki T, Yagura T, Honda G. Sedative effects of vapor inhalation of agarwood oil and spikenard extract and identification of their active components. J Nat Med. 2008;62:41–6.
Tamuli P, Boruah P, Nath SC, Leclercq P. Essential oil of eaglewood tree: a product of pathogenesis. J Essen Oil Res. 2005;17:601–4.
Thanh LV, Do TV, Son NH, Sato T, Kozan O. Impacts of biological, chemical and mechanical treatments on sesquiterpene content in stems of planted Aquilaria crassna trees. Agroforest Syst. 2015;89:973–81.
Tawfik HA, Ewies EF, El-Hamouly WS. Synthesis of chromones and their applications during the last ten years. Int J Res Pharm Chem. 2014;4(4):1046–85.
TRAFFIC East Asia-Taipei and TRAFFIC Southeast Asia. The trade and use of agarwood in Taiwan, Province of China. CITES; 2005.
TRAFFIC Oceania. Convention on International Trade in Endangered Species of Wild Fauna and Flora. Agarwood (Gaharu) harvest and trade in new Guinea [Papua New Guinea and the Indonesia province of Papua]. Twelfth meeting of the Plants Committee, CITES Decisions 11.112 and 11.113 regarding Aquilaria spp. Leiden; 2002. May 13–17.
Turjaman M, Santoso E. Progress research: agarwood cultivation and inoculation technology in Asia. In: Tampubolon AA, et al., editors. National seminar : non-timber forest products. Mataram, Lombok: Forest Research Institute for NTFPs; 2012. 500 p.
Wang JW, Wu JY. Effective elicitors and process strategies for enhancement of secondary metabolite production in hairy root cultures. Adv Biochem Eng Biotechnol. 2013;134:55–89.
Wang AM, Zhang FK, Huang LF, Yin XP, Li HF, Wang QY, Zeng ZW, Xie T. New progress in bio-catalysis and biotransformation of flavonoids. J Med Plants Res. 2010;4(10):847–56.
Wang SL, Hwang TL, Chung MI, Sung PJ, Shu CW, Cheng MJ, Chen JJ. New flavones, a 2-(2-phenylethyl)-4H-chromen-4-one derivative, and anti-inflammatory constituents from the stem barks of Aquilaria sinensis. Molecules. 2015;20(11):20912–25.
Wei JH, Yang Y, Zhang Z, Meng H, Feng JD, Gan BC. Liquid infusion method for producing linaloe on Aquilaria sinensis trees China Patent. CN101755629A; 2010.
Wijitphan P. Method to stimulate resin formation by wounding on the Aquilaria’s trunk. US Patent. US7485309–B1; 2009.
Wu B, Lee JG, Lim CJ, Jia SD, Kwon SW, Hwang GS, Park JH. Sesquiterpenoids and 2-(2-phenylethyl)-4H-chromen-4-one (=2-(2-phenylethyl)-4H-1-benzopyran-4-one) derivatives from Aquilaria malaccensis agarwood. Helv Chim Acta. 2012;95:636–42.
Wyn LT, Anak NA. Wood for the trees: a review of the agarwood (gaharu) trade in Malaysia. Petaling Jaya/Selangor/Malaysia: TRAFFIC Southeast Asia; 2010.
Xu YH, Wang JW, Wang S, Wang JY, Chen XY. Characterization of GaWRKY1, a cotton transcription factor that regulates the sesquiterpene synthase gene (+)-δ- cadinene synthase-A. Plant Physiol. 2004;135:507–15.
Xu Y, Zhang Z, Wang M, Wei J, Chen H, Gao Z, Sui C, Luo H, Zhang X, Yang Y, Meng H, Li W. Identification of genes related to agarwood formation: transcriptome analysis of healthy and wounded tissues of Aquilaria sinensis. BMC Genomics. 2013;14:1–16.
Yagura T, Shibayama N, Ito M, Kiuchi F, Honda G. Three novel diepoxy tetrahydrochromones from agarwood artificially produced by intentional wounding. Tetrahedron Lett. 2005;46:4395–8.
Yang DL, Wang H, Guo ZK, Li W, Mei WL, Dai HF. Fragrant agarofuran and eremophilane sesquiterpenes in agarwood ‘Qi-Nan’ from Aquilaria sinensis. Phytochem Lett. 2014;8:121–5.
Yoshii E, Koizumi T, Oribe T, Takeuchi F, Kubo K. The structure of agarotetrol, a novel highly oxygenated chromone from agarwood (jinko). Tetrahed Lett. 1978;19:3921–4.
Yu ZX, Li JX, Yang CQ, Hu WL, Wang LJ, Chen XY. The jasmonate responsive AP2/ERF transcription factors AaERF1 and AaERF2 positively regulate artemisinin biosynthesis in Artemisia annua L. Mol Plant. 2012;5:353–65.
Zhang XL, Liu YY, Wei JH, Yang Y, Zhang Z, Huang JQ, Chen HQ, Liu YJ. Production of high-quality agarwood in Aquilaria sinensis trees via whole-tree agarwood-induction technology. Chin Chem Lett. 2012;23:727–30.
Zhang Z, Han XM, Wei JH, Xue J, Yang Y, Liang L, Li XJ, Guo QM, Xua YH, Gao ZH. Compositions and antifungal activities of essential oils from agarwood of Aquilaria sinensis (Lour.) Gilg induced by Lasiodiplodia theobromae(Pat.) Griffon. & Maubl. J Braz Chem Soc. 2014a;25:20–6.
Zhang Z, Wei J, Han X, Liang L, Yang Y, Meng H, Xu Y, Gao Z. The sesquiterpene biosynthesis and vessel-occlusion formation in stems of Aquilaria sinensis (Lour.) Gilg trees induced by wounding treatments without variation of microbial communities. Int J Mol Sci. 2014b;15:23589–603.
Author information
Authors and Affiliations
Corresponding author
Editor information
Editors and Affiliations
Rights and permissions
Copyright information
© 2016 Springer Science+Business Media Singapore
About this chapter
Cite this chapter
Rasool, S., Mohamed, R. (2016). Understanding Agarwood Formation and Its Challenges. In: MOHAMED, R. (eds) Agarwood. Tropical Forestry. Springer, Singapore. https://doi.org/10.1007/978-981-10-0833-7_3
Download citation
DOI: https://doi.org/10.1007/978-981-10-0833-7_3
Published:
Publisher Name: Springer, Singapore
Print ISBN: 978-981-10-0832-0
Online ISBN: 978-981-10-0833-7
eBook Packages: Biomedical and Life SciencesBiomedical and Life Sciences (R0)