Abstract
Local site conditions may significantly affect the amplitude and frequency of ground motion during earthquakes. Recordings of recent major earthquakes have demonstrated that soil conditions can generate prominent amplification of ground shaking and can be compared with the amplification predicted by numerical simulation. This study analyzes the ground motion data from the December 2007 and March 2008 moderate Bala earthquake series considering the effect of soil conditions on ground shaking in the southern part of Ankara, the capital city of Turkey. Initially, shear wave velocity profiles of the strong ground motion stations were evaluated to define site classifications for each station in Ankara and its surroundings. Strong motion data collected during the Bala earthquake series (M w range 5.6–4.8) were used to develop event-specific attenuation relationships for peak ground acceleration and spectral acceleration at various periods and different site conditions. Site amplification factors were derived from the regression results from the event-specific attenuation relationships for the Bala earthquake series. The implications of these ground motion amplification factors were also discussed and compared using the current seismic design codes. Finally, the response spectra from the recording stations were used to determine site amplification factors for different site conditions to evaluate the variability of the site response in localized regions in Ankara.
Similar content being viewed by others
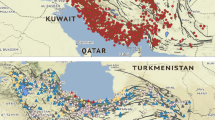
Avoid common mistakes on your manuscript.
Introduction
The Bala earthquake series that occurred in December 20–27, 2007 (M w = 5.7, 5.6, 5.2, 4.8) and March 15, 2008 (M w = 5.2) in the Ankara region have generated prominent interest in the earthquake science community because the Ankara region is considered to be quiet in terms of less frequent seismic activity where moderate earthquakes (5 < M w < 6) have rarely occurred in the inner part of Central Anatolia. However, this perception has changed subsequent to the Bala earthquake series that took place in the southeastern part of Ankara. Another implication is that even though the Bala earthquakes were moderate, they were felt strongly in Ankara which is situated about 50 km northwest of the epicenter. Hence, the effect of local site conditions might have influenced the strong ground motions during the Bala earthquakes. Because of these reasons, even though Ankara may be considered to be situated distant to large seismic activities, the influence of the local site conditions under earthquake-triggered motions, which significantly plays a crucial part in contributing to seismic damage, needs to be investigated. Therefore, the strong motion data from the Bala earthquakes and the impact of their sediment conditions are important because they provide invaluable information regarding ground shaking and site effects during moderate earthquakes. Additionally, ground motion data from the moderate earthquakes may supply valuable insight for comparing the larger ones because the motions are most likely to possess smaller intensity and the source could be less complex than the source mechanisms of larger earthquakes (Rathje 2004).
Since it is considered that seismic activity and relatively large magnitude events are less frequent, a limited number of strong motion stations have been installed within the Ankara region. The stations have generally been installed along the major Fault Systems where large earthquakes have occurred or within expected active areas with a distance of about 80–100 km away from Ankara (i.e., the North Anatolian Fault System). Therefore, the scarcity of strong motion data for the seismic events have made the attenuation of the earthquake ground motions difficult to characterize, thus leading to difficulty in seismic hazard estimation in this region. However, areas of high seismicity, such as the Marmara region in Turkey, present opportunities for characterizing the amplification of ground motions through analyses of strong motion data. The frequency of large magnitude events and the vast array of seismic instruments in operation may provide abundant data for the characterization of ground motions.
Considering the prominent interest and motivation mentioned above, the Ankara region, particularly the city of Ankara, needs to be monitored instrumentally with increased strong motion recordings to provide abundant data regarding ground motion characteristics and site effects during seismic events. With this purpose, Gazi University, Earthquake Engineering Implementation and Research Center (Gazi-DEMAR) has installed five strong and five weak ground motion stations in Ankara and its vicinity at the end of 2007 (Pampal et al. 2007). Fortunately, these stations were able to record some of the strong ground motion data during the Bala earthquake series. Additionally, the Prime Ministry and Emergency Management Presidency, Earthquake Department (AFAD-ED), has also installed temporary strong motion stations around the near-fault area just after the first Bala event in addition to the permanent ground motion network to enlarge the ground motion database.
Studies indicate that shear wave velocity is a significant component to determine the stiffness of the sediment characteristics along with the geometry of subsoil layers in determining the amplitude and frequency of ground shaking, and thus, it is a useful component to identify sediment characteristics quantitatively for determining site response (Wills et al. 2000; Holzer et al. 2005). An evaluation of these local sediment conditions is projected in building code provisions. Latest building code provisions, for instance, the International Building Code, IBC (International Code Council, ICC 2009), adapt site classification methods that utilize the average shear wave velocity (V s ) to a depth of 30 m, where V s (30) is a unique parameter for quantifying seismic sediment characteristics (Borcherdt 2002; Dobry et al. 2000). As an alternative, other site classification methods adopt V s results along with the subsurface lithology to quantify sediment characteristics, for instance, the Turkish Building Code (TBC 1998). Hence, characterizing the in situ measurement of shear wave velocities at strong motion stations and the impact of these soil conditions on the recorded motions is significant for evaluating site effects. Therefore, how soil conditions (i.e., the shear stiffness profile of the subsurface) may change the site amplification characteristic of ground shaking during seismic events can be identified quantitatively. In this study, in situ characterization of shear wave results was performed at strong motion stations using noninvasive seismic testing methods involving Rayleigh-type surface waves. The microtremor array method (MAM; Okada 2003; Hayashi 2008) and the multichannel analysis of surface wave method (MASW; Park et al. 1999) which supply correlative outputs, were utilized as passive and active surface wave techniques, respectively. By using these techniques together, the V s profile at the strong motion stations was acquired. Then, the shear wave velocity results conducted in this study were utilized to determine the site classifications for the strong motion stations.
These recorded strong motion data that have been obtained from the Gazi-DEMAR and AFAD-ED (National Strong-Motion Observation Network, TR-NSMN) network database for the Bala earthquake series represent invaluable information to evaluate ground motion characteristics and site effects during moderate earthquakes. The recorded strong motion data from the Bala earthquakes were used to evaluate the effect of soil conditions on ground shaking. Event-specific attenuation relations were developed for spectral acceleration at various periods within different site conditions to evaluate the variation of ground shaking. Finally, the response spectra from recording stations that are situated within 100 km of the fault were used to determine site amplification factors for different site conditions to evaluate the variability of the site response in localized regions in Ankara. Afterward, the resulting ground motion amplification factors were discussed and compared with the current design codes.
Site characterization of strong motion stations
In the southeast of Ankara, the Bala earthquake series have occurred with a moment magnitude of 5.7 and 5.6 in December 20 and 26, 2007, respectively (Fig. 1a; Earthquake Department of AFAD, AFAD-ED 2007). Apart from one station that is located at a distance of less than 65 km (AFAD-ED, Lodumlu station), unfortunately 10 and 12 strong motion stations at distances greater than 150 km of the faults have recorded these earthquakes, respectively. Therefore, the 5.7 magnitude earthquake event has been excluded from this study because of the limited strong motion data at distances less than 100 km. However, 5 strong motion stations of Gazi-DEMAR along with one station from AFAD-ED mentioned above recorded the 5.6 magnitude earthquake event. Afterward, two moderate earthquakes occurred with moment magnitudes of 5.2 (ED 2007) and 4.8 (Gazi-DEMAR 2007) in December 27, 2007 (Fig. 1a), respectively. Fortunately, a total of 8 and 9 strong motion stations, respectively, from DEMAR and AFAD-ED within 100 km of the fault have also recorded these earthquake events. The last earthquake event occurred with a moment magnitude of 5.2 in March 15, 2008, and a total of 7 strong motion stations within 100 km of the fault have recorded this earthquake event (Gazi-DEMAR and AFAD-ED 2008) (Fig. 1a). These strong motion stations that have recorded the Bala earthquakes are summarized in Table 1, along with the operating institution, the recorded earthquakes, the recorded peak ground acceleration and closest distance to the fault rupture. It should be noted that the shear wave velocity profiles used for this study were not readily available at most of the strong motion stations of AFAD-ED (2007) in the Ankara region due to various reasons (i.e., temporary stations, location change). In addition, the shear wave velocity profiles were measured at the strong motion stations of Gazi-DEMAR before this study (Can et al. 2013). The spatial distributions of these stations are given in Fig. 1a, b.
a Generalized tectonic map showing the large fault systems and numerous fault zones in the central part of Anatolia along with the location of strong motion stations and the Bala earthquake series in the study area. The tectonic features are from Koçyigit (2008a, b). b Enlarged frame of a showing the focal mechanism plots of fault plane solutions of the Bala earthquake series in the study area. Stars with the indicated numbers in the rectangular area display the epicenters of the Bala earthquake series that have occurred in the Ankara region (1–20.12.2007, M w : 5.7; 2–26.12.2007, M w : 5.6; 3–27.12.2007, M w : 5.2; 4–27.12.2007, M w : 4.8; 5–15.03.2008, M w : 5.2). Note that the same legend was also used in a
Source mechanisms of the Bala earthquake series
According to the recent active tectonic studies, the central part of Anatolia is under the influence of compressive stresses operating in approximately NW and N–S directions and includes numerous earthquake centers related to the intermediate zone of deformation between the northern and southern Anatolian ranges and to the faults which form the boundaries between these areas and the ranges (Koçyiğit 2009). This is the main cause of the strike-slip tectonic regimes and related faulting in Central Anatolia. In the central part of Anatolia, large fault systems and numerous fault zones are exposed. The well-identified earthquake sources are given in Fig. 1a. On a regional scale, this region might be affected by the surrounding large-scale fault systems, particularly the North Anatolian Fault System (NAFS), the Tuz Gölü Fault Zone (SLFZ) and the Seyfe Fault Zone (SFZ), which are capable of producing large destructive earthquakes (Koçkar 2006; Koçyiğit 2008).
Some of the prominent examples of the major events that have occurred along these systems are the November 26, 1943 Kastamonu earthquake (M L = 7.3), the February 1, 1944 Gerede earthquake (M L = 7.3) and the August 13, 1951 Çankırı earthquake (M L = 6.9) along the NAFS, and the March 19, 1938 Taşkovan-Akpınar earthquake (M L = 6.6, KOERI 2010) along the SFZ. However, in the inner part of Central Anatolia, namely in the Ankara region, the earthquake sources are considered to be quiet in terms of seismic activity where moderate earthquakes (5 < M w < 6) have rarely occurred. The recent Bala earthquake events of December 20, 2007 (M w = 5.7) and December 26, 2007 (M w = 5.6) along with their aftershock events (M w > 5) have reactivated the NW-trending Afşar Fault Zone. This is the first well-observed and well-recorded moderate-size earthquake activity (M w > 5.5) in the inner part of Central Anatolia.
To understand the fault mechanisms and source effect in this region caused by these earthquakes, fault plane solutions were developed by using faulting mechanisms data set for the December 20–27, 2007 (M w = 5.7, 5.6, 5.2, 4.8) and March 15, 2008 (M w = 5.2) Bala earthquake series (Tan et al. 2010). The focal mechanism plots of fault plane solutions of these earthquakes and their epicentral details are shown in Fig. 1b. Apart from one of them, the fault plane solutions of all of these earthquakes propose a right-lateral strike-slip faulting mechanism along the NW–SE directions. However, the December 27, 2007 (M w = 5.2) event suggests that the fault plane solution indicates the normal fault mechanism (SW dipping) along with the strike-slip component. This might be caused by local extensional forces between the strike-slip fault segments.
The seismological data revealed that the Bala earthquake events occurred on a NW–SE fault segment along the NW-trending Afşar Fault Zone. The fault plane solutions agree well with the NW-trending right-lateral strike-slip faulting (Fig. 1b). The fault plane solutions, the earthquake distributions and the fault pattern indicate that the major deformation zones trend in the NW–SE directions along with many different moderate-size fault concentrations causing a series of M w ≥ 5 earthquakes (Tan et al. 2010). Considering the epicentral distribution of these earthquake events, the major stress direction increasingly moves toward Ankara, the capital city of Turkey.
Due to the similar fault plane solutions and major fault deformation trend of the Bala earthquake series, the major stress direction in an account to estimate the source mechanism of these events relatively similar to each other reveals that only the magnitudes of the stresses rise or fall. Hence, by using these distinctive source mechanism similarities, event-specific attenuation relationships developed for each of the Bala events have been reliably considered together to discuss the implications of the site response characteristics.
Seismic testing methods
In situ characterization of the shear wave velocity profiles was performed at five of the Gazi-DEMAR strong motion stations along with seven of the AFAD-ED strong motion stations in the Ankara region using noninvasive seismic testing methods that rely on the dispersive nature of the Rayleigh-type surface waves in a layered media. The microtremor array method (MAM) (Okada 2003; Hayashi 2008) and the multichannel analysis of surface wave method (MASW) (Park et al. 1999) were utilized in this study as passive and active surface wave techniques, respectively, in order to obtain V s results in the vicinity of the strong motion stations. A combined utilization of these techniques was adapted to satisfy the demands of maintaining a reasonably high sensitivity of shear wave velocity results not only at shallower depths but also extending to the deeper depths (Park et al. 2007; Gosar et al. 2008; Eker et al. 2012).
In this study, the sources of the surface waves were generated by an active and passive way. In the active source, the energy is deliberately generated at a certain location according to the distribution of the geophone. Then, the recording starts when the active source of energy is applied at the surface. The MASW (Park et al. 1999) is categorized as an active surface wave technique. This is in contrast to passive source, motion from ambient noise generated by many natural sources such as wind, wave motion and man-made sources such as traffic, industrial machinery at different and usually unknown locations according to the geophone distribution that is recorded (Hayashi 2008). The microtremor array method (MAM) (Okada 2003; Hayashi 2008) is classified as a passive surface wave method. The selected equipment (i.e., source, geophone number and type) and testing configuration (i.e., offset distance, geophone interval and spread length) is closely based on the aim of the test and the technique to be applied in the study area. This study is particularly considered at a depth greater than 30 m based on recent building code provisions that are used to characterize site conditions at strong motion stations; hence, the appropriate equipment and configuration were selected based on that issue.
The important assumption regarding passive surface wave measurements is that the recorded microtremors are the surface waves which are the fundamental mode of Rayleigh waves (Asten and Boore 2005; Park et al. 2007). It should be noted that passive surface wave methods are named based on the utilized inversion method. The MAM is one of these methods based on spatial autocorrelation (SPAC) inversion (Okada 2003). Passive surface wave methods are used with different configurations to identify the shear wave velocities characterizing deeper layers (Asten and Boore 2005). The resolution of these methods, however, may be low at near-surface locations, and they are not appropriate at quiet locations and the locations where local data quality variations are dependent on proximity to ambient noise, as these conditions cause insufficient passive energy (Tokimatsu 1997; Rix 2005).
Although at various scales, the surface wave techniques are established on the geometrical dispersion, which makes propagation of Rayleigh wave dependent on the frequency in vertically heterogeneous media, high-frequency (short-wavelength) Rayleigh waves only penetrate into the shallow layers and they give knowledge on the mechanical properties of surface layers. However, low-frequency (long-wavelength) Rayleigh waves extend to the deeper parts of the layers and they contain information about material properties at greater depths. The surface wave techniques take this property to identify materials in a wide spectrum of depth (Foti et al. 2011).
Within the context of the geophysical characterization surveys in this study, the MASW and MAM were performed as active and passive surface wave measurements, respectively. The MAM allows gathering more accurate mechanical information of the geological units lying at deeper sections of a soil profile, while the MASW allows more rigorous solution of shear wave velocity profile at shallower depths.
Twenty-four surface wave surveys were obtained at 12 strong motion stations to evaluate the seismic character of the sediment profile in the Ankara region. In situ seismic testing results obtained from the active (MASW) and passive (MAM) surface waves were utilized together before the inversion process is termed as combined surface wave method (CSWM) as stated by Eker et al. (2010, 2012, 2013). All of the surveys were obtained based on a linear array alignment by using twelve 4.5 Hz vertical geophones with 1.5 m spacing for MASW and 10 m spacing for the MAM. All of the surveys were obtained by a GEODE seismograph with 12 channels.
During the active surface wave measurements of MASW, the source was triggered by using a trailer mounted 26.5 kgf drop weight. This impact source unit was developed at the Gazi University-DEMAR to generate surface waves with sufficient energy and the appropriate frequency content for MASW profiling to depths of 30–50 m (Can et al. 2013). Figure 2 shows a view of the drop weight source from the study area. The source is designed to be highly mobile, for rapid deployment, and to be safe for testing in urban environments. The trailer mounted source equipment is operated by a battery-powered electrical engine. It is triggered mechanically through an accelerated movement of the spring loading and able to apply forces between 100 and 1000 kgf by using a force measurement system. The source was triggered at both sides of the array alignment and the offset distances of 5, 10, 15 m as a forward and 5 m as a backward direction to provide lateral continuity of the homogeneity. Based on the general acceptance in the literature, the recording length of time was taken as 2 s with a 1 ms measurement spacing to estimate the surface waves.
In the passive MAM survey, a 10 min recording length was taken as a standard for each of the geophone measurements (Pullammanappallil et al. 2003). During the MAM measurements, the time interval of the sampling was chosen as 2 ms. The SPAC method was applied to obtain the dispersion curve (Okada 2003). Based on the nonlinear least square method, a one-dimensional inversion process has been implemented to construct the phase velocity curves and a V s profile that extended to a depth of 30 m was developed.
At the strong motion stations, a blind way technique was applied to calculate the V s (30) results. Hence, the geophone distance and type along with the offset size were chosen to evaluate the soil profile to a depth of 30 m. This might have led to an opportunity to correlate the results of the MASW and MAM that depend on different sources. Hence, the processed dispersion curves were fit into the dispersion data and shear wave velocity profiles were developed and used in the site classification at the strong motion stations (Fig. 3). These profiles gathered from a combination of the active and passive surface wave techniques give an indication of the lateral variability in the vicinity of the strong motion stations. The combined processed dispersion curves from MAM and MASW along with the corresponding V s profiles for all of the Gazi-DEMAR and AFAD-ED strong motion stations are given in Fig. 3. From Fig. 3, the consistency between these dispersion curves can be directly examined.
Site classification
The site classification methods proposed in this research are an attempt to account for the factors (i.e., local soil profile, stiffness of the sediment, depth of soil thickness) affecting seismic site response while decreasing the amount of data required for site characterization. Shear wave velocity profiles were processed from the combined utilization of the passive and active surface wave methods at 12 strong motion stations. Then, these V s profiles of the lithologic units have been utilized to classify the site classes based on the recent site classification systems which are applied to distinguish between different site conditions at strong motion stations.
The site classification systems mentioned herein are the IBC 2009 (International Code Council, ICC 2009) and the Turkish Building Code (TBC 1998). The IBC 2009 site classification describes site classes that depend on V s (30) (Table 2). The TBC site classification differentiates site classes that are based on an evaluation of the lithology of the surface stratum along with the shear wave velocity results. In this site classification system, the surface layer is assigned to a soil group based on its shear wave velocity (Table 3) and then the site class is assigned based on the soil group and the top layer thickness (Table 4).
The IBC 2009 and the TBC site classifications at the strong motion stations that recorded the Bala earthquake series along with the V s (30) results and surface geology are given in Table 5. It should be mentioned that the surface geology in the Ankara region is classified into three lithologies as Pre-Upper Miocene to Lower Pliocene rock units (referred to as rock in the text), Upper Pliocene to Pleistocene fluvial units (Plio-Pleistocene) and Quaternary terrace and alluvial units (Akyürek et al. 1997; Erol et al. 1980).
Considering the IBC results that depend on the subsurface geology, the V s (30) results at the strong motion stations indicated that the Plio-Pleistocene and Quaternary units fell into site class D, although the V s (30) results for these sediments were dissimilar statistically (Koçkar 2006; Koçkar and Akgün 2007, 2008). Actually, there was no inherent limit between these units as threshold site class values because the V s (30) results in the Quaternary deposits are directly affected from the stiffer deposits of Plio-Pleistocene within the depth of 30 m of the site. Generally, Quaternary alluvium is less than 30 m thick and is difficult to differentiate using surface seismic methods alone without any other supportive site characterization information (e.g., boring logs with engineering geological descriptions) (Koçkar et al. 2010). Additionally, the V s (30) results at the strong motion stations show that the site class boundaries of rock units in IBC (i.e., site class B and C) lead to difficulties in establishing direct relationships between site class and lithology. Therefore, site classes that depend on IBC may not sufficiently categorize these units as a distinct rock site (i.e., very dense soil and soft rock or firm rock). This problem that is related to distinct lithological units was also suggested by Wills et al. (2000) and Holzer et al. (2005) as an inadequacy in the definition of V s (30) threshold limits of the IBC-dependent site categorization. A similar methodology of combining V s (30) and lithology information was also utilized by other researchers (i.e., Park and Elrick 1998; Wills et al. 2000; Wills and Clahan 2006; Wills et al. 2015) in advancing site class information.
The V s results along with the top layer thicknesses are utilized by the TBC to assign site classes (Tables 3, 4). Results at the strong motion stations indicate that the Quaternary site contains soft sediments at the top layer (site class D) and is categorized as TBC Z3 and Z4. The Plio-Pleistocene sites are classified as site class Z3. Interestingly, the TBC site classes for the Plio-Pleistocene sites are site class Z3, which is stiffer than the IBC site class D. Another important comment concerning the TBC site classes is that the Quaternary units with similar TBC site classes tend to have smaller V s (30) values than the Plio-Pleistocene units. Hence, it is important to maintain lithological knowledge along with site class assignment such as the IBC site classification. Considering both of the code based site classification systems, it is clearly seen that the TBC results in more of the basing being characterized as the softest site class as compared with the IBC results due to the top soil layer being accounted exclusively (Koçkar et al. 2008, 2010). Therefore, the IBC and TBC site classes were considered together during this study for assigning the final site category at the strong motion stations.
Site effects
Local site conditions play a major role in the level of ground shaking and in gathering information on soil response (Koçkar and Akgün 2012; Eker et al. 2015). Soil stiffness and depth modify the frequency content, duration and intensity of shaking, which in turn affect the forces acting on structures and the damage pattern constituted by ground shaking. For this reason, it is significant to comprehend quantitatively how site conditions change the characteristics of ground shaking. It is therefore highly desirable to improve the methods or the techniques to characterize site conditions in order to account for the site effect.
This study presents an analysis of the ground motion data from the December 2007 and March 2008 Bala earthquake series in the southern part of Ankara considering the site effects within the Ankara region of Turkey. The recorded strong motion data for the Bala earthquake series represent invaluable information to evaluate ground motion characteristics and site effects during moderate earthquakes. Studies involving numerical simulation and recordings from the Bala earthquakes have been used to evaluate the effect of soil conditions on ground shaking for this study.
Event-specific attenuation relationships
Ground motion attenuation relationships that are generally utilized for empirical or theoretical estimates of expected ground motion from earthquakes are widespread in the literature. However, these researches are generally products of national or international scale including large region and generalized works generated from different earthquake source zones. For example, there are various ground motion-predictive models from Turkey. Especially following the 1999 Kocaeli and Düzce Earthquakes, there are various ground motion-predictive models from the Marmara Region and Turkey as well by considering the ground motion database of the recording stations in the Marmara Region and its vicinity (i.e., Özbey et al. 2004) and entire Turkey (i.e., Gülkan and Kalkan 2002; Kalkan and Gülkan 2004; Akkar and Çağnan 2010). Even though these ground motion models may constitute a well-developed general scientific opinion, they do not entirely reflect the variability of the site response for site-specific conditions or nonlinear soil behavior in localized geographic regions. Because of this reason, the significance of this study has become more prominent and has initiated generation of attenuation relationships worked toward the subject via developing event-specific hypothesis.
Apart from this research in the literature, event-specific attenuation studies are currently limited and one such study was performed for the settlement area from Bolu to Avcılar (Ambarlı) in the Marmara Region of Turkey covering the acceleration recording stations following the 1999 Kocaeli and Düzce Earthquakes (Rathje et al. 2003). Other examples of event-specific studies include Rodriguez-Marek et al. (2001) on the 1989 California-Loma Prieta and Northridge Earthquakes and Rathje (2004) and Rathje et al. (2005) on the 1999 Taiwan-Chi-Chi Earthquake and its aftershocks. These studies almost cover the limited event-specific studies on this subject. The relationships presented in these studies present variation of ground motion for each soil class with respect to distance and thus can be used for reliable estimation of site effect of soil conditions on ground shaking.
Strong motion data from the Bala earthquake series (M w range 5.6–4.8) were used to generate event-specific attenuation relationships for peak ground acceleration and spectral acceleration at various periods within different site conditions. The database consists of computed elastic spectral acceleration values at 5 % damping and peak ground accelerations. For each earthquake event, the number of recordings from the distances less than 100 km for each site class is shown in Table 6. In this table, C/B-Z2 (IBC/TBC) represents soft rock to weathered rock sites, C/C-Z2 represents very dense soil to soft rocks and a rock site is represented by a combination of these categories based on IBC and TBC. D/C-Z3 represents stiff soil, D/D-Z3 or Z4 represents stiff soil to soft soil and a soil site is represented by a combination of these two categories based on IBC and TBC. Table 6 indicates that, for the Bala Earthquakes, there is poor sampling of true rock sites (IBC-A or TBC A- or B-Z1). Only two recording stations are in the proximity of this category (the Çankaya station of Gazi-DEMAR and the Bala-District Police Headquarters station of AFAD-ED). Hence, similar rock categories were sometimes combined to increase the number of recordings for competent sites, although the general response characteristics of rock and very dense soil sites are different (i.e., Rodriguez-Marek et al. 2001). Additionally, to obtain more evenly distributed site categories, IBC stiff soil and TBC soft soil sites were generally combined since they both represent deep soil sites (Rathje et al. 2003).
Considering the distance range, strong motion data from the Bala earthquake series were not evenly distributed with the distance for all site classes. No data fell in the distance range of 0–10 km for any of the earthquakes. Apart from the magnitude of the 5.6 event, most of the strong motion data fell within a distance range of 10–80 km. For the 5.6 magnitude event, the minimum distance recorded was 40 km. In addition, the number of the recordings across site classes was not always in sufficient quantity. For instance, there were limited data for C Sites and D/D-Z4 soft soil sites for the entire earthquake events. For this reason, appropriate categories from the different rock (soft to weathered rock and very dense soil) and soil sites (stiff to soft soil) were also combined as a rock (BC Site) and soil class (D Site), respectively, based on IBC and TBC (Table 6). By doing this, the proposed site classification resulted in a sufficient reduction in the standard error developed in the event-specific attenuation relationships.
The functional form of the event-specific attenuation relationship used in this study is:
where In Y is the natural logarithm of the spectral acceleration at selected periods, T; R is the closest distance to the fault rupture plane in km, σ is an error term that was evaluated by using the ordinary least square method and c 1, c 2 and c 3 are regression coefficients. This functional form is similar to that utilized in the attenuation relationships of Abrahamson and Silva (1997) and Boore et al. (1997) and was also previously used in the attenuation relationships of Rathje (2004). Equation (1) was fit to PGA and Sa at periods of 0.3, 1.0, and 2.0 s, respectively, using the data gathered from strong motion stations within 100 km of the fault. Then, nonlinear regression analyses were performed for the rock and soil sites to obtain event-specific attenuation relationships for acceleration response spectral values at selected periods. For each strong motion station, the geometric mean of the two horizontal components was used in the regression.
Event-specific attenuation relationships were developed for each of the Bala earthquakes. Data from the distances between 1 and 100 km were considered because there is no data within the distance range of 0–1 km for the site classes. The regression coefficients and standard error terms for the site classes (rock and soil class) and each event are given in Table 7.
It should be noted that some of the coefficients in Eq. (1) were constrained to render reasonable relationships. The analyses resulted in large values of c3, which does not agree with the attenuation relationships in the literature. The coefficient c3 was also assumed to be nonnegative for all periods. Moreover, coefficient c3 was kept constant across site conditions to prevent the coupling of uncertainty in the coefficient c3 with the uncertainty in amplification factors (Abrahamson and Silva 1997; Rodriguez-Marek et al. 2001; Rathje 2004). In this regression study, the c3 parameter was calculated for each ground motion parameter using the data from all site categories in an effort to overcome the relatively small number of strong motions in each site category. Subsequently, c 1 and c2 were estimated for each site category utilizing the constrained value of c 3. This constraint was also necessary due to the poor sampling of the rock sites across all distances in the Bala earthquake events.
The event-specific functional form does not seize the directivity effects (Somerville et al. 1997) and hanging wall effect (Abrahamson and Somerville 1996). Directivity affects results in larger response spectral values of earthquakes (M > 6.5) at periods longer than one second for near-fault sites and for large pulse of motion to be oriented in the direction perpendicular to the fault. It presents a systematic bias for the affected near-fault sites. This bias, however, affects all near-fault sites, so the effect on amplification factors should be relatively small (Rodriguez-Marek et al. 2001).
Site amplification factors
The event-specific attenuation relationships were then used to generate site-dependent amplification factors according to a baseline site condition. As a standard practice, a rock site was used as the baseline site condition (Idriss 1991; Abrahamson and Silva 1997). The site-dependent amplification factors are a function of the intensity of rock motion (PGAROCK) and soil conditions (i.e., site class). In our case, this implies that amplification factors are obtained by dividing Eq. (1) for two site conditions. The resulting relationship is the spectral acceleration amplification factor at period T for soil site (D Site) with respect to rock (BC Site). During the evaluation of the amplification ratios (SaSOIL/SaROCK), the most important issue is the development of the event-specific attenuation relationships for the baseline site condition (i.e., rock) because this is the basis of the developed amplification ratios. As mentioned before, the poor sampling of rock data resulted in rock motions that did not give reasonable amplification factors. Hence, the constrained regression results for rock site (BC Site) were used, along with the regression results for soil site (D Site) to generate amplification factors for PGA and spectral acceleration at T = 0.3, 1.0 and 2.0 s, respectively.
Spectral amplification factors developed for each Bala event are shown in Fig. 4. For the sake of comparison, the implications of these ground motion amplification factors were also discussed utilizing a current seismic design code proposed by IBC (2009). In this code system, seismic design procedures prescribe acceleration response spectra for applying the seismic design. Also, site coefficients and maximum considered earthquake spectral response acceleration parameters of short- (F a ) and long-period (F v ) amplification factors adjusted for site class effects have been recommended. Hence, the amplification factors were also tabulated to compare with these current seismic design procedures in Fig. 4. It should be noted that considering the current seismic design provision of IBC (2009), amplification factors (D Site/BC Site) vary from 1.4 to 2.4 at short and long periods, respectively. Note that the categories of B and C Sites were combined as rock site of BC mentioned previously. Although, amplification factors recommended by IBC (2009) which were either B or C Site were not suitable for the BC Site category, straight-line interpolation can be used for intermediate values of amplification factors at short and long periods based on the code regulations. Then, amplification factors of BC Site were assigned as 1.4 within the proposed range limits of IBC (2009) at short periods. Considering Turkish design regulations, the implications of the ground motion amplification factors could not be discussed or compared with the current design procedure proposed by TBC (1998) since such issues are not considered by TBC (1998).
Figure 4 indicates that the Bala earthquake series showed some variability in the amplification factors. For PGA, the regression results indicated similar amplification factors for all of the Bala events. Apart from the M w = 4.8 event, almost similar results were found for spectral acceleration at T = 0.3 s. At shorter periods, the amplification factors of the Bala earthquakes tended to be relatively smaller than those proposed by IBC (2009). Interestingly, the smallest but evenly distributed event of the amplification factor of M w = 4.8 was higher than the larger events. These observations may attribute to the constrained values of the attenuation relationships due to poor sampling of the rock sites and/or lack of near-fault data for the Bala events. The amplification factors at short period proposed by IBC (2009) are also relatively larger than those obtained in this study. As mentioned before, the site classification scheme adopted for the IBC (2009) also differs from that obtained in this study; therefore, the assigned values of the amplification factors of IBC (2009) may be slightly higher than those obtained herein.
At longer periods (T = 1.0 and 2.0 s), the larger event amplification factors of M w = 5.6 and M w = 5.2 (27.12.2007_13:47) were higher than the other events and large amplification factors varying from 2.75 to 3.8 were derived. However, the variability of the data for M w = 5.2 (27.12.2007_13:47) and M w = 4.8 events were greater when compared to the other events (Table 6). For instance, at longer periods, M w = 5.2 (27.12.2007_13:47) event amplification factors were almost similar to the amplification factors of M w = 5.6; M w = 4.8 event amplification factors were higher than the larger events of M w = 5.2 (15.03.2008_10:15). Of all of the events, the more well-recorded and evenly distributed data for M w = 5.2 (27.12.2007_13:47) and 4.8 events provided consistent amplification factors at selected periods. Additionally, these events showed drastically larger amplification factors at longer periods, particularly at T = 2.0 s as compared to the amplification factors proposed by the IBC (2009). In the study area, soil response nonlinearity would also tend to increase the response at larger periods as a consequence of the softening of the site. In other words, the shift toward larger site periods depends on the soil type and input motion and the natural period of a soil site tends to increase due to a decrease in the shear wave velocity. At these sites, the site period might be shifted toward periods containing higher intensity input motion, resulting in large spectral accelerations with an associated increase in peak ground acceleration. Other factors might have also affected the data in this period range (i.e., fault source mechanisms, basin effects and surface waves). Additionally, the large period amplification factors from the obtained site classification in this study displayed more nonlinearity than the IBC code factors. This might be due to the consideration of all of the softest site class categories together for assigning a single soil category (i.e., D Site). If additional strong motion data would have been available, the softest site class categories would have been differentiated for further comparisons to understand the variability of the site response in a clearer fashion.
Conclusions
The strong motion data from the December 2007 and March 2008 Bala earthquake series were analyzed and used to develop event-specific attenuation relationships for PGA and spectral accelerations at periods T = 0.3, 1.0 and 2.0 s, respectively. The event-specific attenuation relationships were developed for each earthquake event using data recorded at distances less than 100 km. Despite the fact that the data set was relatively at a small number and generally clustered in the 10–80 km distance range, appropriate categories from the different rock and soil sites were also combined as a rock and soil site to obtain a more evenly distributed site classification scheme. Therefore, the proposed site classification resulted in an appreciable amount of reduction in the standard error when the event-specific attenuation relationships were developed.
In the site classification procedure proposed in this study, shear wave velocity profiles were developed from the combined usage of the active and passive surface wave methods for the strong motion stations. Then, these shear wave velocity profiles of the near-surface geologic units have been used to characterize site classes according to the IBC (2009) and the Turkish Building Code systems (TBC 1998). During the classification of the sites at the strong motion stations, the IBC and TBC site classes were considered together for assigning the final site category.
Strong motion data from the Bala earthquake series were used to develop earthquake event-specific attenuation relationships for peak ground acceleration and spectral acceleration at various periods for rock and soil site conditions. Amplification factors obtained from the event-specific attenuation relationships indicated that the Bala earthquake events displayed similar amplification factors at shorter periods, but these events generally revealed large amplification factors at larger periods. This trend is consistent with nonlinear soil behavior.
Spectral amplification factors developed for each Bala events were also discussed utilizing a current seismic design code proposed by IBC (2009) for the sake of comparison. Apart from the similarities between the developed and IBC (2009) site amplification factors at short periods, the amplification factors of IBC (2009) were slightly higher than those obtained in this study. This might be due to adopting the site classification scheme for the IBC (2009). On the contrary, the amplification factors of IBC (2009) were drastically smaller amplification factors at longer periods, particularly at T = 2.0 s as compared to the amplification factors proposed in his study. This trend is also consistent with nonlinear soil behavior.
This study showed that major seismic events that might take place around Ankara might affect the city center of Ankara and its surroundings. Although, the Bala earthquakes were moderate, they were felt strongly in Ankara, which is situated about 50 km northwest of the epicenter. Hence, the impact of local site conditions might have influenced the strong ground motions which might have tended to increase the response at larger periods as for the Bala earthquakes. Although Ankara may be considered to be situated distant to the major fault systems, the influence of the local soil conditions under earthquake-triggered motions is expected to play a major role in contributing to seismic damage. Therefore, the strong motion data from the Bala earthquake series and the effect of their soil conditions are significant because they supply invaluable information regarding ground shaking and site effects during moderate earthquakes.
References
Abrahamson NA, Silva WJ (1997) Empirical response spectral attenuation relationships for shallow crustal earthquakes. Seismol Res Lett 68(1):94–127
Abrahamson NA, Somerville PG (1996) Effects of the hanging wall and footwall on ground motions recorded during the Northridge earthquake. Bull Seismol Soc Am 86:S93–S99
AFAD-ED (2007–2008) Republic of Turkey Prime Ministry Disaster and Emergency Management Authority. Earthquake Department, Ankara. http://kyh.deprem.gov.tr/ftpt.htm
Akkar S, Çağnan Z (2010) A local ground-motion predictive model for Turkey, and its comparison with other regional and global ground-motion models. Bull Seismol Soc Am 100(6):2978–2995
Akyürek B, Duru M, Sütçü YF, Papak İ, Şaroğlu F, Pehlivan N, Gönenç O, Granit S, Yaşar T (1997) The surrounding geology of the city of Ankara and natural resources project. MTA compilation no. 9961 (in Turkish)
Asten MW, Boore DM (2005) Blind comparisons of shear-wave velocities at closely spaced sites in San Jose, California. U.S. Geological Survey Open-File Report-1169
Boore DM, Joyner WB, Fumal TE (1997) Equations for estimating horizontal response spectra and peak acceleration from Western North American earthquakes: a summary of recent work. Seismol Res Lett 68(1):128–153
Borcherdt RD (2002) Empirical evidence for site coefficients in building code provisions. Earthq Spectra 18(2):189–217
Can H, Koçkar MK, Felek G (2013) Evaluation of the site characterization of the weak and strong motion stations installed in Ankara and it’s surroundings. Gazi University, DEMAR. Gazi University, Scientific Research Project; BAP-06/2011-22 (in Turkish)
Dobry R, Borcherdt RD, Crouse CB, Idriss IM, Joyner WB, Martin GR et al (2000) New site coefficients and site classification system used in recent building seismic code provisions. Earthq Spectra 16(1):41–67
Eker AM, Akgün H, Koçkar MK (2010) A comparison of local site conditions with passive and active surface wave methods. In: Fifth international conference on recent advances in geotechnical earthquake engineering and soil dynamics symposium in Honor of Professor I. M. Idriss San Diego, CA
Eker AM, Akgün H, Koçkar MK (2012) Local site characterization and seismic zonation study by utilizing active and passive surface wave methods: a case study for the northern side of Ankara, Turkey. Eng Geol 151:64–81
Eker AM, Akgün H, Koçkar MK (2013) A comprehensive study to define local site effects in northern Ankara, Turkey. In: SE-50EEE international conference on earthquake engineering—29 to 31 May, Skopje, R. Macedonia
Eker AM, Akgün H, Koçkar MK (2015) Evaluation of site effect within the tectonic basin in the northern side of Ankara. Eng Geol 192:76–91
Erol O, Yurdakul ME, Algan Ü, Gürel N, Herece E, Tekirli E, Ünsal Y, Yüksel M (1980) Geomorphological map of Ankara. MTA report no: 6875 (in Turkish)
Foti S, Parolai S, Albarello D, Picozzi M (2011) Application of surface-wave methods for seismic site characterization. Surv Geophys 32:777–825
Gazi University, Earthquake Engineering Implementation and Research Center (GAZİ-DEMAR 2007 and 2008). Ankara. http://deprem.gazi.edu.tr/
Gosar A, Stopar R, Roser J (2008) Comparative test of active and passive multichannel analysis of surface waves (MASW) methods and microtremor HVSR method. RMZ Mater Geoenviron 55(1):41–66
Gülkan P, Kalkan E (2002) Attenuation modeling of recent earthquakes in Turkey. J Seismolog 6(3):397–409
Hayashi K (2008) Development of surface-wave method and its application to site investigations. Ph.D. Thesis, Kyoto University
Holzer TL, Bennett MJ, Noce TE, Tinsley JC (2005) Shear wave velocity of surficial geologic sediments in northern California: statistical distributions and depth dependence. Earthq Spectra 21(1):161–177
Idriss IM (1991) Procedures for selecting earthquake ground motions at rock sites. A report to the National Institute of Standards and Technology, University of California at Davis, September, revised March 1993
International Code Council, ICC (2009) International Building Code. Structural and fire- and life-safety provisions (seismic, wind, accessibility, egress, occupancy and roof codes), Whittier, CA
Kalkan E, Gülkan P (2004) Site-dependent spectra derived from ground motion records in Turkey. Earthq Spectra 20(4):1111–1138
Koçkar MK (2006) Engineering geological and geotechnical site characterization and determination of the seismic hazards of Upper Pliocene and Quaternary deposits situated towards the west of Ankara. Ph.D. Dissertation, Middle East Technical University, Ankara
Koçkar MK, Akgün H (2007) Evaluation of site characterizations and site effects of the Ankara Basin, Turkey. In: Kyriazis D. Pitilakis (eds) 4th International conference on earthquake geotechnical engineering-conference, June 25–28 Thessaloniki, Greece, Springer, Berlin. ISBN: 978-14020-5892-9, Paper ID: 1241: 1-10
Koçkar MK, Akgün H (2008) Development of a geotechnical and geophysical database for seismic zonation of the Ankara Basin, Turkey. Environ Geol 55(1):165–176
Koçkar MK, Akgün H (2012) Evaluation of site effects of the Ankara Basin, Turkey. J Appl Geophys 83:120–134
Koçkar MK, Akgün H, Rathje EM (2008) Evaluation of site conditions for the Ankara Basin of Turkey based on seismic site characterization. In: The 14th world conference on earthquake engineering, October 12–17, Beijing, China, ID No: 07-0197
Koçkar MK, Akgün H, Rathje EM (2010) Evaluation of site conditions for the Ankara basin of Turkey based on seismic site characterization of near-Surface geologic materials. Soil Dyn Earthq Eng 30:8–20
Koçyiğit A (2008) The earthquake sources in Ankara and its surroundings. Workshop for Earthquake Hazard and Risk of Ankara, Workshop Book, Gazi University, March 19, Ankara, pp 34–53 (in Turkish)
Koçyiğit A (2009) Seismicity of Ankara and source of the 2005–2007 Afşar (Bala-Ankara) earthquake. Map Mag 141(1):1–12 (in Turkish with English abstract)
KOERI (2010) Earthquake Records. Kandilli Observatory and Earthquake Research Institute. http://www.koeri.boun.edu.tr/sismo/map/tr/index.html
Okada H (2003) The microtremor survey method, Geophysical Monograph Series no. 12. Published by Society of Exploration Geophysicists (SEG), Tulsa
Özbey C, Sarı A, Manuel L, Erdik M, Fahjan Y (2004) An empirical attenuation relationship for Northwestern Turkey ground motion using a random effects approach. Soil Dyn Earthq Eng 24:115–125
Pampal S, Özmen B, Başkaya G, Gel AC, İnan E (2007) Development of weak and strong motion station in and around the Ankara. Gazi University, Scientific Research Project, BAP-00/2007-01 (in Turkish)
Park S, Elrick S (1998) Predictions of shear wave velocities in southern California using surface geology. Bull Seismol Soc Am 88:677–685
Park CB, Miller RD, Xia J (1999) Multi-channel analysis of surface waves. Geophysics 64(3):800–808
Park CB, Miller RD, Xia J, Ivanov J (2007) Multichannel analysis of surface waves (MASW)-active and passive methods. Lead Edge TLE 26(1):60–64
Pullammanappallil S, Honjas W, Louie JN (2003) Determination of 1-D shear wave velocities using the refraction microtremor method. In: Proceedings of the third international conference on the application of geophysical methodologies and NDT to transportation and infrastructure, Orlando, Florida
Rathje EM (2004) Evaluation of site effects during the 1999 Chi-Chi Earthquake and its Aftershocks. Final Project Report to United States Geological Survey Earthquake Hazard Reduction Program; USGS- Award No. 03HQGR0040
Rathje EM, Stokoe KH, Rosenblad BL (2003) Strong motion station characterization and site effects during the 1999 Earthquakes in Turkey. Earthq Spectra 19(3):653–676
Rathje EM, Koçkar MK, Özbey MC (2005) Observed site effects during the 1999 Chi-Chi Earthquake and its aftershocks. Seismol Res Lett 76(2):238
Rix GJ (2005) Near-surface site characterization using surface waves, surface waves in geomechanics—direct and inverse modeling for soil and rocks. Lai CG, Wilmanski K (ed) CISM Lecture Notes, Springer, Wien-Newyork
Rodriguez-Marek A, Bray JD, Abrahamson NA (2001) An empirical geotechnical seismic site response procedure. Earthq Spectra 17(1):65–87
Somerville PG, Smith NF, Graves RW, Abrahamson NA (1997) Modification of empirical strong ground motion attenuation relations to include the amplitude and duration effects of rupture directivity. Earthq Spectra 17(1):199–222
Tan O, Tapırdamaz C, Ergintav S, İnan S, İravul Y, Saatçılar R et al (2010) Bala (Ankara) earthquakes: implications for shallow crustal deformation in Central Anatolian Section of the Anatolian Platelet (Turkey). Turk J Earth Sci 19:449–471
Tokimatsu K (1997) Geotechnical site characterization using surface waves. In: Ishihara K (ed) Proceedings of 1st international conference on earthquake geotechnical engineering. Balkema, Rotterdam. ISBN: 90 5410 578X, 3:1333-1368
Turkish Building Code (1998) Specification for structures to be built in disaster areas. Prime Ministry and Emergency Management Presidency, Earthquake Department (formerly Ministry of Public Works and Settlement, General Directorate of Disaster Affairs), Ankara, Turkey (in Turkish)
Wills CJ, Clahan KB (2006) Developing a map of geologically defined site- condition categories for California. Bull Seismol Soc Am 96(4A):1483–1501
Wills CJ, Petersen M, Bryant WA, Reichle M, Saucedo GJ, Tan S et al (2000) A site- conditions map for California based on geology and shear wave velocity. Bull Seismol Soc Am 90(6B):S187–S208
Wills CJ, Gutierrez CI, Perez FG, Branum DM (2015) A next generation VS30 map for California based on geology and topography. Bull Seismol Soc Am 105(6):1–9
Acknowledgments
The author would like to express his gratitude to Professor Haluk Akgün for his invaluable comments and suggestions that helped to improve the manuscript. The author would also like to express his gratitude to Mr. A. Mert Eker and Mr. Karim Yousefi-Bavil who aided in the processing of the surface wave testing results, to Mr. Hakan Felek who provided field assistance and conducted the surface seismic testing studies and to Mrs Arzu Arslan-Kelam who provided assistance. Additionally, the author would like to acknowledge Mr. Ulubey Çeken who supplied the strong motion data from the temporary stations of AFAD-ED that were installed during the Bala earthquake series. The author would like to acknowledge the anonymous Reviewers for the excellent comments and suggestions that led to the improvement of the manuscript.
Author information
Authors and Affiliations
Corresponding author
Rights and permissions
About this article
Cite this article
Koçkar, M.K. Site characterization of the strong motion stations and evaluation of site effects in the Ankara region during the December 2007 and March 2008 moderate Bala earthquakes. Environ Earth Sci 75, 1128 (2016). https://doi.org/10.1007/s12665-016-5921-x
Received:
Accepted:
Published:
DOI: https://doi.org/10.1007/s12665-016-5921-x