Abstract
Neoadjuvant chemoradiation has been a standard of care for locally advanced rectal cancers. Recent reports suggest that a pathologic complete response to neoadjuvant treatment correlates to improved overall survival. In addition, some series suggest that patients who have a complete response to neoadjuvant therapy may safely defer surgery in favor of a “watch and wait” approach, therefore avoiding the potential complications and adverse bowel function associated with surgery. It is therefore important to understand the clinical and biologic factors which affect the response of rectal cancers to chemoradiation. This review highlights the current literature examining the biomarkers of tumor response to chemoradiation.
Similar content being viewed by others
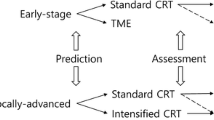
Avoid common mistakes on your manuscript.
Introduction
Despite improvements in cancer screening as well as treatment, colorectal cancer remains the third most commonly diagnosed cancer as well as the third leading cause of death due to cancer in the USA and worldwide [1, 2]. In 2016, there were 95,000 new cases of colon cancer and 39,000 cases of rectal cancer [2].
Locally advanced rectal cancer includes patients that have tumors that have invaded through the muscularis propria, those that penetrate the visceral peritoneum or adjacent organs, and node positive disease. One standard treatment paradigm for locally advanced rectal cancer is neoadjuvant chemoradiation with fluoropyrimidine-based chemotherapy, followed by total mesorectal excision (TME). Several landmark trials established this treatment paradigm including a study by the German Rectal Cancer Study Group that demonstrated increased local control with pre-operative chemoradiation with fluorouracil (5-FU) when compared to post-operative chemoradiation [3•]. The Trans-Tasman Radiation Oncology Group and the Polish Colorectal Study Group compared neoadjuvant short-course radiation therapy (RT) and long-course chemoradiation in separate prospective randomized trials. While both trials failed to show a difference in overall survival, long-course chemoradiation was associated with greater pathologic downstaging and pathologic complete response (pCR) rates [4, 5]. In studies of neoadjuvant therapy for rectal cancers, the pCR rate has been reported as approximately 10–49% and correlates with overall survival [6•, 7, 8]. The intensification of pre-operative treatment has been examined in clinical trials. The addition of oxaliplatin to 5-fluorouracil (5-FU) increases the pCR rate as does “extended” chemoradiation, which includes additional cycles of neoadjuvant chemotherapy prior to surgery [9•, 10•]. However, these potential benefit of intensified therapy must be balanced by the potential risks, and it is critical to identify patients who will derive the most benefit from additional treatment.
Total mesorectal excision has been the standard of care in the operative management of locally advanced rectal cancer, as established by the Dutch TME trial [11]. The operative technique includes low anterior resection (LAR) or abdominoperineal resection (APR) as determined by the location and extent of the tumor. However, there can be significant morbidity with rectal surgery, and preserving optimal bowel function may not always be possible with such surgery. As a result, the carefully selected use of non-operative management (NOM) or a “watch and wait” approach for rectal cancers—using surgery only as a salvage for local recurrence—has been examined [12, 13]. Habr–Gama and colleagues reported their single institutional series of NOM after long-course chemoradiation where clinical complete response (cCR) was assessed by physical exam, CEA levels, and when available, abdominopelvic imaging and EUS [14, 15••]. Interestingly, local recurrence occurred in 31% of patients with a cCR within the first year or later but salvage surgery was possible in 93% of these patients, with an overall local recurrence-free survival of 94% at 5 years with salvage therapy [6•]. Others, including series from the Netherlands and Memorial Sloan Kettering, report similarly promising outcomes with NOM [16•, 17]. These reports are limited by variations in restaging and assessment of cCR, as illustrated by discrepancies found upon pathologic assessment of “non-responders” or local failures [15••, 16•]. In addition, careful and appropriate patient selection is essential for the successful application of this non-operative treatment paradigm.
While NOM continues to be examined in prospective trials, ultimately, the efficacy of this approach is dependent on more reliable clinical and biologic predictors of pCR in patients treated with chemoradiation, as well as on improved radiographic and clinical assessment of cCR. Better understanding of the clinical characteristics that determine treatment response would allow tailored neoadjuvant approaches depending on the probability of achieving pCR. Moreover, it is increasingly critical to define biomarkers that can predict the response to chemoradiation that may be used to guide patient selection and tailored treatment options. Here, we review recent progress in identifying biomarkers that may predict treatment response in the current the molecular and microenvironmental subtypes of colorectal cancer.
Gene Expression
Gene expression profiling is a powerful tool to identify differentially expressed genes in patients based on their response to treatment. Improvements in microarray analysis and decreased costs have allowed oncologists to use gene expression data from tests such as Oncotype DX and MammaPrint to guide clinical decision-making in breast cancer [18]. Similarly, for stage II colon cancer, a 12-gene recurrence score was developed to assess patients who may benefit from adjuvant chemotherapy [19]. Several published reports have attempted to identify gene expression signatures linked to treatment response in rectal cancer. However, these studies rely on small samples and have little concordance beyond the emergence of common pathways such as DNA damage repair. There is also limited data specifically addressing the question whether pre-treatment gene expression can predict pCR after chemoradiation. A study by Kim et al. used microarray data from a training set of 31 patients consisting of 20 patients with a partial response to chemoradiation and 11 patients with a complete response to chemoradiation and a validation cohort of 15 patients [20]. They generated a set of 95 genes displaying differential expression between partial response and complete response to predict response to chemoradiation. Complete response and partial response were accurately predicted in 84% of the 31 training samples and 87% of the 15 validation samples. Brettingham–Moore et al. analyzed the pre-treatment gene expression of 51 patients with locally advanced rectal cancer and correlated this with pathologic and PET/CT metabolic response to chemoradiation [21]. Using a supervised learning algorithm, they generated a predictive classifier and attempted to validate previously published classifiers on their cohort and other data sets including the aforementioned study by Kim et al. [20, 22•, 23]. The authors were unable to identify a significant correlation between response and gene expression signature in their cohort. However, using Ingenuity Pathway Analysis, they did find commonly implicated pathways between their dataset and the other studies including the TNF signaling pathway and the β-estradiol signaling network. Lopes–Ramos and colleagues attempted to identify expression signatures capable of predicting pCR using RNA-Seq analysis of 25 pre-treatment biopsies from patients who underwent 5FU-based neoadjuvant chemoradiation [24]. They identified 27 differentially expressed genes by patients with pCR; however, these signatures had poor predictive value. Currently, the lack of concordance between studies, likely based on limited sample size of patients, indicates that gene expression analysis requires further investigation.
Colorectal cancers are genetically heterogenous, and there is the potential to classify colorectal cancers based on gene expression. Recently, the Colorectal Cancer Subtyping Consortium (CRCSC) used several large datasets including The Cancer Genome Atlas (TCGA) to generate a classification of five consensus molecular subtypes (CMS) in colorectal cancer [25••]. Patients in CMS1 were characterized by hypermutation, microsatellite instability, and strong immune activation; CMS2 had marked WNT and MYC signaling activation; CMS3 was defined by metabolic dysregulation; and CMS4 had prominent transforming growth factor-β activation, stromal invasion, and angiogenesis. This transcriptomic classification system of colorectal cancers may reveal a CMS signature with differential sensitivity to neoadjuvant therapies or reveal genes associated with a treatment response if patients are better defined by CMS subgroup.
Circulating Tumor Markers
Carcinoembryonic antigen (CEA) is a current serum marker for colorectal cancers. CEA is a cell membrane glycoprotein normally expressed by endodermally derived cells in the digestive tract during fetal development and is elevated in a number of epithelial malignancies including colorectal cancer. While it can be a useful non-invasive marker, a Cochrane review of 52 studies using CEA to detect colorectal cancer recurrence found that the lack of sensitivity requires that it be used in conjunction with other diagnostic modalities such as imaging [26]. Currently, CEA levels are primarily used clinically in surveillance and monitoring treatment response in metastatic colorectal cancer. However, baseline CEA levels have also been explored as a predictor of neoadjuvant treatment response. A large retrospective review of 530 patients with rectal adenocarcinoma treated with pre-operative chemoradiation followed by TME was performed looking at the correlation of CEA level and tumor response [27]. The majority of patients in this study received 5-FU with a radiation dose of at least 5040 cGy, which was delivered with a four-field technique from 1998 to 2005 and using intensity-modulated radiation therapy (IMRT) from 2006 to 2011. Overall, they found 20% of patients achieved a pCR. In non-smokers with a pCR, the average pre-treatment CEA level was 2.9 ng/mL when compared to patients who did not achieve a pCR where the CEA level was 8.3 ng/mL. Additionally, those who received a reduced dose of neoadjuvant therapy due to toxicity or side effects were less likely to achieve a pCR. While interesting, this study is limited by the fact that only 57% of the included patients had CEA levels available for analysis. A similar large study conducted at the MD Anderson Cancer Center found that in patients where the data was available, pre-treatment carcinoembryonic antigen (CEA) level >2.5 ng/mL was associated significantly with lower pCR rates on univariate but not multivariate analysis (P = .015) [28]. Another single institution series of 323 patients in China, all with CEA levels recorded, found on multivariate analysis that a CEA level of ≤5 ng/mL was an independent predictor of pCR to chemoradiation [29].
The ratio of pre- to post-treatment CEA levels have also been investigated as a predictor of chemoradiation response. A review of a prospectively maintained database of 141 patients found that in the 13.5% of patients with a pCR, low post-treatment levels of CEA, but not pre-treatment levels, were predictive of pCR [30]. Yang et al. found that in patients with baseline CEA levels >6 ng/mL, both the post-treatment and CEA ratio predicted pCR with a sensitivity of 87.5% and specificity of 76.7% [31].
Circulating cell-free nucleic acids including cell-free DNA (cfDNA) and cell-free RNA (cfRNA) are present in healthy individuals but have also been examined in cancer patients. It has been proposed that in normal conditions, cfDNA consists of smaller fragments from apoptotic cell death, whereas cfDNA derived from tumor necrosis is of large or variable size. Thus, cfDNA level and the integrity index, or ratio between longer and shorter DNA fragments, may be of interest in monitoring treatment response. Agostini et al. investigated the use of cfDNA as a biomarker of response in 63 patients treated with chemoradiation [32]. They found clear differences in levels of cfDNA between healthy individuals and those with rectal cancer. Pre-treatment levels of cfDNA were not associated with treatment response; however, the integrity index was independently associated with response. In another study of 98 patients, lower post-treatment levels of cfRNA and a greater decrease in cfRNA levels independently predicted the tumor response. A similar relationship with the plasma levels of human telomerase reverse transcriptase (hTERT) was also observed [33].
Tumor Microenvironment and Immunity
A promising area of investigation is the role of the immune system and the tumor microenvironment in treatment response. There has been clinical success in tumor immunology, most notably with checkpoint inhibitors, and it is increasingly appreciated that immunologic features can be predictive of outcomes as well a drive development of novel therapies. Interestingly, Galon and colleagues demonstrated that the pattern of tumor immune infiltrate has a significant association with patient outcomes in colorectal cancer [34••]. Using tissue microarrays, they quantified cytotoxic CD8+ tumor-infiltrating T cells (TIL) and of memory T cells in the center of the tumors and at the invasive margin of colorectal tumors to generate an Immunoscore. Notably, they found that their Immunoscore had better prognostication of patient survival than the TNM staging system for colorectal cancers [35, 36]. In locally advanced rectal cancer, patients with pre-operative biopsies with high infiltration of CD3+ or CD8+ T cells were more likely to have a partial or complete response to neoadjuvant therapy compared to those with low densities, 72 vs. 28% [37••]. This work highlights the potential for immunologic characteristics including the Immunoscore to predict clinical outcomes.
High expression of PD-L1 has been linked to poor prognosis in gastrointestinal malignancies and can determine patient selection for checkpoint inhibitors [38]. Saigusa et al. found that in 90 patients who underwent neoadjuvant chemoradiation, high PD-L1 expression was associated with vascular invasion and less infiltrating CD8 T cells was an independent risk factor for tumor recurrence [39]. High density of regulatory T cells (Treg) has been linked to poor prognosis and may inhibit anti-tumor immunity. Shinto et al. found that in pre-treatment biopsies, a high ratio of CD8+ T cells to FoxP3+ Treg was associated with tumor regression [40]. However, other investigators reported that while post-treatment low stromal Treg density was associated with pCR when they analyzed the pre-treatment biopsies from the same cohort, there was no T cell subset that was significantly associated with clinical outcome [41, 42].
Circulating immune cells have also been used as a gauge of systemic immune responses. For example, the neutrophil to lymphocyte ratio (NLR) is a marker of inflammation and a pre-operative prognostic factor in colorectal cancer [43]. However, only 47% of the patients in this study had rectal cancer and included a significant number of patients with more advanced disease. In a study examining the predictive value of NLR, Kim et al. found that elevated NLR (defined as >3) was predictive of a poor response to treatment, as was an elevated CEA or tumor diameter >3 cm [44]. Other studies have also found an association between higher NLR and poor response to neoadjuvant chemoradiation [45, 46]. A study of 51 patients with rectal cancer who underwent pre-operative chemoradiation identified only pre-treatment relative lymphocyte count as significantly associated with tumor downstaging [47]. Conversely, others have reported that NLR does not correlate with response in locally advanced rectal cancer [48, 49]. Thus, the prognostic value of NLR remains unclear and requires further study.
Molecular Imaging
Advanced functional imaging has also added to the evaluation of the initial staging of colorectal cancers, as well as to the evaluation of treatment response. The value of MRI in local staging of rectal cancer is widely accepted. Moreover, the Mercury Study Group established that MRI is an excellent technique for restaging and assessment of circumferential resection margins [50]. They went on to evaluate this in a multiinstitutional prospective trial and found that tumor within 1 mm of the circumferential resection margin on MRI was predictive of worse overall survival, disease free survival, and local recurrence [51••]. Advances in functional MRI techniques has allowed for the evaluation of microscopic properties of tumors and their microenvironment. For example, using the mobility of water protons, diffusion-weighted MRI (DW-MRI) and the apparent diffusion coefficient (ADC) can provide insight into tissue cellularity, structural organization, and extracellular spaces. In rectal cancer, DW-MRI may be helpful in detecting residual tumor after chemoradiation but has also been examined as a tool to predict treatment response. Several studies have reported that low pre-treatment ADC or greater change in ADC with treatment is associated with a tumor response [52,53,54]. Dynamic contrast-enhanced MRI (DCE-MRI) uses contrast to evaluate perfusion of tissues and vascular permeability. In DCE-MRI, Ktrans is a parameter that describes tumor vascularity and permeability. Accordingly, high Ktrans values have been found as a predictor of good response as it is hypothesized that tumors with higher Ktrans may be better oxygenated and allow delivery of chemotherapy [55,56,57]. While these results are promising, the use of functional MRI to predict treatment response remains investigational and the predictive value should be established in larger series.
Positron emission tomography–computed tomography (PET/CT) with F-18 fluorodeoxyglucose (FDG) is used in functional imaging of the increased glucose metabolism in malignant cells. Martoni et al. evaluated 80 patients using FDG-PET/CT at baseline and after pre-operative chemoradiation [58]. Interestingly, they found that non-responders had a higher baseline standard uptake value (SUV). The pre-treatment SUV, post-treatment SUV, and change in SUV before and after treatment, had high sensitivity but poor specificity as a predictor of pCR. Similarly, several studies have found that the change in SUV is not correlated with pCR while others reported that a SUV-based regression index may have utility in assessment of a treatment response [59] [60, 61]. However, FDG-PET/CT may be examined in a larger prospective study to determine the most significant imaging parameters and relevant time points for imaging in predicting responses.
Conclusion
Despite modest progress in identification of factors that may predictors of chemoradiation response in rectal cancer, it remains to be seen if this can be accomplished though molecular, serologic, immunologic, or radiologic features. Examination of pre-operative characteristics has thus far lacked the sensitivity and specificity to be used in clinical practice. Many of these studies are limited by small sample sizes, and the inherent underlying biologic heterogeneity of rectal tumors may be reflected by the range of reported pCR rates despite similar neoadjuvant therapy [7, 8]. The CRCSC generated molecular subtypes may provide a framework in which we might account for heterogeneity and prospectively examine biomarkers [25••]. However, this may be an evolving classification as it has been demonstrated that intratumoral heterogeneity can influence the assignment of tumor transcriptional subtype and a better understanding of the mixed phenotypes may emerge [62•].
Analysis of the tumor microenvironment in pre-treatment biopsies is promising and highlights the importance of the immune system in treatment response. Quantification of the density of CD8+ and memory T cells using the Immunoscore may provide better prognostication than TNM staging [37••]. Early studies with this technique revealed that pre-treatment biopsies had nearly threefold greater infiltration of T cells in patients who went on to have a pCR [37••]. In addition, Saigusa et al. found an inverse relationship between PD-L1 expression and T cell infiltration and others have found that chemoradiation increases the intratumoral density of CD8+ T cells [39, 40]. Most tumors with microsatellite instability (MSI) cluster within the CRCSC generated CMS1 and are also enriched BRAFV400E mutations as well as immune infiltrate. In a phase II trial using pembrolizumab, MSI predicted a response to immunotherapy in metastatic colorectal cancer [63]. Thus, CMS1 and MSI may represent a potential strategy for patient selection in adding immunotherapy to the current standard of care. Moreover, in patients with a low density of T cells or high PD-L1 expression on pretreatment biopsy, the addition of immunomodulation to chemoradiation could improve the rate of pCR and should be evaluated in clinical trials. This is an active area of investigation, and a phase II trial of neoadjuvant chemoradiation and pembrolizumab is underway in patients with stage II–III rectal cancer (NCT02586610).
When evaluating predictors of treatment response, timing is an important consideration. The interval between chemoradiation and surgery may also play a role in the rate of pCR as radiation-induced cell death and tumor regression is time dependent. Some studies have found that a longer interval between completion of neoadjuvant therapy and surgery is associated with higher rates of pCR [29, 64, 65].
A meta-analysis attempted to define treatment-related factors that were associated with pCR, and the pCR rate is associated with a radiation dose of at least 45 Gy and with the administration of 5-FU and a second chemotherapy [66]. Additionally, estimated dose response curves for pre-operative chemoradiation demonstrate a significant relationship with tumor regression with dose levels in the range of 50.4 to 70 Gy [67].
Biomarker characterization off chemoradiation response may allow us to more accurately identify patients for intensification of neoadjuvant therapy or, conversely, who may be suitable for chemoradiation with non-operative management. This has the potential to greatly improve rectal cancer patient outcomes by tailoring appropriate treatment based on the probability of tumor response. Ultimately, our understanding and clinical use of these biomarkers necessitate larger cohort studies and incorporation into prospective clinical trials.
References
Papers of particular interest, published recently, have been highlighted as: • Of importance •• Of major importance
Global Burden of Disease Cancer C, Fitzmaurice C, Dicker D, Pain A, Hamavid H, Moradi-Lakeh M, et al. The global burden of cancer 2013. JAMA Oncol. 2015;1(4):505–27. doi:10.1001/jamaoncol.2015.0735.
Siegel RL, Miller KD, Jemal A. Cancer statistics, 2016. CA Cancer J Clin. 2016;66(1):7–30. doi:10.3322/caac.21332.
• Sauer R, Liersch T, Merkel S, Fietkau R, Hohenberger W, Hess C, et al. Preoperative versus postoperative chemoradiotherapy for locally advanced rectal cancer: results of the German CAO/ARO/AIO-94 randomized phase III trial after a median follow-up of 11 years. J Clin Oncol. 2012;30(16):1926–33. doi:10.1200/JCO.2011.40.1836. This trial established the use of neoadjuvant chemoradiation in locally advanced rectal cancer.
Ngan SY, Burmeister B, Fisher RJ, Solomon M, Goldstein D, Joseph D, et al. Randomized trial of short-course radiotherapy versus long-course chemoradiation comparing rates of local recurrence in patients with T3 rectal cancer: Trans-Tasman Radiation Oncology Group trial 01.04. J Clin Oncol. 2012;30(31):3827–33. doi:10.1200/JCO.2012.42.9597.
Bujko K, Nowacki MP, Nasierowska-Guttmejer A, Michalski W, Bebenek M, Kryj M. Long-term results of a randomized trial comparing preoperative short-course radiotherapy with preoperative conventionally fractionated chemoradiation for rectal cancer. Br J Surg. 2006;93(10):1215–23. doi:10.1002/bjs.5506.
• Habr-Gama A, Gama-Rodrigues J, Sao Juliao GP, Proscurshim I, Sabbagh C, Lynn PB, et al. Local recurrence after complete clinical response and watch and wait in rectal cancer after neoadjuvant chemoradiation: impact of salvage therapy on local disease control. Int J Radiat Oncol Biol Phys. 2014;88(4):822–8. doi:10.1016/j.ijrobp.2013.12.012. A key study demonstrating the feasibility of the watch and wait approach with early salvage surgery.
Maas M, Nelemans PJ, Valentini V, Das P, Rodel C, Kuo LJ, et al. Long-term outcome in patients with a pathological complete response after chemoradiation for rectal cancer: a pooled analysis of individual patient data. Lancet Oncol. 2010;11(9):835–44. doi:10.1016/S1470-2045(10)70172-8.
Martin ST, Heneghan HM, Winter DC. Systematic review and meta-analysis of outcomes following pathological complete response to neoadjuvant chemoradiotherapy for rectal cancer. Br J Surg. 2012;99(7):918–28. doi:10.1002/bjs.8702.
• Rodel C, Graeven U, Fietkau R, Hohenberger W, Hothorn T, Arnold D, et al. Oxaliplatin added to fluorouracil-based preoperative chemoradiotherapy and postoperative chemotherapy of locally advanced rectal cancer (the German CAO/ARO/AIO-04 study): final results of the multicentre, open-label, randomised, phase 3 trial. Lancet Oncol. 2015;16(8):979–89. doi:10.1016/S1470-2045(15)00159-X. The addition of oxaliplatin increased preoperative acute grade 3–4 toxicities but also increased 3 year DFS.
• Garcia-Aguilar J, Chow OS, Smith DD, Marcet JE, Cataldo PA, Varma MG, et al. Effect of adding mFOLFOX6 after neoadjuvant chemoradiation in locally advanced rectal cancer: a multicentre, phase 2 trial. Lancet Oncol. 2015;16(8):957–66. doi:10.1016/S1470-2045(15)00004-2. This study demonstrates increased pCR with intensification of neoadjuvant therapy at the expense of increased toxicities.
van Gijn W, Marijnen CA, Nagtegaal ID, Kranenbarg EM, Putter H, Wiggers T, et al. Preoperative radiotherapy combined with total mesorectal excision for resectable rectal cancer: 12-year follow-up of the multicentre, randomised controlled TME trial. Lancet Oncol. 2011;12(6):575–82. doi:10.1016/S1470-2045(11)70097-3.
Ellis CT, Samuel CA, Stitzenberg KB. National trends in nonoperative management of rectal adenocarcinoma. J Clin Oncol. 2016;34(14):1644–51. doi:10.1200/JCO.2015.64.2066.
Torok JA, Palta M, Willett CG, Czito BG. Nonoperative management of rectal cancer. Cancer. 2016;122(1):34–41. doi:10.1002/cncr.29735.
Habr-Gama A, Perez RO, Proscurshim I, Campos FG, Nadalin W, Kiss D, et al. Patterns of failure and survival for nonoperative treatment of stage c0 distal rectal cancer following neoadjuvant chemoradiation therapy. J Gastrointest Surg. 2006;10(10):1319–1328; discussion 28–9. doi:10.1016/j.gassur.2006.09.005.
•• Habr-Gama A, Perez RO, Nadalin W, Sabbaga J, Ribeiro U Jr, Silva e Sousa AH Jr, et al. Operative versus nonoperative treatment for stage 0 distal rectal cancer following chemoradiation therapy: long-term results. Ann Surg. 2004;240(4):711–7. discussion 7–8 This is the demonstration with long term follow up demonstrating excellent results without surgery in patients with a complete clinical response.
• Maas M, Beets-Tan RG, Lambregts DM, Lammering G, Nelemans PJ, Engelen SM, et al. Wait-and-see policy for clinical complete responders after chemoradiation for rectal cancer. J Clin Oncol. 2011;29(35):4633–40. doi:10.1200/JCO.2011.37.7176. A series from MSKCC also demonstrating the potential of non-operative management after a complete response to neoadjuvant therapy in locally advanced rectal cancer.
Smith JD, Ruby JA, Goodman KA, Saltz LB, Guillem JG, Weiser MR, et al. Nonoperative management of rectal cancer with complete clinical response after neoadjuvant therapy. Ann Surg. 2012;256(6):965–72. doi:10.1097/SLA.0b013e3182759f1c.
van’t Veer LJ, Dai H, van de Vijver MJ, He YD, Hart AA, Mao M, et al. Gene expression profiling predicts clinical outcome of breast cancer. Nature. 2002;415(6871):530–6. doi:10.1038/415530a.
Venook AP, Niedzwiecki D, Lopatin M, Ye X, Lee M, Friedman PN, et al. Biologic determinants of tumor recurrence in stage II colon cancer: validation study of the 12-gene recurrence score in cancer and leukemia group B (CALGB) 9581. J Clin Oncol. 2013;31(14):1775–81. doi:10.1200/JCO.2012.45.1096.
Kim IJ, Lim SB, Kang HC, Chang HJ, Ahn SA, Park HW, et al. Microarray gene expression profiling for predicting complete response to preoperative chemoradiotherapy in patients with advanced rectal cancer. Dis Colon Rectum. 2007;50(9):1342–53. doi:10.1007/s10350-007-277-7.
Brettingham-Moore KH, Duong CP, Greenawalt DM, Heriot AG, Ellul J, Dow CA, et al. Pretreatment transcriptional profiling for predicting response to neoadjuvant chemoradiotherapy in rectal adenocarcinoma. Clin Cancer Res. 2011;17(9):3039–47. doi:10.1158/1078-0432.CCR-10-2915.
• Ghadimi BM, Grade M, Difilippantonio MJ, Varma S, Simon R, Montagna C, et al. Effectiveness of gene expression profiling for response prediction of rectal adenocarcinomas to preoperative chemoradiotherapy. J Clin Oncol. 2005;23(9):1826–38. doi:10.1200/JCO.2005.00.406. This study identified a gene set from pretreatment biopsies collected in the German Rectal Cancer Trial.
Rimkus C, Friederichs J, Boulesteix AL, Theisen J, Mages J, Becker K, et al. Microarray-based prediction of tumor response to neoadjuvant radiochemotherapy of patients with locally advanced rectal cancer. Clin Gastroenterol Hepatol. 2008;6(1):53–61. doi:10.1016/j.cgh.2007.10.022.
Lopes-Ramos C, Koyama FC, Habr-Gama A, Salim AC, Bettoni F, Asprino PF, et al. Comprehensive evaluation of the effectiveness of gene expression signatures to predict complete response to neoadjuvant chemoradiotherapy and guide surgical intervention in rectal cancer. Cancer Genet. 2015;208(6):319–26. doi:10.1016/j.cancergen.2015.03.010.
•• Guinney J, Dienstmann R, Wang X, de Reynies A, Schlicker A, Soneson C, et al. The consensus molecular subtypes of colorectal cancer. Nat Med. 2015;21(11):1350–6. doi:10.1038/nm.3967. This is an excellent resource that for the first time pooled several large datasets to generate consensus molecular subtypes in colorectal cancer.
Nicholson BD, Shinkins B, Pathiraja I, Roberts NW, James TJ, Mallett S, et al. Blood CEA levels for detecting recurrent colorectal cancer. Cochrane Database Syst Rev. 2015;12:CD011134. doi:10.1002/14651858.CD011134.pub2.
Wallin U, Rothenberger D, Lowry A, Luepker R, Mellgren A. CEA—a predictor for pathologic complete response after neoadjuvant therapy for rectal cancer. Dis Colon Rectum. 2013;56(7):859–68. doi:10.1097/DCR.0b013e31828e5a72.
Das P, Skibber JM, Rodriguez-Bigas MA, Feig BW, Chang GJ, Wolff RA, et al. Predictors of tumor response and downstaging in patients who receive preoperative chemoradiation for rectal cancer. Cancer. 2007;109(9):1750–5. doi:10.1002/cncr.22625.
Zeng WG, Liang JW, Wang Z, Zhang XM, Hu JJ, Hou HR, et al. Clinical parameters predicting pathologic complete response following neoadjuvant chemoradiotherapy for rectal cancer. Chin J Cancer. 2015;34(10):468–74. doi:10.1186/s40880-015-0033-7.
Kleiman A, Al-Khamis A, Farsi A, Kezouh A, Vuong T, Gordon PH, et al. Normalization of CEA levels post-neoadjuvant therapy is a strong predictor of pathologic complete response in rectal cancer. J Gastrointest Surg. 2015;19(6):1106–12. doi:10.1007/s11605-015-2814-3.
Yang KL, Yang SH, Liang WY, Kuo YJ, Lin JK, Lin TC, et al. Carcinoembryonic antigen (CEA) level, CEA ratio, and treatment outcome of rectal cancer patients receiving pre-operative chemoradiation and surgery. Radiat Oncol. 2013;8:43. doi:10.1186/1748-717X-8-43.
Agostini M, Pucciarelli S, Enzo MV, Del Bianco P, Briarava M, Bedin C, et al. Circulating cell-free DNA: a promising marker of pathologic tumor response in rectal cancer patients receiving preoperative chemoradiotherapy. Ann Surg Oncol. 2011;18(9):2461–8. doi:10.1245/s10434-011-1638-y.
Pucciarelli S, Rampazzo E, Briarava M, Maretto I, Agostini M, Digito M, et al. Telomere-specific reverse transcriptase (hTERT) and cell-free RNA in plasma as predictors of pathologic tumor response in rectal cancer patients receiving neoadjuvant chemoradiotherapy. Ann Surg Oncol. 2012;19(9):3089–96. doi:10.1245/s10434-012-2272-z.
•• Galon J, Costes A, Sanchez-Cabo F, Kirilovsky A, Mlecnik B, Lagorce-Pages C, et al. Type, density, and location of immune cells within human colorectal tumors predict clinical outcome. Science. 2006;313(5795):1960–4. doi:10.1126/science.1129139. This is the first publication demonstrating the potential of using characteristics of the immune microenvironment to predict outcomes.
Mlecnik B, Tosolini M, Kirilovsky A, Berger A, Bindea G, Meatchi T, et al. Histopathologic-based prognostic factors of colorectal cancers are associated with the state of the local immune reaction. J Clin Oncol. 2011;29(6):610–8. doi:10.1200/JCO.2010.30.5425.
Pages F, Kirilovsky A, Mlecnik B, Asslaber M, Tosolini M, Bindea G, et al. In situ cytotoxic and memory T cells predict outcome in patients with early-stage colorectal cancer. J Clin Oncol. 2009;27(35):5944–51. doi:10.1200/JCO.2008.19.6147.
•• Anitei MG, Zeitoun G, Mlecnik B, Marliot F, Haicheur N, Todosi AM, et al. Prognostic and predictive values of the immunoscore in patients with rectal cancer. Clin Cancer Res. 2014;20(7):1891–9. doi:10.1158/1078-0432.CCR-13-2830. The authors demonstrate that the pretreatment density of T cell infiltrate is predictive of response to neoadjuvant chemoradiation.
Lote H, Cafferkey C, Chau I. PD-1 and PD-L1 blockade in gastrointestinal malignancies. Cancer Treat Rev. 2015;41(10):893–903. doi:10.1016/j.ctrv.2015.09.004.
Saigusa S, Toiyama Y, Tanaka K, Inoue Y, Mori K, Ide S, et al. Implication of programmed cell death ligand 1 expression in tumor recurrence and prognosis in rectal cancer with neoadjuvant chemoradiotherapy. Int J Clin Oncol. 2016;21(5):946–52. doi:10.1007/s10147-016-0962-4.
Shinto E, Hase K, Hashiguchi Y, Sekizawa A, Ueno H, Shikina A, et al. CD8+ and FOXP3+ tumor-infiltrating T cells before and after chemoradiotherapy for rectal cancer. Ann Surg Oncol. 2014;21(Suppl 3):S414–21. doi:10.1245/s10434-014-3584-y.
McCoy MJ, Hemmings C, Anyaegbu CC, Austin SJ, Lee-Pullen TF, Miller TJ, et al. Tumour-infiltrating regulatory T cell density before neoadjuvant chemoradiotherapy for rectal cancer does not predict treatment response. Oncotarget. 2017; doi:10.18632/oncotarget.15048.
McCoy MJ, Hemmings C, Miller TJ, Austin SJ, Bulsara MK, Zeps N, et al. Low stromal Foxp3+ regulatory T-cell density is associated with complete response to neoadjuvant chemoradiotherapy in rectal cancer. Br J Cancer. 2015;113(12):1677–86. doi:10.1038/bjc.2015.427.
Walsh SR, Cook EJ, Goulder F, Justin TA, Keeling NJ. Neutrophil-lymphocyte ratio as a prognostic factor in colorectal cancer. J Surg Oncol. 2005;91(3):181–4. doi:10.1002/jso.20329.
Kim IY, You SH, Kim YW. Neutrophil-lymphocyte ratio predicts pathologic tumor response and survival after preoperative chemoradiation for rectal cancer. BMC Surg. 2014;14:94. doi:10.1186/1471-2482-14-94.
Dou X, Wang RB, Yan HJ, Jiang SM, Meng XJ, Zhu KL, et al. Circulating lymphocytes as predictors of sensitivity to preoperative chemoradiotherapy in rectal cancer cases. Asian Pac J Cancer Prev. 2013;14(6):3881–5.
Hasegawa S, Eguchi H, Tomokuni A, Tomimaru Y, Asaoka T, Wada H, et al. Pre-treatment neutrophil to lymphocyte ratio as a predictive marker for pathological response to preoperative chemoradiotherapy in pancreatic cancer. Oncol Lett. 2016;11(2):1560–6. doi:10.3892/ol.2015.4057.
Choi CH, Kim WD, Lee SJ, Park WY. Clinical predictive factors of pathologic tumor response after preoperative chemoradiotherapy in rectal cancer. Radiat Oncol J. 2012;30(3):99–107. doi:10.3857/roj.2012.30.3.99.
Lino-Silva LS, Salcedo-Hernandez RA, Ruiz-Garcia EB, Garcia-Perez L, Herrera-Gomez A. Pre-operative neutrophils/lymphocyte ratio in rectal cancer patients with preoperative chemoradiotherapy. Med Arch. 2016;70(4):256–60. doi:10.5455/medarh.2016.70.256-260.
Shen J, Zhu Y, Wu W, Zhang L, Ju H, Fan Y, et al. Prognostic role of neutrophil-to-lymphocyte ratio in locally advanced rectal cancer treated with neoadjuvant chemoradiotherapy. Med Sci Monit. 2017;23:315–24.
Group MS. Diagnostic accuracy of preoperative magnetic resonance imaging in predicting curative resection of rectal cancer: prospective observational study. BMJ. 2006;333(7572):779. doi:10.1136/bmj.38937.646400.55.
•• Taylor FG, Quirke P, Heald RJ, Moran BJ, Blomqvist L, Swift IR, et al. Preoperative magnetic resonance imaging assessment of circumferential resection margin predicts disease-free survival and local recurrence: 5-year follow-up results of the MERCURY study. J Clin Oncol. 2014;32(1):34–43. doi:10.1200/JCO.2012.45.3258. This is a well-designed clinical trial designed to assess accuracy of MRI in predicting the pathologic surgical margin.
Lambrecht M, Vandecaveye V, De Keyzer F, Roels S, Penninckx F, Van Cutsem E, et al. Value of diffusion-weighted magnetic resonance imaging for prediction and early assessment of response to neoadjuvant radiochemotherapy in rectal cancer: preliminary results. Int J Radiat Oncol Biol Phys. 2012;82(2):863–70. doi:10.1016/j.ijrobp.2010.12.063.
Genovesi D, Filippone A, Ausili Cefaro G, Trignani M, Vinciguerra A, Augurio A, et al. Diffusion-weighted magnetic resonance for prediction of response after neoadjuvant chemoradiation therapy for locally advanced rectal cancer: preliminary results of a monoinstitutional prospective study. Eur J Surg Oncol. 2013;39(10):1071–8. doi:10.1016/j.ejso.2013.07.090.
Sun YS, Zhang XP, Tang L, Ji JF, Gu J, Cai Y, et al. Locally advanced rectal carcinoma treated with preoperative chemotherapy and radiation therapy: preliminary analysis of diffusion-weighted MR imaging for early detection of tumor histopathologic downstaging. Radiology. 2010;254(1):170–8. doi:10.1148/radiol.2541082230.
Tong T, Sun Y, Gollub MJ, Peng W, Cai S, Zhang Z, et al. Dynamic contrast-enhanced MRI: use in predicting pathological complete response to neoadjuvant chemoradiation in locally advanced rectal cancer. J Magn Reson Imaging. 2015;42(3):673–80. doi:10.1002/jmri.24835.
George ML, Dzik-Jurasz AS, Padhani AR, Brown G, Tait DM, Eccles SA, et al. Non-invasive methods of assessing angiogenesis and their value in predicting response to treatment in colorectal cancer. Br J Surg. 2001;88(12):1628–36. doi:10.1046/j.0007-1323.2001.01947.x.
Intven M, Reerink O, Philippens ME. Dynamic contrast enhanced MR imaging for rectal cancer response assessment after neo-adjuvant chemoradiation. J Magn Reson Imaging. 2015;41(6):1646–53. doi:10.1002/jmri.24718.
Martoni AA, Di Fabio F, Pinto C, Castellucci P, Pini S, Ceccarelli C, et al. Prospective study on the FDG-PET/CT predictive and prognostic values in patients treated with neoadjuvant chemoradiation therapy and radical surgery for locally advanced rectal cancer. Ann Oncol. 2011;22(3):650–6. doi:10.1093/annonc/mdq433.
Huh JW, Min JJ, Lee JH, Kim HR, Kim YJ. The predictive role of sequential FDG-PET/CT in response of locally advanced rectal cancer to neoadjuvant chemoradiation. Am J Clin Oncol. 2012;35(4):340–4. doi:10.1097/COC.0b013e3182118e7d.
Niccoli-Asabella A, Altini C, De Luca R, Fanelli M, Rubini D, Caliandro C, et al. Prospective analysis of 18F-FDG PET/CT predictive value in patients with low rectal cancer treated with neoadjuvant chemoradiotherapy and conservative surgery. Biomed Res Int. 2014;2014:952843. doi:10.1155/2014/952843.
Capirci C, Rubello D, Pasini F, Galeotti F, Bianchini E, Del Favero G, et al. The role of dual-time combined 18-fluorodeoxyglucose positron emission tomography and computed tomography in the staging and restaging workup of locally advanced rectal cancer, treated with preoperative chemoradiation therapy and radical surgery. Int J Radiat Oncol Biol Phys. 2009;74(5):1461–9. doi:10.1016/j.ijrobp.2008.10.064.
• Dunne PD, McArt DG, Bradley CA, O'Reilly PG, Barrett HL, Cummins R, et al. Challenging the cancer molecular stratification dogma: intratumoral heterogeneity undermines consensus molecular subtypes and potential diagnostic value in colorectal cancer. Clin Cancer Res. 2016;22(16):4095–104. doi:10.1158/1078-0432.CCR-16-0032. This work highlights the caveats of using the CMS subtypes and potential source of variability as a result of intratumor heterogeneity.
Le DT, Uram JN, Wang H, Bartlett BR, Kemberling H, Eyring AD, et al. PD-1 blockade in tumors with mismatch-repair deficiency. N Engl J Med. 2015;372(26):2509–20. doi:10.1056/NEJMoa1500596.
Kalady MF, de Campos-Lobato LF, Stocchi L, Geisler DP, Dietz D, Lavery IC, et al. Predictive factors of pathologic complete response after neoadjuvant chemoradiation for rectal cancer. Ann Surg. 2009;250(4):582–9. doi:10.1097/SLA.0b013e3181b91e63.
Wolthuis AM, Penninckx F, Haustermans K, De Hertogh G, Fieuws S, Van Cutsem E, et al. Impact of interval between neoadjuvant chemoradiotherapy and TME for locally advanced rectal cancer on pathologic response and oncologic outcome. Ann Surg Oncol. 2012;19(9):2833–41. doi:10.1245/s10434-012-2327-1.
Sanghera P, Wong DW, McConkey CC, Geh JI, Hartley A. Chemoradiotherapy for rectal cancer: an updated analysis of factors affecting pathological response. Clin Oncol (R Coll Radiol). 2008;20(2):176–83. doi:10.1016/j.clon.2007.11.013.
Appelt AL, Ploen J, Vogelius IR, Bentzen SM, Jakobsen A. Radiation dose-response model for locally advanced rectal cancer after preoperative chemoradiation therapy. Int J Radiat Oncol Biol Phys. 2013;85(1):74–80. doi:10.1016/j.ijrobp.2012.05.017.
Author information
Authors and Affiliations
Corresponding author
Ethics declarations
Conflict of Interest
The authors declare they have no conflict(s) of interest.
Human and Animal Rights and Informed Consent
This article does not contain any studies with human or animal subjects performed by any of the authors.
Additional information
Topical Collection on Radiation Therapy and Radiation Therapy Innovations in Colorectal Cancer
Rights and permissions
About this article
Cite this article
Oh, P., Du, K.L. Biomarkers that Predict Response to Neoadjuvant Chemoradiation in Locally Advanced Rectal Cancer. Curr Colorectal Cancer Rep 13, 276–283 (2017). https://doi.org/10.1007/s11888-017-0376-3
Published:
Issue Date:
DOI: https://doi.org/10.1007/s11888-017-0376-3