Abstract
Everolimus (RAD001, Afinitor®) is an oral, selective mTOR inhibitor recently approved by the US-FDA in combination with exemestane for treatment of hormone receptor positive advanced breast cancer. To date, no molecular predictors of response to everolimus in breast cancer have been identified. We hypothesized predictive markers could be identified using preclinical models. Using a molecularly characterized panel of human breast cancer and immortalized breast epithelial cell lines, we determined sensitivity to everolimus alone or in combination with ER− or HER2− targeted therapy. Gene expression microarrays and comparative genomic hybridization were performed on the cell lines to identify predictors of response to everolimus. Among 13 everolimus-sensitive cell lines, 10/13(77 %) were luminal, while in 26 resistant cell lines, 16/26(62 %) were non-luminal, and 10/26(38 %) were luminal. Only 3/24 non-luminal lines were sensitive, two of which were HER2+. Everolimus enhanced the anti-proliferative effect of both tamoxifen (TAM) and fulvestrant (FUL) in ER+ breast cancer cell lines, as well as trastuzumab in HER2+ cell lines. Everolimus + FUL but not everolimus + TAM reversed acquired resistance to TAM. Everolimus inhibited mTOR in tested cell lines by decreasing S6 phosphorylation, mediating its anti-proliferative effect by G0/G1 cell cycle arrest and induction of apoptosis. Chromosomal amplifications of AURKA (p value = 0.04) and HER2 (p value = 0.03) were each associated with increased sensitivity to everolimus. Transcript expression microarrays identified GSK3A, PIK3R3, KLF8, and MAPK10 among the genes overexpressed in sensitive luminal lines, while PGP, RPL38, GPT, and GFAP were among the genes overexpressed in resistant luminal cell lines. These preclinical in vitro data provide further support for continued clinical development of everolimus in luminal (ER+ or HER2+) breast cancer in combination with targeted therapies. We identified several potential molecular markers associated with response to everolimus that will require validation in clinical material.
Similar content being viewed by others
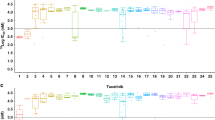
Avoid common mistakes on your manuscript.
Introduction
Molecular profiling of human breast cancers has revealed distinct subtypes that are characterized and often driven by altered signaling pathways [1, 2]. One such signaling pathway that has been shown to be altered in many human cancers is the phosphatidylinositol 3-kinase (PI3K)/AKT/mammalian target of rapamycin (mTOR) pathway. This pathway has been shown to play a critical role in cell proliferation, migration, differentiation, and survival [3]. The mTOR protein is a 289-kD intracellular serine/threonine kinase with activity important in regulating the PI3K pathway through modulation and integration of signals from the intracellular and extracellular environments [4]. mTOR is a component of two multiprotein complexes: mTORC1 and mTORC2 [5]. Activation of mTORC1 leads to production of proteins via phosphorylation of eukaryotic initiation factor 4E binding protein 1 (4E-BP1) and p70 ribosomal S6 kinase 1 (S6K1) [4]. The activation and downstream effects of mTORC2 are less well defined, but evidence suggests a role in cell survival, proliferation, metabolism, and cytoskeletal organization [4, 6–9].
While the PI3K/AKT/mTOR pathway appears to be critically important in numerous normal cell processes, its dysregulation has been demonstrated to be a significant factor in the pathogenesis of multiple cancers [3, 10, 11]. Breast cancers often bear molecular alterations that activate the PI3K/AKT/mTOR pathway such as overexpression of tyrosine kinases, downregulation of the tumor suppressor PTEN [12–15], activation of PI3K [16–18], or overexpression/hyperactivation of AKT [19]. Given the fundamental role of PI3K/AKT/mTOR signaling in cancer pathophysiology, therapeutic agents have been developed to target various components of the pathway. One such agent is everolimus (RAD001, Afinitor®, Novartis, Basel, Switzerland), an orally administered derivative of rapamycin that is a selective allosteric mTORC1 inhibitor [20–23]. Everolimus forms a complex with the intracellular receptor FKBP12 [24, 25] and may indirectly inhibit mTORC2 by sequestering free mTOR so that the assembly of mTORC2 complexes is precluded [8].
Everolimus has received approval by the US Food and Drug Administration (FDA) for treatment of hormone receptor-positive advanced breast cancer in combination with exemestane in post-menopausal patients with non-steroidal aromatase inhibitor-refractory disease, based on the results of a phase III randomized study [26]. Studies evaluating the use of everolimus in combination with HER2-targeted agents have also reported promising initial results [27–31].
To date, no consistent molecular determinants of responsiveness to everolimus have been defined, nor have studies clearly delineated subsets of breast cancer most likely to be sensitive to mTOR inhibition. Some data suggest that activation status of the PI3K/AKT/mTOR pathway may be an indicator of mTOR sensitivity [20, 32–34], while other data show no such association [35]. Large panels of molecularly characterized breast cancer cell lines are valuable tools for better defining molecular subtypes and screening biologically targeted therapies [36–38]. To assess the activity of everolimus in different molecular subtypes of breast cancer and to better understand molecular determinants of response to mTOR inhibition, we assessed the in vitro effects of everolimus as a single agent across a panel of 49 human breast cancer and 3 immortalized breast epithelial cell lines. In addition, we assessed the activity of everolimus in combination with tamoxifen, fulvestrant, and trastuzumab in selected estrogen receptor positive (ER+) and HER2 amplified cell lines, respectively.
Materials and methods
Cell lines, cell culture, and reagents
Our molecularly characterized cell line panel included 49 breast cancer cell lines and 3 immortalized breast epithelial cell lines representing the known molecular subgroups of breast cancer as previously described [38, 39]. Cell lines conditioned for acquired everolimus resistance were established in our laboratories as described previously [38, 39]. Everolimus (Afinitor®) was obtained from Novartis (Basel, Switzerland) and dissolved in DMSO (20 µM).
Point mutation data from the cancer cell line encyclopedia
The cancer cell line encyclopedia (CCLE) project has sequenced 4059 protein-coding genes via solution phase hybrid capture and massively parallel sequencing. These point mutation data were downloaded from the Broad Institute’s publicly available database (http://www.broadinstitute.org/ccle/home) and incorporated into the UCLA Translational Oncology Research Laboratory (TORL)’s database for those cell lines where the two panels overlap. Data were available on 38 of the cell lines in UCLA-TORL’s everolimus response panel. The CCLE has pre-restricted its whole-exome sequencing dataset for several inclusion criteria with the intention of enriching for oncogenic “driver” alterations [40].
Detailed information on cell lines, proliferation assays, comparative genomic hybridization, microarray analysis, flow cytometry analysis of cell cycle and apoptosis, western blot, and statistical analysis have been previously described [37, 38, 41] and can be found in online resource Supplementary Materials and Methods.
Results
Response to everolimus and molecular subtypes of breast cancer
A panel of 49 human breast cancer cell lines and 3 immortalized breast epithelial cell lines were used to test the anti-proliferative effects of everolimus. We observed relatively flat dose response curves over a wide range of concentrations in a majority of cell lines. For this reason, IC50 alone was deemed to be inadequate to classify response. Instead, a non-standard two-criterion cutoff was used to determine sensitivity such that those cell lines with an IC50 below 1 nM and those with more than 80 % growth inhibition at 100 nM were classified as sensitive. Those cell lines with an IC50 over 50 nM were classified as resistant. The rest were classified as intermediately sensitive. Based on these response criteria, 13 cell lines were classified as sensitive, 13 as intermediate, and 26 as resistant to everolimus (Table 1).
Four HER2-amplified lines that were conditioned for acquired resistance to trastuzumab or lapatinib were included in the panel (Table 1). While the BT-474 parental line was everolimus sensitive, the two resistant lines showed intermediate sensitivity. The SK-BR-3 parental line and SK-BR-3-LR were resistant to everolimus, and the SK-BR-3-TR line had intermediate sensitivity.
A total of 13 cell lines were determined to be sensitive to everolimus, and the majority (10/13; 77 %) represented the luminal subtype. Of these, 10/13 (77 %) were ER+ (5 HER2+, 5 HER2−), and 3 were basal/post-EMT (2 ER−/HER2+, 1 ER−/HER2−). While the sensitive lines were enriched for ER expression, not all of the ER+ responded to everolimus with 8/21 (38 %) ER+ classified as resistant (Table 1). The remainder of the lines in the panel was determined to have intermediate sensitivity. Similar response distributions were observed for the cell lines constituting the HER2+ subtype, i.e., 7/21 (33 %) sensitive versus 6/21 (29 %) resistant.
Of 26 resistant cell lines, 16 (62 %) were non-luminal (either basal or post-EMT) or immortalized (ER− and HER2−). In contrast only 3/24 (13 %) non-luminal (basal/post-EMT) lines were sensitive to everolimus, two of which were HER2+. All three immortalized cell lines were classified as resistant.
These findings suggest that, despite enrichment for ER and HER2 in sensitive cell lines and loss of these markers in resistant cell lines, these subtype classifications alone do not fully explain the differences in response to everolimus, and a search for other molecular predictors of response is warranted.
Everolimus enhances the anti-proliferative effect of tamoxifen and fulvestrant in ER-positive breast cancer cell lines
The combinations of everolimus with the estrogen receptor antagonists, TAM and FUL, were evaluated on three ER + luminal breast cancer cell lines with variable everolimus single-agent activity (sensitive; MDA-MB-415 and CAMA-1, and resistant; MCF-7). We observed an enhancement of the anti-proliferative effect of TAM and FUL with everolimus in all three tested cell lines (Supplementary Fig. S1).
Everolimus in combination with hormone pathway and HER2-targeting agents
Combinations of everolimus with TAM or FUL were evaluated in MCF-7 cells conditioned for acquired resistance to tamoxifen by a long-term estrogen deprivation (MCF7-TAMR). The anti-proliferative effect of the everolimus + tamoxifen combination in tamoxifen-resistant MCF7 cells was similar to the effect of single-agent tamoxifen (Supplementary Fig. S2). Conversely, everolimus potentiated the anti-proliferative effect of fulvestrant in this cell line suggesting that everolimus + fulvestrant may be a combination that reverses acquired resistance to tamoxifen.
The combination of everolimus with trastuzumab was evaluated in two HER2+ breast cancer cell lines: one everolimus sensitive (BT-474) and one everolimus resistant (SK-BR-3). In both cell lines, growth inhibition was enhanced with the combination of everolimus and trastuzumab compared to either agent used alone (Supplementary Fig. S3).
Biochemical effects of everolimus on signal transduction
To evaluate differential effects of everolimus on S6 and AKT activation, western blot analyses were performed in a subset of cell lines with variable sensitivities to everolimus. Cells were treated with 100 nM everolimus and harvested at 6 time points between 10 min and 48 h as described in Supplementary Materials and Methods.
We observed a significant inhibition of S6 phosphorylation in all tested cell lines after 30 min exposure to everolimus regardless of sensitivity to growth inhibitory effects (Fig. 1). This was accompanied by a less pronounced decrease in total S6 in sensitive cell lines. Feedback activation of AKT phosphorylation was observed in RAD001 sensitive (BT-474), intermediate (ZR-751), and resistant (KPL-1) cell lines. The effect of everolimus on total AKT was variable among the tested cell lines (Fig. 1).
The effects of everolimus on total and phosphorylated S6 and AKT. The effects of everolimus were measured in a subset of cell lines with a variable sensitivity to everolimus by western blot as described in Supplementary Materials and Methods. All cell lines were treated with 100 nM everolimus for 10 min–48 h. a Sensitive cell lines BT-474 and HCC-1419, b intermediate ZR-75-1, c resistant MDA-MB-231 and KPL-1. There was a significant inhibition of S6 phosphorylation in all tested cell lines and a less pronounced decrease in total S6 in sensitive cell lines. There was an increase in AKT phosphorylation in sensitive BT-474, intermediate ZR-75-1 (peak at 8 h), and resistant KPL-1. The effect of everolimus on total AKT was variable
In summary, we confirmed that everolimus inhibits mTOR in all tested cell lines by decreasing the phosphorylation of its downstream target S6. In addition, we observed a decrease in the total S6 protein in sensitive cell lines. AKT phosphorylation increased most of the cell lines tested irrespective of sensitivity.
Effects of everolimus on cell cycle and apoptosis
The effects of everolimus on the cell cycle were analyzed in a subset of sensitive, intermediate, and resistant cell lines. Cells were exposed to everolimus at 10 nM for 5 days, and then flow cytometry was performed using Nim-DAPI staining. G0/G1 cell cycle arrest was observed in the two sensitive and the one intermediate cell line tested but was not observed in resistant lines (Fig. 2).
Effects of everolimus on cell cycle. Cell lines with variable sensitivity to everolimus were treated with 10 nM everolimus for 5 days. a Sensitive cell lines show a G0/G1 arrest. b In intermediate cell lines, everolimus caused variable effects on the cell cycle. c No effect on the cell cycle was observed in resistant cell lines. The average growth inhibitory effect of 10 nM everolimus for each cell line is shown in parentheses. Solid bars- control samples; striped bars- treated samples. Error bars represent standard error for two separate experiments. *Indicates p < 0.05 compared to control
Similarly, the effect of everolimus on apoptosis was determined in the same subset of cell lines. For this assay, cells were exposed to 100 nM of everolimus for 5 days and then analyzed with a dual stain flow cytometry protocol using Annexin V-FITC and propidium iodide. A significant increase in Annexin-V positive cells was seen in sensitive cell lines (Fig. 3). Together, these data suggest that the anti-proliferative effect of everolimus is mediated by both inhibition of the cell cycle and the induction of apoptosis.
Effects of everolimus on apoptosis. Cell lines with variable sensitivity to everolimus were treated with 100 nM everolimus for 5 days a Sensitive cell lines show an increase in Annexin-V positive cells as compared with no increase in b intermediate or c resistant cell lines. The average growth inhibitory effect (INH) of 100 nM everolimus for each cell line is shown in parentheses. Solid bars- control samples; striped bars- treated samples. Error bars represent standard error for two separate experiments. *Indicates p < 0.05 compared to control
Genotype-response association screening
After determining response to everolimus, we interrogated two large genomic datasets for candidate sensitivity or resistance biomarkers including point mutations from the CCLE database and copy number alterations (CNAs) from our own CGH arrays. Due to the large number of genetic alterations in these datasets relative to the number of cell lines in our study, false positives were a major concern. To minimize this problem, we used the publicly available gene ontology (GO) database to restrict our candidate biomarker set to those alterations most likely to be involved in PI3K/AKT/mTOR signaling (GO IDs: GO:0048015, GO:0014068, GO:0043552, GO:0014065). This gene list was cross referenced against the COSMIC gene census to further limit the list to genes where oncogenic driver mutations have been described. Our final candidate biomarker set consisted of 23 genes where either point mutations or CNAs were identified in our panel. Alterations in each of these genes were tested for an association with everolimus sensitivity or resistance (Table 2) .
Only two genotype-response associations were found to be statistically significant at α = 0.05. Chromosomal amplifications of AURKA (p value = 0.04) and HER2 (p value = 0.03) were each associated with increased in vitro sensitivity to everolimus. Of note, mutation of neither PIK3CA nor PTEN was significantly associated with response to everolimus.
Identification of differentially expressed genes and sensitivity to everolimus
Gene expression profiles were next used to identify genes associated with response to everolimus. First, we compared sensitive cell lines (n = 13) and resistant cell lines (n = 25) (acquired resistance cell lines were excluded). This approach identified 232 differentially expressed probes (p ≤ 0.01), where 137 genes demonstrated increased expression in sensitive cell lines and 79 genes were increased in de novo resistant lines (Supplementary Table S1). Hierarchical clustering of the 38 cell lines across the 232 probes was performed. Two major branches were identified: one branch included 19 luminal lines and one HER2+ non-luminal cell line, and the other branch included all of the non-luminal lines (n = 18) (Supplementary Fig. S4). The 10 everolimus-sensitive luminal cell lines clustered together within the luminal branch.
We next compared only luminal breast cell lines classified as sensitive (n = 10) or resistant (n = 9) to everolimus. A total of 124 probes were differentially expressed (p ≤ 0.01), where 72 genes demonstrated increased expression in sensitive lines, and 48 genes were increased in resistant lines (Supplementary Table S2). Two major branches were identified corresponding to everolimus sensitivity (Supplementary Fig. S5). Genes having a higher average expression level in luminal cell lines sensitive to everolimus included GSK3A, PIK3R3, KLF8, and MAPK10, whereas PGP, RPL38, GPT, and GFAP were found to be higher in resistant luminal cell lines.
Discussion
Dysregulation of the PI3K/AKT/mTOR pathway has been shown to play a role in breast cancer growth, progression, and treatment resistance, making it an attractive target for novel therapeutic agents. Using a large panel of well-characterized human breast cancer cell lines representing the known molecular subtypes of breast cancer, we identified significant enrichment of ER+ and HER2+ cell lines among those classified as sensitive to everolimus. Biochemical studies did not reveal any correlation with the ability of everolimus to block downstream mTOR signaling with growth inhibition given that everolimus blocked S6 activation in both sensitive and resistant cell lines. However, the drug’s ability to induce G0/G1 arrest and apoptosis was only seen in the cell lines classified as sensitive. These observations are consistent with other studies [23]. Interestingly, we also demonstrated that everolimus induces feedback activation of AKT which was a phenomenon thought to be important in escaping the drug’s effect; however, it was observed in both sensitive and resistant cell lines in the current study.
Additionally, combination therapy data indicate that everolimus enhances the anti-proliferative effect of both tamoxifen and fulvestrant in ER+ luminal cell lines. The combination of everolimus plus fulvestrant was active in MCF-7 tamoxifen-resistant cells that had been generated by long-term estrogen deprivation. These findings as well as those from other preclinical studies [42, 43] provide evidence that everolimus may reverse endocrine resistance. Two relevant randomized clinical trials have now been reported in which post-menopausal women with ER+, aromatase inhibitor-resistant advanced breast cancer were randomly assigned to receive an endocrine agent plus placebo or everolimus. In both studies, patient outcomes (clinical benefit rate, time to progression, and progression-free survival) were significantly better when everolimus was added to either tamoxifen [44] or exemestane [26]. While our in vitro experiments did not combine everolimus with an aromatase inhibitor, the data generated add to the growing preclinical and clinical literature that shows inhibiting mTOR may restore sensitivity to hormonal manipulation in the setting of endocrine resistance.
Our in vitro analyses also indicate that HER2-amplified breast cancer cell lines are sensitive to everolimus. These data support the findings from our group [45] and others showing preclinical activity of everolimus and everolimus + trastuzumab in HER2+ breast cancer cells. The clinical activity of everolimus, trastuzumab, and taxane chemotherapy in treatment naïve HER2+ metastatic breast cancer is currently being evaluated in an ongoing randomized phase III study (BOLERO-1, NCT00876395). In addition, we have observed robust preclinical activity of everolimus in HER2+ breast cancer with de novo resistance to trastuzumab [45]. These preclinical data have been validated in several phase I and II clinical trials demonstrating that addition of everolimus to trastuzumab may reverse trastuzumab resistance in some patients [27–29]. Recently, a phase III randomized trial (BOLERO-3) evaluating trastuzumab and vinorelbine plus everolimus or placebo in trastuzumab-pretreated advanced HER2+ disease reported a statistically significant improvement in investigator assessed PFS for everolimus-treated patients [31].
Cell lines classified as non-luminal (basal or post-EMT), or immortalized (ER− and HER2−) were much more likely to be resistant to everolimus. Only one triple-negative cell line was classified as sensitive in our study. These findings are in contrast to a recently reported preclinical evaluation of everolimus in 9 triple-negative breast cancer (TNBC) cell lines [46]. There are several important differences in both experimental design and analytical methodology that may explain this discrepancy. The proliferation assays performed differed in duration of treatment (5 days vs. 4 days), method of cell quantification (cell counting vs. CCK-8 colorimetric assay), and method of calculation of cell growth inhibition. In addition, everolimus is an allosteric inhibitor and has a flat dose–response curve. This observation makes the use of IC50 alone a difficult measure by which to stratify sensitivity/resistance outcomes. Given these concerns, we chose to use a multi-factorial cutoff for sensitivity in our analyses.
Through an unbiased analysis on a large panel of cell lines, we determined that only 38 % of ER+ and 33 % of HER2+ breast cancer cell lines were classified as sensitive to everolimus. These data recapitulate the clinical observations with everolimus [26–29] suggesting that the commonly used subtypes of breast cancer alone do not fully explain the differential response to everolimus and that additional other predictive biomarkers may exist.
In order to identify other genomic biomarkers that may predict differential sensitivity, we used several large genomics datasets with a particular focus on genes involved in PI3K/AKT/mTOR signaling and its regulation. In this analysis, chromosomal amplifications of HER2 and AURKA were each associated with increased sensitivity to everolimus in vitro.
AURKA encodes for the serine/threonine kinase Aurora A and is amplified and overexpressed in multiple malignancies, including breast cancers. It regulates centrosome maturation and mitotic spindle assembly during mitotic progression [47, 48]. Overexpression of Aurora A results in the activation of AKT [49, 50] and cooperates with PI3K/AKT/mTOR pathway in oncogenic transformation [51]. Our findings indicating an association of Aurora A amplification with everolimus sensitivity suggests that activation of PI3K/AKT/mTOR pathway by Aurora A, possibly via activation of AKT, may sensitize breast cancer cells to everolimus. Whether AURKA amplification is a biomarker for everolimus sensitivity will need to be further explored in mechanistic studies and confirmed in clinical settings.
Microarray profiles were used to identify genes whose expression pattern is associated with response to everolimus. When we compared sensitive luminal breast cell lines to resistant luminal cell lines and performed hierarchical clustering, two major branches were identified corresponding to everolimus sensitivity. Genes that had higher average expression in luminal cell lines sensitive to everolimus included GSK3A, PIK3R3, KLF8, and MAPK10, whereas PGP, RPL38, GPT, and GFAP were higher in resistant luminal cell lines. GSK3A, PIK3R3, and KLF8 are known to interact with PI3K/AKT/mTOR signaling, and PGP is reported to be downregulated by PI3K/AKT inhibition in lymphoma cell lines [52–54]. We did not detect any significant difference in gene expression of LKB11 (STK11) or 4EBP1 (EIF4EBP1) in sensitive versus resistant lines. These data require further exploration and validation and currently serve as hypothesis-generating observations.
To date, exploratory analyses from clinical trials evaluating everolimus have failed to consistently identify any predictive biomarkers of response including activation of the PI3K pathway (via mutations or changes in expression of protein components). Similar to other studies [33, 55], we observed no association between PTEN mutation and response to everolimus in vitro. In contrast, an exploratory subset analysis of BOLERO-3 suggests that low PTEN expression may predict greater benefit from everolimus in HER2+ metastatic breast cancers [56]. We also do not observe any association between PIK3CA mutations and response to everolimus in the current study. This is consistent with findings from a subset analysis of the BOLERO-2 trial in which gene alterations in the PI3K pathway did not correlate with response to everolimus [35]. Conversely, the BOLERO-2 analysis did show that patients with tumors that were either wild type or bearing only one alteration among the PIK3CA, PTEN, CCND1, and FGRF1/2 genes had a better PFS compared to those with multiple alterations [35].
Data from the current broad analysis of everolimus response in a large breast cancer cell line panel continue to support further development of everolimus in luminal ER+ and HER2+ breast cancer. These analyses have identified several candidate biomarkers that may predict response to everolimus; however, these markers will require further validation in ongoing clinical trials of the drug in this disease.
Abbreviations
- ANOVA:
-
Analysis of variance
- EMT:
-
Epithelial-to-mesenchymal transition
- ER:
-
Estrogen receptor
- FITC:
-
Fluorescein isothiocyanate
- FUL:
-
Fulvestrant
- IC50 :
-
Drug concentration that provides 50 % growth inhibition
- mTOR:
-
Mammalian target of rapamycin
- HER2:
-
Human epidermal growth factor receptor 2
- Nim-DAPI:
-
Nuclear isolation medium–4,6-diamidino-2-phenylindole dihydrochloride
- OS:
-
Overall survival
- PI3K:
-
Phosphoinositide 3-kinase
- PFS:
-
Progression-free survival
- PTEN:
-
Phosphatase and tensin homolog
- RR:
-
Relative risk
- TAM:
-
Tamoxifen
- TNBC:
-
Triple-negative breast cancer
- TTP:
-
Time to progression
References
Perou CM, Sorlie T, Eisen MB, van de Rijn M, Jeffrey SS, Rees CA, Pollack JR, Ross DT, Johnsen H, Akslen LA, Fluge O, Pergamenschikov A, Williams C, Zhu SX, Lonning PE, Borresen-Dale AL, Brown PO, Botstein D (2000) Molecular portraits of human breast tumours. Nature 406(6797):747–752
Sorlie T, Perou CM, Tibshirani R, Aas T, Geisler S, Johnsen H, Hastie T, Eisen MB, van de Rijn M, Jeffrey SS, Thorsen T, Quist H, Matese JC, Brown PO, Botstein D, Lonning PE, Borresen-Dale AL (2001) Gene expression patterns of breast carcinomas distinguish tumor subclasses with clinical implications. Proc Natl Acad Sci USA 98(19):10869–10874. doi:10.1073/pnas.191367098
Vivanco I, Sawyers CL (2002) The phosphatidylinositol 3-Kinase AKT pathway in human cancer. Nat Rev Cancer 2(7):489–501. doi:10.1038/nrc839
Laplante M, Sabatini DM (2009) mTOR signaling at a glance. J Cell Sci 122(20):3589–3594. doi:10.1242/jcs.051011
Sabatini DM (2006) mTOR and cancer: insights into a complex relationship. Nat Rev Cancer 6(9):729–734. doi:10.1038/nrc1974
Bjornsti MA, Houghton PJ (2004) The TOR pathway: a target for cancer therapy. Nat Rev Cancer 4(5):335–348. doi:10.1038/nrc1362
Shaw RJ, Cantley LC (2006) Ras, PI(3)K and mTOR signalling controls tumour cell growth. Nature 441(7092):424–430. doi:10.1038/nature04869
Sarbassov DD, Ali SM, Kim DH, Guertin DA, Latek RR, Erdjument-Bromage H, Tempst P, Sabatini DM (2004) Rictor, a novel binding partner of mTOR, defines a rapamycin-insensitive and raptor-independent pathway that regulates the cytoskeleton. Curr Biol 14(14):1296–1302. doi:10.1016/j.cub.2004.06.054
Jacinto E, Loewith R, Schmidt A, Lin S, Ruegg MA, Hall A, Hall MN (2004) Mammalian TOR complex 2 controls the actin cytoskeleton and is rapamycin insensitive. Nat Cell Biol 6(11):1122–1128. doi:10.1038/ncb1183
Wullschleger S, Loewith R, Hall MN (2006) TOR signaling in growth and metabolism. Cell 124(3):471–484. doi:10.1016/j.cell.2006.01.016
Zhou X, Tan M, Hawthorne VS, Klos KS, Lan KH, Yang Y, Yang W, Smith TL, Shi D, Yu D (2004) Activation of the Akt/mammalian target of rapamycin/4E-BP1 pathway by ErbB2 overexpression predicts tumor progression in breast cancers. Clin Cancer Res 10(20):6779–6788. doi:10.1158/1078-0432.CCR-04-0112
Stambolic V, Tsao MS, Macpherson D, Suzuki A, Chapman WB, Mak TW (2000) High incidence of breast and endometrial neoplasia resembling human Cowden syndrome in pten ± mice. Cancer Res 60(13):3605–3611
Perez-Tenorio G, Alkhori L, Olsson B, Waltersson MA, Nordenskjold B, Rutqvist LE, Skoog L, Stal O (2007) PIK3CA mutations and PTEN loss correlate with similar prognostic factors and are not mutually exclusive in breast cancer. Clin Cancer Res 13(12):3577–3584. doi:10.1158/1078-0432.CCR-06-1609
Li G, Robinson GW, Lesche R, Martinez-Diaz H, Jiang Z, Rozengurt N, Wagner KU, Wu DC, Lane TF, Liu X, Hennighausen L, Wu H (2002) Conditional loss of PTEN leads to precocious development and neoplasia in the mammary gland. Development 129(17):4159–4170
Schade B, Rao T, Dourdin N, Lesurf R, Hallett M, Cardiff RD, Muller WJ (2009) PTEN deficiency in a luminal ErbB-2 mouse model results in dramatic acceleration of mammary tumorigenesis and metastasis. J Biol Chem 284(28):19018–19026. doi:10.1074/jbc.M109.018937
Yuan TL, Cantley LC (2008) PI3K pathway alterations in cancer: variations on a theme. Oncogene 27(41):5497–5510. doi:10.1038/onc.2008.245
Engelman JA (2009) Targeting PI3K signalling in cancer: opportunities, challenges and limitations. Nat Rev Cancer 9(8):550–562. doi:10.1038/nrc2664
Sun M, Paciga JE, Feldman RI, Yuan Z, Coppola D, Lu YY, Shelley SA, Nicosia SV, Cheng JQ (2001) Phosphatidylinositol-3-OH Kinase (PI3K)/AKT2, activated in breast cancer, regulates and is induced by estrogen receptor alpha (ERalpha) via interaction between ERalpha and PI3K. Cancer Res 61(16):5985–5991
Bellacosa A, de Feo D, Godwin AK, Bell DW, Cheng JQ, Altomare DA, Wan M, Dubeau L, Scambia G, Masciullo V, Ferrandina G, Benedetti Panici P, Mancuso S, Neri G, Testa JR (1995) Molecular alterations of the AKT2 oncogene in ovarian and breast carcinomas. Int J Cancer 64(4):280–285
Boulay A, Lane HA (2007) The mammalian target of rapamycin kinase and tumor growth inhibition. Recent Results Cancer Res 172:99–124
O’Donnell A, Faivre S, Burris HA 3rd, Rea D, Papadimitrakopoulou V, Shand N, Lane HA, Hazell K, Zoellner U, Kovarik JM, Brock C, Jones S, Raymond E, Judson I (2008) Phase I pharmacokinetic and pharmacodynamic study of the oral mammalian target of rapamycin inhibitor everolimus in patients with advanced solid tumors. J Clin Oncol 26(10):1588–1595. doi:10.1200/JCO.2007.14.0988
Tabernero J, Rojo F, Calvo E, Burris H, Judson I, Hazell K, Martinelli E, Cajal SR, Jones S, Vidal L, Shand N, Macarulla T, Ramos FJ, Dimitrijevic S, Zoellner U, Tang P, Stumm M, Lane HA, Lebwohl D, Baselga J (2008) Dose- and schedule-dependent inhibition of the mammalian target of rapamycin pathway with everolimus: a phase I tumor pharmacodynamic study in patients with advanced solid tumors. J Clin Oncol 26(10):1603–1610. doi:10.1200/JCO.2007.14.5482
Boulay A, Zumstein-Mecker S, Stephan C, Beuvink I, Zilbermann F, Haller R, Tobler S, Heusser C, O’Reilly T, Stolz B, Marti A, Thomas G, Lane HA (2004) Antitumor efficacy of intermittent treatment schedules with the rapamycin derivative RAD001 correlates with prolonged inactivation of ribosomal protein S6 kinase 1 in peripheral blood mononuclear cells. Cancer Res 64(1):252–261
Brown EJ, Albers MW, Shin TB, Ichikawa K, Keith CT, Lane WS, Schreiber SL (1994) A mammalian protein targeted by G1-arresting rapamycin-receptor complex. Nature 369(6483):756–758. doi:10.1038/369756a0
Sabatini DM, Erdjument-Bromage H, Lui M, Tempst P, Snyder SH (1994) RAFT1: a mammalian protein that binds to FKBP12 in a rapamycin-dependent fashion and is homologous to yeast TORs. Cell 78(1):35–43
Baselga J, Campone M, Piccart M, Burris HA 3rd, Rugo HS, Sahmoud T, Noguchi S, Gnant M, Pritchard KI, Lebrun F, Beck JT, Ito Y, Yardley D, Deleu I, Perez A, Bachelot T, Vittori L, Xu Z, Mukhopadhyay P, Lebwohl D, Hortobagyi GN (2012) Everolimus in postmenopausal hormone-receptor-positive advanced breast cancer. N Engl J Med 366(6):520–529. doi:10.1056/NEJMoa1109653
Andre F, Campone M, O’Regan R, Manlius C, Massacesi C, Sahmoud T, Mukhopadhyay P, Soria JC, Naughton M, Hurvitz SA (2010) Phase I study of everolimus plus weekly paclitaxel and trastuzumab in patients with metastatic breast cancer pretreated with trastuzumab. J Clin Oncol 28(34):5110–5115. doi:10.1200/JCO.2009.27.8549
Jerusalem G, Fasolo A, Dieras V, Cardoso F, Bergh J, Vittori L, Zhang Y, Massacesi C, Sahmoud T, Gianni L (2011) Phase I trial of oral mTOR inhibitor everolimus in combination with trastuzumab and vinorelbine in pre-treated patients with HER2-overexpressing metastatic breast cancer. Breast Cancer Res Treat 125(2):447–455. doi:10.1007/s10549-010-1260-x
Morrow PK, Wulf GM, Ensor J, Booser DJ, Moore JA, Flores PR, Xiong Y, Zhang S, Krop IE, Winer EP, Kindelberger DW, Coviello J, Sahin AA, Nunez R, Hortobagyi GN, Yu D, Esteva FJ (2011) Phase I/II study of trastuzumab in combination with everolimus (RAD001) in patients with HER2-overexpressing metastatic breast cancer who progressed on trastuzumab-based therapy. J Clin Oncol. doi:10.32.232110.1200/JCO.2010.32.2321
O’Regan R, Ozguroglu M, Andre F, Toi M, Heinrich G, Jerusalem M, Wilks S, Isaacs C, Xu B, Masuda N, Arena FP, Yardley DA, Yap YS, Mukhopadhyay P, Douma S, El-Hashimy M, Taran T, Sahmoud T, Lebwohl DE, Gianni L (2013) Phase III, randomized, double-blind, placebo-controlled multicenter trial of daily everolimus plus weekly trastuzumab and vinorelbine in trastuzumab-resistant, advanced breast cancer (BOLERO-3). J Clin Oncol 31(15 suppl):505
Andre F, O’Regan R, Ozguroglu M, Toi M, Xu B, Jerusalem G, Masuda N, Wilks S, Arena F, Isaacs C, Yap YS, Papai Z, Lang I, Armstrong A, Lerzo G, White M, Shen K, Litton J, Chen D, Zhang Y, Ali S, Taran T, Gianni L (2014) Everolimus for women with trastuzumab-resistant, HER2-positive, advanced breast cancer (BOLERO-3): a randomised, double-blind, placebo-controlled phase 3 trial. Lancet Oncol 15(6):580–591. doi:10.1016/S1470-2045(14)70138-X
Mabuchi S, Altomare DA, Cheung M, Zhang L, Poulikakos PI, Hensley HH, Schilder RJ, Ozols RF, Testa JR (2007) RAD001 inhibits human ovarian cancer cell proliferation, enhances cisplatin-induced apoptosis, and prolongs survival in an ovarian cancer model. Clin Cancer Res 13(14):4261–4270. doi:10.1158/1078-0432.CCR-06-2770
Weigelt B, Warne PH, Downward J (2011) PIK3CA mutation, but not PTEN loss of function, determines the sensitivity of breast cancer cells to mTOR inhibitory drugs. Oncogene 30(29):3222–3233. doi:10.1038/onc.2011.42onc201142
Lu CH, Wyszomierski SL, Tseng LM, Sun MH, Lan KH, Neal CL, Mills GB, Hortobagyi GN, Esteva FJ, Yu D (2007) Preclinical testing of clinically applicable strategies for overcoming trastuzumab resistance caused by PTEN deficiency. Clin Cancer Res 13(19):5883–5888
Rugo HS, Hortobagyi GN, Piccart-Gebhart MJ, Burris HA, Campone M, Noguchi S, Perez AT, Deleu I, Shtivelband M, Provencher L, Masuda N, Dakhil SR, Anderson I, Chen D, Damask A, Huang A, McDonald R, Taran T, Sahmoud T, Baselga J (2013) Correlation of molecular alterations with efficacy of everolimus in hormone-receptor–positive, HER2-negative advanced breast cancer: Results from BOLERO-2. J Clin Oncol 31(suppl 15):509
Neve RM, Chin K, Fridlyand J, Yeh J, Baehner FL, Fevr T, Clark L, Bayani N, Coppe JP, Tong F, Speed T, Spellman PT, DeVries S, Lapuk A, Wang NJ, Kuo WL, Stilwell JL, Pinkel D, Albertson DG, Waldman FM, McCormick F, Dickson RB, Johnson MD, Lippman M, Ethier S, Gazdar A, Gray JW (2006) A collection of breast cancer cell lines for the study of functionally distinct cancer subtypes. Cancer Cell 10(6):515–527. doi:10.1016/j.ccr.2006.10.008
Finn RS, Dering J, Ginther C, Wilson CA, Glaspy P, Tchekmedyian N, Slamon DJ (2007) Dasatinib, an orally active small molecule inhibitor of both the src and abl kinases, selectively inhibits growth of basal-type/”triple-negative” breast cancer cell lines growing in vitro. Breast Cancer Res Treat 105(3):319–326. doi:10.1007/s10549-006-9463-x
Finn RS, Dering J, Conklin D, Kalous O, Cohen DJ, Desai AJ, Ginther C, Atefi M, Chen I, Fowst C, Los G, Slamon DJ (2009) PD 0332991, a selective cyclin D kinase 4/6 inhibitor, preferentially inhibits proliferation of luminal estrogen receptor-positive human breast cancer cell lines in vitro. Breast Cancer Res 11(5):R77. doi:10.1186/bcr2419
Kalous O, Conklin D, Desai AJ, O’Brien NA, Ginther C, Anderson L, Cohen DJ, Britten CD, Taylor I, Christensen JG, Slamon DJ, Finn RS (2012) Dacomitinib (PF-00299804), an irreversible Pan-HER inhibitor, inhibits proliferation of HER2-amplified breast cancer cell lines resistant to trastuzumab and lapatinib. Mol Cancer Ther 11(9):1978–1987. doi:10.1158/1535-7163.MCT-11-0730
Barretina J, Caponigro G, Stransky N, Venkatesan K, Margolin AA, Kim S, Wilson CJ, Lehar J, Kryukov GV, Sonkin D, Reddy A, Liu M, Murray L, Berger MF, Monahan JE, Morais P, Meltzer J, Korejwa A, Jane-Valbuena J, Mapa FA, Thibault J, Bric-Furlong E, Raman P, Shipway A, Engels IH, Cheng J, Yu GK, Yu J, Aspesi P Jr, de Silva M, Jagtap K, Jones MD, Wang L, Hatton C, Palescandolo E, Gupta S, Mahan S, Sougnez C, Onofrio RC, Liefeld T, MacConaill L, Winckler W, Reich M, Li N, Mesirov JP, Gabriel SB, Getz G, Ardlie K, Chan V, Myer VE, Weber BL, Porter J, Warmuth M, Finan P, Harris JL, Meyerson M, Golub TR, Morrissey MP, Sellers WR, Schlegel R, Garraway LA (2012) The Cancer Cell Line Encyclopedia enables predictive modelling of anticancer drug sensitivity. Nature 483(7391):603–607. doi:10.1038/nature11003
Kalous O, Conklin D, Desai AJ, Dering J, Goldstein J, Ginther C, Anderson L, Lu M, Kolarova T, Eckardt MA, Langerod A, Borresen-Dale AL, Slamon DJ, Finn RS (2013) AMG 900, pan-Aurora kinase inhibitor, preferentially inhibits the proliferation of breast cancer cell lines with dysfunctional p53. Breast Cancer Res Treat 141(3):397–408. doi:10.1007/s10549-013-2702-z
Beeram M, Tan QT, Tekmal RR, Russell D, Middleton A, DeGraffenried LA (2007) Akt-induced endocrine therapy resistance is reversed by inhibition of mTOR signaling. Ann Oncol 18(8):1323–1328. doi:10.1093/annonc/mdm170
Treeck O, Wackwitz B, Haus U, Ortmann O (2006) Effects of a combined treatment with mTOR inhibitor RAD001 and tamoxifen in vitro on growth and apoptosis of human cancer cells. Gynecol Oncol 102(2):292–299. doi:10.1016/j.ygyno.2005.12.019
Bachelot T, Bourgier C, Cropet C, Ray-Coquard I, Ferrero JM, Freyer G, Abadie-Lacourtoisie S, Eymard JC, Debled M, Spaeth D, Legouffe E, Allouache D, El Kouri C, Pujade-Lauraine E (2012) Randomized phase II trial of everolimus in combination with tamoxifen in patients with hormone receptor-positive, human epidermal growth factor receptor 2-negative metastatic breast cancer with prior exposure to aromatase inhibitors: a GINECO study. J Clin Oncol 30(22):2718–2724. doi:10.1200/JCO.2011.39.0708
O’Brien NA, McDonald K, Tong L, von Euw E, Kalous O, Conklin D, Hurvitz SA, di Tomaso E, Schnell C, Linnartz R, Finn RS, Hirawat S, Slamon DJ (2014) Targeting PI3K/mTOR overcomes resistance to HER2-targeted therapy independent of feedback activation of AKT. Clin Cancer Res 20(13):3507–3520. doi:10.1158/1078-0432.CCR-13-2769
Yunokawa M, Koizumi F, Kitamura Y, Katanasaka Y, Okamoto N, Kodaira M, Yonemori K, Shimizu C, Ando M, Masutomi K, Yoshida T, Fujiwara Y, Tamura K (2012) Efficacy of everolimus, a novel mTOR inhibitor, against basal-like triple-negative breast cancer cells. Cancer Sci. doi:10.1111/j.1349-7006.2012.02359.x
Carmena M, Earnshaw WC (2003) The cellular geography of aurora kinases. Nat Rev Mol Cell Biol 4(11):842–854. doi:10.1038/nrm1245
Nigg EA (2001) Mitotic kinases as regulators of cell division and its checkpoints. Nat Rev Mol Cell Biol 2(1):21–32. doi:10.1038/35048096
Yang H, He L, Kruk P, Nicosia SV, Cheng JQ (2006) Aurora-A induces cell survival and chemoresistance by activation of Akt through a p53-dependent manner in ovarian cancer cells. Int J Cancer 119(10):2304–2312. doi:10.1002/ijc.22154
Wang X, Zhou YX, Qiao W, Tominaga Y, Ouchi M, Ouchi T, Deng CX (2006) Overexpression of aurora kinase A in mouse mammary epithelium induces genetic instability preceding mammary tumor formation. Oncogene 25(54):7148–7158. doi:10.1038/sj.onc.1209707
Taga M, Hirooka E, Ouchi T (2009) Essential roles of mTOR/Akt pathway in Aurora-A cell transformation. Int J Biol Sci 5(5):444–450
Pilot-Storck F, Chopin E, Rual JF, Baudot A, Dobrokhotov P, Robinson-Rechavi M, Brun C, Cusick ME, Hill DE, Schaeffer L, Vidal M, Goillot E (2010) Interactome mapping of the phosphatidylinositol 3-kinase-mammalian target of rapamycin pathway identifies deformed epidermal autoregulatory factor-1 as a new glycogen synthase kinase-3 interactor. Mol Cell Proteomics 9(7):1578–1593. doi:10.1074/mcp.M900568-MCP200
Zhang L, Huang J, Yang N, Greshock J, Liang S, Hasegawa K, Giannakakis A, Poulos N, O’Brien-Jenkins A, Katsaros D, Butzow R, Weber BL, Coukos G (2007) Integrative genomic analysis of phosphatidylinositol 3’-kinase family identifies PIK3R3 as a potential therapeutic target in epithelial ovarian cancer. Clin Cancer Res 13(18):5314–5321. doi:10.1158/1078-0432.CCR-06-2660
Wang X, Urvalek AM, Liu J, Zhao J (2008) Activation of KLF8 transcription by focal adhesion kinase in human ovarian epithelial and cancer cells. J Biol Chem 283(20):13934–13942. doi:10.1074/jbc.M709300200
Ellard SL, Clemons M, Gelmon KA, Norris B, Kennecke H, Chia S, Pritchard K, Eisen A, Vandenberg T, Taylor M, Sauerbrei E, Mishaeli M, Huntsman D, Walsh W, Olivo M, McIntosh L, Seymour L (2009) Randomized phase II study comparing two schedules of everolimus in patients with recurrent/metastatic breast cancer: NCIC Clinical Trials Group IND. 163. J Clin Oncol 27(27):4536–4541. doi:10.1200/JCO.2008.21.3033
Jerusalem G, Andre F, Chen D, Robinson D, Ozguroglu M, Lang I, White M, Toi M, Taran T, Gianni L (2013) Evaluation of everolimus in HER2+ advanced breast cancer with activated PI3K/mTOR pathway: exploratory biomarker observations from the BOLERO-3 European Cancer Congress 2013 Abstract Book in the European Journal of Cancer 49 (Suppl 2):Abs LBA16
Acknowledgments
DJS received Department of Defense Innovator Award W81XWH-11-1-0104. The work is also funded by a gift to DJS by The Wittich Family Project for Emerging Therapies in Breast Cancer at UCLA’s Jonsson Comprehensive Cancer Center. Dr. Hurvitz receives support from the National Cancer Institute of the National Institutes of Health under Award Number P30CA016042 and from the STOP CANCER Marni Levine Memorial Seed Grant. The content is solely the responsibility of the authors and does not necessarily represent the official views of the National Institutes of Health.
Conflict of interest
Sara Hurvitz: travel to international conferences reimbursed by Novartis, Boehringer-Ingelheim, OBI Pharma and Roche, honorarium from Genentech, research funding by Novartis Pharmceuticals, Corp. Dennis J. Slamon: honoraria from Speakers Bureau- Genentech and Sanofi; ownership interest, Amgen; consultant/advisory board, Novartis Pharmaceuticals. Richard Finn: Novartis Pharmaceuticals, Corp research funding. David Chen and Ronald Linnartz: Novartis Pharmaceuticals, Corp. employment and stock ownership. This work was funded in part by Novartis. Other authors have no potential conflict of interest to disclose.
Author information
Authors and Affiliations
Corresponding author
Electronic supplementary material
Below is the link to the electronic supplementary material.
Rights and permissions
About this article
Cite this article
Hurvitz, S.A., Kalous, O., Conklin, D. et al. In vitro activity of the mTOR inhibitor everolimus, in a large panel of breast cancer cell lines and analysis for predictors of response. Breast Cancer Res Treat 149, 669–680 (2015). https://doi.org/10.1007/s10549-015-3282-x
Received:
Accepted:
Published:
Issue Date:
DOI: https://doi.org/10.1007/s10549-015-3282-x