Abstract
Timber extraction is often cited as detrimental to wildlife ecology. Little information, however, in particular from the Southeast Asian tropics, is available on how exactly logging affects wildlife food security. To address the gap, this paper presents the first high-resolution comparison of fruit production between logged and intact forests in lowland Borneo. In the period of 2004–2008, dry weight of fruit litter was assessed as a proxy for food security of wildlife. The pheno-phases of 1,054 trees in 14 sampling plots were monitored for 54 months. A total of 143,184 fruits from 50 tree families were collected from six sampling transects totalling 810 km in 34 months. Surprisingly, logged forest (mean = 23.3 kg ha−1, SD = 48.9) produced more fruit litter than intact forest (mean = 16.7 kg ha−1, SD = 23.3), although the difference is not significant based on Student’s t test; t(66) = 0.702, p = 0.485. Pheno-phases could not be entirely explained by rainfall and temperature variables. Some evidence, however, indicates tree species composition, stand structure and sunlight exposure were likely determinants of flowering and fruit litter intensity. All things being equal, results imply selective logging if considerately practiced may increase food security for wildlife. The findings, however, should be interpreted with caution since tropical forest phenology and fruit productivity are also driven by a suite of small-scale edaphic attributes and large-scale spatio-temporal meteorological forcing. Although this research deals mainly with Borneo, the principles discussed and insights offered herein are valuable for furthering conversation around sustainable forestry in tropical Asia and elsewhere globally.
Similar content being viewed by others
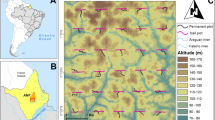
Avoid common mistakes on your manuscript.
Introduction
The Southeast Asia region is a mega-biodiversity hotspot (Mittermeier et al. 2011) with the highest proportion of endemic species globally (Myers et al. 2000). However, nearly 70% of lowland forests were lost to timber extraction and agriculture expansion (Harrison et al. 2020; Verma et al. 2020). Remnant wildlife population is now in a precarious position, and such vulnerability is exemplified in Borneo. The tropical lowland forest of Borneo was intensively logged since the 1960s in the Malaysian Sabah/Sarawak and Indonesian Kalimantan, respectively (Collins et al. 1991; Appanah and Turnbull 1998). Generally, two logging approaches were applied, namely, clear felling and selective logging (Appanah and Turnbull 1998). In clear felling practice, all trees were removed, and bare landscapes were often readapted for industrial agriculture (e.g. oil palm and rubber plantations) (Wicke et al. 2011; Gaveau et al. 2016). Selective logging, on the other hand, removes a small percentage of trees in a single operation because most tree species have no commercial value (Appanah and Turnbull 1998).
Malaysia first practised the selective management system (SMS) in the late 1970s involving felling of all commercial species (> 45 cm dbh for non-dipterocarps; > 50 cm dbh for dipterocarps) in a system of 30-year harvest cycle (Appanah and Turnbull 1998). Inevitably, the SMS was replaced by polycyclic management system (PMS) which prescribed the removal of 12–15 trees ha−1 (Appanah and Turnbull 1998). It was viewed as less destructive since dbh was no longer the felling threshold (i.e. not all large trees should be felled). The PMS approach typically yielded 90 m3 ha−1 and 120 m3 ha−1 in Sarawak and Sabah, respectively (Johns 1988; Meijaard et al. 2005). Nonetheless, although only 3% of the forest was processed with the PMS approach, studies found c. 50% portion of trees and canopy cover was lost and damaged directly or indirectly during and after the felling process (Johns 1988; Whitmore 1990; Saiful and Latiff 2014).
Although selective logging is often hailed as the answer for sustainable forestry, alarmingly, up to 41% of the unselected trees can be uprooted, crushed or suffer bark and canopy damages during the felling of selected trees (Pinard and Putz 1996). Bulldozers paths, skid trails and timber staging areas further damage the forest floor and disturb soil hydrology (Bryan et al. 2013; Tarigan et al. 2018). Whether clear felling or selective logging is deployed, it is easy to imagine how the overall forest ecology and food web vitality would be inevitably impaired. For example, if a disturbed forest is not able to sustain a reasonable level of fruit productivity after a logging exercise, frugivorous and omnivorous wildlife are likely reduced, thus offering less prey to carnivorous wildlife.
A key measure of forest vitality is fruit biomass per hectare (McCarty et al. 2002; Pessoa et al. 2017). All else being equal, forests that produce more fruit biomass are likely more valuable to wildlife. However, phenology in the tropical forest can be erratic. The lack of climatic seasonality in the tropics often gives a superficial notion that fruiting is continuous throughout the year. This is however not true. Mast flowering and fruiting in the tropical lowland forests are irregular and may occur at intervals of 2 to 10 years (Appanah 1985; Ashton et al. 1988; Azmy et al. 2016; Hosaka et al. 2017; Chechina and Hamann 2019), although a minority of tree species like those from the Ficus genus can reproduce several times annually. Past studies linked abiotic factors (e.g. rainfall, temperature) and biotic factors (e.g. pollinators, tree species physiology) for triggering synchronous flowering (e.g. Ng 1977; Ng 1981; van Schaik 1986; Wright and van Schaik 1994 ; Sakai et al. 2006; Satake et al. 2019; Ushio et al. 2020). Nonetheless, the relationship between forest fruit productivity, wildlife and these factors is still not resolutely clarified in Borneo. This raises a worrying prospect as climate change and socio-economic pressure are becoming more intense in the region. To be effective, the formulation of wildlife conservation actions needs better and more data from the field.
The objective was to clarify fruit productivity differences between selective logged and intact forests in the Danum Valley, Sabah, north Borneo. In this study, we revisited our archive and report the analysis of historical data collected during the period of 2004–2008. To drive the analysis, we asked (1) what are the levels of fruit litter productivity in logged and intact forest and (2) are phenological events linked to rainfall and temperature? We hope that the data and findings would be valuable to researchers interested to begin or continue where we left off.
In the context of our work, wildlife is regarded as an array of terrestrial vertebrates, and frugivores are dominant vertebrates in tropical forests (Gautier-Hion et al. 1985; Kitamura et al. 2002). We however observed very few vertebrates in Borneo rely entirely on fruits for sustenance. This is expected as an obligate frugivore would most probably not survive the frequent low or non-fruiting periods. Thus, most vertebrates are partially frugivorous and will consume other food sources. Nonetheless, because flowers, seeds and fruits support other wildlife feeding guilds such as nectarivores, granivores, herbivores and omnivores and these animals in turn become food resources for carnivores in the food web, there is an a priori expectation that the phenology events and fruit litter are plausible proxies for providing an overall picture of wildlife food security.
Materials and methods
In general, two key overarching metrics were sampled during the study, namely, phenology events and fruit litter. The phenology metric was observed as an “above-ground” indicator (i.e. tree canopy), while the fruit litter metric was observed as an “on-ground” indicator (i.e. forest floor). For analysis of timber extraction effects on fruit productivity, we collected and compared data from two strata, namely, (1) logged forest and (2) intact forest. Since rainfall, temperature and El Niño–Southern Oscillation (ENSO) events were explicitly included in 73.4%, 19.3% and 1.4% of phenology studies, respectively (Mendoza et al. 2017), such climatic data were also collected and examined for determining their roles in regulating phenology and fruit litter of our study site.
Study site
Study site comprised Ulu Segama Forest Reserve (USFR) (logged forest stratum) and Danum Valley Conservation Area (DVCA) (intact forest stratum) located within the Sabah Foundation Forest Management Area (9,730 km2) in Sabah, north Borneo (Fig. 1). Lowland evergreen dipterocarp forest and lower montane forest comprise about 90% and 10% of the area, respectively (Marsh and Greer 1992). The lowland evergreen dipterocarp forest is characterized as a tropical forest dominated by trees of the Dipterocarpaceae family located in regions < 500 m above sea level (Slik et al. 2003; Wulffraat et al. 2016). However tree species diversity may vary strongly between locations, even when they were close together, and diversity patterns were more pronounced for genera than for families (Slik et al. 2003). Potts et al. (2002) reported that soil variables were largely responsible for floristic composition between sites in northern Borneo.
Study site and the corresponding phenology and fruit litter sampling plots and transects, respectively. The sampling exercises in logged forest were carried out in Sepat Kalisun, Trail 1981, Trail 1989 and Trail 1990. In intact forest, the sampling exercises were deployed in West Trail, Tembaling, Trail 1, Trail 2 and Trail 3
Historically, selective logging was carried out in the lowland evergreen dipterocarp forest of USFR, averaging at 118 m3/ha (range 73–166 m3 ha−1; about 8 trees ha−1) during the 1970–1990 period (Marsh and Greer 1992). Under a 60-year rotation system, trees of > 60 cm diameter at breast height (dbh) on lowlands with < 20° slope were selectively logged (Whitmore 1984; Marsh 1995). Berry et al. (2010) reported that up to 53% of above-ground biomass was lost as a result of selective logging in the study site.
Sampling design
Phenology
In this study, we consider phenology events and fruit litter (i.e. ripe fallen fruits, including fleshy and non-fleshy remnants) as primary proxies of forest vitality, productivity and carrying capacity since 82–90% of tropical forest tree species produce fruits (Willson et al. 1989; Hanya and Aiba 2010) that are likely passed on to the second trophic level consisting a large range of wildlife community (Dew 2005).
This study adopted the same sampling plots that were examined by Norhayati (2001) to enable phenology data from Norhayati (2001) to be integrated with data from this study. The sampling exercises were stratified into two sites, namely, (1) logged forest and (2) intact forest. In logged forest stratum, the Sepat Kalisun phenology sampling plot was established. In intact forest stratum, the West Trail and Tembaling phenology sampling plots were instituted. In every plot, an area of 20 m width and 100 m length (0.2 hectare) was established, and the three plots were about 500 m apart (Fig. 1 and Table 1). All trees with > 10 cm dbh were marked, numbered and identified to species level, and pheno-phase sampling (i.e. flushing, flowering and fruiting) was carried out in the phenology sampling plots. Canopies of marked trees were visually monitored monthly for pheno-phases with binoculars as practiced in most studies (77%; Mendoza et al. 2017) from July 2004 to December 2008 (54 months).
Fruit litter
Similar to the pheno-phase sampling exercise, the fruit litter sampling transects were also stratified into two sites, namely, (1) logged forest and (2) intact forest; there were three such transects in logged forest and three in intact forest. In logged forest stratum, sampling transects were established in Trail 1981, Trail 1989 and Trail 1990. In the intact forest stratum, sampling transects were established in Tembaling (Trail 1), West Trail (Trail 2) and Borneo Rainforest Lodge (Trail 3). Each transect has an area of 0.7 m width and 2000 m length (0.14 hectare). Transects were at least 500 m apart, and distance markers were placed at 50-m interval in each transect (Fig. 1 and Table 1). The transects were cleared from leaf litter and debris after every fruit litter collection process to ensure fruit litter was visible in subsequent collections 2 weeks later. The bi-monthly sampling exercises were carried out from April 2005 to January 2008 (34 months). Transect in Trail 3 (intact forest stratum) however had to be abandoned from January 2007 onwards due to access difficulties; the path was damaged by flood. The shortcoming was not expected to affect the results since fruit litter data was normalized to the unit of g ha−1 and not the number of transects sampled.
Variable description
Pheno-phases were categorized as follows: (1) flushing (emergence of young leaves), (2) flowering (emergence and development of inflorescence) and (3) fruiting (emergence and development of infructescence). Following Norhayati (2001) who first sampled the designated phenology plots described earlier, a marked tree was considered flushing, flowering or fruiting when its canopy has a coverage of > 4% emerging young leaves, inflorescence or infructescence, respectively. Correspondingly, the presence of flower buds, blooming flowers (anthesis) and opened flowers on the canopy was collectively considered as flowering. Data were recorded through visual estimation until all flushes, flowers and fruits ceased to exist in the canopy of marked trees. The methods and thresholds described followed Norhayati (2001) to ensure data collected in this study can be integrated with data collected by Norhayati (2001) to support a more comprehensive analysis.
The morphology of fruits and leaves on tree crowns was observed with binoculars (10 × 40 magnification) for species identification. Fresh leaves, fallen dry leaves and fruit litter were further scrutinized for taxonomic confirmation, and tree species were identified by resident botanists in Danum Valley Field Centre (DVFC) with reference to the collection of DVFC herbarium and Sabah Forestry Department (Forest Research Centre, Sepilok).
A fruiting event was regarded as the period from the first observed ripe fruit to the time when almost all fruits (> 95%) had fallen. Following Ashton et al. (1988) and Numata et al. (2003), we defined 4–20% and > 20% of marked trees fruiting as minor and major mast fruiting events, respectively. All whole or decomposed fallen seeded structures with fleshy arils and non-fleshy remnants were categorized as “fruits”. Given our intent was to analyse food availability for wildlife, fleshy and tightly clustered inflorescences (i.e. syconium lined with tiny uniovulate flowers) of tree species such as those from the Ficus genus were also considered as “fruits” in an ecological sense.
Collected wet fruit litter was brought back to the laboratory for identification to species level, counted, weighed and dried in a laboratory oven at 80 °C for 48 h. Oven-dried fruit litter was weighed with an electronic scale sensitive to 0.01 g to obtain dry biomass weight. The wet and dry biomass variables were standardized to g ha−1 or kg ha−1 to enable comparison with other similar studies. We further defined a major fruiting event as a period when the dry biomass weight of fruit litter was > 2.5 kg ha−1 month−1.
Climatic data
The study site has a mean temperature of 26.7 °C and mean rainfall of 2,669 mm annually, which is typical of moist aseasonal tropical forest. Wide variations in monthly rainfall, however, may occur as the local climate is also partly driven by atmospheric movements influenced by monsoons or typhoons. Rainfall and temperature data (1985–2008) were obtained from a meteorological station operated by the Royal Society South East Asian Rainforest Research Project in DVCA. Any month recording rainfall of < 100 mm was referred to as a drought month. The Oceanic Niño Index (ONI) was monitored to determine the effects of ENSO on the study site. El Niño conditions (i.e. anomalous warming) were expected to be prevalent when ONI is + 0.5 or higher. In contrast, La Niña conditions were anticipated (i.e. anomalous cooling) when the ONI is − 0.5 or lower (Deliège and Nicolay 2017). Monthly Oceanic Niño Index data were obtained from the Climate Prediction Center, National Oceanic and Atmospheric Administration (NOAA), US Department of Commerce (https://origin.cpc.ncep.noaa.gov/products/analysis_monitoring/ensostuff/ONI_v5.php).
Statistical analyses
Statistical analyses were examined using functions provided in software IBM SPSS version 20. All linearity between variables was examined by Pearson’s correlation test. The Student’s t test was computed to determine differences in fruit litter productivity in logged and intact forests. We applied Ward’s hierarchical clustering algorithm to determine the statistical similarity or Euclidean distance, between the trails of logged and intact forest. To detect potential bias due to temporal autocorrelation, the residual maximum likelihood (REML) method often used in forest phenology studies (e.g. Gleeson and Cullis 1987; Costa e Silva et al. 2019; Bogdziewicz et al. 2020; Gastauer et al. 2020) was applied with function provided in SPSS to account for variances of fixed from random effects. Statistical values with p value equal or lesser than 0.05 were considered significant. The Kaiser–Meyer–Olkin (KMO) and Bartlett’s sphericity tests were also explored to determine whether factor analysis can be applied to explain variance maxima. KMO values of equal or greater than 0.5 were considered significant. Bartlett test p value equal or less than 0.05 was taken as significant. The Shapiro–Wilk test and Q–Q plot were applied to examine normality, and p values equal or greater than 0.05 were taken as the dataset was normally distributed.
Results
Pheno-phase correlations with rainfall and temperature data were mired by outliers when the Pearson linear correlation test was carried out; thus, statistical inferences could not be decisively applied. Similar problem was encountered by studies elsewhere, and the time series visualization method was commonly adopted to support result interpretation (e.g. Sakai et al. 1999; Numata et al. 2003; Hamann 2004; Polansky and Boesch 2013; Pires et al. 2018). Correspondingly, our results presented herein are mainly underpinned by time series illustration provided in Fig. 2.
Phenology
Across 14 sampling plots, we monitored and recorded pheno-phases of 1,054 trees in 54 months. Flushing, flowering and fruiting of various sporadic intensities occurred in logged and intact sampling plots throughout the years (Fig. 2). Comparatively, logged forest sampling plots recorded more percentage of flowering trees but fairly less percentage of fruiting trees during the study period. The West Trail (n = 140 trees; intact forest) had somewhat consistently recorded a higher percentage of fruiting trees although its sampling plots have the least trees as compared to Tembaling (n = 471; intact forest) and Sepat Kalisun (n = 443; logged forest). Data showed flowering occurrences were higher in logged forest but did not translate to higher fruiting occurrences when compared to intact forest (Fig. 2).
Fruit litter
A total length of 810 km was surveyed in six designated sampling transects throughout the sampling period of 34 months. We collected 143,184 fruits from 50 tree families with 176 and 113 trees identified to species and genus level, respectively. Trail 1990 (logged forest; 133.9 kg ha−1) recorded the highest fruit litter followed by Trail 1989 (logged forest; 70.6 kg ha−1) and Trail 2 (intact forest; 54.6 kg ha−1). As mentioned earlier, Trail 3 (intact forest) was abandoned from January 2007 onwards due to access difficulty. With only 21 months’ exercises, Trail 3 recorded a fairly high level of fruit litter at 60.3 kg ha−1. Thus, Trail 3 fruit litter was expected to exceed the amount of Trail 1989, and all sampling transects in the intact forest if it were not abandoned.
All sampling transects recorded low fruit litter from January 2006 to January 2007 (Fig. 2; see C). Since our study focused on wildlife food security, this was taken as the worst-case scenario warranting critical attention. Alarmingly, the monthly average fruit litter was only 0.393 kg ha−1 (SD = 0.366) and 0.241 kg ha−1 (SD = 0.212) in logged and intact forests, respectively, during said period.
Although the stratum of logged forest produced more fruit litter (mean = 23.3 kg ha−1, SD = 48.9) than intact forest (mean = 16.7 kg ha−1, SD = 23.3), the Student’s t test indicated that the mean difference is not significant; t(66) = 0.702, p = 0.485. This implied fruit litter amount in sampling transects of logged and intact forest did not demonstrate a stark dissimilarity. Also, the result could have been affected by outlier data recorded in 2005 and 2007 (Fig. 3). For unknown reason(s), trees in trail 1990 were exceptionally productive (55.5 kg ha−1) in July 2005. Cluster analysis and resultant dendrogram showed some trails in logged, and intact forests were grouped together (Fig. 4).
Result of cluster analysis (left) showing the levels of fruit litter similarity and time series trend (right) of each sampling transect during major fruit litter of April to October period in 2005 and 2007, respectively. Logged forest sampling transects are represented by Trail 1981, Trail 1989 and Trail 1990; intact forest sampling transects are represented by Trail 1, Trail 2 and Trail 3
Two major fruit litter episodes were recorded during the period of April to December 2005 and April to December 2007 (Fig. 2; see B and E). During peak fruit litter in July 2005, the average fruit litter of logged forest (24.4 kg ha−1) was about triple that of fruit litter in intact forest (8.6 kg ha−1). Surprisingly, the period of April to December 2005 recorded a much higher level of fruit litter although the flowering and fruiting percentage of trees were not pronounced (Fig. 2; see B). In contrast, the flowering and fruiting percentage of trees was abnormally high during the period of April to December 2007, and yet, the resultant fruit litter was clearly lower (Fig. 2; see E).
In general, sampling transects in logged and intact forests demonstrated similar monthly fruit litter trend but not intensity. Relatively higher fruit litter was recorded in more sampling transects in logged forest (Trail 1981 mean = 0.9764 kg ha−1; Trail 1989 mean = 2.077 kg ha−1; Trail 1990 mean = 3.941 kg ha−1) than in intact forest (Trail 1 mean = 0.9578 kg ha−1; Trail 2 mean = 1.606 kg ha−1; Trail 3 mean = 2.871 kg ha−1). The trees in Trail 1990 were exceptionally productive (Fig. 3) (55.5 kg ha−1) in July 2005.
An abnormal fruit litter surge occurred in March 2007 (Fig. 2; see F). Close examination showed the surge was contributed by 24 tennis ball sized fruits from a single Diospyros macrophylla tree (Ebenaceae) (total dry weight = 916 g) and a soccer ball sized fruit from a Neesia synandra tree (Malvaceae) (dry weight = 755 g) collected from Trail 2 sampling transects in intact forest. Together, fruit litter from both trees totalled 1671 g or 94% of the total 1716 g fruit litter collected in Trail 2 during that particular sampling exercise. If fruit litter from the two trees were excluded, mean dry fruit litter of intact forest for March 2007 would be < 2.5 kg ha−1 month−1. Consequently, we did not consider the surge in March 2007 as a major fruiting event.
Rainfall
During the study period, 2005 recorded the lowest annual rainfall (2,345.9 mm). The year was also the third driest year in the period of 1985–2009. In general, April and August were typically the driest months annually with mean rainfall of 142.0 mm (SD = 88.8) and 143.7 mm (SD = 62.7), respectively, compared to the mean monthly rainfall of 245.2 mm (SD = 118.3) during the study period. Based on Pearson’s correlation test, the monthly rainfall frequency and intensity could not be statistically explained by ONI (n = 288, R2 = 0.0727, p = 0.27) (Fig. 5), thus implying the ENSO cycle has no direct effect on the climate of the study site.
Flowering, flushing, fruiting and fruit litter dataset could not be statistically associated with rainfall and temperature although values were additionally examined with a time lag of 1–5 months. When a REML analysis was further performed, we found no significant temporal autocorrelation between rainfall with flushing (Tembaling, F = 0.000, p = 0.989; West Trail, F = 0.743, p = 0.394; Sepat Kalisun, F = 0.319, p = 0.575), flowering (Tembaling, F = 1.926, p = 0.172; West Trail, F = 0.482, p = 0.491; Sepat Kalisun, F = 0.023, p = 0.879) and fruiting (Tembaling, F = 0.036, p = 0.851; West Trail, F = 0.302, p = 0.586; Sepat Kalisun, F = 0.032, p = 0.858). The pheno-phases of flushing, flowering and fruiting occurred throughout the year regardless of rainfall intensity. During two major fruit litter episodes in the period of April to December 2005 and April to December 2007, total rainfall was 1837.8 mm (Fig. 2; see G) and 2424.0 mm, respectively (Fig. 2; see H). Although rainfall difference was 586.2 mm, the mean fruit litter of logged forest was about triple that of fruit litter in intact forest as highlighted earlier. Although the results showed rainfall did not consistently drive flushing, flowering, fruiting and fruit litter, we however detected the occurrence of dry months (Fig. 2; see A and D) prior to major fruit litter episodes in the period of April to December 2005 and April to December 2007.
Temperature
The mean minimum temperature was 22.6 °C, with only two values falling below 20 °C on 16–19 January 2005 (19.0–19.9 °C) (Fig. 2; see I) and 17 August 2008 (19.9 °C) (Fig. 2; see K). The temperature drops occurred at night time, and we observed the night sky was cloudless. Nonetheless, these markedly drops in temperature could not be associated with the triggering of flowering as shown in Fig. 2 (see I, J, K). Results suggest flowering tends to occur throughout the year in logged and intact forest regardless of fluctuation or sudden drop of temperature.
Discussions
Rainfall and temperature drops were previously suggested to trigger flowering in our region of study (Opler et al. 1976; Ashton et al. 1988; Appanah 1993; Sakai et al. 1999). Our results however could not support the studies mentioned but agree with a seminal study by Putz (1979, p. 13) who remarked that “… there are many exceptions” and “rainfall per se, or the lack of it, may not be the factor directly responsible for stimulating trees to flower”. Also, results showed logged forest produced more fruit litter than intact forest—which was surprising. The findings were interesting and warrant deliberation as it challenges conventional notions, especially that intact forest is supposed to be ideal for wildlife.
Although some studies agree with our findings since they found vertebrate richness was comparable or relatively higher in logged forests when compared to intact forest (e.g. Bernard et al. 2016; Roopsind et al. 2017), our analyses, however, should be interpreted with caution. We do not suggest logged forest is particularly favourable for wildlife food security, nor we are suggesting rainfall and temperature have absolutely no influence on tropical tree phenology. Consequently, we are now certain there are other proximate abiotic and biotic factors at play. Fruit productivity in aseasonal tropical forest is highly sensitive to a mix of factors (Kinnaird 1992; Appanah 1993; Numata et al. 2003). The outcomes are expected to shift even with just a slight variance in one or two direct or indirect factors. In the following sections, we discuss the possible dynamisms that may have triggered or enhanced fruit productivity, in particular mast flowering and peak fruit litter episodes. The discourse is further integrated with previous research in our study site and elsewhere globally to suggest trends and identify gaps for future research.
Tree physical composition
As mentioned earlier, relatively more monthly fruit litter was recorded in logged forest (mean = 23.3 kg ha−1, SD = 48.9) than in intact forest (mean = 16.7 kg ha−1, SD = 23.3), although the difference is not significant; t(66) = 0.702, p = 0.485. We argue the phenomenon may be explained by tree scarcity resulting from selective logging practices in the study site. With less canopy crowding, we observed sunlight reached more parts of the logged forest canopy. We argue this may have contributed to higher percentage of flowering trees recorded in the pheno-phase sampling plots of logged forest. Following Calle et al. (2010), sunlight exposure is scientifically termed as insolation or incoming solar radiation. Many studies have attributed insolation, some using the term photoperiod, for triggering mast flowering in the aseasonal tropical forest (e.g. Ng 1977; Calle et al. 2010). Studies also show insolation increase explicitly supports higher levels of photosynthesis and carbohydrate production critically needed for the fruit production process (e.g. Greene et al. 2002; Ichie et al. 2013).
In the context of our study site, a possible explanation is, with less canopy crowding (i.e. as a result of selective logging), the overall canopy surface of each tree can receive higher exposure of insolation. This may have resulted in higher flowering and fruiting intensity. Along similar lines, the measure of rainfall may actually be a useful proxy—although not robust enough for statistical inferences. Widespread stormy cloud coverage could shield off sunlight from the forest canopy, and this may be more frequent in certain periods of the year. We shall discuss the cloud coverage factor in greater detail in section "Insolation".
Tree species variation
Tropical forests in Borneo, of course, are a complex mix of tree age and species with a wide spectrum of physical and biological characteristics. As outlined earlier, there were 140 and 471 trees in pheno-phase sampling plots of West Trail and Tembaling Trail, respectively. There were more trees in Tembaling Trail although both localities were in the same stable and intact forest stratum. Also, our results showed trails in the same stratum did not record fruit litter of same intensity. In March 2007, two trees (Diospyros macrophylla and Neesia synandra) contributed 94% of the fruit litter dry weight in Trail 2 during non-fruiting period. Our data implies tree species diversity and fruit production were highly heterogenous and patchy. Such species disparity was also reported in our study area by Norhayati (2001) and other parts of Borneo (Slik et al. 2002; Howlett and Davidson 2003; Brearley et al. 2004).
The fruit litter levels recorded in logged and intact forest of our study site may have been influenced by tree species variation caused by timber extraction practices. Larger tree species were typically targeted during timber extraction exercises. If small tree species happened to produce the most fruit litter, logging practices may have systematically caused higher food production. The notion was first raised by Hanya et al. (2005) who also found forest food production in logged and naturally regenerated forest which was higher than intact forest. They found small tree species that produced more fruits had become dominant after the selective logging exercise in their study site. This was compounded by more sunlight that enhanced the flowering and fruiting capacity of small tree species when the large tree species were fell. These are plausible factors worth investigating in the future.
Many studies also linked tree species variation in the forest with small-scale disturbances, topography, climatic forcing and soil fertility (e.g. Phillips et al. 1994; Givnish 1999; Onyekwelu et al. 2008; Silva-Flores et al. 2014; Khaine et al. 2018). But the studies often fail to explain, as in our case, why two particular trees in Trail 2 were present among many other species. They did not seem to be a part of a larger cluster of D. macrophylla or N. synandra since no other similar species were observed within the vicinity. What causes such heterogeneity? We speculate wildlife diversity present in the forest may also be a factor.
Fruit adaptations, namely, colour, chemistry and morphology, are often hypothesized as an adaption of plant–animal seed–disperser interactions and mutualism (Kitamura et al. 2002; Voigt et al. 2004; Hodgkison et al. 2013; Muñoz et al. 2017). In the tropical forest, 50–75% of tree species bear fruits that are adapted for consumption by vertebrates (Howe and Smallwood 1982). In our study site, Hanya et al. (2020) investigated the foraging behaviours of five primate species and found each species has varying spatial segregation in their horizontal and vertical movements. It is therefore fair to expect foraging behaviour, movement distance and abundance of a wide range of vertebrates to have a delicate effect on tree species dispersal over temporal and spatial scale. Large vertebrates tend to select large fruits, but their population are usually small and prone to extinction from habitat degradation or loss (Kitamura et al. 2002). The quantity of dispersal services for large fruit tree species may likely be less. On the other hand, small fruits are consumed by a wider range of vertebrates, some with aerial capacity (e.g. bats and birds). Trees species with small fruits are likely more prevalent in the forest. Since each vertebrate species has unique foraging behaviour and perhaps predator avoidance strategy, it may disperse seeds in a certain location but not in another, though in the same forest stratum. When such process is further compounded by vertebrates of various sizes and movement dynamism, it will create a complex distribution pattern of seedlings in the forest over time.
Climatic factors
Rainfall
Our results showed phenology event of flushing, flowering, fruiting and fruit litter could not be explained solely or in part by temperature, ONI and rainfall factors. This is not unusual since some studies elsewhere had also showed rainfall and temperature fluxes are not decisive factors in triggering flowering and fruiting in tropical ecosystems (e.g. Kallarackal and Roby 2012; Polansky and Boesch 2013; Harrison et al. 2016). Further placing our results into a broader context, we integrated our data with data from previous research in the study site and encountered some interesting observations (Fig. 6). Although the time series trend could not explicitly show any stable correlation between fruiting episodes and climatic forcing, we could somewhat detect some sporadic conditions that may implicate rainfall.
Time series illustration showing correlation between fruiting and climatic metrics cannot be significantly established when data from this study was consolidated with previous studies (Zakaria 1994; Heydon and Bulloh 1997; Norhayati 2001; Wong et al. 2005). However, visuals from illustration show prolonged rainfall, possibly induced by anomalous cooling of La Niña, may have reduced fruit litter drastically from August 1999 to September 2000 and caused the famine described by Wong et al. (2005)
Malayan sun bears (Helarctos malayanus) and bearded pigs (Sus barbatus) in various stages of emaciation and starvation were detected from August 1999 to September 2000 (Wong et al. 2005). The period was interestingly accompanied by unusual prolonged drop of ONI (anomalous cooling period) accompanied by consistent high rainfall (Fig. 6). During the 1999–2000 famine detected by Wong et al. (2005), rainfall was consistently high. Such condition was also observed in our study as the low fruit litter period coincided with consistent high rainfall period from January 2006 to January 2007 (Fig. 2; see C); the monthly average fruit litter was only 0.393 kg ha−1 (SD = 0.366) and 0.241 kg ha−1 (SD = 0.212) in logged and intact forests, respectively. In the context of wildlife food security, this is alarming and could be the worst-case scenario. In contrast, during major fruit litter period, the climate was punctuated by numerous months of low rainfall (Fig. 2; see G). Our data showed both logged and intact forest were capable of flowering and fruiting throughout the year, regardless of rainfall and temperature fluctuation. Nonetheless, only one major fruit litter and minor fruit litter episodes were recorded. Fruit litter was generally low in other periods of time.
From field observation, we have an a priori reason to deduce that rainfall over an extended period may be detrimental to fruit productivity in tropical forests. Heavy or consistent rainfall causes flowers to drop and result in poor or no fruit set. Fruit buds may also drop prematurely in the same manner. Notably, major fruiting episodes tend to occur at 4–11 years interval in our study site (Fig. 6). However, this may not result in actual fruit litter production. As illustrated in Fig. 2 (see B), low trees flowering percentage can successfully produce high levels of fruit litter.
A total of 111 dipterocarps were reportedly dead from snapping and uprooting after an extreme rainfall event in July 2006 in Kabili-Sepilok Forest Reserve, about 100 km from our study site (Margrove et al. 2015). It would not be surprising if similar incident can occur in our study site, thus affecting fruit litter productivity. On these grounds, although rainfall could not be link to phenology events, we argue that rainfall is a valuable metric after all. Prolonged and sporadic extreme rainfall disrupts fruit setting processes, and this is not a favourable condition in the context of fruit productivity. Crucially, dry periods are imperative to support fruit production in the tropical forest of Borneo. We also speculate excessive rainfall may disturb pollination processes. These concerns should be explored by future research.
Insolation
Day length and temperature do not differ much in regions close to the equator. There are occurrences of insolation cycles, however, in the equatorial regions due to tilt and elliptic orbit of the earth around the sun. Therefore, daily insolation received by the forest canopy is also variable according to different time of the year. In our study site located in the equatorial region, equinoxes occur in March and September resulting in a bimodal cycle of insolation annually. Insolation maxima occur when the sun passes directly overhead during these months and reduces during solstices. The bimodal cycle would gradually combine at latitudes further from the equator. Insolation that reaches the forest canopy is dependent on length of daytime, and this may be different in the tropical and temperate regions according to season. Also, insolation is determined by sunlight angle striking the forest canopy. Since there is very little change in length of daytime in the tropical region, we argue synchronous flowering, or sometimes termed as mast flowering (Fig. 7), in our study site may be triggered by sunlight angle. But what about the bimodal cycle of insolation that occurs annually? Shouldn’t there be two prominent flowering events annually?
Such enigma exemplifies the complexity of tropical ecosystem and brings us back to the subject of cloud coverage pointed out earlier in section "Tree physical composition". Insolation reaching forest canopy may be substantially reduced when cloud cover is widespread during the monsoon season. Additionally, on average, c. 20 tropical typhoons of various intensities pass by the northern region of our study site throughout the year (United Nation Office for Disaster Risk Reduction (2019)), and vortex they produce can draw heavy clouds and rain to north Borneo (Ng et al. 2019). Further to this, despite its fairly small land size, the north Boneo region does not typically experience a homogenous rainfall pattern. The analysis of dataset from 2006 to 2015 suggests there are six groupings with dissimilar rainfall trends in north Borneo (Ng et al. 2019), and pheno-phase disparity is likely between two small neighbouring regions over relatively small distances. This is expected as north Borneo is flanked by the South China Sea, Sulu Sea and Celebes Sea. Intra-seasonal and inter-decadal meteorological forcing and interaction among them would certainly shape the amount of cloud coverage over north Borneo. Other climatic forcing includes the Indo-Australian monsoon system, Madden–Julian Oscillation (MJO), ENSO, Indian Ocean Dipole (IOD) and the Borneo vortex phenomenon (Manton et al. 2001; Juneng and Tangang 2005; Zheng et al. 2010; Xavier et al. 2014). As such, cloud cover over Borneo is highly influenced by the dynamics of episodic and sporadic meteorological forcing. Correspondingly, insolation can vary wildly and therefore affecting the consistency of flowering and fruiting events.
Limitations and future research
Biological and edaphic factors can generally influence fruit litter production. In our study, we did not investigate pollinator efficacy, or discern between male and female flowering trees, or analyse ages and species of trees (i.e. older or certain species of trees may produce more fruits). Although evidence from Wich et al. (2011) tells us that forest fruit productivity is generally higher in Sumatra than Borneo and soil fertility is likely the cause, we did not investigate soil quality of the study site. Borchert et al. (2005) also inform us that tropical phenology may be determined by water stress during dry season and varies widely with soil water storage ability. Research that clarifies such dynamics should be pursued. For broader perspectives, we also did not examine how wildlife persists during fruit scarcity bottleneck periods and what is the significance of asynchronous tree species such as figs in offering fallback food during the bottlenecks. We are curious to know—do climatic cues affect asynchronous tree species, even marginally?
Nonetheless, given mentioned caveats and that the phenology and fruit litter variables included in our study were fairly robust and commonly applied in other studies (Mendoza et al. 2017), we are confident the results accurately reflect relative fruits productivity of the sampling sites. We urge researchers to explore limitations highlighted in future studies. Additionally, Mendoza et al. (2017) reported only 3.2% of phenology studies, however, had included insolation data collection and analysis. This shows the insolation factor is relatively under-studied globally, much less in our region. In the advent of anthropogenic climate change, the flux and intensity of insolation are expected to shift. Will such alteration affect wildlife food security in the upcoming decades? We urge future research to focus on such concerns.
Conclusion
Study outcome underscores the profound effects of logging practices on wildlife food security. Contrary to the notion that intact forest is ideal for wildlife, our results imply food security was better in selectively logged forest. However, the findings should not be disseminated without being framed with caution. Tree species variation and physical structure in logged and intact forests may have played various circumstantial roles in creating the dissimilarity. Sustainable forestry initiatives should therefore consider fruit productivity patterns of tree species before selecting or excluding them for felling operations. We provide evidence that rainfall, ONI and temperature variances have no direct statistical linearity with phenology events and fruit productivity. Prolonged and excessive rainfall, however, is suggested to be detrimental for fruit setting. There are yet unidentified factors that drive phenology events, and we propose cloud cover intensity that affects insolation may be the unifying denominator.
Data availability
The data that support the findings of this study are available from the corresponding author upon reasonable request.
References
Appanah S (1985) General flowering in the climax rain forests of South-east Asia. J Trop Ecol 1(3):225–240. https://doi.org/10.1017/S0266467400000304
Appanah S (1993) Mass flowering of dipterocarp forests in the aseasonal tropics. J Biosci 18:457–474. https://doi.org/10.1007/BF02703079
Appanah S, Turnbull JM (1998) A review of dipterocarps: taxonomy, ecology, and silviculture. Forest Research Institute Malaysia, Kuala Lumpur.
Ashton PS, Givnish TJ, Appanah S (1988) Staggered flowering in the Dipterocarpaceae: new insights into floral induction and the evolution of mast fruiting in the aseasonal tropics. Am Nat 132(1):44–66
Azmy MM, Hashim M, Numata S, Hosaka T, Noor NSM, Fletcher C (2016) Satellite-based characterization of climatic conditions before large-scale general flowering events in Peninsular Malaysia. Sci Rep 6(1):32329. https://doi.org/10.1038/srep32329
Bernard H, Bili R, Matsuda I, Hanya G, Wearn OR, Wong A, Ahmad AH (2016) Species richness and distribution of primates in disturbed and converted forest landscapes in northern Borneo. Trop Conserv Sci 9(4):194008291668010. https://doi.org/10.1177/1940082916680104
Berry NJ, Phillips OL, Lewis SL, Hill JK, Edwards DP, Tawatao NB, Ahmad N, Magintan D, Chey VK, Maryati M, Ong RC, Hamer KC (2010) The high value of logged tropical forest: lesson from northern Borneo. Biodivers Conserv 19:985–997. https://doi.org/10.1007/s10531-010-9779-z
Bogdziewicz M, Szymkowiak J, Bonal R, Hacket-Pain A, Espelta JM, Pesendorfer M et al (2020) What drives phenological synchrony? Warm springs advance and desynchronize flowering in oaks. Agric for Meteorol 294:108140. https://doi.org/10.1016/j.agrformet.2020.108140
Borchert R, Robertson K, Schwartz MD, Williams-Linera G (2005) Phenology of temperate trees in tropical climates. Int J Biometeorol 50(1):57–65. https://doi.org/10.1007/s00484-005-0261-7
Brearley FQ, Prajadinata S, Kidd PS, Proctor J, Suriantata, (2004) Structure and floristics of an old secondary rain forest in Central Kalimantan, Indonesia, and a comparison with adjacent primary forest. For Ecol Manage 195(3):385–397. https://doi.org/10.1016/j.foreco.2004.02.048
Bryan JE, Shearman PL, Asner GP, Knapp DE, Aoro G, Lokes B (2013) Extreme differences in forest degradation in Borneo: comparing practices in Sarawak, Sabah, and Brunei. PLoS ONE 8:e69679. https://doi.org/10.1371/journal.pone.0069679
Calle Z, Schlumberger BO, Piedrahita L, Leftin A, Hammer SA, Tye A, Borchert R (2010) Seasonal variation in daily insolation induces bud break and flowering in the tropics. Trees 24:865–877. https://doi.org/10.1007/s00468-010-0456-3
Chechina M, Hamann A (2019) Climatic drivers of dipterocarp mass-flowering in South-East Asia. J Trop Ecol 35(3):108–117. https://doi.org/10.1017/S0266467419000087
Collins NM, Sayer JA, Whitmore TC (1991) The conservation atlas of tropical forests: Asia and the Pacific. McMillan Press Ltd, London
Costa e Silva J, Potts B, Harrison PA, Bailey T (2019) Temperature and rainfall are separate agents of selection shaping population differentiation in a forest tree. Forests 10:1145. https://doi.org/10.3390/f10121145
Deliège A, Nicolay S (2017) Analysis and indications on long-term forecasting of the Oceanic Niño Index with wavelet-induced components. Pure Appl Geophys 174:1815–1826. https://doi.org/10.1007/s00024-017-1491-4
Dew JL (2005) Introduction: frugivory, phenology, and rainforest conservation. In: Dew J. L., & Boubli J. P. (eds) Tropical Fruits and Frugivores. Springer, Dordrecht.
Gastauer M, Mitre SK, Carvalho CS, Trevelin LC, Sarmento PSM, Meira Neto JAA, al. (2020) Landscape heterogeneity and habitat amount drive plant diversity in Amazonian Canga ecosystems. Landscape Ecol 36:393–406. https://doi.org/10.1007/s10980-020-01151-0
Gautier-Hion A, Duplantier J-M, Quris R, Feer F, Sound C, Decoux J-P, Dubost G, Emmons L, Erand C, Hecketsweiler P, Moungazi A, Roussilhon C, Thiollay J-M (1985) Fruit characters as a basis of fruit choice and seed dispersal in a tropical forest vertebrate community. Oecologia 65:324–337. https://doi.org/10.1007/BF00378906
Gaveau DL, Sheil D, Salim MA, Arjasakusuma S, Ancrenaz M, Pacheco P, Meijaard E (2016) Rapid conversions and avoided deforestation: examining four decades of industrial plantation expansion in Borneo. Scientific Report 6:32017. https://doi.org/10.1038/srep32017
Givnish TJ (1999) On the causes of gradients in tropical tree diversity. J Ecol 87:193–210. https://doi.org/10.1046/j.1365-2745.1999.00333.x
Gleeson AC, Cullis BR (1987) Residual maximum likelihood (REML) estimation of a neighbour model for field experiments. Biometrics 43:277–288. https://doi.org/10.2307/2531812
Greene DF, Messier C, Asselin H, Fortin MJ (2002) The effect of light availability and basal area on cone production in Abies balsamea and Picea glauca. Can J Bot 80:370–377. https://doi.org/10.1139/b02-020
Hamann A (2004) Flowering and fruiting phenology of a Philippine submontane rain forest: climatic factors as proximate and ultimate causes: phenology of a submontane rain forest. J Ecol 92(1):24–31. https://doi.org/10.1111/j.1365-2745.2004.00845.x
Hanya G, Aiba S (2010) Fruit fall in tropical and temperate forests: implications for frugivore diversity. Ecol Res 25(6):1081–1090. https://doi.org/10.1007/s11284-010-0733-z
Hanya G, Zamma K, Hayaishi S, Yoshihiro S, Sugaya TY, S, Kanaoka MM, Hayakawa S, Takahata Y, (2005) Comparisons of food availability and group density of Japanese macaques in primary, naturally regenerated, and plantation forests. Am J Primatol 66:245–262. https://doi.org/10.1002/ajp.20142
Hanya G, Kanamori T, Kuze N, Wong ST, Bernard H (2020) Habitat use by a primate community in a lowland dipterocarp forest in Danum Valley Borneo. Am J Primatol 82:e23157. https://doi.org/10.1002/ajp.23157
Harrison ME, Zweifel N, Husson SJ, Cheyne SM, D’Arcy LJ et al (2016) Disparity in onset timing and frequency of flowering and fruiting events in two Bornean peat swamp forests. Biotropica 48:188–197. https://doi.org/10.1111/btp.12265
Harrison ME, Ottay JB, D’Arcy LJ et al (2020) Tropical forest and peatland conservation in Indonesia: challenges and directions. People and Nat 2:4–28. https://doi.org/10.1002/pan3.10060
Heydon MJ, Bulloh P (1997) Mouse deer densities in a tropical rainforest: the impact of selective logging. J Appl Ecol 34:484–496. https://doi.org/10.2307/2404892
Hodgkison R, Ayasse M, Häberlein C et al (2013) Fruit bats and bat fruits: the evolution of fruit scent in relation to the foraging behaviour of bats in the New and Old World tropics. Funct Ecol 27:1075–1084. https://doi.org/10.1111/1365-2435.12101
Hosaka T, Yumoto T, Chen Y, Sun I, Wright S, Numata S, Nur Supardi NM (2017) Responses of pre-dispersal seed predators to sequential flowering of Dipterocarps in Malaysia. Biotropica 49(2):177–185. https://doi.org/10.1111/btp.12371
Howe HF, Smallwood J (1982) Ecology of seed dispersal. Annu Rev Ecol Syst 13(1):201–228. https://doi.org/10.1146/annurev.es.13.110182.001221
Howlett BE, Davidson DW (2003) Effects of seed availability, site conditions, and herbivory on pioneer recruitment after logging in Sabah Malaysia. For Ecol Manag 184(1–3):369–383. https://doi.org/10.1016/S0378-1127(03)00161-0
Ichie T, Igarashi S, Yoshida S, Kenzo T, Masaki T, Tayasu I (2013) Are stored carbohydrates necessary for seed production in temperate deciduous trees? J Ecol 101:525–531. https://doi.org/10.1111/1365-2745.12038
Janzen DH (1970) Herbivores and the number of tree species in tropical forests. Am Nat 104:501–528
Johns AD (1988) Effects of ‘selective’ timber extraction on rain forest structure and composition and some consequences for frugivores and folivores. Biotropica 20(1):31–37. https://doi.org/10.2307/2388423
Juneng L, Tangang FT (2005) Evolution of ENSO-related rainfall anomalies in Southeast Asia regions and its relationship with atmosphere-ocean variations in Indo-Pacific sector. Clim Dyn 25:337–350. https://doi.org/10.1007/s00382-005-0031-6
Kallarackal J, Roby TJ (2012) Response of trees to elevated carbon dioxide and climate change. Biodivers Conserv 21:1327–1342. https://doi.org/10.1007/s10531-012-0254-x
Khaine I, Woo SY, Kwak M et al (2018) Factors affecting natural regeneration of tropical forests across a precipitation gradient in Myanmar. Forests 9:143. https://doi.org/10.3390/f9030143
Kinnaird MF (1992) Phenology of flowering and fruiting of an East African riverine forest ecosystem. Biotropica 24(2):187–194. https://doi.org/10.2307/2388672
Kitamura S, Yumoto T, Poonswad P et al (2002) Interactions between fleshy fruits and frugivores in a tropical seasonal forest in Thailand. Oecologia 133(4):559–572. https://doi.org/10.1007/s00442-002-1073-7
Manton MJ, Della-Marta PM, Haylock MR et al (2001) Trends in extreme daily rainfall and temperature in Southeast Asia and the South Pacific: 1961–1998. Int J Climatol 21(3):269–284. https://doi.org/10.1002/joc.610
Margrove JA, Burslem DFRP, Ghazoul J et al (2015) Impacts of an extreme precipitation event on dipterocarp mortality and habitat filtering in a Bornean tropical rain forest. Biotropica 47(1):66–76. https://doi.org/10.1111/btp.12189
Marsh CW (1995) Danum Valley conservation area, Danum Valley Class 1 Protection Forest Reserve, Sabah, Malaysia: management plan 1995–2000. Danum Valley Management Committee, Malaysia
Marsh CW, Greer AG (1992) Forest land-use in Sabah, Malaysia - an introduction to Danum Valley. Phil Trans R Soc London Ser B-Biol Sci 335(1275):331–339. https://doi.org/10.1098/rstb.1992.0025
McCarty JP, Levey DJ, Greenberg CH, Sargent S (2002) Spatial and temporal variation in fruit use by wildlife in a forested landscape. For Ecol Manage 164(1–3):277–291. https://doi.org/10.1016/S0378-1127(01)00612-0
Meijaard E, Sheil D, Nasi R, Augeri D et al (2005) Life after logging: reconciling wildlife conservation and production forestry in Indonesian Borneo. Center for International Forestry Research (CIFOR), Jakarta.
Mendoza I, Peres CA, Morellato LPC (2017) Continental-scale patterns and climatic drivers of fruiting phenology: a quantitative Neotropical review. Glob Planet Chang 148:227–241. https://doi.org/10.1016/j.gloplacha.2016.12.001
Mittermeier RA, Turner WR, Larsen FW, Brooks TM, Gascon C (2011) Global biodiversity conservation: the critical role of hotspots, In: Biodiversity Hotspots. Springer, Berlin.
Muñoz MC, Schaefer HM, Böhning-Gaese K, Schleuning M (2017) Importance of animal and plant traits for fruit removal and seedling recruitment in a tropical forest. Oikos 126:823–832. https://doi.org/10.1111/oik.03547
Myers N, Mittermeier RA, Mittermeier CG, Da Fonseca GAB, Kent J (2000) Biodiversity hotspots for conservation priorities. Nature 403:83–858. https://doi.org/10.1038/35002501
Ng FSP (1977) Gregarious flowering of dipterocarps in Kepong, 1976. Malays Forester 40:126–137
Ng FSP (1981) Vegetative and reproductive phenology of dipterocarps. Malays Forester 44:197–215
Ng CKC, Sikui JJ, Nur Syafiqah SK, Nilus R (2019) Precipitation trend and heterogeneity of Sabah, North Borneo. Sepilok Bull 28:19–43
Norhayati A (2001) Frugivores and fruit production in primary and logged tropical rainforests. Ph.D thesis, Universiti Kebangsaan Malaysia.
Numata S, Yasuda M, Okuda T, Kachi N, Noor NSM (2003) Temporal and spatial patterns of mass flowerings on the Malay Peninsula. Am J Bot 90(7):1025–1031. https://doi.org/10.3732/ajb.90.7.1025
Onyekwelu JC, Mosandl R, Stimm B (2008) Tree species diversity and soil status of primary and degraded tropical rainforest ecosystems in South-Western Nigeria. J Trop for Sci 20(3):193–204
Opler P, Frankie G, Baker H (1976) Rainfall as a factor in the release, timing, and synchronization of anthesis by tropical trees and shrubs. J Biogeogr 3(3):231–236. https://doi.org/10.2307/3038013
Pessoa MS, Rocha-Santos L, Talora DC, Faria D, Mariano-Neto E, Hambuckers A, Cazetta E (2017) Fruit biomass availability along a forest cover gradient. Biotropica 49:45–55. https://doi.org/10.1111/btp.12359
Phillips OL, Hall P, Gentry AH, Sawyer SA, Vasquez R (1994) Dynamics and species richness of tropical rain forests. Proc Natl Acad Sci USA 91:2805–2809. https://doi.org/10.1073/pnas.91.7.2805
Pinard MA, Putz FE (1996) Retaining forest biomass by reducing logging damage. Biotropica 28:278–295. https://doi.org/10.2307/2389193
Pires J, Marino N, Silva A, Rodrigues P, Freitas L (2018) Tree community phenodynamics and its relationship with climatic conditions in a lowland tropical rainforest. Forests 9(3):114. https://doi.org/10.3390/f9030114
Polansky L, Boesch C (2013) Long term changes in fruit phenology in a West African lowland tropical rain forest are not explained by rainfall. Biotropica 45:434–440. https://doi.org/10.1111/btp.12033
Potts MD, Ashton PS, Kaufman LS, Plotkin JB (2002) Habitat patterns in tropical rain forests: a comparison of 105 plots in northwest Borneo. Ecology 83:2782–2797. https://doi.org/10.1890/0012-9658(2002)083[2782:HPITRF]2.0.CO;2
Putz FE (1979) Aseasonality in Malaysian tree phenology. Malays Forester 42:1–24
Roopsind A, Caughlin TT, Sambhu H, Fragoso JMV, Putz FE (2017) Logging and indigenous hunting impacts on persistence of large Neotropical animals. Biotropic 49(4):565–575
Saiful I, Latiff A (2014) Effects of selective logging on tree species composition, richness and diversity in a hill dipterocarp forest in Malaysia. J Trop for Sci 26(2):188–202
Sakai S, Momose K, Yumoto T, Nagamitsu T, Nagamasu H, Hamid AA, Nakashizuka T (1999) Plant reproductive phenology over four years including an episode of general flowering in a lowland dipterocarp forest, Sarawak. Malays Am J Bot 86(10):1414–1436. https://doi.org/10.2307/2656924
Sakai S, Harrison RD, Momose K, Kuraji K, Nagamasu H, Yasunari T, Chong L, Nakashizuka T (2006) Irregular droughts trigger mass flowering in aseasonal tropical forests in Asia. Am J Bot 93(8):1134–1139. https://doi.org/10.3732/ajb.93.8.1134
Satake A, Chen YY, Fletcher C, Kosugi Y (2019) Drought and cool temperature cue general flowering synergistically in the aseasonal tropical forests of Southeast Asia. Ecol Res 34:40–49
Silva-Flores R, Pérez-Verdín G, Wehenkel C (2014) Patterns of tree species diversity in relation to climatic factors on the Sierra Madre Occidental. Mexico Plos ONE 9(8):e105034. https://doi.org/10.1371/journal.pone.0105034
Slik JWF, Verburg RW, Kessler PJA (2002) Effects of fire and selective logging on the tree species composition of lowland dipterocarp forest in East Kalimantan. Indones Biodivers Conserv 11(1):85–98. https://doi.org/10.1023/A:1014036129075
Slik JWF, Poulsen AD, Ashton PS, Cannon CH, Eichhorn KAO, Kartawinata K, Lanniari I, Nagamasu H, Nakagawa M, Van Nieuwstadt MGL, Payne J, Saridan A, Sidiyasa K, Verburg RW, Webb CO, Wilkie P (2003) A floristic analysis of the lowland dipterocarp forests of Borneo. J Biogeogr 30:1517–1531. https://doi.org/10.1046/j.1365-2699.2003.00967.x
Tarigan S, Wiegand K, Sunarti SB (2018) Minimum forest cover required for sustainable water flow regulation of a watershed: a case study in Jambi Province. Indones Hydrol Earth Syst Sci 22(1):581–594. https://doi.org/10.5194/hess-22-581-2018
United Nation Office for Disaster Risk Reduction (2019) Disaster risk reduction in the Philippines: status report (2019) United Nations Office for Disaster Risk Reduction (UNDRR). Regional Office for Asia and the Pacific, Bangkok
Ushio M, Osada Y, Kumagai T, Kume T, Pungga R, anak S, Nakashizuka T, Itioka T, Sakai S, (2020) Dynamic and synergistic influences of air temperature and rainfall on general flowering in a Bornean lowland tropical forest. Ecol Res 35(1):17–29. https://doi.org/10.1111/1440-1703.12057
Van Schaik CP (1986) Phenological changes in a Sumatran rain forest. J Trop Ecol 2:327–347. https://doi.org/10.1017/S0266467400000973
Verma M, Symes WS, Watson JEM, Jones KR et al (2020) Severe human pressures in the Sundaland biodiversity hotspot. Conservation Science and Practice 2(3). https://doi.org/10.1111/csp2.169
Voigt FA, Bleher B, Fietz J, Ganzhorn JU, Schwab D, Böhning-Gaese K (2004) A comparison of morphological and chemical fruit traits between two sites with different frugivore assemblages. Oecologia 141(1):94–104. https://doi.org/10.1007/s00442-004-1654-8
Walsh RPD, Newbery DM (1999) The ecoclimatology of Danum, Sabah, in the context of the world’s rainforest regions, with particular reference to dry periods and their impact. Phil Trans R Soc B-Biol Sci 354(1391):1869–1883. https://doi.org/10.1098/rstb.1999.0528
Whitmore TC (1984) Tropical rain forests of the Far East. Clarendon Press, Oxford
Whitmore TC (1990) An introduction to tropical rain forests. Clarendon Press, Oxford
Wich SA, Vogel ER, Larsen MD et al (2011) Forest fruit production is higher on Sumatra than on Borneo. PLoS ONE 6(6):e21278. https://doi.org/10.1371/journal.pone.0021278
Wicke B, Sikkema R, Dornburg V, Faaij A (2011) Exploring land use changes and the role of palm oil production in Indonesia and Malaysia. Land Use Policy 28(1):193–206. https://doi.org/10.1016/j.landusepol.2010.06.001
Willson MF, Irvine AK, Walsh NG (1989) Vertebrate dispersal syndromes in some Australian and New Zealand Plant Communities, with Geographic Comparisons. Biotropica 21(2):133. https://doi.org/10.2307/2388704
Wong ST, Servheen C, Ambu L, Norhayati A (2005) Impacts of fruit production cycles on Malayan sun bears and bearded pigs in lowland tropical forest of Sabah, Malaysian Borneo. J Trop Ecol 21:627–639. https://doi.org/10.1017/S0266467405002622
Wright SJ, van Schaik CP (1994) Light and the phenology of tropical trees. Am Nat 143(1):192–199
Wulffraat S, Greenwood C, Faisal KF, Sucipto D, Chan H, Beukeboom H, Soulisa N, Kinasih A (2016) The environment status of Borneo. WWF Heart of Borneo Programme, World Wildlife Fund (WWF), Jakarta. https://d2ouvy59p0dg6k.cloudfront.net/downloads/supplementary_report_rev_6_web_version_1.pdf
Xavier P, Rahmat R, Cheong WK, Wallace E (2014) Influence of Madden-Julian Oscillation on Southeast Asia rainfall extremes: observations and predictability. Geophys Res Lett 41(12):4406–4412. https://doi.org/10.1002/2014GL060241
Zakaria MH (1994) Ecological effects of selective logging in a lowland dipterocarp forest on avifauna, with special reference to frugivorous birds. Universiti Putra Malaysia.
Zheng XT, Xie SP, Vecchi GA, Liu Q, Hafner J (2010) Indian Ocean Dipole response to global warming: analysis of ocean–atmospheric feedbacks in a coupled model. J Clim 23(5):1240–1253. https://doi.org/10.1175/2009JCLI3326.1
Acknowledgements
We thank the Economic Planning Unit of the Malaysian Federal Government (approval code UPE 40/200/19 SJ.1204), the Danum Valley Management Committee and the Sabah Wildlife Department for permission to conduct this study in Sabah. We are also grateful to C. Ong, N. Abraham, LEAP Spiral, G. Reynolds, M. B. Ola, S. D. Teo, D. Ampingan, C. Wultch, K. S. Kim, T. C. Koh, W. P. Ng, R. Murus, G. Sanders, S. Utung, H. Betts, Ratnasingam, S. Kadir. S. Ahchong, A. James, C. Clarke and all staff of Danum Valley Field Centre for the unwavering support.
We dedicate this paper to field assistant Teck-Chai Koh who met with a fatal accident during this study. We lost a great ecologist and wonderful friend. Teck-Chai will forever remain in our memories and we shall honour him by continuing his passion of tropical ecology research.
Funding
Financial support was generously provided by the Alexander Abraham Foundation; the Columbus Zoo and Aquarium; the Department of State — the USA, via the US Embassy in Malaysia; the International Association for Bear Research and Management; the Lincoln Park Zoo; the Singapore Zoological Gardens; and the Woodland Park Zoo.
Author information
Authors and Affiliations
Contributions
STW conceived the idea and methodology; STW led the field data collection exercises; STW and CKCN analysed the data and produced the manuscript. Both authors contributed critically to the drafts and gave final approval for publication.
Corresponding author
Ethics declarations
Conflict of interest
The authors declare no competing interests.
Rights and permissions
About this article
Cite this article
Wong, S.T., Ng, C.KC. What drives food security for wildlife in logged and intact forests of tropical Borneo?. Int J Biometeorol 66, 753–767 (2022). https://doi.org/10.1007/s00484-021-02234-6
Received:
Revised:
Accepted:
Published:
Issue Date:
DOI: https://doi.org/10.1007/s00484-021-02234-6