Abstract
The present study was carried out in the N’Djamena and Hadjer-Lamis localities (Lac Chad basin). The main objective was to examine the groundwater quality for domestic and agricultural purposes, using hydro-chemical modeling, Water Quality Index and Geographical Information System Technics. Thus, 66 groundwater samples were collected from 22 boreholes for physicochemical and biological analysis. The analytical parameters considered were: pH, electrical conductivity, Ca2+, Mg2+, Na+, K+, NH4+, HCO3−, NO3−, F−, SO42−, Fecal Coliform, Total Coliform, Escherichia Coli, Fecal enterococci and Mesophyll Aerobic Flora. The results revealed that groundwater of the studied zone presents a neutrality (6.40 ≤ pH ≤ 7.57), very weakly to weakly mineralized (92 ≤ electrical conductivity ≤ 590 µS/cm) with only one water type (Ca–Mg–HCO3), characteristic of the homogenous quaternary aquifer, the most used. The decreasing order of magnitude of the cations is as: Ca2+˃Mg2+˃Na+˃K+, while that of anions is HCO3−˃Cl−˃SO42−˃NO3−. The Water Quality Index varied from 188.41 to 866.89, corresponding to poor (9.09%), very poor (18%) and unsuitable (72%) for drinking. The poor quality is linked to the presence of bacteriological species. It should therefore be noted that groundwater in N’Djamena and Hadjer-Lamis can be used to irrigate crops, but it should be purified before being used for drinking purposes. In general, this study provides an overview of the aquifers characteristic in the Lake Chad Basin, and calls on local and administrative authorities and development partners to effectively cooperate in the permanent monitoring and preservation of the quality of this water. This would undoubtedly guarantee their sustainable and efficient management.
Similar content being viewed by others
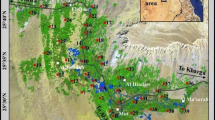
Explore related subjects
Discover the latest articles, news and stories from top researchers in related subjects.Avoid common mistakes on your manuscript.
Introduction
Water is a natural resource that is essential for the full development of communities (United Nation, 2018; Tontsa et al., 2022; Kouassy Kaledjé et al., 2023). It is considered as a vector of socio-economic and cultural development. To corroborate that, it is used in households for drinking and other tasks, in the agri-food and brewing industries, and in agro-sylvo-pastoral activities for the production of goods and services, including the field of leisure (Frsat et al., 2023; Subba Rao et al., 2021a). Access to this precious resource remains a major concern for the international community (Frsat et al., 2023). In term of statistics related to water supply and sanitation around the world made by the World Health Organization (WHO), a single human being requires between 50 and 100 L of water to ensure that its most basic needs are met. In fact, currently, approximately 884 million people still lack access to safe drinking water and more than 2.6 billion do not have access to basic sanitation (Barrie et al., 2023). It is also estimated that approximately 1.5 million children under 5 years of age die each year as a result of water and sanitation-related diseases (Barrie et al., 2023).
On a global scale, water resources face enormous pressures that are both natural and anthropogenic (Sorlini et al., 2013; Seeber et al., 2014; Vetrimurugan Elumalai et al., 2017; Yenugu et al., 2020; Seben et al., 2021; Ngo Billong et al., 2023; Bekele et al., 2023). Indeed, the growing population coupled to numerous community wars, insecurities and climate changes have led to increase demand for water for drinking and irrigation usages. All of these pressures negatively impact these resources from both a qualitative and quantitative perspective. It is why assessment of groundwater quality for drinking and irrigation usages in developing countries is important, since water quality is a fundamental aspect of groundwater management (Li et al., 2019; Wu et al., 2019; Gad et al. 2020).
Central Africa in general and Chad in particular are no exception to the rule, due to its geographical position. The latter is entirely located in a Sudano-Sahelian climate with low rainfall (less than 600 mm/year) that decreases from south to north (Mahamat Nour et al., 2019; Mbadngonel Morbe, 2018; Roudouane et al., 2020). Access to water for domestic needs in most areas of the Lake Chad Basin is primarily from groundwater through boreholes and wells (Abderamane, 2012; Abderamane et al., 2013 and 2017, Mbadngonel Morbe, 2018). Besides, neither the quality nor the productivity of these aquifers are sufficiently known. Likewise, these water resources are subject to multiple problem including, agriculture, industry, high demand for human consumption, lack of hygiene and sanitation and climate change (Frsat et al., 2023; Subba Rao et al., 2021a). According to United Nation (2013), Song et al. (2023), the Sahel is a semi-arid region with a surface area of 3 million km2, representing about 10% of the total area of the African continent. It is among the areas most affected worldwide by the issue of water scarcity as it is vulnerable to the effects of climate change, not only because of the aridity of the climate but also because of environmental degradation.
The city of N'Djamena in particular counts about 1,000,000 inhabitants, but less than 41% have access to drinking water (Abderamane, 2012); Abderamane et al., 2013, 2017; Mahamat Nour et al., 2019). Those authors revealed that access to drinking water in the region is 56%, compared to 16% for sanitation. Based on the literature, current knowledge of the water resources of the Lake Chad basin which is considered as a vulnerable ecological zone is still patchy (Abderamane, 2012; Bon et al., 2020; Mahamat Nour et al., 2019; Ombolo et al., 2016). Some of the research carried out there mostly focused on the surface waters of the Logone and Chari in relation to the alteration and transport of sediment flows. Even though there are recent works on water quality such as those of Bon et al., (2020), Song et al., 2023; they mostly focused on the physico-chemical and isotopic aspects. According to the first authors, the quality of groundwater in the Lac Chad basin, revealed a general good quality for both drinking and irrigation, with the exception of certain boreholes in the 1st, 5th, 8th, and 9th districts of N’Djamena. However, this study did not take into account biological parameters. Song et al., (2023) at the transboundary quaternary aquifer of the Lake Chad Basin scale show that: (1) the multi-layered structure of the regional aquifer was highlighted with shallower and intermediate to deep flow paths; (2) the shallower aquifer is recharged with rainwater and inter-connected with surface water; (3) groundwater chemistry indicates geogenic influences in addition to a strong anthropogenic fingerprint and the intermediate to deep aquifer shows a longer residence time of groundwater, less connection with the surface and no to only a little anthropogenic influence. This work and many other does not in any way state whether these waters are of good quality for human consumption or not. This lack of information regarding the hydro-geochemical and hydro-biological aspects does not favour the rational and objective management of this resource, and even less so in the future when additional needs will be felt even more (intensification of agriculture and use of chemical fertilizers, grazing, population growth and the need for security in relation to climate change). This lack of understanding of water resources and the resulting health, economic, environmental and conservation consequences contrasts with several African and global development agendas, including the Sustainable Development Goals (SDGs). Hence, groundwater quality is an important environmental concern that must be addressed and monitored; indeed, the locality of N’Djamena with arid climate that is sensitive to climate change face a delicate and crucial issue with water quality. Public awareness is needed to ensure water quality preservation. In this regards, the present study intends to (1) investigate groundwater quality based on hydrogeochemical and bacteriological conditions; (2) assess the suitability of the groundwater for drinking and irrigation purposes using Water Quality Index (WQI) and hydro-chemical modeling; (3); to identify the environmental factors controlling mechanisms affecting water quality.
The specificity of the present study is linked to the fact that water quality assessment using the WQI takes into account the biological species which are the pollutant parameters, contrary to several other works which focused exclusively on physicochemical parameters and accessorily on heavy metals. Likewise, statistical analysis was also used to discriminate the studied parameters in order to better understand the N’Djamena hydro-system characteristics. The integration of physicochemical and bacteriological parameters, hydro-geochemical modeling, and WQIs calculation coupled to the geo-statistical analysis and GIS techniques provides a valuable framework for assessing patterns, defining particular environmental problems, and sharing knowledge on water resources, geochemical processes, and water quality. This study is a contribution to a better understanding of groundwater quality in the study area, which would undoubtedly contribute to their efficient management such as the different methods of treatment before consumption and other domestic uses.
Materials and methods
Study area
The studied zone is situated in the southwestern part of Chad, particularly in N’Djamena and Hadjer-Lamis localities, in Central Africa (Fig. 1). The climate is humid monsoon of oceanic origin in the south-western part and the dry Harmattan of continental origin in the north-east (Mbadngonel Morbe, 2018; Souabe, 2013). Based on the hydrological data (precipitation and temperature) obtained in the “Direction des Ressources en Eau et de la Météorologie” of N’Djamena covering the period from 2010 to 2016, annual rainfall varied between 400 and 1000 mm, for the inter-annual mean of 593.5 mm; five rainy months (April to August) and seven dry months (September to March) are observed. The temperature fluctuates from 27.9 to 44.8 °C. The highest temperatures are observed within April–May, while the lowest are observed within December-January. From a geomorphological point of view, altitude varies between 279 and 300 m (Fig. 1).
From a geological point of view, the Lake Chad basin is made up of largely homogenous and continuous sedimentary deposit throughout the basin (Djoret, 2000; Djoret & Favreau, 2014; Louis, 1970; Roudouane et al., 2020). At a local level, however, there are strong horizontal lithological and granulometric heterogeneities. According to Djoret and Favreau (2014), most of the zone is covered by Quaternary sands, below the sands, at about 75 m depth, appear the clays of the Pliocene with a mean thickness of approximately 280 m. Further down, a layer of sand of about 30 m thickness and belonging to the Lower Pliocene is encountered. After that appear sandstones of the Continental Terminal (Tertiary) with a thickness of about 150 m.
At the hydrogeological aspect, illustrated in Figs. 2 and 3, Schneider and Wolf (1992); Schneider (2001); Ngounou Ngatcha (2009); Vassolo (2012); Abderamane et al., (2013) Lemoalle and Magrin (2014) highlight several aquifer types. There are: (1) the recent quaternary aquifer which is made up of small perched aquifers (10–15 m depth). (2) The ancient quaternary water table made up of sandy-clay formations from the quaternary period. (3) The deep aquifers which are found in the middle and lower Pliocene formations and contain a confined aquifer under the clayey sequences of the upper Pliocene (United Nation Development Programme, 2002).
Sampling and analytical methods
Two field campaigns were conducted in August and February 2018, covering the rainy and dry periods. During the August field trip, 22 boreholes were identified (Table 1) in the urban environment. It is important to note that the choice of the sampling sites was made at random, according to the availability of the boreholes and the authorization of the owners or the maintenance agent. The surroundings of these boreholes were also described in order to better explain and discuss the analytical results. After that, these points were spatially represented using SRTM (Shuttle Radar Topography Mission) satellite image. Apart from borehole 7 (B7) and 14 (B14), all others have minimum depth of 30 m. During the same period, the physicochemical parameters like pH, temperature, turbidity, electrical conductivity (EC) were measured in situ using the pH-meter calibrated with two solutions such as pH = 4, and pH = 7 respectively. The turbidity was measured with the turbidy-meter calibrated with the solutions of 00 NTU (nephelometric turbidity units) and 100 NTU. Samples for chemical analysis were collected respecting scrupulously the method described by Rodier (2009); Fahmida et al., (2021); Tontsa et al., (2022). Thus, the bottles intended for sampling were previously rinsed in the laboratory with distilled water. During the sampling, they were also cleaned at least three times with sample water. A few drops of acid (0.1 N HNO3) were added to sample bottles for cations analysis in order to avoid any mineral precipitation. The analyses were carried out in the « Laboratoire d’Analyse des Eaux (LAE) du Ministère de l’Hydraulique et de l’Elevage» of N’Djamena, using high-performance liquid chromatography (HPLC). The concentrations of Ca2+, Mg2+, and SO42− were measured using EDTA titration method with errors of ± 1%, ± 1%, and ± 0.5% respectively. The K+ and Na+ concentrations were determined using flame atomic absorption spectrophotometry with an accuracy of 0.1 mg/L. The anions concentrations were analyzed using argentometric titration with a relative error of ± 1%. All the analyses have been made observing the standard procedures proposed by American Public Health Association (APHA, 2012; Bon et al., 2020; Ngo Billong et al., 2023; Subba Rao et al., 2021a, 2021b). Regarding the bacteriological analyses, samples were collected in August and February. The samples were taken in bottles sterilized by autoclave and with alcohol at 90°. All samples were stored in ice chests at 4 °C and transported in the Wastewater Research Unit laboratory of the University of Yaoundé 1 (Cameroon). The determination of the bacteriological parameters in the samples was carried out by the method described by Djaouda et al., (2014); Sana et al., (2019); Getachew et al., (2021); Tontsa et al., (2022). The bacteria were identified and counted by filtering 100 ml of the water sample through a cellulose ester membrane with a porosity of 0.45 μm. The membranes were then placed on selective media for 24 h at 44 °C. ENDO AGAR lactose medium, Slanetz medium, Slanetz, and Bartley medium were, respectively, used to select and grow bacteriological species (Tontsa et al., 2022).
The concentration of measured bio-indicators is expressed as Colony Forming Unit (CFU) per unit volume of 100 mL based on Eq. (1) below:
Hydro-geochemical data validation
After the physicochemical analysis, the analytical results were validated by calculating the Ionic Balance Error (IBE) of the hydro-chemical elements whose formula is as follows (Eq. 2) (Gad & El Osta, 2020; Mfonka, 2019; Mfonka et al., 2015; Subba Rao et al., 2021a). All the values varied between ± 10 percent, reflecting the good analytical results.
In conclusion, based on the data collection and treatment, the similarity among the analytical results and the fact that they are managed by the specialized laboratories and academic researchers must be noted, for that assures the measurement methods homogeneity and the quality of the results.
Drinking water quality
WQI calculation
There are many methods used to evaluate the water quality index such as Drinking Water Quality Index (DWQI) or Water Quality Index (WQI), Irrigation Water Quality Index (IWQI) and Entropy Weighted Groundwater Quality Index (EWGQI) (Chimankpam Ezugwu et al., 2019; Ngo Billong et al., 2023; Obiri et al., 2021; Prusty & Farooq, 2020; Şener et al., 2017; Yenugu et al., 2020). The WQI is the widely used method (Ngo Billong et al., 2023; Obiri et al., 2021; Yenugu et al., 2020). To evaluate the water quality index in the present study, each of the chemical and biological parameters were assigned different weights (wi) in a scale of 1–5 (Table 2), representing the least effect to the highest effect on water quality respectively, based on their perceived effects on primary health and according to its relative importance in the drinking water quality (Chimankpam Ezugwu et al., 2019; Ngo Billong et al., 2023; Obiri et al., 2021; Prusty & Farooq, 2020; Şener et al., 2017; Yenugu et al., 2020). The highest weight of 5 was assigned to parameters that have critical health effects and whose presence above the critical concentration limits could restrain the usability of the resource for domestic and drinking purposes (Ghouili et al., 2018; Obiri et al., 2021; Yenugu et al., 2020). The relative weight (Wi) is computed from the following equation (Eq. 3):
In this equation, Wi expresses the relative weight, wi the weight of each parameter and “n” the number of parameters. The calculated relative weight (Wi) values of each parameter are given in Table 2. The quality rating (qi) for each parameter is assigned by dividing its concentration in each water sample by its limit values given by World Health Organization (2017).
qi is calculated as follow (Eq. 4):
where qi, Si and Ci are respectively the quality rating, the concentration of each hydro-chemical parameter in each water sample and the drinking water standard for each chemical parameter in milligrams per liter, according to the guidelines for the World Health Organization (2017).
Before gating WQI index value, SI was calculated based on the equation below (Eq. 5):
Finally, the WQI value is calculated with the following equation (Eq. 6):
In Eq. (6), SI is the sub-index of ith parameter; the computed WQI values are classified into five categories presented in Table 3. With regard to the limitations of the method based on the WQI used in the present study, it is important to specify that several authors do not take bacteriological species into account in the process of assessing the drinking water quality. In addition, the weights assigned to certain parameters are random, which does not always guarantee the accuracy of the method.
Irrigation water quality
Sodium absorption ratio (SAR)
The sodium absorption ratio is an important index for determining the suitability of water used in irrigation (Ghouili et al., 2018; Shwan Seeyan, 2020; Abugu et al., 2021). According to these authors, the excess of sodium concentration in groundwater affects the soil properties and reduces the soil permeability. The SAR is calculated from the ratio of sodium to calcium and magnesium, using the following formula (Eq. 7):
Depending on the sodium absorption ratio value, water can be subdivided in to 3 categories: water with SAR ranging from 0 to 3 considered as good, SAR value ranged between 3 and 9 characterized by moderate type and greater than 9 considered unsuitable for irrigation purpose (Ghouili et al., 2018; Shwan Seeyan, 2020; Abugu et al., 2021; Ngo Billong et al., 2023) (Table 4).
Sodium percent (Na%)
Sodium percent is defined as the percentage of soluble sodium in water (Abugu et al., 2021; Ahamed et al., 2015). It is calculated to assess the suitability of groundwater for agricultural purposes. This parameter represents a significant sodium hazard in surface and groundwater. It contributes significantly to the deterioration of the subsoil structure and consequently, reduces its permeability (Ayed et al., 2017; Chimankpam Ezugwu et al., 2019; Gad & El Osta, 2020). According to Ayed et al., (2017), a high sodium concentration in irrigation water leads to its absorption by the clay particles, and it will be substituted by Magnesium and Calcium ions. This substitution reduces the soil permeability. The Sodium percent was calculated as follows (Wilcox, 1955) (Eq. 8):
Permeability index (PI)
The Permeability Index is an irrigation water assessment index used in quantifying the suitability of water for irrigation purposes (Sanjoy et al., 2019). Based on the PI value, irrigation water can be categorized into three classes, namely Classes 1, 2 and 3 (Table 4). Classes 1 (PI ≥ 75%) and 2 (25% < PI < 75%) are considered as suitable for irrigation, while Class 3, with PI ≤ 25% is considered unsuitable for irrigation use. The Permeability Index parameters are evaluated using the equation below (Eq. 9), proposed by Richards (1954) and Wilcox (1955).
Magnesium percent (%Mg)
The magnesium percent is another parameter which describes the water quality for irrigation as long as magnesium and calcium keep the equilibrium state in most waters. The excess of magnesium affects the soil quality (increases the construction of alkalinity) which can decrease the productivity (Frsat et al., 2023; Ramesh & Elango, 2012). The Magnesium percentage was obtained using the equation below (Eq. 10), with the ions concentrations which constitute the formula in meq/l (Ayed et al., 2017; Chimankpam Ezugwu et al., 2019):
Identification of the factors controlling groundwater quality in the study area
Saturation index calculation
The saturation index (SI) is useful to understand the different stages of hydro-geochemical evolution. It also helps in identifying the geochemical processes responsible for hydro-geochemical characteristics (Mfonka et al., 2021; Singh et al., 2017). In this study, the SI has been calculated using Diagram software, following the formula 11 below (Eq. 11):
where, IAP is the ion activity product of the dissociated chemical species in solution and Kt = equilibrium solubility product of the mineral.
According to Merchán et al., (2015), Paternoster et al., (2016), Yang et al., (2017), Singh et al., (2017), When SI < 0, the solution is considered under saturated. When SI = 0, the solution is saturated, and equilibrium is achieved between mineral dissolution and precipitation. When SI > 0, the solution is oversaturated, and extra minerals precipitate.
Gibbs model (1970)
The Gibbs model is the method used to identify and understand the source of groundwater mineralization between water–rock interaction, precipitation and evaporation phenomenon (Venkatesan et al., 2021; Al Haj et al., 2022; Ngo Billong et al., 2023). The Gibbs value and the diagram have been obtained using Diagram software which use the equation below (Eq. 12)
Statistical analysis method
In this research, the statistical analysis related to correlation matrix (CM) and Q-mode hierarchical clustering where specifically performed to identify the most important mecanism affecting the groundwater chemistry and the hydrochemical dynamic in the various groundwater reservoirs of the study. Those technics are used due to their efficiency, specifically in water sciences (Rakotondrabe et al., 2018; Diani et al., 2021; Johnbosco Egbueri and Johnson Agbasi 2021; Tanushree Chakravarty and Susmita Gupta, 2021; Ramadhani Mussa & Chikira Mjemah, 2023). According to Johnbosco Egbueri and Johnson Agbasi 2021; Güler et al., (2002), HCA is one of the most used classification methods in hydrogeochemistry which uses a dissimilarity matrix based on Euclidean distance in order to group samples into groups of relatively similar characteristics. It identifies the differences between samples and group them into several clusters based on their differences (Jiang et al., 2015). The clusters are generated by minimizing the sums of square distance to the mean center, while the correlation matrix makes it possible to establish direct, sometimes indirect or even inverse relation between the parameters taken in pairs, the hierarchical clustering analysis proposes similarity or disimilarity links between these same parameters which are either variables or labels. These technique require an abundant range of data and quite satisfactory results, which is sometime difficult when using simple processing techniques. The 2014 XLSTAT sofware version was used for this purpose.
Inverse distance weighting (IDW) interpolation
In this study, Inverse Distance Weighting (IDW) interpolation method was used to construct the thematic maps which illustrate the spatial distribution of some parameters (WQI, PI and Mg). According to Rakotondrabe et al., (2018); Cabalar et al., (2021) this method is widely used in geology, particularly in hydrogeology, hydro-geochemistry for their subtlety. The general formula is presented as follow (Eq. 13):
where: Ẑ (S0) is the value we are trying to predict for location S0; N is the number of measured sample points surrounding the prediction location that will be used in the prediction; λ are the weights assigned to each measured point that we are going to use. These weights will decrease with distance; Z (S1) is the observed value at the location S1.
The formula to determine the weights is the following (Eq. 14):
As the distance becomes wider, the weight is reduced by a factor of p.
The quantity di0 is the distance between the prediction location, S0 and each of the measured locations Si. The power parameter p influences the weighting of the measured location’s value on the prediction location value; that is, as the distance increases between the measured sample locations and the prediction location (Vetrimurugan Elumalai et al., 2017). The weights for the measured locations that will be used in the prediction are scaled so that their sum is equal to 1. The methodology used in the present study is summarized in the flowchart presented in Fig. 4.
Results and discussion
Physicochemical characteristics of major elements
The statistical description summary of the physicochemical characteristics of the groundwater in the southwestern part of N’Djamena is presented as a box plot in Fig. 5. Globally, it appears that the quality of groundwater is not uniformly distributed, which suggests a high spatial variability due to the involvement of different sources and/or geochemical processes, taking place in the aquifer system (Subba Rao et al., 2021a, 2021b). The pH values of the 22 samples varied from 6.40 to 7.57, with a mean value of 6.75 ± 0.33. These results indicated that, the groundwater of N’Djamena locality are slightly acid to alkaline. Temperature values fluctuated between 28.00 and 31.0, with a mean of 29.40 and a median of 29.45 °C. These high values can be explained by the depths of the boreholes, as well as the arid climate that characterises this environment. For electrical conductivity (EC), the values range from 92 to 590 μS/cm, for a mean of 278.32 ± 139 μS/cm. These indicate the freshwater quality (Rakotondrabé et al., 2017; Ayed et al., 2017). The standard deviations obtained through statistical calculations confirm the opinion of Subba Rao et al., (2021a, 2021b) presented above, indicating that there are many sources and/or geochemical processes taking place in the studied zone. The relatively high values of EC are probably related to (1) the long residence time of the water in the different reservoirs of the studied zone, and (2) the slow renewal of groundwater resources due to the long dry season that characterizes this environment. Some elements present the outlier/extreme values like EC, Turbidity, K+, HCO3−, Cl−, NO3− SO43−. According to Ramadhani Mussa et al., (2023) it is due to the influence of anthropogenic activities and the topography variation of the studied zone (presence of recharge and stagnant groundwater zone). These values are in the same order of magnitude with those obtained by Ayşen Davraz and Burcu Batur (2021) in Yalvaç-Gelendost basin in Turkey. The turbidity’s values are very low (Turb ≤ 4 NTU). Nevertheless, there is an outlet value of about 83 NTU. As regarding the hydrochemical parameters related to cations (Ca2+, Mg2+, K+, Na+) and anions (HCO3−, Cl−, NO3−, SO42−) analytical results have revealed concentration which does not exceed those prescribed by the World Health Organization for human consumption. The decreasing order of magnitude of the cations is as: Ca2+ ˃ Mg2+ ˃ Na+ ˃ K+, while that of anions is HCO3− ˃ Cl− ˃ SO42− ˃ NO3−. It is noted that the concentration of some elements in this study such as Cl− are much lower compared to those obtained by Ayed et al. (2017), Aouiti et al. (2020) in the sedimentary environment of North Africa. These differences are probably linked to the fact that the N’Djamena locality is located in an endoreic sedimentary basin, unlike the other environment which is open to the sea characterized by the saline intrusion in some places and characterized by high evaporation and the presence of salty marshes.
Piper’s diagram
The water types given by the Piper’s diagram is presented in Fig. 6. It reveals in its lower left part that the dominant cations in all the samples are Calcium, followed by Magnesium; whereas anion in its lower right is dominated by HCO−3 only. This results in Ca-Mg-HCO3 water type mainly. The only water type obtained by the diagram software reflects the local homogeneity of the geology of the Lake Chad, as indicated by Louis (1970); Djoret and Favreau (2014). Aquifers in sedimentary environment are more or less continuous; the contrast to those of the basement milieu, where several studies revealed the discontinuous nature of the latter environment; this being linked to the polyphase alteration that occurs there (Mfonka et al., 2021). These results are comparable to those obtained by Song et al. (2023) on a large scale of transboundary quaternary aquifer of the Lake Chad Basin.
Bacteriological investigation results
Table 5 shows that all the samples from the study area contain bacteriological parameters in varying proportions during the dry and rainy seasons. In general, there is an almost complete absence of faecal enterococci in all the investigated boreholes, with the exception of B3, B4, B5 and B11, which contain these parameters either in the dry season (B3), or in the rainy season (B4 and B11), or during both periods (B5). Another peculiarity can be observed in borehole B20 where only TMAFs are displayed. All the water samples of the study zone are not recommended for human consumption based on the bacteriological species. The presence of these pollution indicator parameters in the groundwater of the city of N'Djamena during the two sampling seasons indicates pollution linked to the lack of sanitation facility in the city. Sewerage systems, septic tanks, solid waste, inadequacy of sanitation facilities and the poor waste management could be the various sources of groundwater pollution in the study area as illustrated in Fig. 7 and Table 1 (samples site description). From a health point of view, there are many water-related diseases such as typhoid, amoebic dysentery, cholera, which sometimes leads to epidemics, and other gastroenteritis. These results for parameters such as E. Coli, Total Coliform are significantly lower than those obtained by Akintayo Ojo et al., (2021) in the Ilaro sedimentary environment of southwestern Nigeria.
Water quality index (WQI) results
The WQI for the 22 groundwater samples in the study zone varied from 188.41 to 866.89, with a mean value of 456.75 ± 187.19 (Table 6, Fig. 8). These results correspond to three types of water: “poor”, “very poor” and “unsuitable” for drinking. Those types represent respectively 9.09, 18 and 72% of the total sampled water. The spatial distribution of the WQI (Table 6 and Fig. 8) reveals that, the higher values (> 300) are observed in B3, B4, B5, B6, B7, B8, B9, B10, B11, B12, B14, B16, B17, B18, B19, B21 and B22. The poor quality of these water resources based on the WQI is due to the presence of bacteria species (E. Coli, Fecal coliform, Total Coliform, Fecal enterococci and total mesophyll aerobic flora) in that water. This clearly illustrates the inadequacy of the sanitation system in the locality (Fig. 7), which is common to all countries in the south of Sahara region. According to Frsat et al. (2023), untreated domestic and industrial wastewater caused groundwater pollution, which was the main reason for a decrease in the water quality. The high population requires the city to be developed continuously, while a plan should be established to control the spread and hazards of pollution. Indeed, the majority of boreholes in the Sudano-Sahelian zone draw water from the ancient Quaternary sandy-clay formations, which are generally shallow aquifers or semi-captive below a few meters of Upper Holocene clay levels. Compared to the previous work, the results of the present study are similar to those obtained by Gad and El Osta (2020), in the El Fayoum Depression (Egypt) where the drinking water quality index (DWQI) results revealed that 58% of the groundwater samples are very poor quality, while 42% of samples are unsuitable for drinking uses. On the other hand, WQI in this study differs from those obtained by Aouiti et al., (2020) in the sedimentary zone of Tunisia, Ngo Billong et al. (2023) in the Littoral region of Cameroon, precisely in the Ngoua watershed (Douala). This difference is related to the fact that the latter did not include bacteriological parameters in the water quality assessment. Their results show four water classes. These are the group of excellent (29%) and good (57%) water for depths of less than 100 m and medium (7%) and poor (7%) waters in depths greater than 100 m. Yenugu et al., (2021) in the same sedimentary environments presents three groups of water, the poor, good and excellent water with a very strong dominance of good quality water. The results of the present study are closer to those obtained by Prusty and Farook (2020). The latter obtained five classes of water, ranging between unsuitable to excellent water quality for drinking. Table 7 summarizes the work carried out to assess the water quality based on WQI. While in this study it is the bacteriological parameters that are responsible for the degradation of water quality, is rather the high mineralization that reduces the quality of the water in this coastal area. Based on the previous comparison (Table 7), it is finally concluded that the variations in the WQI of groundwater are not uniform in anywhere, depending upon the parameters used. Depending upon the socioeconomic developmental activities of the society, the researchers will take up the research work on various natural resources from time to time to suggest appropriate strategic management measures for socioeconomic improvement of common habitat from the adverse impacts. Also, assessment of water quality based on WQIs must take into account the local realities of each study area, the habits of the populations and their environment in terms of hygiene and sanitation. Groundwater in sub-Saharan Africa is generally poorly mineralized. It is therefore the biological parameters identified in groundwater in N’Djamena that are the cause of frequent waterborne diseases (Typhoid, malaria…), resulting in serious hygiene and sanitation problems in this part of the world. It is important to remember that although the assessment of water quality for drinking purpose using the WQI presents some imperfections due to the relativity of the weights attributed to each parameters used in the technique, this method remains the least criticized and produces satisfactory results.
Mineralization source of groundwater of the study area
In this study, three main methods were used to asses the factors controlling groundwater mineralization (Ramadhani Mussa et al., 2023) standard hydrochemical method through the saturation index calculation, the Gibbs model (Ramadhani Mussa et al., 2023) and the multivariate statistical analysis technic (correlation matrix, hirarchical clustering analysis).
Saturation indexes
The results on the Saturation Index (SI) calculation are summarized in Table 8. All the value are negative, indicating that all the water samples are under saturated with regard to minerals such as anhydride (CaSO4), aragonite (CaCO3), Calcite (CaCO3), dolomite (CaMg(CO3)2), gypsum (CaSO4.H2O), halite (NaCl), which characterise the sedimentary rock. According to Singh et al., (2017), this under saturation is linked to 1) the dissolution of these minerals, 2) a slow solubility of certains minerals in water (Silicate) and 3) the instability at ambient temperature and pressure of other minerals such as calcite (Singh et al., 2017; Yang et al., 2017). This last interpretation is in accordance with those of Paternoster et al., (2016) who establish the relationships between SI values and depth, They conclude that SI values are stratified with depth, moving from epilimnion to hypolimnion layer.
Gibbs model
According to the Gibbs model (Fig. 9), the entire sample falls under the water–rock interaction domain, characterised by low ratios of Na/(Na + Ca) and Cl/(Cl + HCO3) and moderate concentrations of TDS. It represents the rock weathering as the dominant geochemical factor regulating the ionic chemistry. The fact that all these samples belong to a single domain would explain the continuous and homogenuous character of aquifer systems in the sedimentary zone in general and in the Lake Chad basin in particular on the one hand and the contribution of semi-arid climate source on the groundwater system on the other hand (Subba Rao et al., 2021a, 2021b). The latter results are in accordance with the water type (Ca–Mg–HCO3). These results are similar to those obtained by Song et al., (2023) on a regional scale (transboundary quaternary aquifer of the Lake Chad Basin). These authors show that groundwater quality in the sahelian region are mainly inflenced by water–rock interaction, followed by the anthropogenic activities.
It should also be noted that, in addition to the saturation index and the gibbs model, these authors used several other methods to understand the water mineralisation processs. These include binary diagrams such as Ca2+/Na+ vs HCO−3/Na+, Ca2+/Na + vs Mg2+/Na+, Na+ vs C1−, (Ca2+ + Mg2+) vs HCO−3, Ca2+ + Mg2+)-(HCO−3 + SO2−4) vs (Na+ + K+-C1−), and Ca2+vs SO2−4 (Nagaraju et al., 2018; Hussin et al., 2016 and Mukate et al., 2020) and multivariate statistical analysis. The results of the latter method are presented below.
Soft computing methods for groundwater quality classification in N’Djamena
Correlation matrix
The correlation matrix (Table 9) reveal the weak, (r < 0.4), acceptable (0.5 ≤ r ≤ 0.7), very good (0.7 < r < 0.9) correlations between the hydrochemical and bacteriological parameters analysed. These correlations are positive for some and negative for others. Very good, good and average correlations have been noted between the following pairs of parameters: Total Coliform vs Fecal Coliform (r = 0.94), Total coliform vs Escherichia Coli (r = 0.63), Fecal coliform vs Eshcerichia coli (r = 0.68) for bacteriological parameters. As for the physico-chemical species, the good positive relation between HCO3− and Mg2+ (r = 0.72), HCO3− and K+ (0.86), HCO3− and TZ− (r = 1), NO3− and Ca2+ (0.60), Cl− and Ca2+ (r = 0.62), Na+ and Cl− (r = 0.56) have been identified. The good positive relation exist likewise between physico-chemical parameters and baceriological species such as fecal enterococci and Cl− (r = 0.75), fecal enterococci and NO3−; fecal enterococci and Ca2+ (r = 0.78). This explains the same origin of these parameters (anthropogenic factor). Meanwhile, there are low correlation between the bacteriological species such as fecal enterococci and MAF (r = 0.187), E. Coli and MAF (r = 0.371), Total coliform and and MAF (r = 0.047). According to American Ground Water Trust (2002); Tontsa et al. (2023), the bacteriological contamination of the water resource is not only faecal. Indeed, non-faecal coliform bacteria are very common and can be found almost everywhere on soil particles, insects, plants, animals, walls and furniture at the home.
Q-mode hierarchical clustering
The hierarchical clustering analysis results with physico-chemical and bacteriological parameters as variables (Fig. 10a) present two principles clusters such as cluster A and cluster B; the latter being divided once into two sub-clusters: sub-cluster B1 and B2. Cluster A groups together all parameters that have almost a common origin, namely anthropogenic origin (majority) or not. These are Fecal Coliform (FC), Escherichia Coli (EC), Total coliform (TC), Mesophyll Aerobic Flora (MAF) NH+4 and F−. Cluster B hyghlights all the physico-chemical parameters that may have one or more origins such as geogenic (some cations and anions) or antrhopogenic ((NO3−, SO4−, Na+). These results based on HCA are in accordance with those obtained by Rakotondrabe et al. (2018) who use the same technique to identify three main phenomena which control the water quality in Mari catchment (East-Cameroon) such as hydrolysis of silicate minerals, vegetation and soil leaching; and mining activities. With regard to the hierarchical clustering analysis results highlighting the sampling points as variables (Fig. 10b), two major clusters are identified such as: Cluster A and cluster B. The first cluster includes the following boreholes: B2, B4, B5, B6, B8, B9, B2, B11, B12, B13, B14, B15, B18 and B19 while the second which is subdivided into two sub-clusters (B1 and B2) contains only 08 boreholes wich are distributed as follows: four boreholes (B3, B16, B17 and B21) for sub-cluster B-1 and four other for B-2 (B1, B7, B10 and B20). This large grouping of boreholes in a single cluster reflects the continuity, homogeneity and the broad geometric extension of the aquifers system in sedimentary environment. It should be noted that most of these boreholes, if they are not in contact with the archaic sanitation structures such as septic tanks, they are in permanent contact with the surface water reservoir which is essentially the Logone and Chari river. Based on Barrie et al. (2023) the HCA based on sampling point showed a clear distinction between the location of the point in the Rokel river in Sierra Leone and their closest distance. They also observed that the dendrogram is clustered seasonally, that is formed during the wet months and dry months respectively. All the results of this study relating to the phenomenon and/or mechanisms controlling water quality (geogenic and anthropogenic factors) in the study area are summarized as the conceptual model illustrated in Fig. 11. These results are similar to those obtained by Song et al. (2023). Based on hydrochemical aspect in the transboundary quaternary aquifer of the Lake Chad Basin scale; these authors confirm that groundwater chemistry indicates geogenic influences in addition to a strong anthropogenic fingerprint. The conclude that, the intermediate to deep aquifer shows a longer residence time of groundwater, less connection with the surface and no to only a little anthropogenic influence. This last result explains the absence of biological species in some borehole in N’Djamena locality.
Groundwater suitability for irrigation
Sodium absorption ratio (SAR)
According to the USSL Diagrams obtained by plotting the SAR value against electrical conductivity (Richards, 1954). The twenty-two samples of the study area are situated in two domains (Fig. 12), the C1S1 which corresponds to the low sodium and low salinity, and C2S1 domains which characterize the low sodium and medium salinity water. Those results imply that the groundwater of the study milieu is classified as suitable and moderate water quality for agricultural purpose (Ramesh & Elango, 2012; Rakotondrabe et al., 2017; Sanjoy Shil et al., 2019). According to Tamru and Gopalakrishnan (2021), Venkatesan et al., (2021), the use of groundwater with high content of sodium for irrigation purpose could increase the exchange of sodium content of the soil and thus can decrease the soil permeability and soil structure.
Sodium percent
The Wilcox diagram (Fig. 13) represents the Sodium percent (%Na) against the electrical conductivity. It reveals that the groundwater samples of the study zone are excellent for agricultural purpose with values ranging between 7.80 to 47%.
Permeability index
The permeability index (PI) value ranged from 23.99 to 72.72, with a mean of 42.20 ± 12 (Fig. 14, Table 6). According to Das and Nag (20,015); Sanjoy Shil et al. (2019), 21 samples (95%) belonging to class III are considered as moderately suitable for irrigation, while only one sample correspond to class II and is unsuitable. The strong predominance of class III, as well as the high concentration of samples for %Na, in the excellent range reflects also the geological homogeneity of the Lake Chad basin as described by Djoret and Favreau (2014).
Magnesium index
The groundwater of the South West of N’Djamena has a magnesium ratio in the range of 3.20 to 113.28 for a mean of 40.42 ± 29.97 (Fig. 15, Table 6); two types of water can be distinguished as follows:
-
Suitable water for irrigation with magnesium percentage less than 50. This represents about 59% of the samples including B5, B6, B7, B8, B9, B11, B12, B13, B14, B15, B17, B21, B22;
-
Harmful and unsuitable quality for agricultural purposes for the remaining 49% (B1, B2, B3, B4, B10, B16, B18, B19, B20).
Based on the four parameters (SAR, %Na, Mg%, and PI) used in this study to express the aptitude of groundwater for irrigation, sample B10 is the only one which presents doubtful qualities for irrigation expressed by Mg% and PI consecutively. Based on these previous results, the groundwater in the N’Djamena’s locality are relatively good for irrigation. It can therefore boost the socio-economic development of the locality in general and the agricultural sector in particular.
Socio-economic impact of the groundwater quality on the population of the study area and water quality management measure
Water for human consumption requires well-defined quality standards. The universal standard is prescribed by the World Health Organization, which may be slightly modified in certain countries or regions according to local realities. When the concentration of a physicochemical (NO3−, F−…) or microbiological parameters is abnormally represented in water, the water becomes a source of disease (Subba Rao et al., 2021b). The abnormally high levels of microbiological parameters in the groundwater of N'Djamena are due to the fact that this city and its surroundings do not have a functioning public sewer system; so water from toilets is directed to traditional latrines which, due to economic reasons, are often constructed without any proper casing to impede the contamination of groundwater with microbial parameters. This contamination and poor hygiene practices of the population cause serious diseases like diarrhea, typhoid, cholera and hepatitis A and E. Statistics from Seeber et al., (2014) reveal that 16.7% of the deaths were linked to the water, sewage and hygiene sector. Furthermore, greywater from households is often directly poured into little trenches or fosses, which are located close to properties. This untreated greywater infiltrates, evaporates or accumulates in ponds of different sizes, detectable all around the city.
This study has shown that groundwater quality in N’Djamena and its surroundings is not safe for drinking because it can cause several diseases and other gastrointestinal disorders. The following management measures are proposed to help preserve the water quality status: in view of the quality indices coupled with the environmental characteristics described in the methodology section (Table 1), these water should be subject to regular quality control and microbiological treatment, although they are collected at relatively high depths. However, it can be used for other household tasks such as washing vegetables and cooking food. For consumption, however, they require treatment ranging from simple heating to special treatment. Adequate sanitary systems such as landfills should be provided in order to efficiently and effectively manage the high volumes of agricultural and domestic wastes generated in the area. Beyong the socio-economic interest of the present study, scientifically, they provide better understanding of the hydro-geochemical functioning of aquifer system in the Lac Chad Basin as presented in the conceptual model. This model is the result of a method that is not conventional enough.
Conclusion
The main groundwater resource in the Lake Chad basin in general and N’Djamena (Chad) in particular is a Quaternary aquifer. It is heavily exploited and vulnerable, due to the considerable urbanization of the town. The main objective of the study was to examine the groundwater quality for domestic and agricultural purposes, using hydro-chemical modeling, Water Quality Index (WQI) and Geographical Information System (GIS) Technics. Based on the results obtained, groundwater of N’Djamena has pH which tends to neutrality (6.40 < pH < 7.57). They are very weakly to weakly mineralized with one water type only (Ca–Mg–HCO3). The quality of groundwater in N’Djamena and it surrounding is not uniformly distributed, which suggests a high spatial variability due to the involvement of different sources and/or geochemical processes, taking place in the aquifer system. The concentration of the physicochemical parameters (cations and anions) does not exceed those prescribed by the World Health Organization for human consumption. The decreasing order of magnitude of the cations is as: Ca2+ ˃ Mg2+ ˃ Na+ ˃ K+, while that of anions is HCO3− ˃ Cl− ˃ SO42− ˃ NO3−. The WQI based on physicochemical parameters coupled to bacteriological species varied from 188.41 to 866.89, corresponding to poor, very poor and unsuitable for drinking; it means that the poor quality is due to the presence of bacteriological species. However, it should therefore be noted that groundwater in N’Djamena and Hadjer-Lamis should be purified before being used for drinking purposes. The Gibbs model, and the Q-mode hierarchical clustering indicate that the groundwater quality in the study zone is controlled by two main factors such as water–rock interaction (leaching, dissolution, and ions exchange) and anthropogenic activities. All the samples are under saturated (SI < 0) with regard to anhydride, aragonite, Calcite, dolomite, gypsum, halite, which characterize the sedimentary rock.
Based on the agricultural aspect, the SAR and %Na value of all the samples show that, the groundwater of the study zone has moderate and excellent quality for irrigation, while the MI and PI value of some samples are harmful and unsuitable.
The results presented in this study, particularly the bacteriological analysis provide a baseline reference on the future monitoring of the groundwater in N’Djamena and it surroundings; as suggested strategies of water pollution prevention, the implemented and continuous measure for proper management in the study area is necessary. The local authorities could implement the strategy to limit groundwater contamination with the population collaboration. The great measure could be taken to protect the Quaternary aquifer by constructing the proximate and the near feed areas around the boreholes in the study area.
For the future, more models for hydro-geochemical analysis and isotopic studies can further extend this work. In the same time, the hydrogeological potential coupled to the hydrodynamic characteristics of the aquifer system of the studied zone could be assessed. These further studies will provide a better understanding of this aquifer. This will optimize and rationalize the exploitation of the quaternary aquifer of the Lac Chad basin in general and N’Djamena in particular.
Data availability
All necessary data are included in the article.
References
Abderamane, H. (2012). Étude du fonctionnement hydrogéochimique du système aquifère du Chari Baguirmi (République du Tchad). Doctorate Dissertation. University of Poitiers UFR des sciences fondamentales et appliquées. 319 p.
Abderamane, H., Moussa, I., Abderaman, M., Brahim, H., Moumtaz, R. (2013). Le système aquifère de Chari Baguirmi (République du Tchad). Caractérisation hydrochimique du système en vue de sa gestion durable. Revue Scientifique du Tchad-décembre 2013 ISSN 1017–2769. 9 p.
Abderamane, H., Kengni, L., Iddo, E. S., Njueya Kopa, A. (2017). Assessment of the vulnerability of groundwater to pollution in N’Djamena (Chad) using DRASTIC index method. International Journal of Current Resources. 9(10) : 58911–58919. http://www.journalcra.com.
Abugu, H. O., Egwuonwu, P. F., Ihedioha, J. N., Ekere, N. R. (2021). Hydrochemical evaluation of river Ajali water for irrigational application in agricultural farmland. Applied Water Science. 11:71: https://doi.org/10.1007/s13201-021-01395-4.
Ahamed, A. J., Loganathan, K., & Jayakumar, R. (2015). Hydrochemical characteristics and quality assessment of groundwater in Amaravathi river basin of Karur district: Tamil Nadu South India. Sustainable Water Resourse Management, 1, 273–291.
Akintayo Ojo, O., Opeyemi Ogunyinka, O., Oluwatoyin Daramola, O., & Olaoye, F. F. A. (2021). Hydro-chemical and microbial assessments of water resources around cassava mills in a sedimentary formation of Ilaro. Southwest Nigeria. Applied Water Science, 11, 62. https://doi.org/10.1007/s13201-021-01384-7
Al Haj, R., Merheb, M., Halwani, J., & Ouddane, B. (2022). Hydrogeochemical characteristics of groundwater in the Mediterranean region: A meta-analysis. Physics and Chemistry of the Earth, 129, 103351. https://doi.org/10.1016/j.pce.2022.103351
Alhassan, I. H., Muntasir Shareef, A., Ghanim, H., & Farid Alatar, M. (2023). Hydrochemistry and water quality of shallow groundwater in the Tikrit area of Salah Al Din Province. Iraq. Applied Water Science, 13, 197. https://doi.org/10.1007/s13201-023-02008-y
American Ground Water Trust. (2002). Coliform and E. coli bacteria, vol 2. The American well owner
Aouiti, S., Hamzaoui, A. F., El Melki, F., Hamdi, M., Celico, F., & Zammouri, M. (2020). Groundwater quality assessment for different uses using various water quality indices in semi-arid region of central Tunisia. Environmental Science and Pollution Research. https://doi.org/10.1007/s11356-020-11149-5
APHA. (2012). Standard methods for the examination of water and wastewater (22nd ed.). American Public Health Association.
Ayed, B., Jmal, I., Sahal, S., Mokadem, N., Saidi, S., Boughariou, E., & Bouri, S. (2017). Hydrochemical characterization of groundwater using multivariate statistical analysis: The Maritime Djeffara shallow aquifer (Southeastern Tunisia). Environmental Earth Science, 76, 821. https://doi.org/10.1007/s12665-017-7168-6
Ayşen Davraz and Burcu Batur. (2021). Hydrogeochemistry characteristics of groundwater and health risk assessment in Yalvaç-Gelendost basin (Turkey). Applied Water Science, 11, 67. https://doi.org/10.1007/s13201-021-01401-9
Barrie, A., Agodzo, S. K., Frazer, W. R., Awuah, E., & Bessah, E. (2023). A multivariate statistical approach and water quality index for water quality assessment for the Rokel river in Sierra Leone. Heliyon, 9, e16196. https://doi.org/10.1016/j.heliyon.2023.e16196
Bekele, Y., Kebede, B., & Kuma, T. (2023). Assessing the role of community participation in integrated watershed management in Dandi Lake watershed Dandi district, West Showa, Oromia. Ethiopia. Applied Water Science, 13, 207. https://doi.org/10.1007/s13201-023-02009-x
Bon, A. F., Abderamane, H., Ewodo Mboudou, G., Aoudou Doua, S., Asone Banakeng, L., Bontsong Boyomo, S. B., Piih, S. L., & Wangbara Damo, B. (2020). Parametrization of groundwater quality of the Quaternary aquifer in N’Djamena (Chad), Lake Chad Basin: Application of numerical and multivariate analyses. Environmental Science and Pollution Research. https://doi.org/10.1007/s11356-020-10622-5
Cabalar, A. F., Karabas, B., Mahmutluoglu, B., & Yildiz, O. (2021). An IDW-based GIS application for assessment of geotechnical characterization in Erzincan Turkey. Arabian Journal of Geoscience, 14, 2129. https://doi.org/10.1007/s12517-021-08481-6
Chakravarty, T., & Gupta, S. (2021). Assessment of water quality of a hilly river of south Assam, northeast India using water quality index and multivariate statistical analysis. Environmental Challenge, 5, 100392. https://doi.org/10.1016/j.envc.2021.100392
Chimankpam Ezugwu, K., Obialo Onwuka, S., Johnbosco Egbueri, C., Chinanu Unigwe, O., & Daniel Ayejoto, A. (2019). Multi-criteria approach to water quality and health risk assessments in a rural agricultural province, southeast Nigeria. HydroResearch, 2, 40–48. https://doi.org/10.1016/j.hydres.2019.11.005
Das, S., & Nag, S. K. (2015). Deciphering groundwater quality for irrigation and domestic purposes: A case study in Suri I and II blocks: Birbhum District, West Bengal India. Journal of Earth System Sciences, 124(5), 965–992.
Diani, K., Amar, H., Azmi, R., Elbelrhiti, H., Nouayti, N., & Najlaa, F. (2021). Surface water quality assessment in the semi-arid area by a combination of heavy metal pollution indices and statistical approaches for sustainable management. Environmental Challenge, 5, 100230. https://doi.org/10.1016/j.envc.2021.100230
Djaouda, M., Njiné, T., Liang, S., Menye, D. E., Gaké, B., Zébazé Togouet, S. H., & Nola, M. (2014). Bacteriological quality of well waters in Garoua, North Cameroon. Water Quality Exposure Health, 6, 161–176. https://doi.org/10.1007/s12403-014-0120-z
Djoret, D. (2000). Etude de la recharge de la nappe du Chari Baguirmi par les méthodes chimiques et isotopiques. Doctorate Dissertation. Université d’Avignon et des Pays de Vaucluse. 146 p.
Djoret, D. and Favreau, G. (2014). Ressources en eau souterraine et relations avec le Lac”. In Lemoalle J. Magrin G. (dir.): Le développement du lac Tchad : situation actuelle et futurs possibles. Marseille. IRD Editions. Collection Expertise collégiale, 59–78.
Johnbosco Egbueri and Johnson Agbasi C. (2022). Data-driven soft computing modelingof groundwaterquality parameters in southeastNigeria: comparing the performances of different lagoritms https://doi.org/10.1007/s11356-022-18520-8
Elsayed Gabr, M., & Soussa, H. (2023). Assessing surface water uses by water quality index: Application of Qalyubia Governorate, Southeast Nile Delta. Egypt. Applied Water Science, 13, 181. https://doi.org/10.1007/s13201-023-01994-3
Fahmida, P., Morshedul, M. H., & Shafi, T. M. (2021). Recent status of water quality. Environmental Challange. https://doi.org/10.1016/j.envc.2021.100416
Frsat, A. A., Kaywan, O. A., Ata, A., Mehdi Karami, M., & Hüseyin, G. (2023). Spatio-temporal variations of groundwater quality index using geostatistical methods and GIS. Applied Water Science, 13, 206. https://doi.org/10.1007/s13201-023-02010-4
Gad, M., & El Osta, M. (2020). Geochemical controlling mechanisms and quality of the groundwater resources in El Fayoum Depression. Egypt. Arabian Journal of Geosciences, 13, 861. https://doi.org/10.1007/s12517-020-05882-x
Getachew, A., Tadie, A., Chercos, D. H., Guadu, T., Alemayehu, M., Gizaw, Z. G., Hiwot, M. G., & Cherkos, T. (2021). Bacteriological quality of household drinking water in North Gondar Zone Ethiopia: A community-based cross-sectional study. Applied Water Science, 11, 189. https://doi.org/10.1007/s13201-021-01515-0
Ghouili, N., Hamzaoui-Azaza, F., Zammouri, M., Zaghrarni, M. F., Jarraya, F. H., & Condesso de Melo, M. T. (2018). Groundwater quality assessment of the Takelsa phreatic aquifer (Northeastern Tunisia) using geochemical and statistical methods: Implications for aquifer management and end-users. Environmental Science and Pollution Research. https://doi.org/10.1007/s11356-018-3473-1
Güler, C., Thyne, G. D., McCray, J. E., & Turner, K. A. (2002). Evaluation of graphical and multivariate statistical methods for classification of water chemistry data. Hydrogeological Journal, 10, 455–474.
Hussin, N. H., Yusoff, I., Tahir, W., Mohamed, I., Ibrahim, A. I. N., & Rambli, A. (2016). Multivariate statistical analysis for identifying water quality and hydrogeochemical evolution of shallow groundwater in Quaternary deposits in the Lower Kelantan River Basin. Malaysian Peninsula. Environmental Earth Science, 75, 1081. https://doi.org/10.1007/s12665-016-5705-3
Jiang, Y., Guo, H., Jia, Y., Cao, Y., & Hu, C. (2015). Principal component analysis and hierarchical cluster analyses of arsenic groundwater geochemistry in the Hetao basin, Inner Mongolia. Geochemistry, 75, 197–205. https://doi.org/10.1016/j.chemer.2014.12.002
Kouassy Kalédjé, P. S., Mfonka, Z., Bouelet Ntsama, I. S., Kpoumié, A., Fouépé Takounjou, A. L., & Ndam Ngoupayou, J. R. (2023). Groundwater quality assessment in the catchment area of Kadey (East-Cameroon): Water quality index approach. Sustainable Water Resource Management, 9, 137. https://doi.org/10.1007/s40899-023-00916-0
United Nation, (2018). La convention sur l’eau : répondre aux défis mondiaux de l’eau. Pp 19 e-ISBN:978–92–1–363105–8
Lemoalle, J., Magrin, G. (2014). Le développement du lac Tchad: situation actuelle et futurs possibles. Marseille, IRD Editions, coll. Expertise collégiale, 21p + clé USB.
Li, P., He, X., Li, Y., & Xiang, G. (2019). Occurrence and health implication of fluoride in groundwater of loess aquifer in the Chinese Loess Plateau: A case study of Tongchuan. Northwest China. Exposure and Health, 11(2), 95–107. https://doi.org/10.1007/s12403-018-0278-x
Louis, P. (1970). Contribution de la géophysique à la connaissance géologique du bassin du Lac Tchad. Mémoire ORSTOM n°42. p. 303.
Mahamat Nour, A., Vallet-Coulomb, C., Bouchez, C., Ginot, P. J., Doumnang, C., Sylvestre, F., & Deschamps, P. (2019). Geochemistry of the lake chad tributaries under strongly varying hydro-climatic conditions. Aquatic Geochemistry. https://doi.org/10.1007/s10498-019-09363-w
Mbadngonel Morbe, C. (2018). Ressource en eau dans le centre urbain de N’Djamena (Tchad) : qualité et vulnérabilité. Master degree dissertation. University of Yaoundé 1, Cameroon. pp70.
Merchán, D., Auqué, L. F., Acero, P., Gimeno, M. J., & Causapé, J. (2017). Geochemical processes controlling water salinization in an irrigated basin in Spain: Identification of natural and anthropogenic influence. Science of the Total Environment, 502, 330–343. https://doi.org/10.1016/j.scitotenv.2014.09.041
Mfonka, Z. (2019). Hydrodynamique et vulnérabilité des nappes en zone de socle fissuré et al téré: Cas de la localité de Foumban dans le bassin versant du Nchi (Ouest-Cameroun). PhD Dissertation, University of Yaoundé 1, Yaoundé, 289.
Mfonka, Z., Kpoumié, A., Ngouh, A. N., Mouncherou, O. F., Nsangou, D., Rakotondrabe, F., Fouépé Takounjou, A., Zammouri, M., Ndam Ngoupayou, J. R., Ndjigui, P. D. (2021). Water Quality Assessment in the Bamoun Plateau, Western-Cameroon: Hydrogeochemical Modelling and Multivariate Statistical Analysis Approach. Journal of Water Resource and Protection. https://doi.org/10.4236/jwarp.2021.132007.
Mfonka, Z., Ndam Ngoupayou, J. R., Ndjigui, P. D., Zammouri, M., Kpoumie, A., & Rasolonana, E. (2015). Hydrochimie et potabilité des eaux du bassin versant du Nchi dans le plateau Bamoun (Ouest Cameroun). International Journal of Biological and Chemical Sciences, 9, 2000–2018. https://doi.org/10.4314/ijbcs.v9i4.39
MINEPAT, (2021). National Development Strategy 2020–2030 for structural transformation and inclusive development. Cameroon National report. pp 207.
Mukate, S. V., Panaskar, D. B., Wagh, V. M., & Baker, S. J. (2020). Understanding the influence of industrial and agricultural land uses on groundwater quality in semiarid region of Solapur, India. Environmental Development and Sustainability, 22, 3207–3238. https://doi.org/10.1007/s10668-019-00342-3
Nagaraju, A., Balaji, E., Sun, L. H., & Thejaswi, A. (2018). Processes controlling groundwaterchemistry from Mulakalacheruvu area, Chittor district, Andhra Pradesh, South India: A statistical approach based on hydrochemistry. Journal of Geological Society of India, 91, 425–430. https://doi.org/10.1007/s12594-018-0875-0
Ngo Billong, P. T., Feumba, R., & Ndjigui, P. D. (2023). Hydrogeochemical appraisal of groundwater quality in Ngoua watershed (Douala-Cameroon): Implication for domestic purposes. Scientific African, 22, e01910. https://doi.org/10.1016/j.sciaf.2023.e01910
Ngounou Ngatcha, B. (2009). Water Resources Protection in the Lake Chad Basin in the Changing Environment. European Water 25/26: 3:12
Obiri, S., Addico, G., Mohammed, S., Anku, W. W., Darko, H., & Collins, O. (2021). Water quality assessment of the Tano Basin in Ghana: A multivariate statistical approach. Applied Water Science, 11, 49. https://doi.org/10.1007/s13201-021-01374-9
Ombolo, A., Ambomo Bineli, E., Mboudou, E. G., Madi, A., Dassou, F. E., & Samba, P. A. (2016). The physicochemical seasonal effect on the quality of well drinking water in Lake Chad Basin: Case study Mayo Tsanaga (Far North region of Cameroun). Journal of Environmental Science, 5(10), 51–60.
Paternoster, M., Mongelli, G., Caracausi, A., & Favara, R. (2016). Depth influence on the distribution of chemical elements and saturation index of mineral phases in twins maar lakes: The case of the Monticchio lakes (southern Italy). Journal of Geochemical Exploration, 163, 10–18. https://doi.org/10.1016/j.gexplo.2016.01.001
Prusty, P., & Farooq, S. H. (2020). Application of water quality index and multivariate statistical analysis for assessing coastal water quality. Environmental Processing, 7, 805–825. https://doi.org/10.1007/s40710-020-00453-4
Rakotondrabe, F., Ndam Ngoupayou, J. R., Mfonka, Z., Rasolomanana, E. H., Nyangono Abolo, A. J., & Ako Ako, A. (2018). Water quality assessment in the Bétaré-Oya gold mining area (East-Cameroon): Multivariate statistical analysis approach. Science of the Total Environment, 610(611), 831–844. https://doi.org/10.1016/j.scitotenv.2017.08.080
Rakotondrabe, F., Ndam Ngoupayou, J. R., Mfonka, Z., Rasolomanana, E. H., Nyangono Abolo, A. J., Banakeng, L. A., Ako Ako, A., & Rakotondrabe, M. H. (2017). Assessment of Surface Water Quality of Bétaré-Oya Gold Mining Area (East-Cameroon). Journal of Water Resource and Protection, 9, 960–984. https://doi.org/10.4236/jwarp.2017.98064
Ramadhani Mussa, K., & Chikira Mjemah, I. (2023). Using hydrogeochemical facies and signatures for groundwater characterization and evolution assessment in aquifers with contrasting climate and geology in Tanzania. Applied Water Science, 13, 201. https://doi.org/10.1007/s13201-023-01977-4
Ramesh, K., & Elango, L. (2012). Groundwater quality and its suitability for domestic and agricultural use in Tondiar river basin Tamil Nadu. India. Environmental Monitoring Assessment, 184, 3887–3899.
Richards, L. A. (1954). Diagnostics and Improvement of Saline and Alkali Soil (p. 160). U.S. Department of Agriculture.
Rodier, J., (2009). Analyse de l’eau. Dunod. 9ème Ed. Paris. pp. 1579.
Roudouane, H. T., Mbey, J. A., Bayiga, E. C., & Ndjigui, P. D. (2020). Characterization and application tests of kaolinite clays from Aboudeia (Southeastern Chad) in fired bricks making. Scientific African. https://doi.org/10.1016/j.sciaf.2020.e00294
Sana, A., Rida, F., Zamir, A., Soomro Munawar, H., Sajid Rashid, A., & Hafiza, S. R. (2019). Bacteriological quality assessment of water supply schemes (WSS) of Mianwali, Punjab. Pakistan. Environmental Earth Science, 78, 458. https://doi.org/10.1007/s12665-019-8455-1
Schneider, J. L., Wolff, J. P. (1992). Carte géologique et cartes hydrogéologiques à 1/1,500,000 de la République du Tchad : Mémoire explicatif. Document du BRGM N° 209, Vol. 2. Éditions du BRGM.
Schneider, J. L. (2001). Carte de valorisation des eaux souterraines A1: 1 500 000. Document DGDH. 706p.
Seben, D., Toebe, M., Wastowski, A. D., Hofstätter, K., Volpatto, F., Zanella, R., Prestes, O. D., & Golombieski, J. I. (2021). Environmental Challenges 5: 100266 Water quality variables and emerging environmental contaminant in water for human consumption in Rio Grande do Sul. Brazil. Environmental Challenge, 5, 100266. https://doi.org/10.1016/j.envc.2021.100266
Seeber, K., Wilczok, C., Djoret, D., Magaji, A. B. (2014). Microbial and chemical drinking water analysis in N’Djamena. Technical report
Seeyan, S. (2020). Hydrochemical assessment and groundwater quality of Koysinjaq area in Kurdistan Region-Iraq. Arabian Journal of Geoscience, 13(491), 491. https://doi.org/10.1007/s12517-020-05410-x
Şener, Ş, Şener, E., & Davraz, A. (2017). Evaluation of water quality using water quality index (WQI) method and GIS in Aksu River (SW-Turkey). Science of the Total Environment, 584(585), 131–144. https://doi.org/10.1016/j.scitotenv.2017.01.102
Shil, S., Umesh Kumar, S., & Pankaj, M. (2019). Water quality assessment of a tropical river using water quality index (WQI): Multivariate statistical techniques and GIS. Applied Water Science, 9, 168. https://doi.org/10.1007/s13201-019-1045-2
Singh, C. K., Kumar, A., Shashtri, S., Kumar, A., Kumar, P., & Mallick, J. (2017). Multivariate statistical analysis and geochemical modeling for geochemical assessment of groundwater of Delhi, India. Journal of Geochemical Exploration, 175, 59–71. https://doi.org/10.1016/j.gexplo.2017.01.001
Song, F.; Nlend, B. Boum-Nkot, S.N., Huneau, F., Ndondo, G.N.; Garel, E., Leydier, T., Celle, H., Djieugoue, B., Ntamak-Nida, M.-J., Jacques Etame. (2023). Groundwater Resources of the Transboundary Quaternary Aquifer of the Lake Chad Basin: Towards Better Management via Isotope Hydrology. Resources 12:138. https://doi.org/10.3390/resources12120138
Sorlini, S., Palazzini, D., Sieliechi, J. M., & Ngassoum, M. B. (2013). Assessment of physical-chemical drinking water quality in the logone valley (Chad-Cameroon). Sustainability, 5, 3060–3076. https://doi.org/10.3390/su5073060
Souabe, S. (2013). Evaluation d'impacts socioéconomiques de l'interdiction de l'utilisation des sachets plastiques « Leyda » dans la ville de N’Djamena. Memoire. Master Institut régional multisectoriel de technologie appliquée, de planification et d’évaluation de projets (Gabon), 100 p.
Subba Rao, N., Dinakar, A., & Karuna Kumari, B. (2021a). Appraisal of vulnerable zones of non-cancer-causing health risks associated with exposure of nitrate and fluoride in groundwater from a rural part of India. Environmental Resources, 202, 111674. https://doi.org/10.1016/j.envres.2021.111674
Subba Rao, N., Dinakar, A., Sravanthi, M., & Karuna Kumari, B. (2021b). Geochemical characteristics and quality of groundwater evaluation for drinking, irrigation, and industrial purposes from a part of hard rock aquifer of South India. Environmental Science and Pollution Resources. https://doi.org/10.1007/s11356-021-12404-z
Tamru, T. A., & Gopalakrishnan, G. (2021). Evaluation of groundwater quality for drinking and irrigation purposes using GIS-based water quality index in urban area of Abaya-Chemo sub-basin of Great Rift Valley. Ethiopia. Applied Water Science, 11, 148. https://doi.org/10.1007/s13201-021-01482-6
Tontsa, L., Temgoua, E., Bertrand, G., Kengni, L., Makaya Mvoubou, M. C., Njueya Kopa, A., & Mba, F. F. (2022). Environmental factors controlling contamination of alternative water supply points in the Lefock semi-urban watershed. Cameroon Western Highlands. Environmental Earth Science, 82, 17. https://doi.org/10.1007/s12665-022-10699-w
United Nations Development Programme (2002) Bilan diagnostic des ressources en eau: schéma directeur de l’eau et de l’assainissement du Tchad. Parti 1 document. 120 p.
United Nations. (2013). The Sahel: One Region, Many Crises. Africa Renewal. Available online: https://www.un.org/africarenewal/magazine/december-2013/sahel-one-region-many-crises. Accessed on 15 Aug 2023.
Vassolo, S. (2012). Groundwater need assessment Lake Chad Basin. Bundesanstalt für Geowissenschaften und Rohstoffe (BGR) Report. 26 pp.
Venkatesan, S., Arumugam, S., Bagyaraj, M., Preethi, T., & Parthasarathy, P. (2021). Spatial assessment of Groundwater Quantity and Quality: A case study in parts of Chidambaram Taluk, Cuddalore District, Tamil Nadu. India. Sustainable Water Resource Management., 7, 103. https://doi.org/10.1007/s40899-021-00585-x
Vetrimurugan, E., Brindha, K., Bongani, S., Elango, L. (2017). Spatial interpolation methods and geostatistics for mapping groundwater contamination in a coastal area. Environmental Science and Pollution Research. https://doi.org/10.1007/s11356-017-8681-6.
Wilcox, L. V. (1955). Classification and Use of Irrigation Waters. US Department of Agriculture. Washington DC (Circular No. 969).
World Health Organization. (2017). Guidelines for Drinking Water Quality (3rd ed.). World Health Organization.
Wu, J., Zhou, H., He, S., Zhang, Y. (2019). Comprehensive understanding of groundwater quality for domestic and agricultural purposes in terms of health risks in a coal mine area of the Ordos basin, north of the Chinese Loess Plateau. Environental Earth Sciences 78(15):446. https://doi.org/10.1007/s12665-019-8471-1
Yang, P., Cheng, Q., Xie, S., Wang, J., Chang, L., Yu, Q., Zhan, Z., & Chen, F. (2017). Hydrogeochemistry and geothermometry of deep thermal water in the carbonate formation in the main urban area of Chongqing, China. Journal of Hydrology, 549, 50–61. https://doi.org/10.1016/j.jhydrol.2017.03.054
Yenugu, S. R., Vangala, S., Badri, S. (2020). Monitoring of groundwater quality for drinking purposes using the WQI method and its health implications around inactive mines in Vemula‑Vempalli region. Kadapa District. South India. Applied Water Science 10:202. https://doi.org/10.1007/s13201-020-01284-2
Acknowledgements
The authors would like to thanks the Editor in Chief of International Journal of Energy and Water Resources, Professor H. A. HASSANI, The Managing Editor, Professor S. MIRKIA and the two anonymous reviewers for their valuable suggestions to improve the quality of this manuscript. Thanks are also extended to Professor NDJIGUI Paul-Désiré, the Head of Department of Earth Sciences, Faculty of Science, University of Yaoundé 1 (Cameroon) for their support.
Author information
Authors and Affiliations
Contributions
Material preparation, data collection, analysis, conception and design, first draft were performed by MZ. All authors commented on previous versions of the manuscript. All authors read and approved the final manuscript.
Corresponding author
Ethics declarations
Conflict of interest
The authors declare that they have no conflict of interest.
Rights and permissions
Springer Nature or its licensor (e.g. a society or other partner) holds exclusive rights to this article under a publishing agreement with the author(s) or other rightsholder(s); author self-archiving of the accepted manuscript version of this article is solely governed by the terms of such publishing agreement and applicable law.
About this article
Cite this article
Mfonka, Z., Morbe, C.M., Nsangou, D. et al. Groundwater quality assessment for drinking and agricultural purposes under arid climate in N’Djamena, Chad (Central Africa). Int J Energ Water Res (2024). https://doi.org/10.1007/s42108-024-00297-w
Received:
Accepted:
Published:
DOI: https://doi.org/10.1007/s42108-024-00297-w