Abstract
In this study, gelatin from camel bones (Camelus dromedarius) at three different ages (2.5, 4.5 and 7 years old) was extracted using heating and chemical pretreatment methods. The extraction process was conducted by using hydrochloric acid (0.5 M) (at room temperature 25°c for 72 h) to demineralize the bones and partially hydrolyze the collagen followed by neutralizing with 4% ammonium sulphate. Extraction of camel bone gelatin (CBG) was carried out with distilled water (1:3, w/v) at 75 °C for 3 h, followed by extraction temperature at 90 °C for 1 h resulting in a yield of dry bases ranging from 21.3 to 23.9%. Camel bone gelatin had high protein content (89.31–93.3%), optimum moisture (6.0%), and low ash content (3.62%). The rheological properties, ATR-FTIR and bloom gel, were also investigated. The gel strength (GS) of the camel bone gelatin ranged from 107 to 192 g. The gelatin produced showed good quality and did not differ from other commercial gelatins. The produced gelatin could be useful for food and pharmaceutical implementation.
Similar content being viewed by others
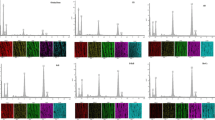
Explore related subjects
Discover the latest articles, news and stories from top researchers in related subjects.Avoid common mistakes on your manuscript.
Introduction
Gelatin is one of the most common biopolymers. It forms a thermo-reversible gel with a melting temperature similar to body temperature [1, 2]. The usage of gelatin in food industry could be utilized in confections for chewiness, texture, and foam stabilization, also it can provide creaminess, fat reduction, and mouthfeel in low-fat spread products, while in dairy it is used as stabilization and texturization agent, it is also used in baked goods as emulsification, gelling, and stabilization, and provide water-binding in meat [3, 4].
The collagen is difficult to dissolve in water, even at high temperatures. The principle of hydrolysis is to break down the triple helix of collagen while keeping the protein chain intact through a process known as partial hydrolysis [5]. To purify gelatin, the raw materials must undergo several treatments and procedures to produce a useful product. Partial alkaline hydrolysis pretreatment before the final extraction has been used on an industrial basis to classify gelatin into types A and B [6]. Gelatin is widely used as a gelling and thickening agent in food products [7, 8]. The gelatin yield and quality depend on the extraction conditions such as pretreatment, pH, temperature, and time [9]. However, the extraction conditions might alter the gelatin characteristics that depend on the length of polypeptide chains [10, 11].
Gelatin has a wide range of characteristics and functions due to its biological content based on different amino acids (AAs), especially proline and hydroxyproline [12]. These two dominant AAs are responsible for the stability of the collagen structure, melting and gelling temperatures, and gel strength (GS) of different types of gelatin [13]. Multiple gelatin sources have been found to have various physicochemical, functional, thermal, and rheological properties [14]. Thus, mammalian gelatin should be produced at high levels due to its industrial and health benefits.
Gelatin production (GP) worldwide was estimated at 326,000 tons, while European countries contributed around 121,800 tons. About 3–4% assessed the annual growth of gelatin production from 2000 to 2007. Gelatin is mainly produced from pig skin (46%), bovine hides (29.4%), bovine bones (23.1%), and other sources (1.5%) [15]. From religious and ethical perspectives, new gelatin sources must be identified because both Muslim and Judaism communities prohibit pig sources, and bovine products are prohibited from use by Hindus [16]. Another drawback of gelatin extracted from bovine sources is that they are usually costly. Therefore, producers usually choose porcine gelatin for commercial uses.
Currently, gelatin requirements are increasing exponentially due to its enormous usage in the food, pharmaceutical, and cosmetic industries. Thus, alternative gelatin resources greatly interest all communities [17]. In the last decade, researchers produced gelatin from different resources such as fish, bovine, and chicken [17, 18]. Fish gelatin (FG) is considered gelatin from a natural resource with increasing consideration as an alternative to porcine and bovine gelatin [15, 19]. Given the weak gel properties of FG, the extraction of gelatin from other sources such as camel byproducts is essential. For this reason, alternative gelatin sources such as camel gelatin from skin and bone tissues are under investigation. Therefore, gelatin extraction from camel bones is urgently needed for Muslim consumers because of relatively reduced fish gel properties compared with mammalian gels [20].
The worldwide camel population is ~ 35 million heads [21]. More than 85% are to be found in Africa and the Middle East. Camels are widely available in Saudi Arabia with estimates of 1.4 million heads [22]. A big number of camels are slaughtered each year around the world. The camel carcase makes 19% bones [23]. As a result, tons of hides, hooves, and bones are thrown away, causing environmental nuisance and health risks. Thus, utilization of the inedible camel portions to produce high-value products may minimize environmental nuisance. Interestingly, camel hides and bones are potential sources of gelatin. Recently, Al-Hassan demonstrated that gelatin could be extracted from camel skin through a simple process [9]. Al-Kahtani et al. reported that camel bone gelatin (CBG) structure changes after demineralization [24]. However, the utilization of a camel’s bones for gelatin production has not been fully explored. Gelatin from camel hides and bones may provide a new gelatin source that might be acceptable to people who avoid other gelatin sources (bovine and porcine) for religious or ethical reasons. Using byproducts from camels such as hides and bones can be beneficial in food, pharmaceutical, and other applications.
Ammonium sulfate was used as a neutralizing agent in the extraction process in this study. However, a reduction in gelatin strength can be observed with increases in the ammonium sulfate concentration. Nevertheless, the salt does not damage the gelatin functional groups, such as amide A, amide I, amide II, and amide III [19].
Thus, this study aimed to extract gelatin from camel bones (at different age range) and determine its physicochemical, thermal, and rheological properties. The structures of CBG were analyzed by attenuated total reflectance–Fourier transforms infrared (ATR-FTIR) spectroscopy.
Materials and methods
Materials
Fresh camel bones from healthy slaughtered camels aged 2.5, 4.5, and 7 years old were obtained from male camel legs. All bones were collected from the slaughtering house in Burydah City, Saudi Arabia. Food grade bovine gelatin (type A) was from Egypt (220 bloom/40 Mesh). Analytical grade FG (type B, 130 bloom), 36.5 g/mol hydrochloric acid (HCl), and ammonium sulfate were purchased from Panreac (EU).
Extraction of gelatin
Extraction was conducted by following the method of Ferraro et al. [25] with slight modifications. Bone samples (three samples at 100 g each) were defatted and cleaned of meat by heating in a water bath at 90 °C for 2 h, washed, and dried in a ventilated oven at 60 °C for 24 h. Dried bones were crushed into 3 × 3 cm particles before pretreatment. The bones were treated in hydrochloric acid (0.5 M) for 72 h at room temperature (25 °C) to demineralize them and partially hydrolyze collagen, followed by washing with cold water (< 20 °C) to remove the acid. The bones were then neutralized by ammonium sulfate (4% w/v). The extraction was carried out with distilled water (1:3 w/v) at 75 °C for 3 h and at 90 °C for 1 h. The prepared gelatin solution was filtered using cheesecloth, followed by centrifugation at 15,000 rpm at 30 °C for 5 min. The supernatant was dried in a ventilated oven at 60 °C for 24 h.
pH measurement, ash, and moisture
According to the standard British Institute [26] methods, the pH values of CBG were investigated by using a Hack pH meter (HQ411d, Spain). According to a previously reported method, the moisture and ash contents of the extracted gelatin powder were estimated (AOAC, 1999).
Protein content analysis
Protein content (PC) of CBG was determined by the Kjeldahl method (AOAC, 1999) with slight modifications. In short, approximately 2 g of CBG was accurately measured. The results were expressed using a nitrogen conversion component factor of 5.55 for gelatin (AOAC, 1984).
Amino acid analysis
The amino acid composition was analyzed by high-performance liquid chromatography (HPLC; Agilent HPLC1100 series, Agilent Technologies, USA) following White et al. [27]. The analysis was carried out with a Diode-Array Detector and a Pico-Tag-amino acid column with a gradient of Pico-Tag solvents A and B (Eluent A: 0.14 M sodium acetate, pH 6.40 containing 0.05% triethylamine mixed with acetonitrile [94:6 v:v]; Eluent B: [60:40] acetonitrile: water, v:v) at 38 ºC and a flow rate of 1 ml/min at 254 nm. The gelatin was hydrolyzed with 6 N HCl at 110 ºC for 24 h before analysis. Analyses were carried out in duplicate and results were expressed as percentage (w/w).
ATR-FTIR spectra analysis
The spectra of the camel gelatin powder samples were characterized by ATR-FTIR using an ALPHA-Eco FT-IR spectrometer with an Eco-Zn Se-sampling module (Bruker Optics, Germany). The spectra under the region of 4000–600 cm−1 were recorded. The obtained results were compared with the spectra of commercial bovine and FG.
Gel strength analysis
According to the United Kingdom specifications, the bloom value of CBG was analyzed (British Standards Institution, 1975). The gel solution was prepared by dissolving the gelatin powder in distilled water at 60 °C until a clear solution was obtained, cooled to 25 °C, and placed in bloom jars. A texture analyzer TAG XT2 (Stable Microsystems, Surrey, UK) was utilized to characterize the GS. The analyzer had a load cell of 5 kg and a cross-head rate of 1 mm/s with a toned lower part plunger (0.5 in dimension).
Rheological measurements
A controlled stress rheometer (Discovery hybrid rheometer (DHR-2) TA Instrument, USA) was used to characterize the rheological behavior of the gelatin solutions via cone-plate geometry (40 mm, angle = 2°, and gap = 52 μm). The viscoelastic properties of the gelatin solutions (6.67% w/v) were measured in the range of 5–35 °C (heating scan) and 35–5 °C (cooling scan) with a heating/cooling rate of 1 °C/min. The frequency of oscillation was 1 Hz, and the applied stress was 3 Pa. The elastic modulus (G'), viscous modulus (G"), and phase angle (tan δ) were recorded as a function of the temperature.
Statistical analyses
Each assay of CBG was conducted three times using the same extract. ANOVA (SPSS 18.0®) was performed to determine the significant differences among data at a confidence level of p ≤ 0.05.
Results and discussion
Gelatin extraction
The results of the experiments, including gelatin extraction from the bones, rheological and thermal characterization, and analysis of the physicochemical properties of the gelatin from the camel bones, are shown in Tables 1 and 2. Gelatin from camel bones at ages 2.5, 4.5, and 7 years was extracted in a short period because the gelatin extraction method was optimized using chemical and heat pre-treatments. The new procedure allowed for gelatin powder production in 4 days with a yield percentage of the three different ages of bones as 21.3% ± 1.7%, 23.9% ± 1.4%, and 22.4% ± 2.1%. This gelatin extraction method was shorter in time compared with gelatin extracted from bones of young and adult Nile perch (9–12 days) [28], collagen from bovine bones (6 days) [25], and camel bone (5 days) [24]. In this study, partial hydrolysis of collagen was applied using HCl, resulting in type A gelatin. Hydrolysis was performed by soaking the camel bones in 0.5 M HCl for 72 h. The ionic strength of the solution at acidic pH facilitated the swelling process. The gelatin yield is affected by different factors such as a source of gelatin, extraction method, acid concentration, temperature, pH, and time [29]. Earlier studies demonstrated that ammonium sulfate salt concentration plays a significant role in gelatin strength. Nevertheless, the salt will not damage its fingerprint when characterized via FTIR, which can clearly show functional groups, such as amide A, amide I, amide II, and amide III [19]. The finding of this study was in agreement with the work of Al-Kahtani et al. [24], who reported an extracted gelatin yield of 21.33% from camel bones. The effect of age on the yield of collagen from bovine bones resulted in a higher yield in young bones than in old bones from cows (10.6–16.9% and 7.9–9.6%, respectively) (Ferraro et al. 2017). High extraction temperatures reduced the gelatin yield from adult Nile perch bones by threefold when the temperature increased from 50 to 70 °C (33–9.6%) and onefold (33.3–22.6%) for young Nile perch bones (Muyonga et al. 2004). The variation in times (4 and 8 h) and different acid pretreatments on unicorn leatherjacket skins led to varying gelatin yields of 5.23–9.18% and 6.12–11.54% (wb) [15].
pH, moisture, and ash
The pH of gelatin showed no differences among all extracted gelatin samples (Table 1). The pH of the CBG obtained was between 5.62 ± 0.1 and 5.73 ± 0.1. The pH of gelatin was between 3.8 and 5.5 [30]. The pH level is thought to affect the functional properties of gelatin [31]. Thus, there are various gelatin pH levels. For example, shark gelatin has a pH of 4.34 [31]. Jamilah and Harvinder [32] reported slightly lower pH values of 3.05 and 3.91 for red and black tilapia skin gelatin, respectively. These results were slightly lower than the findings reported by Ratnasari et al. [29], who recorded a pH of 5.8 for catfish gelatin. Although there were no significant differences in pH among samples, there were significant differences observed in GS and MPs that were not related to pH.
The moisture content of the extracted CBG was between 4.5 ± 0.2% and 6.0 ± 0.3%. The standard requirement of the moisture content of gelatin is 8%–13% (GMIA., 2012). The moisture level of CBG indicated that it had slightly lower moisture than other types of gelatin. The ash content was higher in the gelatin obtained from camel bones than in the other gelatin samples (Table 3). The ash contents of CBG were 4.31 ± 1.1%, 3.62 ± 0.3%, and 6.23 ± 1.4% for CBG (2.5 Y), CBG (4.5 Y), and CBG (7 Y), respectively. Low inorganic matter in gelatin commonly refers to the absence of any salt content in the final gelatin extraction; this may have been generated during pretreatment and acid hydrolysis [15]. Gelatin extracted from bone usually has more ash compared to gelatin obtained from other raw materials such as skin. Zilhadiae at. [33] determined the proximate composition of goatskin gelatin and bovine skin gelatin and reported their ash contents (%) are 0.11 ± 0.02% and 0.39 ± 0.16%, respectively. The ash content of another type of gelatin from the skins of cobia (Rachycentron canadum) on a wet basis is 1.0 ± 0.2 g/100 g [34]. Ratnasari et al. [29] reported that the ash content of FG is only 0.377%. Jongjareonrak et al. [35] suggested that the raw material used for preparing gelatin is the main factor that determines good gelatin quality. It should contain high amounts of protein and low levels of moisture, ash, and fat. Some of the raw material contents, such as residual chemicals after processing or mixing with other ingredients, may affect the gelatin contents. However, the high ash contents found in gelatin from all different ages compared with other types of gelatin may be due to some camel particularities.
Protein content
The total protein obtained in CBG was in the range of 89.31 ± 2.6% and 93.3% ± 1.2%. The PCs of commercial bovine and commercial FG powders were 93.13 ± 0.6% and 87.22% ± 0.7%, respectively. The PCs of all samples were significantly higher (p < 0.05) than that of commercial FG. The PC is a significant component of CBG. In general, both acid and ammonium sulfate pretreatments resulted in high yields of protein. Therefore, the hydrolysis process and extraction techniques probably increased the PC during CBG extraction. However, the protein yield depends on the acid and alkaline pretreatment and their concentrations, time, and extraction process [36]. Gelatin properties such as GS, viscosity, gelling points, and MPs are more influenced by the extraction process than the PC and amino acid content. The presence of protein at the maximum yield of gelatin extraction, lipid, and ash content is necessary for adequate gelatin quality control [37].
Rheology and thermal analysis
The rheological properties of gelatin samples are shown in Table 2 and Figs. 1 and 2. The gelatin viscosity of all samples showed no significant difference (p > 0.05) among camel samples with the value of 20 mPa·s compared with commercial bovine gelatin (3.8 ± 0.1 mPa·s) and commercial FG (7.7 ± 0.1 mPa·s). The high viscosity of these samples may be due to impurities with higher ash contents compared with commercial gelatin. The higher the viscosity values, the higher the MPs and gelling points, and the stronger the gel [38]. Different viscosity values have been reported based on the source of gelatin and the extraction procedure involved. The viscosity values of bovine gelatin and porcine gelatin are usually 9.8 and 5 mPa·s, respectively [39]. However, the viscosity depends on the gelatin sources and the extraction of pretreatment and conditions.
Modulus storage (G') and loss modulus (G'') suggested that the extracted camel gelatin samples are flexible. Our findings showed that the MPs of the gels (6.67%) were 23.5 °C, 26.7 °C, and 24.3 °C with gelling points of 15.07 °C, 18.21 °C, and 18.12 °C for ages 2.5, 4.5, and 7 years, respectively. Overall, the G' values were considerably more significant than the loss modulus (G") in both the heating and cooling scans. Strengthening times at 5 °C for the gelatin ranged between 25 and 32 s, which indicated a considerably fast gelling gel. The gelling time depends on the raw materials and extraction procedure used. The gelatin sources can be identified by the gelling and MPs because mammalian sources are more likely to have higher MPs than fish sources [40].
Gel strength
Gel strength is a central functional property of gelatin. The gel strength is related to the amino acid profile. The gel strength of gelatin is usually categorized into three groups: low (< 150 g), medium (150–220 g), and substantial (220–300 g) bloom values (Mhd Sarbon et al. 2013). Based on the results obtained, the gelatin extracted from CBG-2.5Y and CBG-4.5Y were classified as medium bloom at 178 ± 9.6 and 192 ± 8.9 g, respectively. The CBG-7Y gel was grouped as low bloom with a value of 107 ± 6.5 g. The gel strength of commercial bovine and commercial fish gelatin were 226 ± 1.4 and 110 ± 2.1 g, respectively (Table 2). Fish gelatin is always identified as a low bloom number. The gel strength of some fish skins reported as a medium was as follows: 181 g in Sepia officinalis [41], 206 g in sharkskin gelatin [31], 128 and 180 g in reddish colored and dark-colored tilapia, respectively [32], and 121 g in Aluterus monoceros [15]. Proline and hydroxyproline are the imino acids responsible for the stability of the collagen structure by forming hydrogen bonds between water molecules and hydroxyl groups in gelatin. These bonds influence the properties of gelatin, including gel strength. Gelatin with low proline and hydroxyproline levels has a lower gel strength than gelatin with high levels of proline and hydroxyproline [41].
Amino acid composition
The results of the amino acid analysis are shown in Table 3. They were compared with amino acid values previously reported for gelatin from bovine and pig skins. The major amino acid compositions of CBG are glycine (19.81–21.13%), proline (9.04–10.76%), glutamic acid (7.36–10.71%), alanine (7.20–9.23%), and hydroxyproline (6.71–8.56%). In comparison with glycine (32.20% and 31.20%), proline (13.10% and 8.57%), glutamic acid (7.10 and 12.3%), and hydroxyproline (9.80%–N/A) for porcine and bovine, respectively. As a result of the formation of a triple helical structure in gelatin, a high glycine and imino acid content (proline and hydroxyproline) can influence the physical and rheological properties of the gelatin, including the viscoelasticity, gel strength, and melting and gelling temperatures. The amino acid profile does not seem to be affected by the age of the animals. However, the most exciting fact is the uniformity of high glycine in gelatin from all species; approximately 20% of the total amino acids found in gelatin.
From the preceding considerations, camel gelatin is a promising candidate to replace imported food and medicines that contain pigskin gelatin. Hydroxyproline is a significant component of the protein collagen and plays a vital role in the stability of the collagen triple helix. It can be used as an indicator to determine the amount of collagen. The amino acid composition of camel gelatin was in line with mammalian species. Muyonga et al. [28] found that proline and hydroxyproline form approximately 30% of mammalian gelatin amino acids, while Farris et al. [42] found this ratio to be only 23% in pigskin gelatin. The results of this study were in line with the findings of Farris et al. [42], who reported no cysteine present in pigskin gelatin. Meanwhile, fish collagen has an unstable structure compared with mammalian collagen due to its low hydroxyproline content. However, the critical gelatin components from chicken skin were estimated by Mhd Sarbon et al. [43], and they were identified to be glycine (33.7%), proline (13.42%), alanine (10.08%), glutamic acid (5.84%), and arginine (5.57%). In comparison with goatskin gelatin, chicken skin gelatin has a similar amount of glycine and proline. CBG showed that the minor elements (less than 2%) included isoleucine (1.41%), methionine (1.13%), tyrosine (1.11%), histidine (1.05%), and cysteine (0. 01%). Mhd Sarbon et al. (2013) reported similar results for cysteine. However, amino acids are classified into four categories based on their polarity: nonpolar, polar, acidic, and alkaline amino acids. These amino acids contribute to the functional properties of gelatin, such as gel strength, foaming properties, and emulsion activity index. The total amount of nonpolar amino acids in CBG ranged from 33 to 36%, and the total content of polar amino acid was 29%.
ATR-FTIR scanning
ATR-FTIR spectra of the extracted CBGs, commercial bovine and commercial FG are shown in Fig. 3. The O–H stretching band at 3309.38 cm−1 and the C-H stretching band at 2941.08 cm−1 were observed for amide A (3400–3300 cm−1). The band at 1339.32 cm−1 was present in O–H of water. The band at 1543.13 cm−1 was due to -NH twisting (amide II) caused by the bending vibrations of NH groups and stretching vibrations of CN groups [44]. The band at 1647.98 cm−1 was due to C = O stretching as amide I. The amide I band is probably the most useful measure for analysing protein secondary structure by infrared spectroscopy [45]. The amide III peak arises from many factors, including CN stretching vibrations, NH deformation from amide linkages, and wagging vibrations from CH2 groups on glycine and proline [19]. Furthermore, Pshenitsyna et al. [46] reported that the absorption band of amide II is often used for structural characterization; amide I and amide II (1650 and 1550 cm−I, respectively) are associated with the stretching vibrations of C = O and C-N groups and the deformation vibrations of NH groups, respectively. The use of ammonium sulfate can separate FG components with different GS where the GS decreased gradually with increases in the salt saturation; however, the salt did not destroy the four characteristics of the FTIR peaks of amide A, amide I, amide II, and amide III [19].
Conclusion
CBG with different characteristics was extracted with good yield using hot water after acid pretreatment and neutralization with ammonium sulfate. HCl was used for bone demineralization and the swelling process. The properties of the extracted gelatins differed from one another based on the age of the camel bone. The extracted gelatin showed good gel, chemical, and physical properties similar to other mammalian gelatins. These findings are significant for locations where camel bones are considered waste products that are not reprocessed. Thus, the high gel strength and viscosity of the prepared gels indicate that camel bone is a new potential source of gelatin for use in the food and pharmaceutical industries.
Change history
06 July 2021
A Correction to this paper has been published: https://doi.org/10.1007/s11694-021-01047-w
References
Y.-H. Kuan, A.M. Nafchi, N. Huda, F. Ariffin, A.A. Karim, Effects of sugars on the gelation kinetics and texture of duck feet gelatin. Food Hydrocolloids 58, 267–275 (2016). https://doi.org/10.1016/j.foodhyd.2016.02.025
R. Hashemi Tabatabaei, S.M. Jafari, H. Mirzaei, A. Mohammadi Nafchi, D. Dehnad, Preparation and characterization of nano-SiO2 reinforced gelatin-k-carrageenan biocomposites. Int. J. Biol. Macromol. 111, 1091–1099 (2018). https://doi.org/10.1016/j.ijbiomac.2018.01.116
A. Tügen, B. Ocak, Ö. Özdestan-Ocak, Development of gelatin/chitosan film incorporated with lemon essential oil with antioxidant properties. J. Food Meas. Charact. 14(6), 3010–3019 (2020). https://doi.org/10.1007/s11694-020-00547-5
J. Pan, H. Lian, M. Shang, W. Jin, R. Hao, Y. Ning et al., Physicochemical properties of Chinese giant salamander (Andrias davidianus) skin gelatin as affected by extraction temperature and in comparison with fish and bovine gelatin. J. Food Meas. Charact. 14(5), 2656–2666 (2020). https://doi.org/10.1007/s11694-020-00512-2
E. Ali, S. Sultana, S.B.A. Hamid, M. Hossain, W.A. Yehya, A. Kader et al., Gelatin controversies in food, pharmaceuticals, and personal care products: authentication methods, current status, and future challenges. Crit. Rev. Food Sci. Nutr. 58(9), 1495–1511 (2018). https://doi.org/10.1080/10408398.2016.1264361
A. Abedinia, A.M. Nafchi, M. Sharifi, P. Ghalambor, N. Oladzadabbasabadi, F. Ariffin et al., Poultry gelatin: characteristics, developments, challenges, and future outlooks as a sustainable alternative for mammalian gelatin. Trends Food Sci. Technol. 104, 14–26 (2020)
N. Zhang, H. Liu, L. Yu, X. Liu, L. Zhang, L. Chen et al., Developing gelatin–starch blends for use as capsule materials. Carbohyd. Polym. 92(1), 455–461 (2013). https://doi.org/10.1016/j.carbpol.2012.09.048
A. Abedinia, F. Ariffin, N. Huda, A.M. Nafchi, Preparation and characterization of a novel biocomposite based on duck feet gelatin as alternative to bovine gelatin. Int. J. Biol. Macromol. 109, 855–862 (2018)
A.A. Al-Hassan, Gelatin from camel skins: extraction and characterizations. Food Hydrocolloids 101, 105457 (2020). https://doi.org/10.1016/j.foodhyd.2019.105457
A. Abedinia, F. Ariffin, N. Huda, A.M. Nafchi, Extraction and characterization of gelatin from the feet of Pekin duck (Anas platyrhynchos domestica) as affected by acid, alkaline, and enzyme pretreatment. Int. J. Biol. Macromol. 98, 586–594 (2017)
M. Alshafiee, M.K. Aljammal, D. Markl, A. Ward, K. Walton, L. Blunt et al., Hot-melt extrusion process impact on polymer choice of glyburide solid dispersions: the effect of wettability and dissolution. Int. J. Pharm. 559, 245–254 (2019). https://doi.org/10.1016/j.ijpharm.2019.01.038
M.C. Gómez-Guillén, J. Turnay, M.D. Fernández-Dı́az, N. Ulmo, M.A. Lizarbe, P. Montero, Structural and physical properties of gelatin extracted from different marine species: a comparative study. Food Hydrocolloids 16(1), 25–34 (2002). https://doi.org/10.1016/S0268-005X(01)00035-2
I.J. Haug, K.I. Draget, O. Smidsrød, Physical and rheological properties of fish gelatin compared to mammalian gelatin. Food Hydrocolloids 18(2), 203–213 (2004). https://doi.org/10.1016/S0268-005X(03)00065-1
M.H. Norziah, A. Al-Hassan, A.B. Khairulnizam, M.N. Mordi, M. Norita, Characterization of fish gelatin from surimi processing wastes: thermal analysis and effect of transglutaminase on gel properties. Food Hydrocolloids 23(6), 1610–1616 (2009). https://doi.org/10.1016/j.foodhyd.2008.12.004
M. Ahmad, S. Benjakul, Characteristics of gelatin from the skin of unicorn leatherjacket (Aluterus monoceros) as influenced by acid pretreatment and extraction time. Food Hydrocolloids 25(3), 381–388 (2011). https://doi.org/10.1016/j.foodhyd.2010.07.004
D. Fernández, P. Montero, M.C. Gómez-Guillén, Effect of freezing fish skins on molecular and rheological properties of extracted gelatin. Food Hydrocolloids 17(3), 281–286 (2003). https://doi.org/10.1016/S0268-005X(02)00078-4
M.C. Gómez-Guillén, B. Giménez, M.E. López-Caballero, M.P. Montero, Functional and bioactive properties of collagen and gelatin from alternative sources: a review. Food Hydrocolloids 25(8), 1813–1827 (2011). https://doi.org/10.1016/j.foodhyd.2011.02.007
A. Ishaq, U. Rahman, A. Sahar, R. Perveen, A.J. Deering, A.A. Khalil et al., Potentiality of analytical approaches to determine gelatin authenticity in food systems: a review. LWT 121, 108968 (2020). https://doi.org/10.1016/j.lwt.2019.108968
X.-M. Sha, Z.-C. Tu, W. Liu, H. Wang, Y. Shi, T. Huang et al., Effect of ammonium sulfate fractional precipitation on gel strength and characteristics of gelatin from bighead carp (Hypophthalmichthys nobilis) scale. Food Hydrocolloids 36, 173–180 (2014). https://doi.org/10.1016/j.foodhyd.2013.09.024
N.A. Nik Muhammad, N. Huda, A.A. Karim, N.A. Mohammadi, Effects of acid type extraction on characterization and sensory profile of duck feet gelatin: towards finding bovine gelatin alternative. J. Food Meas. Charact. 12(1), 480–486 (2018). https://doi.org/10.1007/s11694-017-9661-8
M.A.A. Abri, B. Faye, Genetic improvement in dromedary camels: challenges and opportunities. Front. Genet. (2019). https://doi.org/10.3389/fgene.2019.00167
MEWA. Ministry of Enviroment Water and Agriculture, Animal Population Members (MEWA, Riyadh, 2017)
O.K. Yousif, S.A. Babiker, The desert camel as a meat animal. Meat Sci. 26(4), 245–254 (1989). https://doi.org/10.1016/0309-1740(89)90010-7
H.A. Al-Kahtani, I. Jaswir, E.A. Ismail, M.A. Ahmed, A. Monsur Hammed, S. Olorunnisola et al., Structural characteristics of camel-bone gelatin by demineralization and extraction. Int. J. Food Prop. 20(11), 2559–2568 (2017). https://doi.org/10.1080/10942912.2016.1244543
V. Ferraro, B. Gaillard-Martinie, T. Sayd, C. Chambon, M. Anton, V. Santé-Lhoutellier, Collagen type I from bovine bone. Effect of animal age, bone anatomy and drying methodology on extraction yield, self-assembly, thermal behaviour and electrokinetic potential. Int. J. Biol. Macromol. 97, 55–66 (2017). https://doi.org/10.1016/j.ijbiomac.2016.12.068
B.S. Institution, Methods for Sampling and Testing Gelatine (Physical and Chemical Methods) (British Standards Institution, London, 1975)
J.A. White, R.J. Hart, J.C. Fry, An evaluation of the waters pico-tag system for the amino-acid analysis of food materials. J. Autom. Chem. 8, 867320 (1986). https://doi.org/10.1155/S1463924686000330
J.H. Muyonga, C.G.B. Cole, K.G. Duodu, Fourier transform infrared (FTIR) spectroscopic study of acid soluble collagen and gelatin from skins and bones of young and adult Nile perch (Lates niloticus). Food Chem. 86(3), 325–332 (2004). https://doi.org/10.1016/j.foodchem.2003.09.038
I. Ratnasari, S. Yuwono, H. Nusyam, S. Widjanarko, Extraction and characterization of gelatin from different fresh water fishes as alternative sources of gelatin. Int. Food Res. J. 20(6), 3085 (2013)
Gmia, Gelatin Handbook (Gmia Inc, New York, 2012)
K. Shyni, G.S. Hema, G. Ninan, S. Mathew, C.G. Joshy, P.T. Lakshmanan, Isolation and characterization of gelatin from the skins of skipjack tuna (Katsuwonus pelamis), dog shark (Scoliodon sorrakowah), and rohu (Labeo rohita). Food Hydrocolloids 39, 68–76 (2014). https://doi.org/10.1016/j.foodhyd.2013.12.008
B. Jamilah, K.G. Harvinder, Properties of gelatins from skins of fish—black tilapia (Oreochromis mossambicus) and red tilapia (Oreochromis nilotica). Food Chem. 77(1), 81–84 (2002). https://doi.org/10.1016/S0308-8146(01)00328-4
J.S. Zilhadia, H. Yahdiana, J. Irwandi, A. Effionora, Characterization and functional properties of gelatin extracted from goatskin. Int. Food Res. J. 25(1), 275–281 (2018)
R.S.G. Silva, S.F. Bandeira, L.A.A. Pinto, Characteristics and chemical composition of skins gelatin from cobia (Rachycentron canadum). LWT Food Sci. Technol. 57(2), 580–585 (2014). https://doi.org/10.1016/j.lwt.2014.02.026
A. Jongjareonrak, S. Rawdkuen, M. Chaijan, S. Benjakul, K. Osako, M. Tanaka, Chemical compositions and characterisation of skin gelatin from farmed giant catfish (Pangasianodon gigas). LWT Food Sci. Technol. 43(1), 161–165 (2010). https://doi.org/10.1016/j.lwt.2009.06.012
H. Yang, Y. Wang, P. Zhou, J.M. Regenstein, Effects of alkaline and acid pretreatment on the physical properties and nanostructures of the gelatin from channel catfish skins. Food Hydrocolloids 22(8), 1541–1550 (2008). https://doi.org/10.1016/j.foodhyd.2007.10.007
A. Sanaei, F. Mahmoodani, S. See, S. Yusop, A. Babji, Optimization of gelatin extraction and physico-chemical properties of catfish (Clarias gariepinus) bone gelatin. Int. Food Res. J. 20(1), 423 (2013)
V. Gudipati, Fish gelatin: a versatile ingredient for the food and pharmaceutical industries, in Marine Proteins and Peptides. ed. by S.K. Kim (Wiley, Chichester, 2013), pp. 271–295
N.F. Mohtar, C. Perera, S.-Y. Quek, Optimisation of gelatine extraction from hoki (Macruronus novaezelandiae) skins and measurement of gel strength and SDS–PAGE. Food Chem. 122(1), 307–313 (2010). https://doi.org/10.1016/j.foodchem.2010.02.027
G. Boran, S.J. Mulvaney, J.M. Regenstein, Rheological properties of gelatin from silver carp skin compared to commercially available gelatins from different sources. J. Food Sci. 75(8), E565–E571 (2010). https://doi.org/10.1111/j.1750-3841.2010.01543.x
R. Balti, M. Jridi, A. Sila, N. Souissi, N. Nedjar-Arroume, D. Guillochon et al., Extraction and functional properties of gelatin from the skin of cuttlefish (Sepia officinalis) using smooth hound crude acid protease-aided process. Food Hydrocolloids 25(5), 943–950 (2011). https://doi.org/10.1016/j.foodhyd.2010.09.005
S. Farris, J. Song, Q. Huang, Alternative reaction mechanism for the cross-linking of gelatin with glutaraldehyde. J. Agric. Food Chem. 58(2), 998–1003 (2010). https://doi.org/10.1021/jf9031603
N. Mhd Sarbon, F. Badii, N.K. Howell, Preparation and characterisation of chicken skin gelatin as an alternative to mammalian gelatin. Food Hydrocolloids 30(1), 143–151 (2013). https://doi.org/10.1016/j.foodhyd.2012.05.009
M. Nagarajan, S. Benjakul, T. Prodpran, P. Songtipya, Effects of bleaching on characteristics and gelling property of gelatin from splendid squid (Loligo formosana) skin. Food Hydrocolloids 32(2), 447–452 (2013). https://doi.org/10.1016/j.foodhyd.2013.02.003
W.K. Surewicz, H.H. Mantsch, New insight into protein secondary structure from resolution-enhanced infrared spectra. Biochim. Biophys. Acta (BBA) 952, 115–130 (1988). https://doi.org/10.1016/0167-4838(88)90107-0
V. Pshenitsyna, N. Molotkova, M. Noskova, B.Y. Aksel’Rod, Display of the amide II absorption band in the spectra of reaction products of urea with formaldehyde. J. Appl. Spectrosc. 29(3), 1098–1101 (1978)
Acknowledgements
The author would like to express his thanks to King Abdul-Aziz City for Science and Technology (KACST) Saudi Arabia for the financial support under project No: A.T. 34-305.
Author information
Authors and Affiliations
Contributions
AAA Investigation, Conceptualization, Methodology, Validation, Formal analysis, Writing-original draft, Funding. AMN: Software, Data curation, Validation, Supervision, AMA Investigation; FA: Investigation; HMM: Investigation, Resources.
Corresponding authors
Ethics declarations
Conflict of interest
The authors have declared that they have no conflict of interest.
Animal and human rights
This article does not contain any studies with human or animal subjects.
Additional information
Publisher's Note
Springer Nature remains neutral with regard to jurisdictional claims in published maps and institutional affiliations.
The original version of this article, unfortunately, contained a mistake. The second and third author’s affiliations mixed up and the correct affiliations are as follow: A. M. Abdel-Salam Dairy Science Department, National Research Centre, Dokki, Cairo, 12622, Egypt F. Al Nasiri Saudi Food and Drug Administration, Riyadh, 13312, Saudi Arabia Also, the article has two corresponding authors, A. A. Al-Hassan and Abdorreza Mohammadi Nafchi These errors do not affect any results or conclusions. The original article has been corrected.
Rights and permissions
About this article
Cite this article
Al-Hassan, A.A., Abdel-Salam, A.M., Al Nasiri, F. et al. Extraction and characterization of gelatin developed from camel bones. Food Measure 15, 4542–4551 (2021). https://doi.org/10.1007/s11694-021-01029-y
Received:
Accepted:
Published:
Issue Date:
DOI: https://doi.org/10.1007/s11694-021-01029-y