Abstract
Fungal laccases are typically unstable at high pH and temperature conditions, which limit their application in the decolorization of textile wastewater. By contrast, the highly stable bacterial laccases can function within a wider pH range and at high temperatures, thus have significant potential in treatment for textile wastewater. In our previous work, a thermo-alkali-stable CotA-laccase gene was cloned from Bacillus pumilus W3 and overexpressed in Escherichia coli. In this study, the robust CotA-laccase achieved efficient secretory expression in Bacillus subtilis WB600 by screening a suitable signal peptide. A maximum CotA-laccase yield of 373.1 U/mL was obtained at optimum culture conditions in a 3-L fermentor. Furthermore, the decolorization and detoxification of textile industry effluent by the purified recombinant CotA-laccase in the presence and absence of redox mediators were investigated. Among the potential mediators that enhanced effluent decolorization, acetosyringone (ACS) was the most effective. The toxicity of the CotA-laccase-ACS-treated effluent was greatly reduced compared with that of the crude effluent. To the best of our knowledge, this study is the first to report on the heterologous expression of CotA-laccase in B. subtilis. The recombinant strain B. subtilis WB600-5 has a great potential in the industrial production of this bacterial enzyme, and the CotA-laccase-ACS system is a promising candidate for the biological treatment of industrial textile effluents.
Similar content being viewed by others
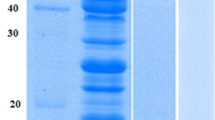
Explore related subjects
Discover the latest articles, news and stories from top researchers in related subjects.Avoid common mistakes on your manuscript.
Introduction
Effluents produced by textile industries are often strongly colored; thus, their disposal into receiving waters reduces light penetration and subsequently disrupts the photosynthetic activity of aquatic plants (Singh et al. 2011; Giardina et al. 2010). Affected wastewaters may also be toxic to aquatic organisms because of the presence of metals, chlorides, and dye breakdown products (Khlifi et al. 2010). High concentrations of these dyes can cause waterborne diseases and increase the biological oxygen demand of receiving waters. Approximately 10 to 15 % of the total dyes from various textile and other industries are discharged in wastewater, causing extensive pollution worldwide (Robinson et al. 2001; Keharia and Madamvar 2003). Therefore, the treatment of industrial effluents that contain aromatic compounds is necessary prior to their discharge to the environment (Singh et al. 2011).
Currently, textile effluents are treated by expensive physicochemical methods that do not generally degrade the pollutants but only cause dye accumulation as sludge, which creates disposal problems. Therefore, biological processes have attracted considerable attention because of their cost effectiveness and environment friendliness (Mendes et al. 2011; Rodríguez Couto and Toca Herrera 2006).
Laccases are multicopper-containing enzymes that can catalyze the oxidation of an extensive range of phenolic and nonphenolic aromatic compounds, along with the reduction of molecular oxygen to water (Singh et al. 2011; Giardina et al. 2010). Laccase-catalyzed dye degradation is a well-studied phenomenon. The use of laccases has been suggested for the biobleaching of industrial textile dyes, including azo, anthraquinonic, triarylmethane, and indigoid dyes (Rodríguez Couto and Toca Herrera 2006).
Laccases are widely distributed among fungi, higher plants, and bacteria (Claus 2003). Fungal and plant laccases function better at low pH and temperature conditions. By contrast, highly stable bacterial laccases can function within a wider pH range and at high temperatures (Singh et al. 2011; Sharma et al. 2007). Bacterial enzymes are also less dependent on metal ions and less susceptible to inhibitory agents than other enzymes (Singh et al. 2011). The biological treatment of textile dyeing industrial wastewaters usually requires enzymes to remain active under high pH and temperature conditions or under high concentrations of organic solvents (Klibanov 2001). Thus, fungal laccases are mostly unsuitable for commercial purposes (Huang et al. 2011). Meanwhile, bacterial laccases, such as Bacillus CotA-laccase, have significant potential industrial applications in the decolorization of industrial textile dye effluents (Sharma et al. 2007).
The major obstacle for the commercial application of bacterial laccases is the lack of sufficient enzyme stocks, as the production yield from natural sources is usually low (Singh et al. 2011; Dubé et al. 2008). The recombinant production of enzymes in easily cultivable hosts is a suitable means to achieve high productivity. Escherichia coli is a commonly used host for heterologous protein expression because of its fast growth and easy genetic manipulation (Banerjee et al. 2009). To date, several bacterial laccases have been successfully expressed in E. coli (Santhanam et al. 2011). However, all these recombinant enzymes are located intracellularly, which complicates purification and thus increases production cost. Extracellular protein expression is potentially an ideal solution to this problem. The Gram-positive bacterium Bacillus subtilis is a well-established host for the industrial-scale production of extracellular proteins. Different from E. coli, B. subtilis is endotoxin free and is generally regarded as safe (Degering et al. 2010).
We have recently characterized a novel CotA-laccase cloned from Bacillus pumilus W3. CotA-laccase was overexpressed by E. coli in its biologically active form. The purified recombinant CotA-laccase was highly stable in high pH and temperature conditions and exhibited considerable tolerance to organic solvents and NaCl. CotA-laccase can efficiently decolorize azo and anthraquinonic dyes under alkaline condition (pH 9.0) when combined with a mediator, making this enzyme highly potent for the industrial decolorization of textile dyeing effluents (Guan et al. 2014). In the present study, the recombinant strain B. subtilis WB600-5 with high-level CotA-laccase secretory production was constructed by searching a suitable signal peptide. The key medium components (corn starch, peptone, and yeast extract) and temperature were optimized in a shaker flask to further increase the expression of CotA-laccase. The enzyme production of B. subtilis was conducted in a 3-L fermentor under optimal conditions. The decolorization and detoxification capabilities of CotA-laccase on dye effluents from a textile factory were tested with or without a mediator.
Materials and methods
Strains, plasmids, and chemicals
E. coli DH5α was used to clone and construct genes and plasmids. B. subtilis WB600 was used as the host for CotA-laccase secretory production. The seven pMA0911 plasmid derivatives were provided by Dr. Yu Xia (Jiangnan University, China). 2,2′-Azino-bis(3-ethylbenzothiazoline-6-sulfonate) (ABTS), 2,6-dimethoxyphenol (2,6-DMP), syringaldazine (SGZ), p-coumarate, m-coumarate, syringic acid, vanillic acid, acetosyringone (ACS), vanillin, syringaldehyde, and methylsyringate were all purchased from Sigma-Aldrich (MO, USA). The bacterial DNA extraction kit, gel extraction kit, Ex Taq, and Pyrobest DNA polymerase were purchased from TaKaRa (Dalian, China). All other chemicals used were standard reagent grade.
Construction and expression of recombinant plasmids in B. subtilis WB600
The CotA-laccase gene of B. pumilus W3 (GenBank no. KF040050) was amplified by PCR from our previously constructed plasmid pColdII-cotA (Guan et al. 2014) using the primers Gp11 (5′-TCAGGAGAATTCATGAACCTAGAAAAATTTGT-3′) and Gp22 (5′-ACTGAGGGATCCTTACTGGATGATATCCATCG-3′), which introduced unique EcoRI and BamHI restriction sites respectively (underlined). The resulting 1557-bp DNA fragment was purified, digested with EcoRI and BamHI, and subsequently ligated into each of the seven pMA0911 derivatives that harbor the signal peptides oppA, bpr, yvgO, amyX, wapA, yclQ, and nprE to generate the corresponding plasmids pMA0911-1, pMA0911-2, pMA0911-3, pMA0911-4, pMA0911-5, pMA0911-6, and pMA0911-7 (Brockmeier et al. 2006) (Table 1). Each recombinant plasmid was transformed into the host strain B. subtilis WB600 to obtain the recombinant strains WB600-1, WB600-2, WB600-3, WB600-4, WB600-5, WB600-6, and WB600-7, respectively (Zhang et al. 2013). The vector pMA0911 without insert was transformed into B. subtilis WB600 and used as the control.
The transformants were grown at 37 °C for 8 h on LB agar plates containing 100 μg/mL kanamycin, 0.5 mM ABTS, and 0.1 mM CuSO4. The transformants with dark green halo around their colonies were considered positive clones. The presence of the CotA-laccase gene in the transformants, and no mutations introduced was further confirmed by colony PCR and subsequently sequencing (Sangon Biotech, China). The colonies were grown at 37 °C for 36 h in conical flasks that contain 25 mL of culture medium.
Characterization of the purified recombinant CotA-laccase
The recombinant CotA-laccase was purified from the highest laccase-producing strain WB600-5, which harbored the recombinant plasmid pMA0911-5. Briefly, solid ammonium sulfate was added to the supernatant to 75 % saturation at 0 °C. The precipitate was collected and dissolved in phosphate-buffered saline (pH 7.4) and dialyzed overnight against the same buffer. After dialysis, the enzyme solution was filtered using water film (10 kDa cutoff), and then injected into an AKTA purifier (GE Healthcare, PA, USA) by anion-exchange chromatography (Q-Sepharose HP). The molecular weight of the recombinant CotA-laccase was determined by sodium dodecyl sulfate polyacrylamide gel electrophoresis (SDS-PAGE).
Laccase activity was assayed in a citrate-phosphate-borate buffer (100 mM, pH 3.0–9.0) at 30 °C, with ABTS, SGZ, and 2,6-DMP as the substrates, as previously described by Guan et al. (2014). One unit of enzyme activity was defined as the amount of enzyme required to oxidize 1 μmol of substrate per minute. Assays for the detection of optimum temperature, temperature stability, optimum pH, and pH stability for CotA-laccase activity were also conducted as previously described (Guan et al. 2014).
Optimization of the recombinant CotA-laccase production
The effects of culture conditions (corn starch concentration, peptone concentration, yeast extract concentration, and temperature) on the recombinant production of CotA-laccase in B. subtilis WB600-5 were examined. Various concentrations of corn starch (0, 6, 12, 18, 24, 30, 36, and 42 g/L), peptone (2, 4, 6, 8, 10, 12, 14, and 16 g/L), and yeast extract (0, 3, 6, 9, 12, 15, 18, and 21 g/L) were added into the culture medium to determine their effects on the production of CotA-laccase. The effect of temperature (25, 28, 31, 34, 37, and 40 °C) on the production of CotA-laccase was also investigated.
Production of recombinant CotA-laccase in a 3-L fermentor
The optimized culture medium for the optimum CotA-laccase yield by B. subtilis WB600-5 was used to scale-up fermentation in a 3-L fermentor (NBS, NJ, USA). The medium was supplemented with 18 g/L corn starch, 10 g/L peptone, 12 g/L yeast extract, 10 g/L NaCl, and 100 μg/mL kanamycin. The fermentation was performed with a working volume of 1.6 L at 37 °C for 60 h. The stirring speed of fermentation was 600 rpm, and the volume of ventilation was 1 vvm.
Industrial effluent used and decolorization tests
Industrial effluent was supplied by a textile factory (Nanjing, China) that utilizes different dyes and other chemicals, including detergents, salts, and surfactants. The effluent characteristics are summarized in Table 2.
Effluent decolorization was conducted using the purified CotA-laccase. Unless otherwise indicated, all experiments were performed in 10-mL disposable cuvettes in a final reaction volume of 6 mL. The reaction mixture contained 0.1 M Tris-HCl (pH 9.0), 20 to 80 % effluent, and purified CotA-laccase (1 to 9 U/mL) with or without a laccase mediator. The reaction was initiated by the addition of CotA-laccase, and the reaction mixture was incubated in the dark at 60 °C with mild shaking. The controls were run in parallel without CotA-laccase addition. The percentage of effluent decolorization was spectrophotometrically calculated as the relative decrease in absorbance at 600 nm. All reactions were performed in triplicates, and three replicas were made.
Effect of laccase mediators on effluent decolorization
The effects of p-coumarate, ABTS, m-coumarate, syringic acid, vanillic acid, ACS, vanillin, syringaldehyde, and methylsyringate as laccase mediators on effluent decolorization were tested. All compounds were used at concentrations ranging from 0 to 1 mM in the reaction mixture with 30 % crude effluent in 0.1 M Tris-HCl (pH 9.0). The reaction was initiated by the addition of 9 U/mL CotA-laccase, and the reaction mixture was incubated in the dark for 10 h at 60 °C.
Effect of effluent concentration on decolorization by the CotA-laccase-ACS system
The effect of effluent concentration (30, 40, 50, 60, 70, or 80 %) in the reaction mixture with 9 U/mL CotA-laccase and 1 mM ACS in 0.1 M Tris-HCl (pH 9.0) on decolorization was studied. The controls were run in parallel without CotA-laccase addition. The decolorization percentage of the effluent was spectrophotometrically calculated as the relative decrease in absorbance at 600 nm. All reactions were performed in triplicates.
Toxicity analysis
Toxicity assays were performed on the basis of the inhibitory effects of the raw and treated effluents on the growth of the yeast Saccharomyces cerevisiae (Papaefthimiou et al. 2004; Pereira et al. 2009; Mendes et al. 2011) and on the reproduction of the nematode Caenorhabditis elegans (Anderson et al. 2001; Mendes et al. 2011). The experiments were performed as previously described (Mendes et al. 2011).
Results and discussion
Screening an optimal signal peptide for the high secretory expression of CotA-laccase in B. subtilis WB600
Applying an appropriate signal peptide is critical to optimize the extracellular expression of desired proteins (Brockmeier et al. 2006). To date, many signal peptides have been characterized in detail; however, predicting which signal peptide results in the optimal secretion and yield of a given extracellular target protein remains impossible (Degering et al. 2010). Therefore, seven signal peptides were investigated in this study to find an effective signal peptide for the extracellular expression of CotA-laccase in B. subtilis WB600.
The DNA fragment that encodes for CotA-laccase was amplified by PCR from the previously constructed plasmid pColdII-cotA (Guan et al. 2014), and then subcloned into each of the seven pMA0911 derivatives that harbor different signal peptides between the EcoRI and BamHI restriction sites. The seven recombinant plasmids were subsequently transformed into the host strain B. subtilis WB600, and the corresponding recombinant strains were comparatively studied for laccase activity in flask cultures. The extracellular laccase titers of the seven recombinant strains, namely, WB600-1 (oppA), WB600-2 (bpr), WB600-3 (yvgO), WB600-4 (amyX), WB600-5 (wapA), WB600-6 (yclQ), and WB600-7 (nprE), were 22.9, 31.2, 5.4, 27.8, 238.5, 23.7, and 7.5 U/mL, respectively. The intracellular laccase activities of the corresponding recombinants were 3.2, 4.6, 0.8, 3.9, 8.4, 3.5, and 0.9 U/mL, respectively. Obviously, the recombinant strain WB600-5 demonstrated the highest extracellular laccase activity, which was 10- to 40-fold higher than those of the other recombinants. A comparison of the titers of extracellular and intracellular CotA-laccase revealed that CotA-laccase was mostly transported by wapA. Lu et al. (2013) have recently reported that a thermo-alkali-stable CotA-laccase gene from Bacillus licheniformis LS04 is overexpressed in Pichia pastoris and that the maximum extracellular laccase activity is 227.9 U/L in flask cultivation.
These data indicate that the transportation rate directed by a signal peptide is closely related to the targeted secretory protein. Signal peptides play a crucial role in the efficient translocation of secretory proteins by the secretion (Sec) machinery. Signal peptides interact with the SecA protein, the signal recognition particle, and the signal peptidase (Harwood and Cranenburgh 2008; Degering et al. 2010). The interaction between the signal peptide and the mature protein also influences protein export (Choo and Ranganathan 2008; Kaderbhai et al. 2004). Therefore, selecting an efficient signal peptide for any given target protein is highly important.
Properties of purified recombinant CotA-laccase
The results of SDS-PAGE analysis demonstrated that one major band of the CotA-laccase protein (~65 kDa) was secreted into the culture medium of the best-performing strain WB600-5. The recombinant CotA-laccase was purified from the culture supernatant by anion-exchange chromatography (data not shown).
The effects of pH and temperature on the activity and stability of purified CotA-laccase were subsequently determined. The properties of CotA-laccase are similar to those of the purified recombinant CotA-laccase that was overexpressed intracellularly in soluble form by E. coli (Guan et al. 2014). The secretory CotA-laccase exhibits an optimal activity at pH 7.8 and 80 °C with 2,6-DMP as the substrate; the related ranges of pH and temperature stability are pH 6.5 to 11.5 and 20 to 90 °C, respectively, suggesting stability at alkaline pH and high temperatures. In addition, the overexpressed enzyme in E. coli is highly tolerant to NaCl and organic solvents (Guan et al. 2014).
Optimization of culture conditions for enhancing CotA-laccase production
In view of the best-performing strain WB600-5, the secretory yield of CotA-laccase increased as corn starch concentration increased from 0 to 18 g/L, with a maximum CotA-laccase yield of 280.4 U/mL at 18 g/L corn starch (Fig. 1a). However, the CotA-laccase yield decreased when more than 18 g/L corn starch was added. The dry cell weight increased as the concentration of corn starch increased up to 36 g/L.
Optimization of medium constituents and process conditions for CotA-laccase production by the recombinant strain Bacillus subtilis WB600-5. Histogram, CotA-laccase activity; black dot, dry cell weight. a Effect of corn starch concentration, b peptone concentration, c yeast extract concentration, and d temperature on CotA-laccase production
As shown in Fig. 1b, the addition of peptone within a suitable concentration range can greatly enhance the yield of CotA-laccase. The maximum CotA-laccase yield of 329.7 U/mL was achieved when 10 g/L peptone was added. In addition, cell density increased with increasing peptone concentration.
As shown in Fig. 1c, the recombinant CotA-laccase activity increased with increasing yeast extract concentration. The maximum CotA-laccase activity of 346.2 U/mL was achieved at 12 g/L yeast extract. Yeast extract concentrations higher than 12 g/L did not favor CotA-laccase production.
The effects of different temperatures (25, 28, 31, 34, 37, and 40 °C) on CotA-laccase production and cell growth were also investigated (Fig. 1d). The highest CotA-laccase yield (351.7 U/mL) and cell density (3.8 g/L) of B. subtilis were obtained at 37 °C. The yield of recombinant CotA-laccase significantly decreased at low (below 37 °C) or high (above 37 °C) temperatures. These results indicated the important influence of temperature on the expression of recombinant CotA-laccase in B. subtilis.
Production of CotA-laccase in a 3-L fermentor
The expression efficiency of the engineered strain B. subtilis WB600-5 was further explored using a 3-L fermentor. As shown in Fig. 2, the maximum cell concentration of 4.6 g/L was obtained in the fermentor during the growth phase. The expression of CotA-laccase continuously increased and then peaked at 373.1 U/mL after 36 h. One of the most significant obstacles to commercial application of bacterial laccases is the lack of sufficient enzyme stocks (Singh et al. 2011; Lu et al. 2013). The high titer of CotA-laccase indicates that B. subtilis WB600-5 is a suitable engineered host for the industrial production of the robust bacterial enzyme.
Decolorization of effluent by purified recombinant CotA-laccase
The degradation ability of the purified recombinant CotA-laccase obtained from B. subtilis WB600-5 was assessed by incubating 1 to 9 U/mL CotA-laccase in 30 % effluent. The reaction mixture was incubated at 60 °C, and decolorization was monitored by measuring its absorbance at 600 nm at 2-h intervals. However, the maximum decolorization rate was only approximately 24 % when 9 U/mL CotA-laccase was used without a mediator after 10 h of incubation (Fig. 3). These results concurred with our previous findings that the decolorization of anthraquinonic and azo dyes alone by the purified recombinant CotA-laccase from E. coli is limited, with 39 to 46 % partial color removal (Guan et al. 2014). Industrial effluents may contain not only dyes but also salts at very high ionic strength and extreme pH values, chelating agents, precursors, by-products, and surfactants (Wesenberg et al. 2003; Khlifi et al. 2010). The use of a laccase-mediated system over CotA-laccase catalysis could provide a feasible solution to this problem.
Activity of purified CotA-laccase concentration on the decolorization of textile factory effluent. The reaction was performed in 0.1 M Tris-HCl (pH 9.0) with 30 % effluent and purified CotA-laccase without a mediator. The mixture was incubated in the dark at 60 °C for 0 to 10 h with mild shaking. (Number of replicas, 3)
Effect of mediators on effluent decolorization
Of the nine compounds (p-coumarate, ABTS, m-coumarate, syringic acid, vanillic acid, ACS, vanillin, syringaldehyde, and methylsyringate) screened as mediators in the decolorization of 30 % effluent by the purified recombinant CotA-laccase at different concentrations, ACS produced the highest decolorization rate, i.e., >80 % in 10 h (Fig. 4). The decolorization of effluent increased with increasing ACS concentration. The nontoxic, natural phenolic mediator ACS is a promising laccase mediator in the enzymatic bleaching and delignification of kraft pulp (Cañas and Camarero 2010; Camarero et al. 2007). Synthetic mediators suffer from severe drawbacks, such as high cost and formation of toxic compounds, which limit their industrial use; natural mediators are cheap and environment-friendly alternatives (Cañas and Camarero 2010). Thus, ACS is highly suitable for industrial effluent decolorization.
Effect of laccase mediators on the decolorization of textile factory effluent by purified CotA-laccase. 1, p-coumarate; 2, ABTS; 3, m-coumarate; 4, syringic acid; 5, vanillic acid; 6, ACS; 7, vanillin; 8, syringaldehyde; 9, methylsyringate. All compounds were used at concentrations ranging from 0 to 1 mM in the reaction mixture with 30 % effluent in 0.1 M Tris-HCl (pH 9.0) and 9 U/mL CotA-laccase; the reaction mixture was incubated in the dark at 60 °C for 10 h with mild shaking. (Number of replicas, 3)
In the current work, the decolorization rate in the presence of syringic acid was inversely proportional to the mediator concentration. For the other mediators, the decolorization rate of the textile effluent by recombinant CotA-laccase slightly increased. However, the rate decreased at high mediator concentrations. This inhibition of decolorization can be attributed to toxicity at high radical concentrations during the laccase oxidation of the mediators. The efficiency of laccase-mediator systems in the decolorization reaction principally depends on mediator concentration and laccase activity (Wong and Yu 1999; Khlifi et al. 2010). The feasibility of the laccase-mediator systems in biotransformation reactions depends on the redox reversibility of the radical-substrate reaction, as well as on the balance between the stability and reactivity of the mediator radical, which should not inhibit enzyme activity (Camarero et al. 2005).
Effect of effluent concentration on effluent decolorization by the CotA-laccase-ACS system
Effluent concentration affects the efficiency of color removal. The CotA-laccase-ACS system (9 U/mL; 1 mM) decolorized the effluent by 87, 78, 72, 65, 60, and 52 % at effluent concentrations of 30, 40, 50, 60, 70, and 80 %, respectively, over a 10-h incubation period (Fig. 5). The behavior observed indicated that the CotA-laccase oxidation rate of the substrates increased as the substrate concentration increased until saturation. Color removal efficiency decreases with increasing concentrations of textile industrial wastewater using the laccase from Trametes trogii in the presence of mediators (Khlifi et al. 2010). Young and Yu (1997) also reported that high dye concentrations decrease decolorization rates. The use of bacterial laccase in the textile industry is rapidly expanding; in particular, laccase is used not only to decolorize textile effluents but also to bleach textiles and synthetize dyes (Singh et al. 2011). At present, the cost-effective commercial-scale production of thermostable bacterial laccases has been established by Metgen (http://www.metgen.fi/), a company focusing on high-performance specialty enzymes for the clean technology industry in Finland (Santhanam et al. 2011). The trade name of the product is MetZyme (Product No. 10-101-LY). MetZyme is active for a broad pH range and thermostable up to 75 °C; this product has applications in wastewater treatment, pulp and paper industry, and advanced biofuels.
Effect of textile factory effluent concentration on the decolorization activity of the CotA-laccase-ACS system. The reaction was performed in 0.1 M Tris-HCl (pH 9.0) with 30 to 80 % effluent concentration, 9 U/mL CotA-laccase, and 1 mM ACS. The mixture was incubated in the dark at 60 °C for 10 h with mild shaking. (Number of replicas, 3)
Evaluation of the toxicity of the raw and treated effluents
Textile effluents are complex because they comprise a mixture of auxiliaries, salts, surfactants, degradation products, and unknown refractory pollutant components (Singh et al. 2011; Wesenberg et al. 2003). The toxicity of crude effluent and the corresponding enzymatic products treated by the CotA-laccase-ACS system was tested on the basis of the inhibitory effects on S. cerevisiae growth and C. elegans reproduction. Relatively low levels of toxicity (≤40 % inhibition for 100 % treated effluent; ≤ 21 % inhibition for 60 % treated effluent; ≤ 13 % inhibition for 30 % treated effluent) were observed on both bioassays (Fig. 6). The effluents treated with several synthetic mediators, such as 1-hydroxybenzotriazole and N-hydroxyphthalimide, exhibit high toxicities because of the generation of unknown reaction products during dyeing (Cañas and Camarero 2010; Khlifi et al. 2010). These data suggest that the products of textile effluent oxidation mediated by the nontoxic, natural ACS are not harmful to yeast and C. elegans. Thus, ACS may be used to solve toxicity problems in effluent treatment, and the CotA-laccase-ACS system is promising for the degradation and detoxification of textile effluents.
Conclusions
Seven signal peptides were screened and explored for the production of a thermo-alkali-stable CotA-laccase in B. subtilis WB600. The signal peptide wapA showed the best secretion efficiency and resulted in a high titer of 238.5 U/mL. The maximum CotA-laccase yield in a 3-L fermentor reached 373.1 U/mL under optimal culture conditions. This result indicated the remarkable potential of the constructed B. subtilis WB600-5 to produce the bacterial enzyme on an industrial scale. Of the nine redox mediators screened, the natural mediator ACS demonstrated the highest decolorization rate. Overall, the CotA-laccase-ACS system is highly effective in the degradation and detoxification of industrial textile effluents.
References
Anderson G, Boyd W, Williams P (2001) Assessement of sublethal endpoints for toxicity testing with the nematode Caenorhabditis elegans. Environ Toxicol Chem 20:833–838
Banerjee S, Salunkhe SS, Apte-Deshpande AD, Mandi NS, Mandal G, Padmanabhan S (2009) Over-expression of proteins using a modified pBAD24 vector in E. coli expression system. Biotechnol Lett 31:1031–1036
Brockmeier U, Caspers M, Freudl R, Jockwer A, Noll T, Eggert T (2006) Systematic screening of all signal peptides from Bacillus subtilis: a powerful strategy in optimizing heterologous protein secretion in gram-positive bacteria. J Mol Biol 362:393–402
Camarero S, Ibarra D, Martinez MJ, Martinez AT (2005) Lignin-derived compounds as efficient laccase mediators for decolorization of different types of recalcitrant dyes. Appl Environ Microbiol 71:1775–1784
Camarero S, Ibarra D, Martínez AT, Romero J, Gutiérrez A, del Río JC (2007) Paper pulp delignification using laccase and natural mediators. Enzyme Microb Technol 40:1264–1271
Cañas AI, Camarero S (2010) Laccases and their natural mediators: biotechnological tools for sustainable eco-friendly processes. Biotechnol Adv 28:694–705
Choo KH, Ranganathan S (2008) Flanking signal and mature peptide residues influence signal peptide cleavage. BMC Bioinform 9(Suppl. 12):S15
Claus H (2003) Laccases and their occurrence in prokaryotes. Arch Microbiol 179:145–150
Degering C, Eggert T, Puls M, Bongaerts J, Evers S, Maurerand KH, Jaeger KE (2010) Optimization of protease secretion in Bacillus subtilis and Bacillus licheniformis by screening of homologous and heterologous signal peptides. Appl Environ Microbiol 76:6370–6376
Dubé E, Shareck F, Hurtubise Y, Daneault C, Beauregard M (2008) Homologous cloning, expression, and characterization of a laccase from Streptomyces coelicolor and enzymatic decolourisation of an indigo dye. Appl Microbiol Biotechnol 79:597–603
Giardina P, Faraco V, Pezzella C, Piscitelli A, Vanhulle S, Sannia G (2010) Laccases: a never-ending story. Cell Mol Life Sci 67:369–385
Guan ZB, Song CM, Zhang N, Zhou W, Xu CW, Zhou LX, Zhao H, Cai YJ, Liao XR (2014) Overexpression, characterization, and dye-decolorizing ability of a thermostable, pH-stable, and organic solvent-tolerant laccase from Bacillus pumilus W3. J Mol Catal B Enzym 101:1–6
Harwood CR, Cranenburgh R (2008) Bacillus protein secretion: an unfolding story. Trends Microbiol 16:73–79
Huang WT, Tai R, Hseu RS, Huang CT (2011) Overexpression and characterization of a thermostable, pH-stable and organic solvent-tolerant Ganoderma fornicatum laccase in Pichia pastoris. Process Biochem 46:1469–1474
Kaderbhai MA, Davey HM, Kaderbhai NN (2004) A directed evolution strategy for optimized export of recombinant proteins reveals critical determinants for preprotein discharge. Protein Sci 13:2458–2469
Keharia H, Madamvar D (2003) Bioremediation concept for treatment of dye containing wastewater: a review. Indian J Exp Biol 41:1068–1075
Khlifi R, Belbahri L, Woodward S, Ellouz M, Dhouib A, Sayadi S, Mechichi T (2010) Decolourization and detoxification of textile industry wastewater by the laccase-mediator system. J Hazard Mater 175:802–808
Klibanov A (2001) Improving enzymes by using them in organic solvents. Nature 409:241–246
Lu L, Wang TN, Xu TF, Wang JY, Wang CL, Zhao M (2013) Cloning and expression of thermo-alkali-stable laccase of Bacillus licheniformis in Pichia pastoris and its characterization. Bioresour Technol 134:81–86
Mendes S, Farinha A, Ramos CG, Leitão JH, Viegas CA, Martins LO (2011) Synergistic action of azoreductase and laccase leads to maximal decolourization and detoxification of model dye-containing wastewaters. Bioresour Technol 102:9852–9859
Papaefthimiou C, Cabral Mde G, Mixailidou C, Viegas CA, Sa-Correia I, Theophilidis G (2004) Comparison of two screening bioassays, based on the frog sciatic nerve and yeast cells, for the assessment of herbicide toxicity. Environ Toxicol Chem 23:1211–1218
Pereira L, Coelho AV, Viegas CA, Santos MM, Robalo MP, Martins LO (2009) Enzymatic biotransformation of the azo dye Sudan Orange G with bacterial CotA-laccase. J Biotechnol 139:68–77
Robinson T, McMullan G, Marchant R, Nigam P (2001) Remediation of dyes in textile effluents: a critical review on current treatment technologies with a proposed alternative. Bioresour Technol 77:247–255
Rodríguez Couto S, Toca Herrera JL (2006) Industrial and biotechnological applications of laccases: a review. Biotechnol Adv 24:500–513
Santhanam N, Vivanco JM, Decker SR, Reardon KF (2011) Expression of industrially relevant laccases: prokaryotic style. Trends Biotechnol 29:480–489
Sharma P, Goel R, Capalash N (2007) Bacterial laccases. World J Microb Biot 23:823–832
Singh G, Bhalla A, Kaur P, Capalash N, Sharma P (2011) Laccase from prokaryotes: a new source for an old enzyme. Rev Environ Sci Biotechnol 10:309–326
Wesenberg D, Kyriakides I, Spiros NA (2003) White-rot fungi and their enzymes for treatment of industrial dye effluents. Biotechnol Adv 22:161–187
Wong Y, Yu J (1999) Laccase-catalysed decolorization of synthetic dyes. Water Res 33:3512–3520
Young L, Yu J (1997) Ligninase-catalysed decolourization of synthetic dyes. Water Res 31:1187–1193
Zhang J, Kang Z, Ling Z, Cao W, Liu L, Wang M, Du G, Chen J (2013) High-level extracellular production of alkaline polygalacturonate lyase in Bacillus subtilis with optimized regulatory elements. Bioresour Technol 146:543–548
Acknowledgments
This work was supported by the National Natural Science Foundation of China (31472003 & 31101331), the Open Project Program of the Key Laboratory of Industrial Biotechnology, Ministry of Education, China (KLIB-KF201304), a Project Funded by the Priority Academic Program Development of Jiangsu Higher Education Institutions, the 111 Project (No. 111-2-06), and the Jiangsu province “Collaborative Innovation Center for Advanced Industrial Fermentation” industry development program.
Author information
Authors and Affiliations
Corresponding authors
Additional information
Responsible editor: Gerald Thouand
Rights and permissions
About this article
Cite this article
Guan, ZB., Shui, Y., Song, CM. et al. Efficient secretory production of CotA-laccase and its application in the decolorization and detoxification of industrial textile wastewater. Environ Sci Pollut Res 22, 9515–9523 (2015). https://doi.org/10.1007/s11356-015-4426-6
Received:
Accepted:
Published:
Issue Date:
DOI: https://doi.org/10.1007/s11356-015-4426-6