Abstract
Aims
In natural ecosystems, plants generally promote the acquisition of nitrogen (N) through the input of carbon (C) into the soil. The present study aimed to clarify how changes in C input affect N uptake by plants.
Methods
In situ 15N labeling ((15NH4)2SO4 and K15NO3) was performed in grazed and ungrazed Leymus chinensis grasslands located in Inner Mongolia, northern China. Clipping and shading treatments were used to reduce C allocation to roots by limiting photosynthesis because grazing can produce such similar effect through defoliation.
Results
Grazing increased the allocation of photosynthate to roots and the relative biomass of Carex duriuscula but decreased the relative biomass of L. chinensis. Grazing decreased 15N-NH4+ recovery (grazed: 30.9% vs. ungrazed: 39.3%) but increased 15N-NO3− recovery (grazed: 26.9% vs. ungrazed: 17.6%), which may be due to changes in the NH4+/NO3− ratio and species composition. Clipping and shading reduced 15N recovery, mainly because they decreased C supply for N uptake by reducing photosynthesis.
Conclusions
These findings indicate that the reduction of the C input to soil decreases 15N recovery by plants in temperate grasslands, which contributes to our understanding of the trade-off between C and N and grassland N cycles.
Similar content being viewed by others
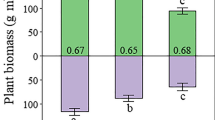
Explore related subjects
Discover the latest articles, news and stories from top researchers in related subjects.Avoid common mistakes on your manuscript.
Introduction
Nitrogen (N) is one of the most important essential nutrients limiting net primary production in many terrestrial ecosystems (LeBauer and Treseder 2008). Ammonium (NH4+) and nitrate (NO3−) are two major forms of inorganic N for plant growth (Masclaux-Daubresse et al. 2010) and are primarily derived from soil organic matter (SOM) mineralization by soil microorganisms (Schimel and Bennett 2004). Numerous studies have revealed differences in the uptake of NH4+ and NO3− by plants due to the chemical properties of nitrate vs. ammonium, e.g., energy consumption (Gessler et al. 1998), ionic toxicity (Lotze and Schramm 2000), and ionic mobility (Owen and Jones 2001). As NH4+ can be directly incorporated into amino acids, its uptake is faster and requires less energy than that required for NO3−, as this ion must be reduced to NH4+ by nitrate reductase before its assimilation (Gessler et al. 1998). However, high NH4+ uptake can cause cell toxicity (Lotze and Schramm 2000). Compared to NO3−, NH4+ has lower mobility in soil solution, because it attaches easily to negatively charged soil colloids (Owen and Jones 2001). The uptake of NH4+ and/or NO3− can alter pH in the root zone, affect the relative uptake of nutrients, and further disturb plant growth (Guo et al. 2002; Brück and Guo 2006). As a result, NH4+ and NO3− often have distinct fates in the ecosystem. Thus, clarifying the fates of NH4+ and NO3−, especially their retention by plants, is beneficial for understanding terrestrial N cycling and can help inform the development of strategies to mitigate N loss.
Grasslands are widely distributed terrestrial ecosystems, accounting for 26% of the ice-free land worldwide (Foley et al. 2011). These ecosystems can influence carbon dioxide (CO2) levels in the atmosphere as they are potential carbon (C) sinks (Li et al. 2008; Smith 2014). Grazing is the most extensive land use mode for grasslands, and is the major driver of grassland degradation. Generally, grazing affects grasslands through herbivory, physical impact, and deposition. Because herbivores consume plant leaves, stems, and other tissues (Díaz et al. 2007; Zheng et al. 2011a), grazing can alter plant community composition, structure, and productivity (Fig. 1). For example, grazing negatively affected the aboveground production in a Stipa grandis/Leymus chinensis-dominated steppe community (Schönbach et al. 2011), while it increased the allocation of C and N to the belowground biomass and promoted N retention and C input to the soil (Hui and Jackson 2006). Additionally, grazing often changes the composition and chemical properties of plant litter and affects litter decomposition (Carrera et al. 2008; Semmartin et al. 2008), thus influencing nutrient cycling in the soil (Bakker et al. 2004; Quested et al. 2007). For example, grazing retarded N cycling through a reduction in N-rich and palatable species and an increase in N-poor species with low litter quality (Shan et al. 2011). Thereby, grazing can change C and N inputs from the roots to the soil (Piñeiro et al. 2009). Herbivores (e.g., cattle and sheep) can also trample plants, disturb soil surfaces, and compact soils through hoof action. As a result, trampling can affect soil microbial activity and SOM mineralization by reducing soil water content and aeration (Lavado and Taboada 1988). Grazing animals affect nutrient cycling by depositing N-rich urine and dung (McNaughton et al. 1997). Overall, grazing can affect the availability of nutrients in the soil by changing the interactions between plants and soil and influencing the utilization and retention of NO3− and NH4+ by plants, and thus their fate (Fig. 1). A prerequisite to understanding and managing the effects of N limitation on plant growth, as well as C sequestration in grasslands, is to clarify the utilization and retention of NO3− and NH4+ by plants.
A scheme for the effect of grazing on the exchange of carbon input and nitrogen uptake in a temperate grassland. Grazing removes part of the aboveground biomass and reduces litter coverage. This process accelerates the evaporation of soil surface water, and thus decreases litter decomposition and SOM mineralization. Substantially livestock trampling decreases soil porosity and limits the diffusion of CO2 and oxygen, thereby inhibiting SOM mineralization and the availability of soil N in the grazed grassland. The arrows indicate the direction of changes in ecological processes and its thickness indicates the magnitude of the changes. White plus sign (+) indicates that carbon input can increase mineralization of soil organic matter (SOM), while white minus sign (−) indicates that increased soil bulk density and reduced soil moisture due to trampling decrease SOM mineralization
Most previous studies have been conducted in alpine grasslands. For example, our previous studies investigated the fates of NH4+ and NO3− in alpine grasslands and found that their fates were distinctly different approximately two months after 15N addition. More 15N was recovered in plants than in microorganisms, but plants and soil microorganisms recovered more 15N-NO3− than 15N-NH4+ (Xu et al. 2003). Similar results were observed 11–13 months after 15N labeling, but SOM recovered more 15N-NH4+ than 15N-NO3− (Xu et al. 2004). A study comparing the effects of overgrazing on the fates of inorganic and organic N in alpine grasslands showed that overgrazing-induced crusts led to lower total 15N recovery from inorganic N than from organic N (Zhang et al. 2017). Compared to alpine grasslands, research on the N cycle in temperate grasslands has mostly focused on net N mineralization (Wang et al. 2006; Liu et al. 2010), N2O emission (Du et al. 2006), and plant-microbe competition for N (Liu et al. 2016; Ouyang et al. 2016). The fates of NH4+ and NO3− in semi-arid temperate grasslands in China remain largely unknown, although these ecosystems comprise nearly 12.5% of the global grassland area (Liu et al. 2010). Given that the climate and water conditions in temperate grasslands are very different from those in alpine grasslands, it is unreasonable to estimate N retention by plants in temperate grasslands based on the results obtained from alpine grasslands.
Additionally, plants under N-limited conditions provide a large amount of available C as root exudates to fuel rhizosphere microorganisms. In return, rhizosphere microorganisms produce extracellular enzymes to accelerate SOM mineralization and release available N to meet their own needs, as well as those of plants (Kuzyakov and Xu 2013; Sun et al. 2014). Such changes in labile C input via root exudation are very common in grasslands, because grazing animals can strongly affect photosynthesis and production through defoliation (Jaramillo and Detling 1988; Ferraro and Oesterheld 2002). These findings indicate that grazing could affect the uptake of N by plants by modifying root exudation (Sun et al. 2018), but this hypothesis remains unexplored in temperate grasslands.
It is well known that grazing can reduce plant height and leaf area directly by defoliation (Díaz et al. 2007; Zheng et al. 2011a). As a result, grazing can affect light availability and plant photosynthesis, thus altering C allocation and fluxes in grasslands. In contrast to grazing, clipping (removing photosynthetic organs) and shading (reducing light intensity) are two important manipulation approaches to reduce the substrate supply to soil from the photosynthesis due to reduced leaf area and light availability (Wan and Luo 2003). Therefore, clipping and shading treatments are often used to simulate grazing effects on grasslands, especially clipping treatment (Dahl et al. 2016). To explore the potential effect of reduced C input via root exudation on NH4+ and NO3− retention by plants, a 15N-labeling field experiment together with clipping and shading treatments was conducted in grazed and ungrazed temperate grasslands in Hulunbeier, Inner Mongolia, for 28 days. The present study aimed to test the following hypotheses: (1) Plants can recover more 15N in grazed than in ungrazed grasslands due to compensatory growth and changes in species composition; (2) Clipping and shading reduce 15N recovery by plants because they decrease the production of the photosynthate used as energy for NH4+ and NO3− uptake by roots; and (3) Plants recover more 15N-NH4+ than 15N-NO3−, because NH4+ is the dominant N form in the soil and plants prefer to absorb the dominant N form.
Materials and methods
Study site
The experiment was conducted at the Hulunbeier Grassland Ecosystem Observation and Research Station (Hulunbeier station) in Inner Mongolia, North China (49°22′42″–49°22′92″N, 120°01′54″–120°02′32″E, 628 m above sea level). The climate is semi-arid inland, with an annual mean temperature ranging from −2 to 1 °C and annual mean precipitation ranging from 380 to 400 mm. Most precipitation occurs in summer, from May to August (Meng et al. 2009). The grassland vegetation is dominated by L. chinensis, and the associated species are mainly Carex duriuscula, Cleistogenes squarrosa, Stipa baicalensis, Potentilla bifurca, and Bupleurum scorzonerifolium. This vegetation type is generally called “Leymus chinensis” grassland. The soil is chestnut soil (IUSS 2014), corresponding to Calcic-orthic Aridisol according to The United States Department of Agriculture (USDA) Soil Taxonomy (Soil Survey Staff 2003).
Experimental settings
In June 2013, one ungrazed temperate grassland and one grazed temperate grassland were selected for the 15N labeling experiment. Both grasslands were about 5 km away from Hulunbeier station. The ungrazed grassland was developed from the grazed grassland by enclosing a portion of the grazed grassland in 2007 to exclude grazing in that portion. This grassland was dominated by L. chinensis and covered approximately an area of 36 ha. The grazed grassland was exposed to perennial grazing mainly by Sanhe cattle in the past 30 years. Soil properties in the upper layer (15 cm) in grazed and ungrazed grasslands are presented in Table 1. Four quadrants (100 × 100 cm) were randomly selected in the grazed or ungrazed grasslands for species investigations (Table 2).
To test whether clipping and shading affect 15NH4+ and 15NO3− recovery by plants, we randomly established sampling plots (20 × 20 cm), separated by at least 1 m, in the grazed and ungrazed grasslands. The sampling plots were provided a clipping treatment, a shading treatment, or control treatment. For the clipping treatment, aboveground parts were harvested 1 cm above the soil surface, and the plants grew freely during the remaining experimental period. The shading treatment consisted of covering the plots with a black mesh to reduce available light intensity up to 90%. At the time of introducing the abovementioned treatments, plant species composition and growth stage were similar in either of the two grasslands. For each treatment, we labelled the plants using two 15N-forms (i.e., 15NH4+ and 15NO3−). There were four replicates for either of two 15N forms under each abovementioned treatment. To calculate the recovery of different N-forms, we also established four plots as references of the natural abundance of 15N of plants in each of the two grasslands. In total, we have 56 plots (3 different treatments [1 clipping +1 shading +1 control] × 2 grasslands × 2 15N-forms × 4 replicates +4 references of the natural abundance of 15N in plants × 2 grasslands).
Based on previous measurements on inorganic N concentrations in the investigated soil, 15N was applied at 1 μg N g−1 soil to avoid a fertilization effect. The tracers ((15NH4)2SO4, 10% 15N enrichment or K15NO3, 10% 15N enrichment) were dissolved in distilled water and injected into the soil at 2.5-cm depth. To ensure an equal distribution of 15N tracers, each plot was divided into nine blocks, and the 15N solution was injected in each block. The reference plots indicating the natural abundance of 15N in plants were injected with the same amount of water.
Sampling and analyses
Three hours after 15N labeling, plant and soil samples were harvested from both treated and control plots, and these were considered as initial soil cores. At the same time, clipping and shading treatments were implemented in grazed and ungrazed grasslands. To test whether sampling time affects the biomass and N recovery, plant and soil samples were collected on days 7, 14, and 28 after 15N labeling. Plants were harvested from a 7 × 7 cm area within each plot using scissors. Soil cores (5 cm in diameter; 10 cm depth) were taken from these areas.
Fresh soil samples were immediately transferred to the laboratory. Living roots were carefully removed from soil cores, and the soil was sieved through a 2-mm mesh and stored at −20 °C. The characteristics of soils including moisture content, inorganic N (NH4+ and NO3−) and microbial biomass C (MBC) were measured within three days, while soil bulk density was determined within two weeks after soil collection.
To determine soil moisture content, fresh soil (15 g) samples were placed in an oven at 105 °C for 24 h. Ten grams of fresh soil was extracted with 40 mL 0.05 M K2SO4 for 1 h, under continuous shaking at 150 r min−1. Filtered extracts were then used to measure NO3−-N and NH4+-N using an auto-analyzer (AA3; Bran-Luebbe, Norderstedt, Germany). Bulk density was determined from undisturbed soil cores treated at 105 °C for 48 h (Van Reeuwijk 1993).
Living roots were rinsed with tap water, submerged in 0.5 mM CaCl2 solution for 30 min, and again rinsed with distilled water to remove the 15N adsorbed on the root surface (Xu et al. 2011). Shoots and roots were then dried at 75 °C for 48 h and ground into a fine powder using a ball mill (MM2; Retsch, Haan, Germany). Plant materials (about 2 mg) were weighed into tin capsules to analyze total N and 15N/14N ratio using a continuous-flow gas isotope ratio mass spectrometer (MAT253; Finnigan MAT, Bremen, Germany), coupled with a ConFlo III device (Finnigan MAT) and an elemental analyzer (EA 1112; CE Instruments, Milan, Italy).
Calculations and statistics
The 15N atom percent excess (APE) was calculated as the difference between the atom% 15N in shoots or roots of plants from 15N labeled and control plots:
The 15N uptake (mg 15N m−2), defined as the amount of 15N recovered from different N pools (shoots and roots), was calculated by multiplying APE (%) by the moles of N in the plants as follows:
The percentage of 15N recovered in plants (15Nrecovery) was calculated using the following equation:
Where 15Nuptake (g m−2) refers to the 15N mass uptake by plants (shoots and roots), and 15Nadded (g m−2) refers to the total 15N mass added to the soil per square meter.
Considering the rapid turnover of NO3− and NH4+, N uptake rates (μg N g−1 dry weight root h−1) was only calculated over three hours by multiplying 15Nuptake by the amount of native NH4+ or NO3− in the soil and then dividing it by the labeling time in hours and the amount of added 15N-NH4+ or 15N-NO3 (Xu et al. 2011).
All results are presented as mean values ± standard errors of the mean. We used the repeated measures analysis of variance (ANOVA) to estimate the effects of time, grazing, treatment (clipping, shading, and control) and their interactions on aboveground biomass, belowground biomass, and total biomass, and to test the effects of time, grazing, treatment, N form (NH4+ and NO3−), and their interactions on 15N recovery in plants. A one-way ANOVA was used to assess the effect of treatment on the recovery of 15N by plants according to sampling time. All analyses were performed after checking for normality and homogeneity of variance and were conducted using the SPSS 16.0 software package (IBM Corp., Armonk, NY, USA). Significant differences were tested at P < 0.05.
Results
Species composition and above- and belowground biomass
Long-term grazing considerably changed plant species composition and vegetation coverage (Table 2). The dominance of plant species shifted from L. chinensis in the ungrazed grassland to C. duriuscula in the grazed grassland. Vegetation coverage was reduced from 70% in the ungrazed grassland to 26.7% in the grazed grassland (Table 2).
Grazing altered the aboveground and belowground biomass of temperate grasslands (P < 0.001, Table 3). The ungrazed grassland had higher values of total aboveground biomass than the grazed grassland, while the belowground biomass showed the opposite pattern (Fig. 2, P < 0.001, Table 3). This resulted in a higher root/shoot ratio in untreated (control, CK) grazed grassland (CK: root/shoot ratio = 4.32 ± 027) than in CK ungrazed grassland (CK: root/shoot ratio = 3.06 ± 0.48) (Fig. 2, P = 0.02).
Aboveground and belowground plant dry mass differences (mean ± SE) among the different times and treatments in grazed and ungrazed grasslands (n = 8). Root/Shoot ratio represents the last sampling (The P value represents the difference of root/shoot ratio between grazed and ungrazed grasslands). Uppercase letters indicate the significant difference of the biomass among three treatments, while lowercase letters represent significant difference of the biomass at different times for either of treatment at P = 0.05
Sampling time had no effect on the aboveground biomass (P = 0.54, Table 3) but significantly affected belowground biomass (P < 0.001, Table 3). Changes in aboveground and belowground biomass showed similar trends under each of the three treatments in both grazed and ungrazed grasslands.
Furthermore, aboveground and belowground biomasses were lower under the clipping and shading treatments than under the control treatment (P < 0.001 for aboveground biomass, P = 0.02 for belowground biomass, P < 0.001 for total biomass, Table 3, Fig. 2).
Plant N uptake rate and soil microbial biomass C
Uptake rates of NH4+-N were higher than those of NO3−-N in grazed and ungrazed grasslands (Fig. 3a, P < 0.05). The uptake rate of NO3−-N by plants was about 10 times higher in the grazed grassland (19.84 ± 1.36 μg N h−1 g−1 root) than in the ungrazed grassland (1.92 ± 0.22 μg N h−1 g−1 root) (Fig. 3a, P < 0.001). Uptake rates of NH4+ by plants were similar in grazed (30.02 ± 3.33 μg N h−1 g−1 root) and ungrazed (36.69 ± 6.96 μg N h−1 g−1 root) grasslands (Fig. 3a, P = 0.60). There was a significant difference in MBC between shading and control treatments in the grazed grassland (Fig. 3b, P < 0.05).
a N uptake rate by plants (3 h) in grazed and ungrazed grasslands. All values are means ± standard errors (n = 12). b Microbial biomass carbon in soil under clipping, shading and control treatments on 28 days in grazed and ungrazed grasslands. Values are means ± standard errors (n = 8). Lowercase letters represent significant difference level at P = 0.05
Recovery of 15N by plants
In the control treatment, 15N-NH4+ recovery in the aboveground biomass was higher in the ungrazed (34.43 ± 9.92%) than in the grazed grassland (23.85 ± 2.56%) 28 days after labeling (Fig. 4a, b, P < 0.05). On the contrary, grazing increased 15N-NO3− recovery (grazed: 21.45 ± 4.28%; ungrazed: 14.59 ± 2.10%) (Fig. 4c, d). Overall, 15N recovery in the aboveground biomass increased over time in grazed and ungrazed grasslands (Fig. 4a–d).
Significant effects and interactions were found for time (P < 0.001), treatment (P < 0.001), and N form (P < 0.001), while grazing had no effect (P = 0.06) on 15N recovery in aboveground biomass (Table 4). Clipping and shading treatments decreased 15N recovery in the aboveground biomass in grazed and ungrazed grasslands, which was consistent with the changing trend in the aboveground biomass (Figs. 2 and 4a–d). 15N-NH4+ recovery was higher than 15N-NO3− recovery under control treatments in both grazed and ungrazed grasslands (Fig. 4a–d).
Grazing had a remarkable effect on 15N recovery by roots (P < 0.001, Table 4). Concretely, 15N recovery by roots under the control treatment was higher in the grazed grassland (15N-NH4+ recovery: 7.03 ± 1.43%; 15N-NO3− recovery: 5.47 ± 0.21%; Fig. 4e, g, Table 4) than in ungrazed grassland (15N-NH4+ recovery: 4.89 ± 0.30%; 15N-NO3− recovery: 3.01 ± 0.21%; Fig. 4 f, 4 h, Table 4) 28 days after the 15N labeling.
Treatment had a significant effect on 15N recovery by roots 28 days after labeling (P = 0.01, Table 4), and 15N recovery was highest under clipping treatment in the grazed grassland (15N-NH4+ recovery: 7.26 ± 0.38%; 15N-NO3− recovery: 6.42 ± 0.31%; Fig. 4e, g) and lowest in the ungrazed grassland (15N-NH4+ recovery: 1.95 ± 0.82%; 15N-NO3− recovery: 2.23 ± 0.65%. Fig. 4f, h). Under both grasslands, the value of 15N recovered (15N-NH4+ and 15N-NO3−) by roots under shading treatment was lower than that under control treatment, but it was higher than that under clipping treatment (Fig. 4).
Under the control treatment, the effect of grazing on the total 15N recovery (shoots + roots) of the two N forms differed. Total 15N recovery from NH4+ in grazed grassland was lower than in the ungrazed grassland, while total 15N recovery from NO3− showed the opposite trend 28 days after labeling (Fig. 5). In addition, total 15N recovery in plants differed significantly between NH4+ and NO3− (P < 0.001, Table 4), with higher values of 15N-NH4+ recovery than 15N-NO3− recovery under the control treatment in both grasslands (P < 0.001, Table 4, Fig. 5). Overall, clipping and shading decreased total 15N recovery in grazed and ungrazed grasslands (Fig. 5). However, the response of 15N-NH4+ and 15N-NO3− recovery by plants to clipping or shading was different in grazed and ungrazed grasslands. Under clipping/shading treatments, there was higher 15N-NO3− recovery in the grazed grassland and higher 15N-NH4+ recovery in the ungrazed grassland (Fig. 5).
Discussion
Grazing effects on plant biomass allocation
Our results indicate that aboveground biomass in the grazed grassland was lower than that in the ungrazed grassland (Fig. 2), which is consistent with previous studies (Ren et al. 2012). This is because livestock removes a part of the stems and leaves, thereby decreasing the leaf area index and the photosynthetic rate of grasses (Díaz et al. 2001; Zheng et al. 2010). Moreover, grazing can lead to changes in the dominant species. For instance, grazing inhibits the growth of taller grasses and promotes the rapid growth of shorter plants that are less accessible to grazers (Golodets et al. 2010), thus reducing the primary productivity of the community. In the current study grazing shifted the dominance of plant species from C. duriuscula in the grazed grassland to L. chinensis in the ungrazed grassland (Table 2). Such a shift in plant species composition could lead to the observed discrepancy in the aboveground biomass between grazed and ungrazed grasslands, because it is known that C. duriuscula is more dwarf than L. chinensis.
The response of belowground biomass to grazing varies with grassland type. Grazing may increase (Frank et al. 2002; Patton et al. 2007), decrease (Semmartin and Oesterheld 2001; Leriche et al. 2003), or have no effect (Turner et al. 1993; McNaughton et al. 1988) on belowground biomass. In the present study, grazing induced an increase in belowground production, possibly because the lower soil inorganic N contents (NH4+ and NO3−) in the grazed grassland (Table 1) lead plants to increase C allocation to roots to acquire more available N (Dijkstra et al. 2008). Additionally, the higher root/shoot ratios in the grazed grassland (Fig. 2) can enhance the resistance of plants to external disturbances (Wang et al. 2003). Therefore, an adaptation of plants to grazing might be to regulate the allocation patterns of photosynthate to shoots and roots (Wang et al. 2003) and increase root density and length to compensate for aboveground shoot removal (Zong et al. 2012).
Aboveground biomass showed different responses to clipping and shading between the two grasslands. The instantaneous photosynthesis of the remaining leaves could increase in response to defoliation (Detling et al. 1979; Nowak and Caldwell 1984; Zhao et al. 2008), possibly due to more chlorophyll synthesized within remaining leaves (Oesterheld and McNaughton 1991). However, the faster photosynthesis of the remaining leaves cannot compensate for the much larger reduction in leaf area such that photosynthesis of the whole plant is decreased. With the growth of new leaves, plant biomass gradually increases.
By comparison, aboveground biomass started to decrease seven days after shading, largely because the continuous decrease in light intensity reduced the photosynthetic rate of plants (Zheng et al. 2011b). Although grazing can increase root biomass to compensate for growth, clipping decreased root biomass. This might be due to a trade-off between aboveground and belowground biomass caused by the compensatory growth of aboveground biomass after clipping treatment (Distelfeld et al. 2014).
Grazing effects on soil mineral N, plant N uptake rate, and soil MBC
Many studies have shown that grazers can influence microbial mineralization and immobilization, leading to changes in N availability for plants (Hamilton and Frank 2001; Rossignol et al. 2006). In the present study, we did not measure mineralization and immobilization, but determined the concentration of mineral N, which was lower in grazed than in ungrazed grassland (Table 1). A possible explanation is that grazing removes part of the aboveground biomass and reduces litter coverage (Schmitt et al. 2013; Wei et al. 2016). This process accelerates the evaporation of soil surface water, and thus decreases litter decomposition and SOM mineralization (Lavado and Taboada 1988; Wang et al. 2010). Additionally, livestock trampling decreases soil porosity and limits the diffusion of CO2 and oxygen, thereby inhibiting SOM mineralization and the availability of soil N (Li et al. 2013). Grazing increased the NO3− uptake rate but reduced the uptake rate of NH4+. One possible explanation is that grazing changed plant species composition and the dominant species differ in their preference for N forms (Xu et al. 2011; Wang et al. 2016).
The increasing MBC was consistent with the higher belowground biomass in the grazed grassland under control treatment (Figs. 2 and 3b). This could be ascribed to increased root exudates caused by higher root biomass, which promote microbial growth in the rhizosphere.
Grazing effects on plant 15N recovery
The increase of 15N recovery in aboveground biomass over time (Fig. 4a–d) is ascribed to continuous 15N transfer from soil and roots to shoots (Kuzyakov and Xu 2013). Higher 15N recovery of roots in grazed than in the ungrazed grassland 28 d after labeling, is consistent with the higher belowground biomass in the grazed grassland.
In the ungrazed grassland, the higher 15N-NH4+ recovery than 15N-NO3− recovery observed under three treatments (Fig. 5) might be related to the N acquisition strategy of the dominant species, L. chinensis, which is a type of rhizomatous clonal grass with a well-developed underground rhizome that enables L. chinensis to spread quickly and escape rapidly from stressful microenvironments (Li et al. 2007). Therefore, the N absorption strategy of L. chinensis will be more flexible and more dependent on the availability of N forms in the soil and L. chinensis in ungrazed grassland absorbed more NH4+ because this ion was the main component of the soil N pool.
Higher plant 15N recovery (15N-NH4+ + 15N-NO3−) in the grazed grassland than in ungrazed grassland confirms our first hypothesis. This result could be ascribed to a large amount of N being redistributed for the re-growth of plants after grazing and increasing the N uptake by plants (Fig. 5). Although NH4+ was higher than NO3− in both grazed and ungrazed grasslands, grazing decreased the total 15N-NH4+ recovery but increased the 15N-NO3− recovery (Fig. 5), partly supporting our third hypothesis. This difference in 15N recovery of both N forms in grazed and ungrazed grasslands is due to changes in inorganic N ratios as well as plant composition. For example, both laboratory and field observations showed that the NH4+/NO3− ratios in the soil affect plant uptake of NH4+ or NO3− (Houlton et al. 2007). In the present study, the grazing-induced decrease in soil NH4+/NO3− ratio led to a reduction in NH4+ uptake by plants (Table 1). In addition, plant species in this kind of temperate grassland have distinct access to various forms of N in the soil (Ouyang et al. 2016; Wang et al. 2016). Changes in dominant plant species in ungrazed and grazed grasslands (i.e., L. chinensis replaced by C. duriuscula) could lead to higher 15NO3− recovery in the grazed grassland. More NO3− than NH4+ taken up by plants under clipping and shading treatments in grazed grassland could also be ascribed to changes in the species composition caused by grazing. Concretely, grazing promotes the growth of C. duriuscula (grazing-resistant, root-developed) and reduces the relative biomass of L. chinensis. This sedge could preferentially take up NO3− and increase the 15N recovery, but this needs further investigation. Besides, a substantial change in C allocation to belowground in grazed and ungrazed grasslands could be another reason for the differences in 15N uptake by plants.
Compared with the control treatment in both grazed and ungrazed grasslands, clipping and shading treatments reduced 15N recovery by plants (Fig. 5), confirming our second hypothesis. The strong reduction of leaves or light intensity after clipping or shading led to very low photosynthesis. Consequently, the energy used to pump up nutrients was minimal and resulted in the lower 15N recovery (Shahzad et al. 2012).
Overall, our results demonstrate that plant C input alters the uptake of N and its retention by plants in temperate grasslands. Grazing increases C input to the soil and promotes plant N acquisition, thereby compensating for the loss of C from the aboveground parts and increasing resistance to grazing due to the shift in plant dominance.
Conclusions
In temperate grasslands, the decrease in N availability in grazed grassland is mainly due to the reduction of SOM mineralization caused by animal trampling and enhanced transpiration caused by a reduction in vegetation coverage. Consequently, the growth of plants in the grazed grassland is more N limited compared with plants in the ungrazed grassland. Moreover, plants take up more N (NH4+ + NO3−) for root compensatory growth to offset the loss of stems and leaves on the ground in the grazed grassland. The effects of grazing on 15N-NH4+ and 15N-NO3− recovery in plants largely depend on soil NH4+/NO3− ratio and changes in plant species composition. Clipping and shading reduce N uptake by plants through decreasing the energy supply for the uptake of mineral N. This study only considered N retention by plants, N retention in SOM and microbial biomass should also be taken into account in future studies to understand the fate of N in temperate grasslands. In addition, the N uptake strategies of dominant species (L. chinensis and C. duriuscula) in grazed and ungrazed grasslands need to be investigated to understand the effects of grazing and clipping/shading treatments on N uptake by dominant plant species.
References
Bakker ES, Olff H, Boekhoff M, Gleichman JM, Berendse F (2004) Impact of herbivores on nitrogen cycling: contrasting effects of small and large species. Oecologia 138(1):91–101
Brück H, Guo S (2006) Influence of N form on growth photosynthesis of Phaseolus vulgaris L. plants. J Plant Nutr Soil Sci 169(6):849–856
Carrera AL, Bertiller MB, Larreguy C (2008) Leaf litterfall, fine-root production, and decomposition in shrublands with different canopy structure induced by grazing in the Patagonian Monte, Argentina. Plant Soil 311(1–2):39–50
Dahl M, Deyanova D, Lyimo LD, Näslund J, Samuelsson GS, Mtolera MS, Björk M, Gullström M (2016) Effects of shading and simulated grazing on carbon sequestration in a tropical seagrass meadow. J Ecol 104(3):654–664
Detling JK, Dyer MI, Winn DT (1979) Net photosynthesis, root respiration, and regrowth of Bouteloua gracilis following simulated grazing. Oecologia 41(2):127–134
Díaz S, Noy-Meir I, Cabido M (2001) Can grazing response of herbaceous plants be predicted from simple vegetative traits? J Appl Ecol 38(3):497–508
Díaz S, Lavorel S, McIntyre SUE et al (2007) Plant trait responses to grazing–a global synthesis. Glob Chang Biol 13(2):313–341
Dijkstra FA, Pendall E, Mosier AR, King JY, Milchunas DG, Morgan JA (2008) Long-term enhancement of N availability and plant growth under elevated CO2 in a semi-arid grassland. Funct Ecol 22(6):975–982
Distelfeld A, Avni R, Fischer AM (2014) Senescence, nutrient remobilization, and yield in wheat and barley. J Exp Bot 65(14):3783–3798
Du R, Lu D, Wang G (2006) Diurnal, seasonal, and inter-annual variations of N2O fluxes from native semi-arid grassland soils of inner Mongolia. Soil Biol Biochem 38(12):3474–3482
Ferraro DO, Oesterheld M (2002) Effect of defoliation on grass growth. A quantitative review. Oikos 98(1):125–133
Foley JA, Ramankutty N, Brauman KA, Cassidy ES, Gerber JS, Johnston M, Mueller ND, O’Connell C, Ray DK, West PC, Balzer C, Bennett EM, Carpenter SR, Hill J, Monfreda C, Polasky S, Rockström J, Sheehan J, Siebert S, Tilman D, Zaks DPM (2011) Solutions for a cultivated planet. Nature 478(7369):337–342
Frank DA, Kuns MM, Guido DR (2002) Consumer control of grassland plant production. Ecology 83(3):602–606
Gessler A, Schneider S, Von Sengbusch D et al (1998) Field and laboratory experiments on net uptake of nitrate and ammonium by the roots of spruce (Picea abies) and beech (Fagus sylvatica) trees. New Phytol 138(2):275–285
Golodets C, Kigel J, Sternberg M (2010) Recovery of plant species composition and ecosystem function after cessation of grazing in a mediterranean grassland. Plant Soil 329(1–2):365–378
Guo S, Brück H, Sattelmacher B (2002) Effects of supplied nitrogen form on growth and water uptake of french bean (Phaseolus vulgaris L.) plants: nitrogen form and water uptake. Plant Soil 239(2):267–275
Hamilton EW, Frank DA (2001) Can plants stimulate soil microbes and their own nutrient supply? Evidence from a grazing tolerant grass. Ecology 82(9):2397–2402
Houlton BZ, Sigman DM, Schuur EAG, Hedin LO (2007) A climate-driven switch in plant nitrogen acquisition within tropical forest communities. P Natl Acad Sci USA 104(21):8902–8906
Hui D, Jackson RB (2006) Geographical and interannual variability in biomass partitioning in grassland ecosystems: a synthesis of field data. New Phytol 169(1):85–93
IUSS Working Group WRB (2014) World reference base for soil resources 2014. International soil classification system for naming soils and creating legends for soil maps. World Reference Base for soil resources 2014: international soil classification system for naming soils and creating legends for soil maps
Jaramillo VJ, Detling JK (1988) Grazing history, defoliation, and competition: effects on shortgrass production and nitrogen accumulation. Ecology 69(5):1599–1608
Kuzyakov Y, Xu X (2013) Competition between roots and microorganisms for nitrogen: mechanisms and ecological relevance. New Phytol 198(3):656–669
Lavado RS, Taboada MA (1988) Water, salt and sodium dynamics in a Natraquoll in Argentina. Catena 15(6):577–594
Lebauer DS, Treseder KK (2008) Nitrogen limitation of net primary productivity in terrestrial ecosystems is globally distributed. Ecology 89(2):371–379
Leriche H, Le Roux X, Desnoyers F, Benest D, Simioni G, Abbadie L (2003) Grass response to clipping in an African savanna: testing the grazing optimization hypothesis. Ecol Appl 13(5):1346–1354
Li J, MaXF GP, Bao GZ (2007) Studies on clonal plasticity of Leymus chinensis from habitats with different degree of salt and alkali in the Songnen plain. J Shenyang Normal Univ 25:506–509
Li X, Fu H, Li X, Guo D, Dong X, Wan C (2008) Effects of land-use regimes on carbon sequestration in the loess plateau, northern China. New Zeal J Agr Res 51(1):45–52
Li X, Zhang C, Fu H, Guo D, Song X, Wan C, Ren J (2013) Grazing exclusion alters soil microbial respiration, root respiration and the soil carbon balance in grasslands of the loess plateau, northern China. Soil Sci Plant Nutr 59(6):877–887
Liu XR, Dong YS, Ren JQ, Li SG (2010) Drivers of soil net nitrogen mineralization in the temperate grasslands in Inner Mongolia, China. Nutr Cycl Agroecosyst 87(1):59–69
Liu Q, Qiao N, Xu X, Xin X, Han JY, Tian Y, Ouyang H, Kuzyakov Y (2016) Nitrogen acquisition by plants and microorganisms in a temperate grassland. Sci Rep 6:22642
Lotze HK, Schramm W (2000) Ecophysiological traits explain species dominance patterns in macroalgal blooms. J Phycol 36(2):287–295
Masclaux-Daubresse C, Daniel-Vedele F, Dechorgnat J, Chardon F, Gaufichon L, Suzuki A (2010) Nitrogen uptake, assimilation and remobilization in plants: challenges for sustainable and productive agriculture. Ann Bot 105(7):1141–1157
McNaughton SJ, Ruess RW, Seagle SW (1988) Large mammals and process dynamics in African ecosystems. BioScience 38(11):794–800
McNaughton SJ, Banyikwa FF, McNaughton MM (1997) Promotion of the cycling of diet-enhancing nutrients by African grazers. Science 278(5344):1798–1800
Meng X, Li X, Xin X, Zhou Y (2009) Study on community characteristics and α diversity under different grazing intensity on Leymus chinensis (Trin.) Tzvel. Meadow steppe of Hulunbeier. Acta Agrestia Sinica 17(2):239–244
Nowak RS, Caldwell MM (1984) A test of compensatory photosynthesis in the field: implications for herbivory tolerance. Oecologia 61(3):311–318
Oesterheld M, Mcnaughton SJ (1991) Effect of stress and time for recovery on the amount of compensatory growth after grazing. Oecologia 85(3):305–313
Ouyang S, Tian Y, Liu Q, Zhang L, Wang R, Xu X (2016) Nitrogen competition between three dominant plant species and microbes in a temperate grassland. Plant Soil 408(1–2):121–132
Owen AG, Jones DL (2001) Competition for amino acids between wheat roots and rhizosphere microorganisms and the role of amino acids in plant N acquisition. Soil Biol Biochem 33(4–5):651–657
Patton BD, Dong X, Nyren PE, Nyren A (2007) Effects of grazing intensity, precipitation, and temperature on forage production. Rangel Ecol Manag 60(6):656–665
Piñeiro G, Paruelo JM, Jobbágy EG, Jackson RB, Oesterheld M (2009) Grazing effects on belowground C and N stocks along a network of cattle exclosures in temperate and subtropical grasslands of South America. Glob Biogeochem Cycles 23(2)
Quested H, Eriksson O, Fortunel C, Garnier E (2007) Plant traits relate to whole-community litter quality and decomposition following land use change. Funct Ecol 21(6):1016–1026
Ren H, Schönbach P, Wan H, Gierus M, Taube F (2012) Effects of grazing intensity and environmental factors on species composition and diversity in typical steppe of Inner Mongolia, China. PLoS One 7(12):e52180
Rossignol N, Bonis A, Bouzillé JB (2006) Consequence of grazing pattern and vegetation structure on the spatial variations of net N mineralisation in a wet grassland. Appl Soil Ecol 31(1–2):62–72
Schimel JP, Bennett J (2004) Nitrogen mineralization: challenges of a changing paradigm. Ecology 85(3):591–602
Schmitt A, Pausch J, Kuzyakov Y (2013) Effect of clipping and shading on C allocation and fluxes in soil under ryegrass and alfalfa estimated by 14C labelling. Appl Soil Ecol 64:228–236
Schönbach P, Wan H, Gierus M, Bai Y, Müller K, Lin L, Susenbeth A, Taube A (2011) Grassland responses to grazing: effects of grazing intensity and management system in an Inner Mongolian steppe ecosystem. Plant Soil 340(1–2):103–115
Semmartin M, Oesterheld M (2001) Effects of grazing pattern and nitrogen availability on primary productivity. Oecologia 126(2):225–230
Semmartin M, Garibaldi LA, Chaneton EJ (2008) Grazing history effects on above- and below-ground litter decomposition and nutrient cycling in two co-occurring grasses. Plant Soil 303(1–2):177–189
Shahzad T, Chenu C, Repinçay C, Mougin C, Ollier JL, Fontaine S (2012) Plant clipping decelerates the mineralization of recalcitrant soil organic matter under multiple grassland species. Soil Biol Biochem 51:73–80
Shan Y, Chen D, Guan X, Zheng S, Chen H, Wang M, Bai Y (2011) Seasonally dependent impacts of grazing on soil nitrogen mineralization and linkages to ecosystem functioning in Inner Mongolia grassland. Soil Biol Biochem 43(9):1943–1954
Smith P (2014) Do grasslands act as a perpetual sink for carbon? Glob Chang Biol 20(9):2708–2711
Soil Survey Staff (2003) Keys to soil taxonomy. Department of agriculture: Natural resources conservation service
Sun Y, Xu XL, Kuzyakov Y (2014) Mechanisms of rhizosphere priming effects and their ecological significance. Chin J Plant Ecol 38(1):62–75
Sun Y, Schleuss PM, Pausch J, Xu XL, Kuzyakov Y (2018) Nitrogen pools and cycles in Tibetan Kobresia pastures depending on grazing. Biol Fertil Soils 54(5):569–581
Turner CL, Seastedt TR, Dyer MI (1993) Maximization of aboveground grassland production: the role of defoliation frequency, intensity, and history. Ecol Appl 3(1):175–186
Van Reeuwijk LP (1993) Procedures for soil analysis (No. 9). International Soil Reference and Information Centre
Wan S, Luo Y (2003) Substrate regulation of soil respiration in a tallgrass prairie: results of a clipping and shading experiment. Glob Biogeochem Cycles 17(2)
Wang R, Gao Q, Chen Q (2003) Effects of climatic change on biomass and biomass allocation in Leymus chinensis (Poaceae) along the North-East China transect (NECT). J Arid Environ 54(4):653–665
Wang C, Wan S, Xing X, Zhang L, Han X (2006) Temperature and soil moisture interactively affected soil net N mineralization in temperate grassland in Northern China. Soil Biol Biochem 38(5):1101–1110
Wang C, Han X, Xing X (2010) Effects of grazing exclusion on soil net nitrogen mineralization and nitrogen availability in a temperate steppe in northern China. J Arid Environ 74(10):1287–1293
Wang R, Tian Y, Ouyang S, Xu X, Xu F, Zhang Y (2016) Nitrogen acquisition strategies used by Leymus chinensis and Stipa grandis in temperate steppes. Biol Fert Soils 52(7):951–961
Wei J, Liu W, Wan H, Cheng J, Li W (2016) Differential allocation of carbon in fenced and clipped grasslands: a 13C tracer study in the semiarid Chinese loess plateau. Plant Soil 406(1–2):251–263
Xu XL, Ouyang H, Pei ZY, Zhou CP (2003) Fate of 15N labeled nitrate and ammonium salts added to an alpine meadow in the Qinghai-Xizang plateau. China Acta Bot Sin 45(3):276–281
Xu XL, Ouyang H, Pei ZY, Zhou CP (2004) Long-term partitioning of ammonium and nitrate among different components in an alpine meadow ecosystem. Acta Bot Sin 46(3):279–283
Xu XL, Ouyang H, Cao G, Richter A, Wanek W, Kuzyakov Y (2011) Dominant plant species shift their nitrogen uptake patterns in response to nutrient enrichment caused by a fungal fairy in an alpine meadow. Plant Soil 341(1–2):495–504
Zhang L, Unteregelsbacher S, Hafner S, Xu XL, Schleuss PM, Miehe G, Kuzyakov Y (2017) Fate of organic and inorganic nitrogen in crusted and non-crusted Kobresia grasslands. Land Degrad Dev 28(1):166–174
Zhao W, Chen SP, Lin GH (2008) Compensatory growth responses to clipping defoliation in Leymus chinensis (Poaceae) under nutrient addition and water deficiency conditions. Plant Ecol 196(1):85–99
Zheng SX, Ren HY, Lan ZC, Li WH, Wang KB, Bai YF (2010) Effects of grazing on leaf traits and ecosystem functioning in Inner Mongolia grasslands: scaling from species to community. Biogeosciences 7(3):1117–1132
Zheng S, Lan Z, Li W, Shao R, Shan Y, Wan H, Taube F, Bai Y (2011a) Differential responses of plant functional trait to grazing between two contrasting dominant C3 and C4 species in a typical steppe of Inner Mongolia, China. Plant Soil 340(1–2):141–155
Zheng Y, Mai B, Wu R, Feng Y, Sofo A, Ni Y, Sun J, Li J, Xu J (2011b) Acclimation of winter wheat (Triticum aestivum, cv. Yangmai 13) to low levels of solar irradiance. Photosynthetica 49(3):426–434
Zong N, Shi PL, Song ML (2012) Chipping alters the response of biomass allocation pattern under nitrogen addition in an alpine meadow on the Tibetan plateau. J Nat Resour 27(10):1696–1707
Acknowledgements
This study was supported by the National Key R&D Program of China (2017YFA0604802) and the National Natural Science Foundation of China (31470560 and 41601318). We thank Dr. Yanjiu Liu for his help in the field and statistics. We also thank to the Hulunbeier Grassland Ecosystem Observation and Research Station to provide us help in the field work.
Author information
Authors and Affiliations
Corresponding authors
Additional information
Responsible Editor: Feike A. Dijkstra.
Rights and permissions
About this article
Cite this article
Pang, R., Sun, Y., Xu, X. et al. Effects of clipping and shading on 15NO3− and 15NH4+ recovery by plants in grazed and ungrazed temperate grasslands. Plant Soil 433, 339–352 (2018). https://doi.org/10.1007/s11104-018-3844-x
Received:
Accepted:
Published:
Issue Date:
DOI: https://doi.org/10.1007/s11104-018-3844-x