Abstract
Purpose
To investigate whether multiphasic MDCT enhancement profiles can help to identify PTEN expression in clear cell renal cell carcinomas (ccRCCs). Lack of PTEN expression is associated with worsened overall survival, a more advanced Fuhrman grade, and a greater likelihood of lymph mode metastasis.
Methods
With IRB approval for this retrospective study, we derived a cohort of 103 histologically proven ccRCCs with preoperative 4-phase renal mass MDCT from 2001–2013. Following manual segmentation, a computer-assisted detection algorithm selected a 0.5-cm-diameter region of maximal attenuation within each lesion in each phase; a 0.5-cm-diameter region of interest was manually placed on uninvolved renal cortex in each phase. The relative attenuation of each lesion was calculated as [(Maximal lesion attenuation − cortex attenuation)/cortex attenuation] × 100. Absolute and relative attenuation in each phase were compared using t tests. The performance of multiphasic enhancement in identifying PTEN expression was assessed with logistic regression analysis.
Results
PTEN-positive and PTEN-negative ccRCCs both exhibited peak enhancement in the corticomedullary phase. Relative corticomedullary phase attenuation was significantly greater for PTEN-negative ccRCCs in comparison to PTEN-positive ccRCCs (33.7 vs. 9.5, p = 0.03). After controlling for lesion stage and size, relative corticomedullary phase attenuation had an accuracy of 84% (86/103), specificity of 100% (84/84), sensitivity of 11% (2/19), positive predictive value of 100% (2/2), and negative predictive value of 83% (84/101) in identifying PTEN expression.
Conclusion
Relative corticomedullary phase attenuation may help to identify PTEN expression in ccRCCs, if validated prospectively.
Similar content being viewed by others
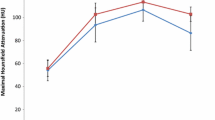
Explore related subjects
Discover the latest articles, news and stories from top researchers in related subjects.Avoid common mistakes on your manuscript.
An estimated 210,000 new cases of renal cell carcinoma (RCC) are diagnosed annually worldwide, accounting for nearly 2% of all adult cancers globally [1]. Within the International Society of Urological Pathology Vancouver classification [2], the most common histologic subtypes of renal cell carcinoma are clear cell, papillary, and chromophobe, accounting for 70%–80%, 14%–17%, and 4%–8% of RCCs, respectively [3, 4]. Of these common subtypes, clear cell RCC has the worst prognosis with a 5-year survival rate of 44%–69%, accounting for 94% of metastatic RCC [5,6,7,8,9]. Postoperatively determined pathologic stage and grade have been traditionally utilized to predict patient prognosis in RCC. However, these methods are invasive and may be unreliable due to heterogeneity within patient groups. As an alternative to the traditional approach, molecular biomarker analyses have recently been studied to address these shortcomings.
Identifying imaging biomarkers to predict specific gene and protein expression has the potential to be of great clinical value. Molecular analyses are becoming an increasingly important part of patient care, and imaging studies will soon need to provide information beyond the size and location of individual lesions. Within this new paradigm of personalized medicine, imaging features may help predict or correlate with a variety of histologic, cytologic, and molecular characteristics of individual lesions and may potentially help to guide therapy.
Multiple recent studies have shown that the magnitude of enhancement on multiphasic multidetector computed tomography (MDCT) can help distinguish clear cell RCC from other common RCC subtypes (papillary and chromophobe) and common benign mimics (oncocytomas and lipid-poor angiomyolipomas) [10,11,12,13,14,15,16,17]. Clear cell RCCs typically exhibit peak enhancement in the corticomedullary phase with peak enhancement that is significantly greater than that of other solid renal cortical tumors [10,11,12,13,14,15,16,17]. However, there are currently no published imaging biomarkers to predict the expression of phosphatase and tension homolog (PTEN) in clear cell RCCs. PTEN is a prognostically significant molecular marker in RCC. Lack of PTEN expression in RCC has been associated with worsened overall survival, a more advanced Fuhrman grade, and a greater likelihood of lymph node metastasis [18]. The purpose of this study was to investigate whether multiphasic MDCT enhancement can help to identify PTEN expression in clear cell RCCs.
Materials and methods
Patients
With Institutional Review Board approval for this Health Insurance Portability and Accountability Act-compliant retrospective study and a waiver of informed consent, we queried our institution’s pathology database to derive all histologically proven cases of clear cell RCC from December 2001 to August 2013. Of the approximately 1900 histologically proven cases of clear cell RCC obtained in this query, approximately 1770 lesions were excluded due to a lack of a preoperative dedicated four-phase renal mass protocol MDCT performed at our institution. An additional 31 cases were excluded due to a lack of availability of tissue specimens for immunohistochemical staining. This resulted in a cohort of 103 clear cell RCCs, representing 98 unique patients. Five patients had two lesions each. The mean time from multiphasic MDCT imaging to nephrectomy or biopsy was 66 days. The mean age at the time of nephrectomy or biopsy was 62 years, as shown in Table 1. Histopathology was obtained from partial nephrectomy [45 specimens (44%)], radical nephrectomy [31 specimens (30%)], and percutaneous 20-gauge core needle biopsy [27 specimens (26%)]. For tumors that were diagnosed at partial or radical nephrectomy, the T stage of each lesion was obtained by reviewing pathology reports for the resected specimens. For tumors that were diagnosed at percutaneous core needle biopsy, the T stage of each lesion was approximated based upon preoperative MDCT.
MDCT examination
All CT examinations were performed at our institution using multidetector row CT [primarily 64 (2005–present) or 16-detector (2003–2008) row helical scanners (Sensation 64 or Sensation 16, Siemens Medical Solutions, Erlangen, Germany), or 4-detector (2000–2003) scanners (Lightspeed Qx/i, GE Medical Systems, Milwaukee, WI)]. Images were obtained with the patient breath holding with the following parameters: 120 kVP, variable mA, section thickness interval of 3 mm. A 4-phase renal mass protocol MDCT was performed for each patient. Each patient first underwent an unenhanced scan of the abdomen from the top of the diaphragm to the iliac crest. Then, following the injection of 100–150 mL of non-ionic intravenous iohexol based upon patient weight (Omnipaque 350, GE Healthcare, Milwaukee, WI) with a power injector at 3–4 mL per second, a bolus tracking algorithm (CareBolus, Siemens Medical Solutions; SmartPrep, GE Medical Systems) was utilized. For bolus tracking, a region of interest was placed in the thoracoabdominal aorta junction with a trigger set at 150 HU followed by imaging in the corticomedullary (40 s after the threshold is reached), nephrographic (90 s after the threshold is reached), and excretory (8 min after the threshold is reached) phases. Images were then sent to our picture archiving and communications system (Centricity, GE Medical Systems, Milwaukee, WI) for interpretation.
CT image analyses
Each lesion was segmented in its entirety on axial images in all phases using proprietary non-commercial Food and Drug Administration (FDA) 510(k) cleared software to create a three-dimensional contour. Lesion contours were drawn slightly internal to the lesion’s outer margin preclude partial volume averaging artifact [12, 19,20,21,22]. Each lesion contour was reviewed by two abdominal fellowship-trained genitourinary radiologists with 10 (MD) and 19 years (SSR) of experience, who were blinded to all clinical, histopathologic, and immunohistochemical findings. If there was any disagreement between the two readers regarding the appropriate placement of a contour, the case was discussed by both readers until a consensus was reached. A computer-assisted detection (CAD) algorithm utilized each whole lesion three-dimensional contour to select a 0.5-cm-diameter circular region of maximal attenuation within each lesion in each of the four phases. Pixels with attenuation > 300 Hounsfield units (HU) were excluded to exclude calcifications. Each CAD-selected region of maximal attenuation was reviewed by both readers for appropriate placement. A similar small region of interest approach to quantifying lesion attenuation has been utilized in prior studies examining multiphasic MDCT enhancement as a means of differentiating clear cell RCC from papillary RCC, chromophobe RCC, oncocytoma, and lipid-poor angiomyolipoma [12], identifying prognostically significant cytogenetic abnormalities in clear cell RCC (gain of chromosome 12, gain of chromosome 20, loss of the Y chromosome, and loss of chromosome 8p) [21, 23,24,25], identifying the level of carbonic anhydrase-IX expression in clear cell RCC [26], and differentiating type 1 from type 2 papillary RCC [20]. A similar approach has also been used to investigate multiphasic MR enhancement as a means of differentiating clear cell RCC from papillary RCC, chromophobe RCC, and oncocytoma [22]. For each lesion, a 0.5-cm-diameter region of interest was manually placed on uninvolved renal cortex in each phase, and these regions of interest were also reviewed by both readers for appropriate placement. In each phase, the relative attenuation (compared to uninvolved renal cortex) of each lesion was calculated as [(Maximal lesion attenuation − cortex attenuation)/cortex attenuation] × 100. Maximal lesion attenuation refers to the mean attenuation of the 0.5-cm circular region of maximal lesion attenuation. Cortex attenuation refers to the mean attenuation of the 0.5-cm circular region of interest manually placed on uninvolved renal cortex. Lesion size was determined by identifying the maximal lesion diameter on axial images.
Immunohistochemistry for PTEN
Surgical pathology specimens obtained from nephrectomy or percutaneous core needle biopsy were stained with a mouse monoclonal anti-PTEN antibody CM 278 (Biocare Medical, Pacheco, CA). Immunohistochemical staining was assessed by a fellowship-trained genitourinary pathologist (AS) to determine the presence of positively staining cells in each specimen.
Statistical analyses
To compare the maximal and relative attenuation values between PTEN-positive and PTEN-negative clear cell RCCs, t tests were performed using the hierarchical testing of the fixed sequence procedure by the order of contrast-enhanced phases (corticomedullary, nephrographic, and excretory). A multiple test procedure was controlled using a family-wise error rate at 5% [27]. The corticomedullary phase, which is the first contrast-enhanced phase acquired in our MDCT protocol, was placed first in the hierarchical testing as prior studies have shown that this phase is most important in differentiating clear cell RCC from other common RCC subtypes (papillary and chromophobe) and oncocytoma [10,11,12, 22] and in identifying prognostically significant chromosomal abnormalities in clear cell RCC [23]. Logistic regression models were constructed to evaluate the performance of attenuation parameters in identifying PTEN expression, after controlling for lesion size and stage. To account for potential clustering effects, the data were also analyzed while including only one lesion per patient in each group. For patients with multiple lesions, the representative lesion included was selected using a random number generator. Analyses were performed using SPSS 23 for Windows (IBM Corp, Armonk, NY).
Results
Lesions
Of the 103 clear cell RCCs in our study, immunohistochemical staining revealed that 19 lesions (18%) were PTEN positive and 84 lesions (82%) were PTEN negative. Baseline characteristics for lesions in each group are provided in Table 1. There was no significant difference in mean lesion size between PTEN-positive and PTEN-negative clear cell RCCs (4.2 cm vs. 4.0 cm, p = 0.85). The majority of PTEN-positive and PTEN-negative clear cell RCCs (68% in the PTEN-positive cohort and 76% in the PTEN-negative cohort) were either stage T1a or T1b at the time of diagnosis.
Multiphasic attenuation parameters
Both PTEN-positive and PTEN-negative clear cell RCCs exhibited peak maximal attenuation in the corticomedullary phase (Fig. 1). There was no significant difference in the maximal attenuation of PTEN-positive and PTEN-negative clear cell RCCs in the corticomedullary phase (Table 2); no further testing (in the nephrographic or excretory phases) was reported by the fixed sequence procedure. When including only one lesion per group per patient, relative corticomedullary phase attenuation (compared to uninvolved renal cortex) was significantly greater for PTEN-negative clear cell RCCs in comparison to PTEN-positive clear cell RCCs (35.5 vs. 9.5, p = 0.02, Table 3). However, no significant differences were found in nephrographic phase (p = 0.98), and no further testing was reported by the fixed sequence procedure. When including all lesions in the study cohort, the relative corticomedullary phase attenuation (compared to uninvolved renal cortex) was significantly greater for PTEN-negative clear cell RCCs in comparison to PTEN-positive clear cell RCCs (33.7 vs. 9.5, p = 0.03, Fig. 2, Table 4). No significant differences were found in nephrographic phase (p = 0.93), and no further testing was reported by the fixed sequence procedure. CT images of typical lesions in the PTEN-positive and PTEN-negative subcohorts are presented in Fig. 3. Immunohistochemically stained slides of typical lesions in the PTEN-positive and PTEN-negative subcohorts are presented in Fig. 4.
Corticomedullary phase axial MDCT images of a PTEN-positive clear cell RCC in a 71-year-old woman and a PTEN-negative clear cell RCC in a 67-year-old woman. Representative CAD-selected regions of maximal attenuation are shown in blue. A PTEN-positive clear cell RCC with a maximal corticomedullary phase attenuation of 141 HU. B PTEN-negative clear cell RCC with a maximal corticomedullary attenuation of 211 HU
Logistic regression modeling
A logistic regression model was constructed to evaluate the performance of relative corticomedullary phase attenuation in identifying PTEN expression in clear cell RCCs, after controlling for lesion size and lesion stage. After controlling for these variables, relative corticomedullary phase attenuation had an odds ratio of 0.985 (p = 0.06). The model (with a classification cut-off of 0.5) had an accuracy of 84% (86/103), specificity of 100% (84/84), sensitivity of 11% (2/19), positive predictive value of 100% (2/2), and negative predictive value of 83% (84/101) in differentiating PTEN-positive from PTEN-negative clear cell RCCs.
Discussion
Consistent with prior published studies evaluating the multiphasic MDCT enhancement of renal tumors, clear cell RCCs in our study cohort typically exhibited peak enhancement in the corticomedullary phase [10,11,12,13,14,15,16,17]. In this current study, we found that relative corticomedullary phase attenuation (compared to uninvolved cortex) may help to identify PTEN expression in clear cell RCCs. PTEN-positive clear cell RCCs had a significantly lower relative corticomedullary phase attenuation than PTEN-negative clear cell RCCs. In a logistic regression model that controlled for lesion size and lesion stage, relative corticomedullary phase attenuation had an accuracy of 84% (86/103), specificity of 100% (84/84), sensitivity of 11% (2/19), positive predictive value of 100% (2/2), and negative predictive value of 83% (84/101) in differentiating PTEN-positive from PTEN-negative clear cell RCCs.
PTEN is a prognostically significant molecular marker in RCC. It is a tumor suppressor that downregulates several important signal transduction pathways, including the hypoxia-inducible factor 1-α (HIF-1α), PI3 kinase, and mitogen-activated protein (MAP) kinase pathways. Lack of PTEN expression in RCC has been associated with worsened clinical outcomes and worsened overall survival rates [18]. PTEN-negative RCCs typically have a more advanced Fuhrman grade and a greater likelihood of lymph mode metastasis than PTEN-positive RCCs [18]. Additionally, prior studies have shown that the loss of PTEN expression is negatively correlated with clinical response to anti-vascular endothelial growth factor (VEGF) and anti-epidermal growth factor (EGF) therapies [28]. Tumor resistance to anti-VEGF therapies is thought to be due to a switch from VEGF-dependent or EGF-dependent angiogenesis to HIF-1α-dependent and PI3 kinase-dependent angiogenesis [28]. Furthermore, given the importance of PTEN in a variety of signal transduction pathways, microRNA therapies that target PTEN have been the focus of recent investigations. Liu et al. found that PTEN is a direct target of miR-193a-3p and that downregulation of miR-193a-3p inhibited cellular proliferation in RCC cell lines [29].
In our cohort, 82% (84/103) of the clear cell RCCs were PTEN negative. This percentage of PTEN-negative RCCs is higher than in other published studies. In the study by Zhu et al., 59% (51/87) of RCCs were PTEN negative [18]. PTEN is a downregulator of the HIF-1α and PI3K signal transduction pathways. The PI3K signaling cascade activates serine/threonine kinase AKT. Activated AKT phosphorylates multiple substrates that regulate numerous cellular functions, including cellular growth, metabolism, and tumorigenesis [30]. Data from the Cancer Genome Atlas have shown that alterations in the PI3K signaling pathway are frequently encountered in clear cell RCC [30]. Additionally, prior studies have shown that the loss of PTEN expression is negatively correlated with clinical response to anti-VEGF and anti-EGF therapies [28]. Because of the prognostic significance of PTEN expression, a simple, non-invasive means of identifying PTEN expression in clear cell RCCs may be helpful in assessing patient prognosis and may potentially guide further management, if validated prospectively.
Our study has several potential limitations. First, while all lesions in our study were evaluated with a dedicated four-phase renal mass protocol MDCT, due to the retrospective nature of this study, we could not account for variability of absolute attenuation within the same phase related to differences among scanners. However, our institution’s four-phase renal mass MDCT protocol was designed to standardize scanning parameters as much as possible. Second, this was a preliminary, discovery-phase study investigating the relationship between PTEN expression and multiphasic MDCT attenuation. Our findings should be validated in an independent testing cohort. Although we do not have a definitive mechanistic explanation for the relationship between PTEN expression and relative corticomedullary phase attenuation, we suspect that this may be related to differences in angiogenesis. PTEN is an important modulator of angiogenesis through its inhibitory effects on the HIF-1α signal transduction pathway, which promotes angiogenesis [28]. Third, clear cell RCCs commonly exhibit intratumoral heterogeneity with regard to genetic mutations and resultant protein expression [31]. Because of this heterogeneity, the degree of PTEN expression may vary at different sites within a particular tumor. In addition, the CAD-selected region of maximal attenuation may not necessarily correspond to the portion of the tumor that was immunohistochemically stained for PTEN expression.
Fourth, T stage assessment differed between lesions that were diagnosed at partial or radical nephrectomy and those that were diagnosed at percutaneous core needle biopsy. The T stage of lesions diagnosed at partial or radical nephrectomy was obtained by reviewing pathology reports for the resected specimens, while the T stage of lesions diagnosed at percutaneous core needle biopsy was approximated based upon preoperative MDCT. Fifth, in a logistic regression model that controlled for lesion size and lesion stage, relative corticomedullary phase attenuation had an accuracy of 84% (86/103) in identifying PTEN expression in clear cell RCCs, which is not yet adequate to definitively guide further treatment. However, our findings suggest that there may be a consistent relationship between PTEN expression in clear cell RCCs and multiphasic MDCT enhancement, if validated in a large prospective trial.
To our knowledge, our study is the first proof of concept study to demonstrate an MDCT-based difference between PTEN-positive and PTEN-negative clear cell RCCs. Because of the prognostic significance of PTEN expression, a non-invasive means of identifying PTEN expression preoperatively may help to guide clinical decision making and patient counseling. Furthermore, with baseline multiphasic MDCT imaging prior to surgery or ablation, new lesions may be non-invasively evaluated to determine if their enhancement profiles are similar to previously treated lesions. Future work is needed to determine whether our findings can be replicated in a prospective cohort and to determine whether our findings can be replicated with gadolinium-enhanced magnetic resonance imaging and contrast-enhanced ultrasound imaging. Future work should also evaluate other imaging techniques, such as positron emission tomography, magnetic resonance diffusion-weighted imaging, and magnetic resonance elastography, to identify additional imaging biomarkers of PTEN expression.
References
Gore ME, Larkin JM (2011) Challenges and opportunities for converting renal cell carcinoma into a chronic disease with targeted therapies. Br J Cancer 104(3):399–406. https://doi.org/10.1038/sj.bjc.6606084
Srigley JR, Delahunt B, Eble JN, et al. (2013) The international society of urological pathology (ISUP) vancouver classification of renal neoplasia. Am J Surg Pathol 37(10):1469–1489. https://doi.org/10.1097/PAS.0b013e318299f2d1
Kovacs G, Akhtar M, Beckwith BJ, et al. (1997) The Heidelberg classification of renal cell tumours. J Pathol 183(2):131–133.https://doi.org/10.1002/(sici)1096-9896(199710)183:22%3C131::aid-path931%3E3.0.co;2-g
Truong LD, Shen SS (2011) Immunohistochemical diagnosis of renal neoplasms. Arch Pathol Lab Med 135(1):92–109. https://doi.org/10.1043/2010-0478-rar.1
Cheville JC, Lohse CM, Zincke H, Weaver AL, Blute ML (2003) Comparisons of outcome and prognostic features among histologic subtypes of renal cell carcinoma. Am J Surg Pathol 27(5):612–624
Hoffmann NE, Gillett MD, Cheville JC, et al. (2008) Differences in organ system of distant metastasis by renal cell carcinoma subtype. J Urol 179(2):474–477. https://doi.org/10.1016/j.juro.2007.09.036
Amin MB, Corless CL, Renshaw AA, et al. (1997) Papillary (chromophil) renal cell carcinoma: histomorphologic characteristics and evaluation of conventional pathologic prognostic parameters in 62 cases. Am J Surg Pathol 21(6):621–635
Beck SD, Patel MI, Snyder ME, et al. (2004) Effect of papillary and chromophobe cell type on disease-free survival after nephrectomy for renal cell carcinoma. Ann Surg Oncol 11(1):71–77
Moch H, Gasser T, Amin MB, et al. (2000) Prognostic utility of the recently recommended histologic classification and revised TNM staging system of renal cell carcinoma: a Swiss experience with 588 tumors. Cancer 89(3):604–614
Young JR, Margolis D, Sauk S, et al. (2013) Clear cell renal cell carcinoma: discrimination from other renal cell carcinoma subtypes and oncocytoma at multiphasic multidetector CT. Radiology 267(2):444–453. https://doi.org/10.1148/radiol.13112617
Lee-Felker SA, Felker ER, Tan N, et al. (2014) Qualitative and quantitative MDCT features for differentiating clear cell renal cell carcinoma from other solid renal cortical masses. AJR 203(5):W516–W524. https://doi.org/10.2214/ajr.14.12460
Coy H, Young JR, Douek ML, et al. (2017) Quantitative computer-aided diagnostic algorithm for automated detection of peak lesion attenuation in differentiating clear cell from papillary and chromophobe renal cell carcinoma, oncocytoma, and fat-poor angiomyolipoma on multiphasic multidetector computed tomography. Abdom Radiol (New York) 42(7):1919–1928. https://doi.org/10.1007/s00261-017-1095-6
Zhang J, Lefkowitz RA, Ishill NM, et al. (2007) Solid renal cortical tumors: differentiation with CT. Radiology 244(2):494–504. https://doi.org/10.1148/radiol.2442060927
Kim JK, Kim TK, Ahn HJ, et al. (2002) Differentiation of subtypes of renal cell carcinoma on helical CT scans. AJR 178(6):1499–1506. https://doi.org/10.2214/ajr.178.6.1781499
Sheir KZ, El-Azab M, Mosbah A, El-Baz M, Shaaban AA (2005) Differentiation of renal cell carcinoma subtypes by multislice computerized tomography. J Urol 174(2):451–455 ((discussion 455)). https://doi.org/10.1097/01.ju.0000165341.08396.a9
Jinzaki M, Tanimoto A, Mukai M, et al. (2000) Double-phase helical CT of small renal parenchymal neoplasms: correlation with pathologic findings and tumor angiogenesis. J Comput Assist Tomogr 24(6):835–842
Ruppert-Kohlmayr AJ, Uggowitzer M, Meissnitzer T, Ruppert G (2004) Differentiation of renal clear cell carcinoma and renal papillary carcinoma using quantitative CT enhancement parameters. AJR 183(5):1387–1391. https://doi.org/10.2214/ajr.183.5.1831387
Zhu C, Wei J, Tian X, Li Y, Li X (2015) Prognostic role of PPAR-γ and PTEN in the renal cell carcinoma. Int J Clin Exp Pathol 8(10):12668–12677
Rosenkrantz AB, Matza BW, Portnoy E, et al. (2014) Impact of size of region-of-interest on differentiation of renal cell carcinoma and renal cysts on multi-phase CT: preliminary findings. Eur J Radiol 83(2):239–244. https://doi.org/10.1016/j.ejrad.2013.10.020
Young JR, Coy H, Douek M, et al. (2017) Type 1 papillary renal cell carcinoma: differentiation from type 2 papillary RCC on multiphasic MDCT. Abdom Radiol (New York) 42(7):1911–1918. https://doi.org/10.1007/s00261-017-1091-x
Young JR, Coy H, Douek M, et al. (2017) Clear cell renal cell carcinoma: identifying the gain of chromosome 12 on multiphasic MDCT. Abdom Radiol (New York) 42(1):236–241. https://doi.org/10.1007/s00261-016-0868-7
Young JR, Coy H, Kim HJ, et al. (2017) Performance of relative enhancement on multiphasic MRI for the differentiation of clear cell renal cell carcinoma (RCC) from papillary and chromophobe RCC subtypes and oncocytoma. AJR 208(4):812–819. https://doi.org/10.2214/ajr.16.17152
Young JR, Margolis D, Sauk S, et al. (2014) Clear cell renal cell carcinoma: multiphasic MDCT enhancement can predict the loss of chromosome 8p. Abdom Imaging 39(3):543–549. https://doi.org/10.1007/s00261-014-0092-2
Young JR, Young JA, Margolis DJ, et al. (2016) Clear cell renal cell carcinoma: identifying the gain of chromosome 20 on multiphasic MDCT. Abdom Radiol (New York) 41(11):2175–2181. https://doi.org/10.1007/s00261-016-0813-9
Young JR, Coy H, Douek M, et al. (2017) Clear cell renal cell carcinoma: identifying the loss of the Y chromosome on multiphasic MDCT. AJR 209(2):333–338. https://doi.org/10.2214/ajr.16.17010
Young JR, Coy H, Kim HJ, et al. (2018) Utility of multiphasic multidetector computed tomography in discriminating between clear cell renal cell carcinomas with high and low carbonic anhydrase-IX expression. Abdom Radiol (New York) . https://doi.org/10.1007/s00261-018-1546-8
Westfall PH, Krishen A (2001) Optimally weighted, fixed sequence and gatekeeper multiple testing procedures. J Stat Plan Inference 99(1):25–40. https://doi.org/10.1016/S0378-3758(01)00077-5
Rodriguez S, Huynh-Do U (2012) The role of PTEN in tumor angiogenesis. J Oncol 2012:11. https://doi.org/10.1155/2012/141236
Liu L, Li Y, Liu S, et al. (2017) Downregulation of miR-193a-3p inhibits cell growth and migration in renal cell carcinoma by targeting PTEN. Tumour Biol 39(6):1010428317711951. https://doi.org/10.1177/1010428317711951
Guo H, German P, Bai S, et al. (2015) The PI3K/AKT pathway and renal cell carcinoma. J Genet Genom 42(7):343–353. https://doi.org/10.1016/j.jgg.2015.03.003
Gerlinger M, Rowan AJ, Horswell S, et al. (2012) Intratumor heterogeneity and branched evolution revealed by multiregion sequencing. N Engl J Med 366(10):883–892. https://doi.org/10.1056/NEJMoa1113205
Acknowledgements
This study was funded by the Society of Abdominal Radiology Howard S. Stern Research Grant. We thank the UCLA Computer Vision and Imaging Biomarker (CVIB) group, including Dr. Jonathan Goldin, Dr. Matthew Brown, Moe Moe Ko, and War War Ko, for their assistance with this study.
Author information
Authors and Affiliations
Corresponding author
Ethics declarations
Conflict of interest
The authors declare that they have no conflicts of interest.
Ethical approval
All procedures performed in studies involving human participants were in accordance with the ethical standards of the institutional and/or national research committee and with the 1964 Helsinki declaration and its later amendments or comparable ethical standards.
Informed consent
The Institutional Review Board waived the requirement of informed consent for this study.
Rights and permissions
About this article
Cite this article
Young, J.R., Coy, H., Kim, H.J. et al. Clear cell renal cell carcinoma: identifying PTEN expression on multiphasic MDCT. Abdom Radiol 43, 3410–3417 (2018). https://doi.org/10.1007/s00261-018-1672-3
Published:
Issue Date:
DOI: https://doi.org/10.1007/s00261-018-1672-3