Abstract
Purpose
Injury or degeneration of the meniscus has been associated with the development of osteoarthritis of the knee joint. Meniscal allograft transplant (MAT) has been shown to reduce pain and restore function in patients who remain symptomatic following meniscectomy. The purpose of this study is to evaluate and compare the three-dimensional (3D) strain in native medial menisci compared to allograft-transplanted medial menisci in both the loaded and unloaded states.
Methods
Ten human cadaveric knees underwent medial MAT, utilizing soft-tissue anterior and posterior root fixation via transosseous sutures tied over an anterolateral proximal tibial cortical bone bridge. The joint was imaged first in the non-loaded state, then was positioned at 5° of flexion and loaded to 1× body weight (650 ± 160 N) during MR image acquisition. Anatomical landmarks were chosen from each image to create a tibial coordinate system, which were then input into a custom-written program (Matlab R2014a) to calculate the 3D strain from the unloaded and loaded marker positions. Six independent strains were obtained: three principal strains and three shearing strains.
Results
No statistically significant difference was found between the middle and posterior strains in the native knee compared to the meniscus allograft. This would suggest that soft-tissue fixation of meniscal allografts results in similar time zero principal and shear strains in comparison to the native meniscus.
Conclusion
These results suggest that time zero MAT performs in a similar manner to the native meniscus. Optimizing MAT strain behavior may lead to potential improvements in its chondroprotective effect.
Similar content being viewed by others
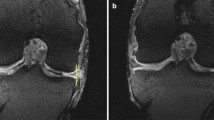
Avoid common mistakes on your manuscript.
Introduction
In the face of irreparable meniscal injury, treatment options include partial or total meniscectomy. As noted by Fairbanks in 1948, meniscectomy causes deleterious effects on the mechanics of the knee joint [5], resulting in pain, swelling, and mechanical symptoms. This is due to disruption of load distribution, increased contact stress, and loss of stability [4, 14]. Studies have shown that total meniscectomy can result in a 14-fold increase in the development of osteoarthritis [8]. This has led to further clinical investigation on the impact of meniscus loss, as well as further study of meniscal repair and rehabilitative techniques to preserve meniscal function [13, 18]. As such, meniscus preservation by means of repair should be attempted where possible, with techniques such as meniscus transplantation considered in applicable cases [13, 14].
Meniscal allograft transplantation (MAT) has been shown to reduce pain and help restore function in patients who are symptomatic following a full or partial meniscectomy [4, 14]. It has been shown that MAT improves symptoms, function, and quality of life at 7–14 years of follow-up [20]; however, there is still a paucity of data regarding the long-term chondroprotective effects of MAT [12, 21].
Three broad categories of surgical technique have been described each utilizing either bone plugs, bone bridges/slots, or soft-tissue fixation only, to attach the meniscus roots to the tibial plateau. To date, there is no consensus as to which technique produces the best clinical results. Cadaveric studies favor bony fixation over suture-only fixation to ensure that tibial contact forces and hoop stresses are recreated as accurately as possible; however, this has only been assessed by measuring meniscus graft extrusion and contact pressures in the tibiofemoral compartment under the meniscus graft [9, 16, 17]. This is not a good measure of in-situ strain within the transplanted tissue itself. To the authors’ knowledge, in-situ strain has never been measured in native and transplanted menisci, particularly under physiologic load. This study, therefore, aims to evaluate and compare the three-dimensional (3D) strain in native medial menisci and in allograft-transplanted medial menisci in both the loaded and the unloaded states utilizing a novel 3D imaging technique. The hypothesis of this study was that MAT can closely recapitulate the in-situ strain distribution of the native meniscus using an all arthroscopic soft-tissue fixation surgical techniques.
Materials and methods
Ten fresh-frozen human cadaveric knees (5 males; mean age 67) were evaluated using a novel MRI-compatible loading device which is capable of positioning and loading cadaveric knee joints using simulated quadriceps and hamstrings forces [7]. This loading device consists of three hydraulic actuators which can position the joint between 0° and 110° of flexion. The knee joint has five degrees of freedom with load applied in a physiologically relevant way by simulating muscle forces of the quadriceps and hamstring. Specimens were prepared for imaging as outlined below, and then, positioned and micro-computed tomography (CT) images were acquired with and without a simulated muscle load applied.
Specimen preparation
The cadaveric knees were prepared as previously described by Gonzalez-Lecuna et al. to enable insertion in the loading device [3]. Eight Teflon beads measuring 0.8 mm in diameter were then inserted in two tetrahedral formations in the middle 1/3 and posterior 1/3 of the native medial meniscus under arthroscopic guidance using an 18G spinal needle. The knee was manually manipulated through ten cycles of flexion and extension prior to testing. The joint was placed into the loading device and set to 5° of flexion using a hand-held goniometer.
MAT surgical technique
Meniscal allografts were sourced from the Musculoskeletal Transplant Foundation (MTF) and size-matched using fluoroscopic tibial plateau coronal width measurements with magnification marker as per the Pollard method [19]. Eight 0.8 mm Teflon beads were inserted in two tetrahedral formations in the middle 1/3 and posterior 1/3 of the medial meniscus allograft using an 18G spinal needle. The native medial meniscus was then removed arthroscopically and replaced with a meniscus allograft utilizing soft-tissue anterior and posterior root fixation with transosseous sutures tied over an anterolateral proximal tibial cortical bone bridge.
Imaging protocol
Micro-CT imaging was acquired using a 154 µm resolution volumetric cone-beam micro-CT scanner (GE Locus Ultra) with an approximately 16 s image acquisition time. The specimen was imaged first in the non-loaded state, following which load was applied to the knee joint via the muscle cables until a joint load of approximately 1× body weight (650 ± 160 N) was reached. Another image was then acquired in the loaded state. The imaging process was then repeated for the knee following MAT.
Meniscus strain measurement
To quantify the strain in the tissue, the centroid 3D coordinates of each marker were recorded using MicroView 3D Image Viewer 2.5.0-2589 [11]. To create a tibial coordinate system, anatomical landmarks were chosen from each image. These landmarks were the center of the medial and lateral femoral contact areas on the tibial plateau and the center most distal point on the tibia. The line from the lateral to medial landmark is the medial–lateral axis (positive medial) and the mid-point between those two points to the distal landmark is the superior–inferior axis (positive superior). A cross product of those two axes determines the anterior–posterior axis. Another cross product of the superior–inferior and anterior–posterior axes is taken to form a new medial lateral axis to ensure that all three axes are orthonormal. Marker coordinates were then transformed into the tibial coordinate system to establish anatomical directions for calculations of strain. These coordinates were then input into a custom-written program (Matlab R2014a) to calculate the three-dimensional strain from the unloaded and loaded marker positions as described by Waldman et al [22]. The markers that formed the vertices of the tetrahedron were non-coplanar and defined a volume no greater than 9 mm3 and no less than 0.1 mm3. Preliminary results showed this to be a reasonable range of volume measurements, above or below which the results became erratic. The tetrahedron must be small enough that the tissue properties can be assumed to be homogeneous throughout [22].
Six independent strains were obtained, three principal strains, and three shearing strains, according to the following equation:
where Δso is the original distance between the markers prior to loading and Δs is the distance between the markers after loading, E is the strain, Δai is the distance from the first marker to the tibial coordinate system, and Δaj is the distance from the second marker to the tibial coordinate system [22]. This equation yields six algebraic equations which can be solved to obtain the six strain components of the symmetric strain tensor.
A separate knee joint (70 years, male) was used to evaluate the repeatability of this method. Five repeated loading trials and image acquisition sequences were conducted on this knee. For the five repeatability trials, a maximum standard deviation of 2.51% was found for all six directions of strain [11].
This laboratory study was performed under the standard Western University institutional approval for the use of anonymous human cadaveric tissue.
Statistical analysis
Ten knees were selected in this study to determine differences in principal strains, based upon a standard number of specimens required for cadaveric testing. Statistical analysis using repeated-measures ANOVA (IBM SPSS Statistics 22) was conducted to determine differences in strain between native and allograft menisci. Significance was assumed to be present for P < 0.05.
Results
Figure 1 shows the average 3D strain found in eight knees for the middle 1/3 of the meniscus for both loaded and unloaded conditions. Figure 2 shows the average 3D strain found in eight knees for the posterior 1/3 of the meniscus for both conditions. Medial–lateral (ML), anterior–posterior (AP), and superior–inferior (SI) principal strains are shown. Directions ML/AP, ML/SI, and AP/SI indicate shearing strains.
Strain profile in the mid-body position of the medial meniscus. a Intact meniscus; b medial MAT using soft-tissue anterior and posterior root fixation via transosseous tunnels tied over a cortical bone bridge. Positive bars represent tensile strain, whereas negative bars represent compressive strains
Strain profile in the posterior horn position of the medial meniscus. a Intact meniscus; b medial MAT using soft-tissue anterior and posterior root fixation via transosseous tunnels tied over a cortical bone bridge. Positive bars represent tensile strain, whereas negative bars represent compressive strains
No statistically significant difference was found between the middle and posterior strains in the native knee compared to the meniscus allograft.
Discussion
The most important finding of this study is that, at time zero, contemporary soft-tissue surgical techniques of MAT result in similar principal and shear strain values to that of native menisci. To our knowledge, this is the first study to quantitatively study regional 3D meniscal strain in human knees under the simulated physiological loading with the purpose of evaluating differences between native menisci and MAT. This could lead to a more standardized method of comparison to elucidate the efficacy of different surgical fixation techniques for MAT, as well as for the development of meniscal prostheses or scaffolds.
The results for the MAT, especially for the middle position, seem to follow the same pattern as the intact meniscus in which the meniscus responds and deforms in response to tibiofemoral loading. This causes hoop stresses [13], which may result in fibers in the ML direction being compressed and fibers in the AP direction being tensioned as the meniscus is mainly anchored at the anterior and posterior horn attachments. The tensile strain in the middle region of the meniscus in the SI direction is accompanied by the compressive strain in the ML direction. In the posterior region of the meniscus, the SI compression is accompanied with tensile strains in the AP direction. These results and the similarities in the strain distributions between intact and medial MAT are corroborated by other research showing positive outcomes with soft-tissue fixation alone [1, 2, 6, 14, 20].
Whilst some biomechanical studies comparing bone and soft-tissue fixation have shown favorable results with bone fixation, clinical studies have shown that both methods alleviate symptoms and produce good mid-to-long-term results [10]. Literature reviews of studies evaluating the different methods have yet to determine which fixation technique is most efficacious [10, 12, 19]. It has been suggested that soft-tissue fixation techniques may be superior to bone fixation techniques in causing less intra-articular damage, as well as the ability to be conducted fully arthroscopically [1]. In addition, the use of bone fixation requires a higher degree of accuracy in size-matching of the allograft. Accurate size-matching is crucial pre-operatively, and an inaccurately size-matched graft may significantly affect the outcomes of the procedure [12]. Establishing which fixation method has the best results could lead to the standardized protocols to help improve outcomes following MAT, and potentially result in greater chondroprotective effects.
The limitations of this study include the limited sample size of knees tested given the variability of the results. However, in-vitro testing is both costly and time-consuming, and this initial study of ten cadaveric knee joints offers an initial baseline of the magnitude and distribution of strain in the native meniscus. There was also variability in the state of the meniscus of each knee; four of the ten knees had noticeable deterioration or tears in the tissue, and the average age of the specimens (67 years) represented an older population. There was also some variability in the location of the markers placed in the meniscus as the markers were placed manually using arthroscopy. However, efforts were made to ensure that the markers were as equidistant as possible and tetrahedron volume was no greater than 9 mm3 to ensure that the tissue contained within the tetrahedron can be assumed homogeneous [22]. The knee flexion angle of 5° was set using a hand-held goniometer which has been shown to have variability of only ± 1.56° [15].
The clinical relevance of this study is that following MAT, utilizing a soft-tissue only fixation technique, time zero-strain behavior of the transplanted tissue behaves in a similar manner to that of the native meniscus. It is unclear what effects cyclic loading over time would have on this model in an in-vivo environment. As such, further work is required to establish what surgical techniques may best recapitulate normal physiological strains in the meniscal allograft over time. This model may assist in determining this information.
Conclusion
A new method of measuring of 3D strain in an in-vitro cadaveric model is presented which can be used to compare differences in surgical fixation techniques for MAT. Strain measurements for medial MAT were found to replicate the range found within the native menisci and no significant differences in the distribution of strain were uncovered.
References
Abat F, Gelber PE, Erquicia JI, Pelfort X, Gonzalez-Lucena G, Monllau JC (2012) Suture-only fixation technique leads to a higher degree of extrusion than bony fixation in meniscal allograft transplantation. Am J Sports Med 40:1591–1596
Abat F, Gelber PE, Erquicia JI, Tey M, Gonzalez-Lucena G, Monllau JC (2013) Prospective comparative study between two different fixation techniques in meniscal allograft transplantation. Knee Surg Sports Traumatol Arthrosc 21:1516–1522
Alentorn-Geli E, Seijas Vazquez R, Garcia Balletbo M, Alvarez Diaz P, Steinbacher G, Cusco Segarra X, Rius Vilarrubia M, Cugat Bertomeu R (2011) Arthroscopic meniscal allograft transplantation without bone plugs. Knee Surg Sports Traumatol Arthrosc 19:174–182
Chen L, Gordon K, Hurtig M (2014) Design and validation of a cadaveric knee joint loading device compatible with magnetic resonance imaging and computed tomography. Med Eng Phys 36:1346–1351
De Coninck T, Huysse W, Verdonk R, Verstraete K, Verdonk P (2013) Open versus arthroscopic meniscus allograft transplantation: magnetic resonance imaging study of meniscal radial displacement. Arthroscopy 29:514–521
Fairbank TJ (1948) Knee joint changes after meniscectomy. J Bone Jt Surg Br 30B:664–670
Gonzalez-Lucena G, Gelber PE, Pelfort X, Tey M, Monllau JC (2010) Meniscal allograft transplantation without bone blocks: a 5- to 8-year follow-up of 33 patients. Arthroscopy 26:1633–1640
Ha JK, Shim JC, Kim DW, Lee YS, Ra HJ, Kim JG (2010) Relationship between meniscal extrusion and various clinical findings after meniscus allograft transplantation. Am J Sports Med 38:2448–2455
Hergan D, Thut D, Sherman O, Day MS (2011) Meniscal allograft transplantation. Arthroscopy 27:101–112
Jones A, Sealey R, Crowe M, Gordon S (2014) Concurrent validity and reliability of the simple goniometer iPhone app compared with the universal goniometer. Physiother Theory Pract 30:512–516
Kolaczek S, Hewison C, Caterine S, Ragbar MX, Getgood A, Gordon KD (2016) Analysis of 3D strain in the human medial meniscus. J Mech Behav Biomed Mater 63:470–475
Lee BS, Kim JM, Kim JM, Kim KA, Bin SI (2015) Patient-related risk factors for the extrusion of lateral meniscal allograft transplants. Arthroscopy 31:699–706
Lubowitz JH, Verdonk PC, Reid JB 3rd, Verdonk R (2007) Meniscus allograft transplantation: a current concepts review. Knee Surg Sports Traumatol Arthrosc 15:476–492
Makris EA, Hadidi P, Athanasiou KA (2011) The knee meniscus: structure-function, pathophysiology, current repair techniques, and prospects for regeneration. Biomaterials 32:7411–7431
Marcacci M, Zaffagnini S, Marcheggiani Muccioli GM, Grassi A, Bonanzinga T, Nitri M, Bondi A, Molinari M, Rimondi E (2012) Meniscal allograft transplantation without bone plugs: a 3-year minimum follow-up study. Am J Sports Med 40:395–403
McDermott ID, Lie DT, Edwards A, Bull AM, Amis AA (2008) The effects of lateral meniscal allograft transplantation techniques on tibio-femoral contact pressures. Knee Surg Sports Traumatol Arthrosc 16:553–560
Myers P, Tudor F (2015) Meniscal allograft transplantation: how should we be doing it? A systematic review. Arthroscopy 31:911–925
Oh KJ, Sobti AS, Yoon JR, Ko YB (2015) Current status of second-look arthroscopy after meniscal allograft transplantation: review of the literature. Arch Orthop Trauma Surg 135:1411–1418
Pollard ME, Kang Q, Berg EE (1995) Radiographic sizing for meniscal transplantation. Arthroscopy 11:684–687
Samitier G, Alentorn-Geli E, Taylor DC, Rill B, Lock T, Moutzouros V, Kolowich P (2015) Meniscal allograft transplantation. Part 2: systematic review of transplant timing, outcomes, return to competition, associated procedures, and prevention of osteoarthritis. Knee Surg Sports Traumatol Arthrosc 23:323–333
Smith NA, Parkinson B, Hutchinson CE, Costa ML, Spalding T (2015) Is meniscal allograft transplantation chondroprotective? A systematic review of radiological outcomes. Knee Surg Sports Traumatol Arthrosc 24:2923–2935
Waldman LK, Fung YC, Covell JW (1985) Transmural myocardial deformation in the canine left ventricle. Normal in vivo three-dimensional finite strains. Circ Res 57:152–163
Acknowledgements
This study was supported by a research grant from the Musculoskeletal Transplant Foundation.
Funding
This study was funded by a research grant from the Musculoskeletal Transplant Foundation (MTF).
Author information
Authors and Affiliations
Corresponding author
Ethics declarations
Conflict of interest
The authors declare that they have no conflict of interest.
Ethical approval
This article does not contain any studies with human participants or animals performed by any of the authors.
Rights and permissions
About this article
Cite this article
Kolaczek, S., Hewison, C., Caterine, S. et al. 3D strain in native medial meniscus is comparable to medial meniscus allograft transplant. Knee Surg Sports Traumatol Arthrosc 27, 349–353 (2019). https://doi.org/10.1007/s00167-018-5075-3
Received:
Accepted:
Published:
Issue Date:
DOI: https://doi.org/10.1007/s00167-018-5075-3