Abstract
Sodium dodecyl sulfate (SDS) is one of the most commonly used detergent, which exhibits excellent biocidal activity against various bacteria and fungi. It is commonly employed in many detergent formulations and is employed for disinfection purposes. It is shown to be toxic to fishes, aquatic animals and is also inhibitory to microbes and cyanobacteria. We had isolated a strain belonging to Pseudomonas aeruginosa N1, from a detergent contaminated pond situated in Varanasi city India, which was able to degrade and metabolize SDS as a source of carbon. In the present investigation, we have studied chemotactic response of this strain towards SDS. The results clearly indicate that this strain showed chemotactic response towards SDS. The nature of chemotaxis was found to be metabolism dependent as glucose grown cells showed a delayed chemotactic response towards SDS. This is first study that reported chemotaxis response for P. aeruginosa towards anionic detergent SDS.
Similar content being viewed by others
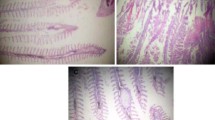
Avoid common mistakes on your manuscript.
Introduction
Chemotaxis is defined as movement of bacteria in response to a chemical gradient [1]. It has been reported that members of the genus Pseudomonas are known to degrade and metabolize a wide variety of xenobiotic compounds and also that they show chemotactic response towards many of these compounds. These include naphthalene [2], chlorinated benzoates and 4-hydroxybenzoate [3, 4], methyl parathion and p-nitrophenol [5], benzene, toluene, and trichloroethylene [6], nitro-benzoates and amino-benzoates [7], and aromatic acids [8]. It is believed that chemotaxis plays an important role in metabolism of xenobiotic compounds as it increases the availability of the chemical to the microbe and plays a positive role in metabolism of xenobiotic compounds [9]. Chemotaxis can be broadly divided in two main types, metabolism dependent and metabolism independent. In metabolism independent chemotaxis, bacteria sense the concentration of chemicals outside the cell with the help of ligand-binding transmembrane receptors known as methyl-accepting chemotaxis proteins (MCPs) [9], binding of ligand to MCP causes flagellar motility [10]. However, in metabolism dependent movement, chemotactic response requires metabolism of the attractant/chemical [11].
Sodium dodecyl sulfate (SDS) is a widely used anionic detergent having excellent biocidal activity against a wide array of microorganisms. It is employed in many industrial and household products [12]. Detergents like other xenobiotic compounds are discharged in water bodies in huge amounts, where they show there detrimental effects [13]. SDS is shown to be toxic to aquatic animals [14] and also to other animals and humans who consume water contaminated with detergents [15]. It is also toxic to microbes [16]. In aquatic systems, it has the tendency to adsorb to surfaces of sediments [17]. Biodegradation of SDS is well studied in Pseudomonas sp. [18]. It is initiated by the enzyme alkyl sulfatase, which catalyses the cleavage of sulphate group forming 1-dodecanol [18]. Which is subsequently oxidized by β-oxidation pathway and is utilized as a carbon source.
We had isolated a strain belonging to Pseudomonas aeruginosa, capable of metabolizing SDS as a sole source of carbon from a detergent contaminated pond situated at Naipura in Varanasi city, India [19]. In the present investigation, we have characterized its chemotactic response towards this detergent. Results indicate that this strain was chemotactic towards SDS in a metabolism dependent manner. To our knowledge, this is the first report of chemotaxis P. aeruginosa towards an anionic detergent SDS.
Materials and Methods
Chemicals and Culture Media
SDS (98.5 % purity) was purchased from Sigma-Aldrich, Inc, St. Louis, MO, USA. The phosphate buffered medium (PBM) contained (g/L): K2HPO4 1.0, KH2PO4 1.0, NH4Cl 1.0, MgSO4·7H2O 0.20, NaCl 0.5, CaCl2 0.02, pH 7.5. The medium also contained trace elements (1 mL of stock) having (g/L): FeCl3·6H2O 0.24, CoCl2·6H2O 0.04, CuSO4·5H2O 0.06, MnCl2·4H2O 0.03, ZnSO4·7H2O 0.31, Na2MoO4·2H2O 0.03. After autoclaving, filter sterlized SDS was added as the sole carbon source at a final concentration of 1 g/L (to be used for growth experiments). Luria–Bertani medium contained (g/L): tryptone, 10.0, NaCl, 5.0, yeast extract, 5.0 and pH was adjusted to 7.0 (to be used for inoculum preparation).
Isolation of SDS Degrading Bacteria
Pseudomonas aeruginosa strain N1 was isolated from a detergent contaminated pond situated in Naipura, Varanasi city, India, by enrichment technique in PBM medium containing SDS as a sole carbon source. This pond was frequently used for washing of clothes [19].
SDS Degradation Study
SDS degradation studies were performed following the method of Chaturvedi and Kumar [19], with minor modification. In biodegradation experiments one flask containing 100 mL PBM was supplemented with SDS (1 g/L) and another flask was supplemented with SDS and glucose (1 g/L) respectively. All the experiments were performed in triplicate and result was depicted as mean ± standard deviation.
Analytical Methods
Growth of bacteria was monitored spectrophotometrically at 600 nm in a UV-Vis spectrophotometer (Genway, Essex, UK) (OD of 0.1 at 600 nm corresponds to 108 cell/mL) [20] and the concentration of SDS was determined by methylene blue active substance method [19].
Preparation of Enzyme Extracts
The bacterial cells were grown in PBM supplemented with SDS. During exponential phase of growth, the cells were harvested by centrifugation of the cultures at 5,000 g for 10 min at 4 °C in a Sorvall RC-5B superspeed refrigerated centrifuge (Sorvall Instruments, DuPont, Wilmington, DE, USA). Cell pellets were washed with 10 mL 10 mM Tris–HCl and was resuspended in 2.5 mL 10 mM Tris–HCl (pH 7.5) (1/100 the original volume). The enzyme extracts were prepared following the method of Ellis et al. [21].
Alkyl Sulfatase Assay and NATIVE PAGE Zymography
Alkyl sulfatase assay and zymography of alkyl sulfatase were carried out following the method of Ellis et al. [21]. Alkyl sulfatase activity in the crude enzyme extracts was performed in triplicate and results were depicted as mean ± standard deviation.
Drop Plate Assay for Chemotaxis
Cells were grown in 50 mL PBM containing SDS (1 g/L). During exponential phase of growth, the cells were centrifuged at 12000 rpm for 10 min at 4 °C in a Sorvall RC-5B superspeed refrigerated centrifuge (Sorvall Instruments, DuPont, Wilmington, DE, USA) and washed twice with 10 mL PBM and re-suspended in 2.0 mL PBM. Drop plate assay medium consisted of PBM, 0.01 % TTC (it is added to visualize bacteria as it is converted to red color farmezan crystals by the action of succinate dehydrogenase in actively growing bacteria), and 2 mL cell suspension in 0.3 % agar. 10 and 30 mg SDS was placed in the center of two petri plates and chemotactic response was observed after 9 h of incubation at 37 °C. In control plates, glucose (10 mg) was placed instead of SDS [22]. To evaluate the response of SDS grown cells towards both SDS and Glucose, SDS grown cells were mixed with semi solid PBM medium and poured in petri plates according to the drop plate assay method. In negative control, heat killed cells (killed by autoclaving at 121 °C for 20 min) was used in place of cell suspension.
Swarm Plate Assay for Chemotaxis
PBM containing 0.16 % agar was autoclaved and cooled to 40–45 °C. To the medium filter sterilized SDS at a final concentration of 1 g/L and filter sterilized TTC at a final concentration of 0.01 % were added The medium was poured and plates were allowed to set. Cells were point inoculated on the centre of plate by means of a sterile tooth pick and incubated at 37 °C. Formation of rings was observed after 9 h of incubation. In control plates, glucose (1 g/L) was added instead of SDS [22].
Results and Discussion
In the present investigation, chemotactic response of P. aeruginosa strain N1 towards SDS has been evaluated. This strain metabolized SDS as a sole carbon source and complete metabolism of 1 g/L SDS was observed after 12 h of incubation (Fig. 1a). Biodegradation of SDS by this isolate was also studied in the presence of glucose, which showed that this strain showed a slow rate of SDS degradation in presence of glucose (Fig. 1b). After 8 h of incubation, it was observed that in flask containing SDS (1 g/L), 0.52 g of SDS was degraded and growth rate was 1.5 × 106 cell/mL/h. Under similar conditions, in flask containing SDS and glucose (1 g/L) each, 0.22 g of SDS was degraded and growth rate was 5.9 × 106 cells/mL/h. This indicates that glucose is the preferred carbon source for the bacterium and therefore it was first metabolized. High rate of SDS degradation was observed in later stages which might be due to low levels of glucose in the culture medium. Similar reports have been made by Abboud et al. [23] in a mixed consortium of Acinetobacter calcoaceticus and Pantoea agglomerans. In their study, significant reduction in SDS degradation was observed in presence of carbon sources such as glucose, sucrose, and maltose respectively. These results do not confirm that alkyl sulfatase, key enzyme in SDS metabolism is inducible in nature. Inducible nature of alkyl sulfatase was confirmed by estimation of alkyl sulfates activity in crude cell extracts of strain N1 grown in presence of SDS and glucose separately. It was observed that during exponential phase of growth, specific enzyme activity was 0.134 ± 0.004 U mg protein−1 in SDS grown cells. However, in glucose grown cells no alkyl sulfatase activity was observed. This result was further confirmed by NATIVE PAGE zymography of crude cells extracts of SDS and glucose grown cells of P. aeruginosa strain N1. It became evident from (Online Resource 1) that in cells grown in presence of SDS a single band of alkyl sulfatase was visible. Whereas, in cells grown in presence of glucose, no band was observed. So, it was concluded that the enzyme alkyl sulfatase was inducible in nature.
Chemotactic response of this isolate towards SDS was studied by swarm plate assay, results indicated that P. aeruginosa strain N1 was chemotactic towards SDS. When this isolate was point inoculated on swarm assay plates, it metabolized SDS and a growth ring was observed. This isolate showed chemotaxis towards glucose also (Online Resource 2). The growth rate of this isolate was different, when grown in minimal medium (PBM) containing glucose and SDS. In presence of SDS, the growth was too slow. The lag phase was long (4 h) as compared to that in glucose (1.5 h) and also the cell yields were also low 3.24 × 107 cells/mL after 16 h of incubation, as compared to 7.56 × 107 cell/mL after 12 h of incubation in presence of glucose. This was also observed in the swarm plate assay. In plates containing glucose, the rings were observed after 7–8 h of inoculation of the culture but in plates containing SDS, the rings were observed after 12 h of inoculation. This was clearly due to different growth rates of this strain in presence of glucose and SDS, respectively. Results observed are in accordance with previous reports, which indicate that biodegradable xenobiotic compounds also act as chemoattractants [2, 5–7]. Many aromatic acids have been reported to be attractants for Pseudomonas putida PRS2000 [8]. Chemotaxis of Burkholderia sp. strain SJ98 towards different NACs has also been reported [22]. It was observed that strain SJ98 was chemotactic towards various substituted nitrophenols [24]. Hawkins and Harwood [25] have reported the chemotaxis of Ralstonia eutropha JMP123 towards 2,4-D.
Chemotactic response was further confirmed by drop plate assay, the results confirmed the chemotactic response of this strain towards SDS (Online Resource 3B). In control plates, glucose grown cells were poured and glucose was placed in the centre. Chemotactic response in control plates was observed after 4 h of incubation (Online Resource 3A), however in test plates containing SDS, the response was visible after 4–5 h, but it was faint as compared to control. After 9 h of incubation, it was clearly visible. However, in both control and test plate’s one notable difference was observed. In control plates containing glucose, it was observed that the cells had migrated up to the centre of the plate, where glucose was placed. In test plates containing SDS, it was seen that the cells did not migrate to the centre of the plate but they stayed away from it, forming a ring. In order to confirm whether the chemotactic response of the isolate was due to flagellar dependent motility and was not due to interaction of SDS with bacterial cell wall (as both are negatively charged). Drop plate assay was performed by employing heat killed cells (negative control). It was observed that heat killed cells did not show any response towards SDS. Drop plate assay was performed by varying the concentration of SDS. In one plate 10 mg SDS was placed (Online Resource 3B) and in another plate 30 mg SDS was placed (Online Resource 3C) and chemotactic response was observed. It was seen that, in the plate containing 30 mg SDS, the ring had a greater diameter as compared to ring formed in presence of 10 mg SDS. It was observed that by increasing concentrations of SDS, the diameter of the ring increased, but these strains were still chemotactic towards SDS. This might be due to the fact that SDS was toxic to the bacterium. Hence, they stayed at a point where the concentration of SDS was well tolerated by the bacterium and a ring was observed.
When chemotactic response of this isolate towards SDS was confirmed, it was important to study whether the chemotactic response was metabolism dependent or metabolism independent. It has been shown previously that the enzyme alkyl sulfatase involved in biodegradation of SDS is inducible and in glucose grown cell no alkyl sulfatase activity was observed. So, chemotactic response of glucose grown cells towards SDS was tested using drop plate assay method. It was observed that glucose grown cells were chemotactic towards SDS (Online Resource 3E). Further, chemotactic response of SDS grown cells towards glucose was tested (Online Resource 3D). SDS-grown cell showed a delayed chemotactic response towards glucose. It was postulated that glucose is a preferable carbon source, so the cells were chemotactic towards glucose. It was important to study the response of SDS grown cells towards both SDS and glucose (Online Resource 3F). It was seen that the cells were chemotactic towards both SDS and glucose. Chemotactic response towards 1-dodecanol (first metabolite generated after SDS degradation) was evaluated (Online Resource 3G). The strain showed chemotactic response towards 1-dodecanol also. This confirms metabolism dependent chemotaxis of this strain towards SDS. In negative control, response of heat killed cells towards SDS and glucose was checked (Online Resource 3 H&I). It was observed that heat killed cells did not show chemo taxis. It has been reported that Escherichia coli exhibits metabolism-dependent chemotaxis towards glycerol [26]. Rhodobacter sphaeroides shows chemotaxis towards a wide range of amino acids, organic acids, and sugars [27]. Metabolism-dependent chemotaxis is similar to other bacterial behavioral responses collectively known as energy taxis, defined as a behavioral response to stimuli affecting cellular energy levels [28]. The overall process of energy taxis is similar to chemotaxis, the main difference lies in the mechanism whereby the signal for energy taxis is sensed by signal-transduction pathway [29]. Studies have demonstrated that this process involves specialized energy sensors that detect changes in intracellular energy levels. An increase or decrease in energy level is perceived as a signal [30]. Molecules that take part in this process are terminal electron acceptors, light, redox-active compounds and metabolizable substrates that are electron donors [31]. The best example of this kind of receptor system is exhibited by Aer protein of E. coli, which is responsible for aerotaxis. Aer is present on cell membrane and it consists of a single, N terminal sensory domain called PAS domain, which is present in cytoplasm. PAS domain binds to FAD cofactor. Oxidation and reduction of this cofactor generates a signal, which is perceived by Aer protein [32]. Based on data of growth behavior and enzymatic pathway of SDS degradation of P. aeruginosa strain N1, it is evident that this strain has metabolism dependent chemotactic response. This is a tentative conclusion; a detailed study is required to elucidate the exact mechanism of chemotaxis towards SDS as exhibited by P. aeruginosa strain N1.
Abbreviations
- SDS:
-
Sodium dodecyl sulfate
- PBM:
-
Phosphate buffered medium
- TTC:
-
2,3,5-Triphenyl tetrazolium chloride
- NACs:
-
Nitro aromatic compounds
- 2,4-D:
-
2,4-Dichloro phenoxy acetic acid
References
Pandey G, Jain RK (2002) Bacterial chemotaxis toward environmental pollutants: role in bioremediation. Appl Environ Microbiol 68:5789–5795
Gordillo F, Chávez FP, Jerez CA (2007) Motility and chemotaxis of Pseudomonas sp. B4 towards polychlorobiphenyls and chlorobenzoates. FEMS Microbiol Ecol 60:322–328
Liu H, Wang SJ, Zhou NY (2005) A new isolate of Pseudomonas stutzeri that degrades 2-chloronitrobenzene. Biotechnol Lett 27:275–278
Ohtsubo Y, Kudo T, Tsuda M, Nagata Y (2004) Strategies for bioremediation of polychlorinated biphenyls. Appl Microbiol Biotechnol 65:250–258
Paul D, Singh R, Jain RK (2006) Chemotaxis of Ralstonia sp. SJ98 towards p-nitrophenol in soil. Environ Microbiol 8:1797–1804
Krell T, Lacal J, Reyes-Darias JA, Jimenez-Sanchez C, Sungthong R, Ortega-Calvo JJ (2012) Bioavailability of pollutants and chemotaxis. Curr Opin Biotechnol 24:451–456
Parales RE (2004) Nitrobenzoates and aminobenzoates are chemoattractants for Pseudomonas strains. Appl Environ Microbiol 70:285–292
Pandey J, Chauhan A, Jain RK (2009) Integrative approaches for assessing the ecological sustainability of in situ bioremediation. FEMS Microbiol Rev 33:324–375
Parales RE, Harwood CS (2002) Bacterial chemotaxis to pollutants and plant-derived aromatic molecules. Curr Opin Microbiol 5:266–273
Ferrández A, Hawkins AC, Summerfield DT, Harwood CS (2002) Cluster II che genes from Pseudomonas aeruginosa are required for an optimal chemotactic response. J Bacteriol 184:4374–4383
Egbert MD, Barandiaran XE, Di Paolo EA (2010) A minimal model of metabolism-based chemotaxis. PLoS Comput Biol 6:e1001004
Hossein F, Malekzadeh F, Amir mozafari N, Ghaemi N (2007) Biodegradation of anionic surfactants by isolated bacteria from activated sludge. Int J Environ Sci Tech 4:127–132
Lima TM, Procópio LC, Brandão FD, Carvalho AM, Tótola MR, Borges AC (2011) Biodegradability of bacterial surfactants. Biodegradation 22:585–592
Rosety M, Ordonez FJ, Rosety RM, Rosety JM, Rosety I, Carrasco C, Ribelles A (2001) Comparative study of the acute toxicity of anionic surfactants alkyl benzene sulfonate (ABS) and sodium dodecyl sulfate (SDS) on gilthead, Sparus aurata L., eggs. Histol Histopathol 4:1091–1095
Rebello S, Asok AK, Joseph SV, Joseph BV, Jose L, Mundayoor S, Jisha MS (2013) Bioconversion of sodium dodecyl sulphate to rhamnolipid by Pseudomonas aeruginosa: a novel and cost-effective production strategy. Appl Biochem Biotechnol 169:418–430
Bhagwat AA, Leow YN, Liu L, Dharne M, Kannan P (2012) Role of anionic charges of periplasmic glucans of Shigella flexneri in overcoming detergent stress. Foodborne Pathog Dis 9:632–637
Cserháti T, Forgács E, Oros G (2002) Biological activity and environmental impact of anionic surfactants. Environ Int 28:337–348
Ying GG (2006) Fate, behavior and effects of surfactants and their degradation products in the environment. Environ Int 32:417–431
Chaturvedi V, Kumar A (2011) Diversity of culturable sodium dodecy sulfate (SDS) degrading bacteria isolated from detergent contaminated ponds situated in Varanasi city India. Int Biodeter Biodegr 65:961–971
Sondi I, Salopek-Sondi B (2004) Silver nanoparticles as antimicrobial agent: a case study on E. coli as a model for gram-negative bacteria. J Colloid Interf Sci 275:177–182
Ellis AJ, Hales SG, Ur-Rehman NG, White GF (2002) Novel alkyl sulfatases required for biodegradation of the branched primary alkyl sulfate surfactant 2-butyloctyl sulfate. Appl Environ Microbiol 68:31–36
Pandey J, Sharma NK, Khan F, Ghosh A, Oakeshott JG, Jain RK, Pandey G (2012) Chemotaxis of Burkholderia sp. Strain SJ98 towards chloronitroaromatic compounds that it can metabolise. BMC Microbiol 12:19
Abboud MM, Khleifat KM, Batarseh M, Tarawneh KA, Al-Mustafa A, Al-Madadhah M (2007) Different optimization conditions required for enhancing the biodegradation of linear alkylbenzenesulfonate and sodium dodecyl sulfate surfactants by novel consortium of Acinetobacter calcoaceticus and Pantoea agglomerans. Enz Microbial Tech 41:432–439
Arora PK, Sasikala C, Ramana CV (2012) Degradation of chlorinated nitroaromatic compounds. Appl Microbiol Biotechnol 93:2265–2277
Hawkins AC, Harwood CS (2002) Chemotaxis of Ralstonia eutropha JMP123(pJP4) to the herbicide 2,4-dichlorophenoxyacetate. Appl Environ Microbiol 68:968–972
Alexandre G, Greer-Phillips S, Zhulin IB (2004) Ecological role of energy taxis in microorganisms. FEMS Microbiol Rev 28:113–126
Lamanna AC, Ordal GW, Kiessling LL (2005) Large increases in attractant concentration disrupt the polar localization of bacterial chemoreceptors. Mol Microbiol 57:774–785
Szurmant H, Ordal GW (2004) Diversity in chemotaxis mechanisms among the bacteria and archaea. Microbiol Mol Biol Rev 68:301–319
Baker MD, Wolanin PM, Stock JB (2006) Signal transduction in bacterial chemotaxis. BioEssays 28:9–22
Greer-Phillips SE, Alexandre G, Taylor BL, Zhulin IB (2003) Aer and Tsr guide Escherichia coli in spatial gradients of oxidizable substrates. Microbiology 149:2661–2667
Xie Z, Ulrich LE, Zhulin IB, Alexandre G (2010) PAS domain containing chemoreceptor couples dynamic changes in metabolism with chemotaxis. Proc Natl Acad Sci USA 107:2235–2240
Bibikov SI, Barnes LA, Gitin Y, Parkinson JS (2000) Domain organization and flavin adenine dinucleotide-binding determinants in the aerotaxis signal transducer Aer of Escherichia coli. Proc Natl Acad Sci USA 97:5830–5835
Acknowledgments
V.C. is grateful to Council for Scientific and Industrial Research (CSIR), New Delhi, India for the award of Junior Research Fellowship. This study was partly supported by a research grant sanctioned to A.K. by the Department of Biotechnology, Govt. of India, New Delhi (BT/PR1239/AGR./02/065/98).
Author information
Authors and Affiliations
Corresponding author
Electronic supplementary material
Below is the link to the electronic supplementary material.
12088_2013_426_MOESM1_ESM.tif
NATIVE PAGE Zymography of alkyl sulfatase of P. aeruginosa strain N1.Lane1. SDS grown cells, Lane 2. Glucose grown cells (TIFF 192 kb)
12088_2013_426_MOESM2_ESM.tif
Swarm Plate assay for chemotaxis. (A) Chemotaxis in presence of Glucose (Control), (B) Chemotaxis in presence of SDS. (TIFF 804 kb)
12088_2013_426_MOESM3_ESM.tif
Drop plate assay for bacterial chemotaxis. (A) chemotactic response towards Glucose,(B) SDS (10 mg), (C) SDS (30 mg), (D) response of SDS grown cells towards glucose, (E) response of glucose grown cells towards SDS, (F) response of SDS grown cells towards glucose and SDS, (G) response towards 1-dodecanol, (H) response of heat killed cells toward glucose(negative control), (I) response of heat killed cells towards SDS (negative control). (TIFF 2171 kb)
Rights and permissions
About this article
Cite this article
Chaturvedi, V., Kumar, A. Metabolism Dependent Chemotaxis of Pseudomonas aeruginosa N1 Towards Anionic Detergent Sodium Dodecyl Sulfate. Indian J Microbiol 54, 134–138 (2014). https://doi.org/10.1007/s12088-013-0426-8
Received:
Accepted:
Published:
Issue Date:
DOI: https://doi.org/10.1007/s12088-013-0426-8