Abstract
The Yamuna River, a tributary of the holy Ganga, is heavily polluted in the Delhi-NCR region, India and has been gaining attention due to the excessive foaming of the river over the past few years. This can be directly or indirectly related to the overuse of surfactants and the discharge of untreated domestic and textile wastewater into the river. To determine the surfactant load and investigate potential surfactant-degrading bacteria in the region, 96 water samples from four sites in the Okhla Barrage stretch of the river were collected and analysed. The results showed that the selected sites have surfactant concentrations more than the permissible limit (1.00 mgL−1). Also, at most of the sites, the concentration crossed the desirable limit of BIS (0.2 mgL−1) during the period of analysis. The concentration of anionic surfactant reported in the region was found in the range of 0.29 mgL−1 and 2.83 mgL−1. A total of 38 different bacteria were isolated using selective media from the same water samples, out of which 7 bacterial isolates were screened for sodium dodecyl sulphate (SDS) tolerance activity. Based on 16S rRNA gene sequencing, 2 species, namely Pseudomonas koreensis YRW-02 and Pseudomonas songnenensis YRW-05 have been identified and their degradation potential was assessed at different SDS concentrations. The results showed that our strains YRW-02 and YRW-05 degraded 78.29 and 69.24% of SDS respectively. Growth optimization was also performed at different substrate concentrations, pH, and temperature to investigate optimum degradation conditions. This study plays a significant role in assessing the surfactant load and also gives a promising background for future use in in-situ bioremediation experiments.
Similar content being viewed by others
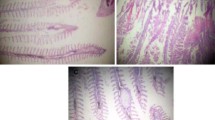
Explore related subjects
Discover the latest articles, news and stories from top researchers in related subjects.Avoid common mistakes on your manuscript.
Introduction
The river Yamuna is the second-largest tributary of the holy Ganga and is considered sacred in Hindu mythology. It originates from the glacier Yamunotri in Uttarakhand, India and travels a length of 1,376 km while passing through 5 Indian states, including the National Capital Territory of Delhi. Approximately 57 million people rely on Yamuna waters and serve for over 70% of water supplies to the NCT of Delhi [24]. It is an important river in the northern Indian plains and accounts for more than 90% of irrigation practices [15]. In its entire 22 km stretch in Delhi, which is less than 2% of the total length of the river, 16 out of 23 drains are pouring their effluents into the Yamuna in between the Wazirabad downstream to Okhla upstream stretch, 4 drains join the river at Okhla Barrage downstream and 3 drains join further at Agra Canal and Gurgaon Canal [3, 11]. Yamuna in Delhi reports approximately 80% of the total pollution in the river "Brief on the Yamuna: WHAT AILS THE YAMUNA?” [9]. Monitoring of water quality parameters of the river over the past years has indicated high levels of heavy metals [6, 40], pesticides [45, 46], pharmaceuticals [7, 27], and various other organic and inorganic pollutants [43, 51]. The occurrence of these contaminants in the river water has been mainly attributed to industrial, municipal, and domestic sewage discharge of waste [5, 55, 56].
During the last decade, there have been several reports of excessive foaming in the Okhla Barrage stretch of the Yamuna River [65]. In fact, foaming in the river has been a regular phenomenon, which has recently gathered a lot of media attention. Unfortunately, no scientific study has been conducted yet to address this issue. Although some studies have suggested that the foam in the river is caused due to the presence of detergents [8, 34, 48, 57], no substantial work in the same field has been done so far.
A major constituent of detergents, surfactants have been categorised as ‘contaminants of emerging concern’ due to their excessive usage and recent studies suggesting their toxicity to various living organisms Kaczerewska et al. [23, 26, 66]. Multiple studies have been conducted where bacteria from surfactant-contaminated sites have been isolated and exploited for their surfactant-degrading capabilities [12, 17, 25, 58]. Extremophiles have exceptional adaptation capabilities that make them reservoirs of biocatalysts and enzymes that can be used remarkably in the bioremediation of contaminants. Psychrophiles are organisms exhibiting optimal growth below 15 °C and maximum growth at 20 °C [29, 30]. They have been largely studied for their role in the bioremediation of xenobiotics. Psychrotolerant microbes on the other hand show growth at much lower rates at low temperatures and exhibit optimum growth at temperatures ranging between 20–40 °C, similar to mesophilic organisms [29]. Marine psychrotolerant Pseudomonas sp. from the Mediterranean sea has been reported to show effective azo dye decolorization. The same species also showed heavy tolerance to lead and was able to resist and accumulate 700–800 ppm of the metal [39]. A psychrotolerant Rahnella sp. isolated from the soil samples of apple orchards in Western Himalayan regions effectively degraded organophosphate profenofos [61]. Pseudomonas syringae and P. fluorescens isolated from Sub-arctic river Glerá in Northern-Iceland were found to possess surfactant-degrading abilities. The species were psychrotropic in nature, associated with freshwater environments in the cold environment [41].
Bioremediation helps in naturally breaking down the pollutants, offering an eco-friendly approach to environmental cleanup. By harnessing the power of microorganisms and plants, bioremediation can degrade contaminants and restore contaminated ecosystems [31]. For an effective remediation approach, quantification of pollutants is the first step towards its mitigation. Therefore, in the current study, we aimed at analysing the average concentration of anionic surfactants in the Yamuna river. We isolated and characterized bacteria possessing surfactant-degrading capabilities from the same environment and investigated their growth potential in different environmental conditions so as to make it closer to the field conditions.
Materials and methods
Reagents, chemicals, and bacteriological media
Various bacteriological media and reagents used in the study include nutrient agar (NA), nutrient broth, starch agar, King’s B media, buffered peptone water, Oxidase test disc, Kovac’s reagent, nitrate broth, Methyl red- Vogues Proskauer broth (MR-VP agar), Simmon’s citrate agar, Gram’s staining kit, DNA isolation kit were obtained from Hi-Media, Mumbai, India. Hydrogen peroxide, Ethanol, Sulphanilic acid, Isopropyl alcohol (70%), Alpha-Napthylamine, Sodium chloride, α-Napthol, methyl red indicator, Sodium dodecyl sulphate (SDS) (purity ≥ 98.0%), were of analytical grade and procured Sigma Laboratories, India. Chloroform (purity ≥ 99.8%) was obtained from Fischer Scientific, India, and Methylene blue was purchased from Qualikems, India.
Study area and sample collection
Collection, preservation, and transportation of water samples to the laboratory and analysis were executed as per standard methods. Water samples were collected in 500 mL acid-washed containers with air-tight caps, after filtering with Whatman filter paper No. 1 (pore size 11 µm), as prescribed in the standard procedure [2]. The containers were soaked in 10% nitric acid and rinsed with deionized water 2–3 times. All the samples were immediately carried to the laboratory and stored at 4 °C until analysis. The surfactants were determined by the methylene blue active substances (MBAS) assay (APHA, 5440-C) using UV–vis spectrophotometer (Agilent Cary 60-G6860A). Bureau of Indian Standard (BIS), formerly known as Indian Standards Institution (ISI) is the National Standards Body of India which develops and publishes Indian Standards. As per BIS: 10,500 (1991), a concentration of 0.20 ppm is considered the desirable limit and 1.0 ppm is the permissible limit for surfactants in water (Table 1).
Analytical method (MBAS Assay) for quantification of anionic surfactants
The evaluation of anionic surfactant concentration was done by a standard method with methylene blue dye and chloroform as solvent (APHA, 5540 C, 2017). Anionic surfactants form a blue-coloured complex with methylene blue, which is extracted over chloroform and the absorbance is measured at 652 nm using a UV/Visible spectrophotometer (Cary 60, Agilent Technologies). This method is popularly known as methylene blue active substances assay (MBAS Assay).
Quantification and isolation
Quantification of bacteria in each water sample was performed by serially diluting (ratio 1:10; 25 mL sample in 225 mL of 0.85% saline) the sample and following the pour plate method using NA and incubating the samples at 37 °C. The total number of colonies on each media was calculated using the formula:
where, N is the sum of colony-forming units in one ml of sample, d stands for the dilution factor from which the primary counts were attained, n1 and n2 correspond to the number of plates considered in the first and second dilution respectively, and ΣC indicates the sum of all colonies counted on all the plates. The colonies were observed under a microscope, and identical colonies were re-streaked on NA plates to obtain pure cultures. These cultures were then subjected to SDS-tolerance tests [14].
Screening of SDS-tolerant bacterial strains
For screening of SDS-tolerant strains, the bacterial isolates were further grown in minimal media having the following composition (per liter): 3 g K2HPO4, 10 g KH2PO4, 2 g NH4NO3, 0.3 g MgSO4·7H2O, 0.1 g NaCl, 0.01 g CaCl2·2H2O, 0.01 g MoO3, 0.001 g, MnCl2, 0.001 g CuCl2, 0.001 g FeSO4, 0.001 g ZnSO4 [17]. SDS (NaSO4C12H25; molecular weight 288.38 gmol−1) at concentrations of 12.5, 25, 50, 100, 200, 300, and 500 mgL−1 respectively, was added using a 0.45 µm nylon syringe filter. This SDS acted as the only source of carbon for the bacteria. The cultures were cultivated at 20 °C temperatures and were observed for turbidity as an indicator of bacterial growth.
Optimization of growth conditions
Variations in bacterial growth at different pH, incubation temperatures, and substrate (SDS) concentrations were observed. Multiple experiments were performed to assess the tolerance of the bacterial isolate at SDS concentrations of 12.5, 25, 50, 100, 200, 300, and 500 mgL−1, at varying pH (5.0, 7.0, and 8.0), and incubation temperatures of 4 °C, 20 °C or 37 °C. Bacterial growth was estimated by measuring optical density (OD) using a UV/visible spectrophotometer at 600 nm [28].
Biodegradation studies
Biodegradation tests were carried out in 250 mL conical flasks in sterile condition containing 100 ml of minimal media for strains that exhibited tolerance towards SDS. Based on the results obtained during growth optimization, the parameters selected for the biodegradation study were as follows: 50 mgL−1 concentration of SDS, 7 pH, and 4 °C, and 37 °C temperature for YRW-02 and YRW-05 strains respectively. A loopful of freshly grown (24 h old) bacterial isolates were dissolved in 0.85% saline for the preparation of inoculum for each strain. The OD of each inoculum was adjusted to 25% transmittance at 530 nm using a UV/Visible spectrophotometer [17].
To cross-verify the number of colonies present per ml of adjusted inoculum, validation was done using the serial dilution pour-plate method [13].
A 50 mgL−1 working solution of SDS was prepared from a stock solution of 100 mgL−1. The flasks were incubated at 4 °C and 37 °C respectively, and stirred at 150 rpm for 216 h under aerobic conditions. At every 24-h interval, samples were withdrawn for assessing the SDS concentration using MBAS assay.
Morphological and biochemical characterization
The bacterial isolates exhibiting tolerance towards SDS were subjected to morphological, biochemical, and molecular identification. Colony morphology with respect to shape, size, margin, elevation, and colour was observed under a light microscope (Olympus BX41).
Biochemical tests viz., Catalase, Oxidase, Citrate, Starch, Nitrate, Indole, Fluorescence and Voges-Proskaauser [13] were performed.
16S gene sequencing
DNA was extracted using GenElute™ Bacterial Genomic DNA kit (Sigma-Aldrich, USA). The ~ 1.5 kbp, 16S-rDNA fragment was amplified using high–fidelity PCR polymerase, and the primers used were 16S forward (GGATGAGCCCGCGGCCTA) and 16S reverse (CGGTGTGTACAAGGCCCGG). The PCR amplicon was purified for the removal of contaminants and was also resolved on agarose gel. The PCR product was sequenced bi-directionally and the sequence data were aligned and analyzed to identify the bacteria and its closest neighbors using NCBI's (National Center for Biotechnology) Blast software. Based on the maximum identity score, the first ten sequences were selected and aligned by importing them to multiple alignment software programs Clustal W. The phylogenetic tree was constructed using the neighbor-joining algorithm, by the software MEGA 10 [33]. The stability within the phylogenetic tree clades was determined with a 1000-replication bootstrap analysis.
Results
Quantification of anionic surfactants
The results of quantification of anionic surfactants at four sites in the Kalindi Kunj, Yamuna riverfront show a significantly higher concentration during all times of analysis. Site-wise results from November have been summarized in Table S1 (supplementary material). During the analysis, it was observed that site L1, which was the area of most foaming, reported the maximum concentration of anionic surfactants in all sampling months, followed by L2, L3, and then L4, which was the area near the river bank. The highest and lowest concentration of anionic surfactant in the study area was reported to be 2.83 mgL−1 (at L1) and 0.29 mgL−1 (at L4) respectively. This investigation also showed that there was no significant month-wise difference in anionic surfactant concentrations in the study area. The reduction in concentrations from L1 to L4 could be due to the dilution of surfactants in the river water. The results show a clear relation between the area of most foaming (L1) with significantly high anionic surfactant concentration (with a mean concentration of 1.98 mgL−1 for the study duration), establishing that high surfactant concentration is one of the major reasons for foaming in the region (Fig. 2).
Quantification and isolation of bacteria
At the four sampling sites across the Okhla Barrage stretch of river Yamuna, the total bacterial population was found in the range of \(1.3\times {10}^{2}\) -\(2.6\times {10}^{9}\) CFU mL−1. A detailed site-wise bacterial population is given in Table 2. The maximum bacterial population was found near the banks (L4) and was found to decrease on moving from the banks towards the area of most foaming (L1). The observed bacterial population is also indicative of the anti-bacterial property of surfactants as some samples with high surfactant concentration showed less bacterial count and vice-versa. However, a stable and constant trend has not been observed in this regard which also indicates interference of other chemical and biological contaminants.
On the basis of colony morphology observed on NA during the enumeration process, 38 isolates with identical colonies were selected for further screening of SDS-tolerant strains.
Screening of SDS-tolerant bacterial strains
All bacterial isolates were subjected to SDS tolerance at 12.5, 25, 50, 100, 200, 300, and 500 mgL−1 concentrations. On the basis of turbidity observed in the samples in 48–72 h, 26 isolates did not show any growth at any SDS concentration, even at increasing the incubation period to 96–120 h. For the remaining 12 samples, bacterial growth was observed in 2 isolates after 24 h and in 10 isolates after 96 h of incubation, respectively in 12.5, 25, and 50 mgL−1 SDS concentrations, one of which was also able to grow in 100 mgL−1 concentration. None of these 12 isolates show any turbidity in concentrations above 200 mgL−1. Thus, the two isolates exhibiting growth in 24 h out of the total thirty-eight were found suitable for further studies as they were able to tolerate SDS and utilize it for their growth in the least incubation time.
Optimization of growth conditions
The most suitable temperature and pH for the growth of YRW-02 were found to be 4 °C, followed by 20 °C and 7 pH. A slight difference was observed between pH 5 and 8, with 8 pH exhibiting better growth conditions. YRW-02 showed the highest growth at 50 mgL−1 (OD = 0.4269), relatively lower growth at 100 (OD = 0.2966) and 200 mgL−1 (OD = 0.1371), followed by complete inhibition of growth at 300 and 500 mgL−1 (OD = 0.0093, 0.0051) concentration of SDS. No bacterial growth was seen at 37 °C. Thus, the best growth conditions for YRW-02 were observed at a temperature of 4 °C and 7 pH, at 500 mgL−1 substrate concentration with SDS as a sole carbon source. Figure 3. gives a graphical representation of different growth parameters and their results for the strain YRW-02.
As compared to YRW-02, the strain YRW-05 showed the highest growth at 100 mgL−1 at 37 °C (OD = 0.358) and at 20 °C (OD = 0.3213) at the same substrate concentration, followed by growth at 50 mgL−1(OD = 0.311) at 37 °C, which further decreased from 200 mgL−1 (OD = 0.148) to negligible growth at 500 mgL−1 (OD = 0.0091), indicating the limit of tolerance for the strain. Contrary to YRW-02, YRW-05 did not show any growth at 4 °C, however, neutral pH was found to favour optimum growth in both strains. Hence, a neutral pH, 37 °C temperature, and 100 mgL−1 SDS concentration were found to be the optimum growth conditions for YRW-05. Figure 4. gives an illustration of changing growth parameters on the growth of strain YRW-05 in graphical form.
Biodegradation studies
The MBAS assay was used for the calculation of the concentration of SDS in the flasks under observation. As per the observations made during the growth optimization experiments, flasks with YRW-02 strain as inoculum were incubated at 4 °C, and flasks with YRW-05 strain as inoculum were incubated at 37 °C. Both sets of flasks at their respective incubation temperatures showed OD values in decreasing order, starting from 0 h, suggesting the degradation of SDS by the bacterial strains. For YRW-02, OD values continued to decrease till 96 h and did not show any further reduction in SDS concentration. Whereas the strain YRW-05 showed a decreasing trend in SDS concentration till 120 h and stabilized thereafter. YRW-02 and YRW-05 degraded 50 mgL−1 of SDS to 10.124 and 15.157 mgL−1 respectively. Figure 4 gives a combined graphical representation of SDS degradation with the two strains. Table S2 (supplementary material) and Fig. 5 shows an hour-wise change in the concentration and percentage degradation of SDS with YRW-02 and YRW-05. Thus, a maximum degradation of 78.29 and 69.24% was achieved with these two strains.
Morphological and biochemical characterization of potential SDS-degrading bacterial strains
On microscopic analysis, both YRW-02 and YRW-05 were found to be Gram’s negative strains. Both were motile with one or more flagellum, rod-shaped, and non-spore-forming. Colony characteristics showed YRW-02 to possess yellow, circular, and convex colonies with smooth margins, while YRW-05 had radial, yellow-orange flat colonies with wrinkled margins.
Biochemical tests revealed that YRW-02 showed fluorescence on King’s B medium, was negative for catalase, starch, and nitrate but positive for oxidase and citrate test. YRW-05 was found to be negative for Indole and positive for Voges-Proskauer, nitrate, and starch tests. Table 3. describes all the microscopic, morphological, and biochemical tests performed on the strains YRW-02 and YRW-05.
16S rRNA sequencing
A total of 188 and 172 ng of DNA was extracted from YRW-02 and YRW-05 strains respectively. On performing 16S rRNA sequencing, YRW-02 was found to bear 99.74% similarity with Pseudomonas koreensis strain Ps 9–14 and YRW-05 was found to be 98.96% similar to Pseudomonas songnenensis strain NEAU-ST5-5. The 16S rRNA phylogenetic tree confirmed that YRW-02 and YRW-05 corresponded to Pseudomonas koreensis (Fig. 6) and Pseudomonas songnenensis (Fig. 7) respectively.
Discussion
Apart from providing the basic necessities of life, rivers in India have shaped history, culture, and faith and are regarded as mythological entities. However, the condition of a few Indian rivers is deteriorating due to increasing load of pollution. The presence of toxic substances viz. pharmaceutical products, household chemicals, agrochemicals, microplastics are emerging pollutants in wastewater [35, 37]. The generation of immense sewage discharge load on the treatment plants leads to inefficient water treatment and it has been a major reason for the ever-increasing pollution of rivers and other water bodies [59]. Surfactants are one of the major groups of contaminants which exist in almost all the urban, rural, and industrial wastewater [16]. The adverse effects of surfactants on different environmental components have been well-studied and thus, surfactants have been rightly classified as ‘contaminants of emerging concern’ [4]. Surfactants not only reduce the quality of water but are also responsible for the excessive foaming of the water bodies. Among all types of surfactants, anionic surfactants are most widely used in household detergents and personal care products, and industries [16].
Limited research has been done in terms of the quantification of anionic surfactants in water bodies. Patel and Patel, [47] aimed at determining the levels of anionic surfactants in municipal wastewater wherein the concentration of sodium lauryl sulphate was found in the range of 1.0—42.2 mgL−1. A study conducted on Hugli river, Kolkata, India, reveals the presence of anionic surfactants in surface and groundwater samples, where a concentration ranging between 0.013–0.425 mgL−1 was reported [19]. Assessment of surfactants in the Ganga Canal was carried out by a group of researchers wherein a maximum of 2.5 mgL−1 of anionic surfactants were reported [53]. Another study on the assessment of water systems in and around Tirupati, India, showed the presence of anionic surfactants in the range of 0.01–0.092 mgL−1 [49]. Water samples from 10 different locations were procured to assess the concentration of surfactants in the flowing water of Gomti river, India. The results obtained were suggestive of significantly higher concentrations of surfactants in the river ranging between 0.1005 and 0.2758 mgL−1 [36]. Considering the alarming effects, the present study was conducted on a small stretch of the Yamuna river, which is the region reported to exhibit most foaming throughout the year, was investigated for anionic surfactant concentrations. Over a span of six months, our results show anionic surfactants concentration in the range of 0.29–2.83 mgL−1. These results suggest that high concentrations of surfactants in the region are one of the major reasons for the foaming of the river in this region, which can be due to untreated discharge of effluents from the industries and households.
Several studies have reported the isolation of surfactant-degrading bacteria from surfactant-contaminated wastewater and water bodies. River-water sample from New Calabar river, Nigeria were evaluated for the presence of detergent-tolerating bacteria. The study concluded that microbial isolates of Vibrio, Flavobacterium, Klebsiella, Pseudomonas, Enterobacter, Bacillus, Escherichia, Shigella, Citrobacter, Proteus, Anabaena and an actinomycete was readily utilizing liquid detergents and shampoos present in the river water [44]. In a study undertaken in Turkey, water samples from a detergent-polluted river were screened for SDS-degrading bacteria where a Delftia acidovorans was found to optimally grow at 1 gL−1 of SDS as a sole carbon source and degraded 87% of the substrate in 11 days of incubation [64]. In a study where SDS-degrading bacteria was isolated from a detergent-contaminated pond, Pseudomonas putida was found to be a potential strain as it showed complete degradation of 0.3% SDS in 16 h of incubation [12]. Multiple studies have reported a variety of SDS-degrading bacteria comprising many strains of Pseudomonas sp. with different variables and degradation efficiencies [12, 54, 63]. In this study, we observed that the YRW-02 strain showed the highest growth at a concentration of 50 mgL−1 and pH 7. Increasing the concentration from 300–500 mgL−1 was seen to be unfavourable for the growth of strains.
Extremophilic microbes are a great reservoir of enzymes and thus their role in bioremediation has been significant. Psychrophilic and psychrotolerant bacteria have also been extensively studied for their roles in the degradation of various contaminants, signifying their biotechnological importance. Studies of degradation of chlorpyrifos by psychrophilic Shewanella sp. [20], hydrocarbons by psychrophilic Oleispira Antarctica [18], pyrene by Shewanella sp. [50], malathion by psychrotolerant Ochrobactrum sp. [62], naphthalene and chrysene by psychrotolerant Bacillus sp. [32] have been reported in the past. In the current study, a psychrophilic Pseudomonas koreensis and a psychrotolerant P. songnenensis, isolated from the surfactant-contaminated stretch of Yamuna River, have been found to utilize SDS as a sole carbon source. Other psychrophilic and psychrotolerant species from the Pseudomonas genus have also been utilised for the degradation of various contaminants. In this study, we observed that YRW-02 and YRW-05 showed maximum degradation of 78.29 and 69.24% respectively. 16S RNA gene sequencing revealed the two species to be P. koreensis and P. songnenensis. Comparing the results obtained during this study with previous literature from the recent past, it was observed that Pseudomonas sp. has been associated with SDS degrading potentiality. For example, P. frederiksbergensis have been reported for the degradation of long-chain alkanes [1], P. putida, P. synxantha, and P. azotoformans for the degradation of p-xylene [42], another Pseudomonas sp. for degrading phenol [52], P. palleroniana for the degradation of bisphenol [60], and P. putida for the degradation of nitrobenzene [38]. A study conducted in Antarctica identified an SDS-degrading psychrophilic Pseudomonas sp. that utilized 90% of SDS in 8 days of incubation. This strain optimally grew at 10 °C and pH of 7.25 [21]. These results were in agreement with the results obtained in the current study wherein cold-adapted Pseudomonas sp. was found to degrade SDS.
Conclusion
The quantification of anionic surfactants in the Okhla Barrage region of the Yamuna river, Delhi was carried out in this study. This stretch of the river has been reported for maximum foaming in the past few years, thus our study establishes the presence of anionic surfactants as one of the major reasons for foaming in the water. Anionic surfactants in the range of 0.29–2.83 mgL−1 were found in the river water samples. The same water samples were used for the isolation and identification of native SDS-degrading bacteria that can be utilised for the bioremediation of surfactant pollution in the river. Two potential strains, P. koreensis YRW-02 and P. songnenensis YRW-05, with SDS-degrading capabilities, were successfully isolated in the study. Psychrophilic P. koreensis YRW-02 was found to degrade 78.29% of SDS in 96 h at 4 °C while, psychrotolerant P. songnenensis YRW-05 degraded 69.24% SDS in 120 h of incubation at a temperature of 37 °C. Several studies have reported surfactants to cause blood toxicity, eyes, and skin irritation and thus their determination in river water as raw water supply and drinking water are important since surfactants have become a threat to our water supply network. Previously, both P. koreensis and P. songnenensis have been utilised for bioremediation purposes, however, their applicability in the degradation of surfactants has not been much explored. The variation in the mechanism of degradation by both species can be further studied with respect to varying environmental parameters such as temperature, pH, etc. These initial results suggest the possibility of P. koreensis and P. songnenensis being further used in environmental remediation studies.
Data Availability
All the data pertaining to this manuscript has been included in the manuscript.
References
Abdel-Megeed, A. (2013). Potential degradation of certain alkanes by Pseudomonas frederiksbergensis. J Pure Appl Microbiol, 7(3), 13–21.
APHA. (1998). Standard methods for examination of water and wastewater. American Public Health Association.
Arif, M., Kumar, R., & Parveen, S. (2020). Reduction in water pollution in Yamuna River due to lockdown under covid-19 pandemic. https://doi.org/10.26434/chemrxiv.12440525.v1
Arora, J., Ranjan, A., Chauhan, A., Biswas, R., Rajput, V. D., Sushkova, S., Mandzhieva, S., Minkina, T., & Jindal, T. (2022). Surfactant pollution, an emerging threat to ecosystem: Approaches for effective bacterial degradation. Journal of Applied Microbiology, 133(3), 1229–1244. https://doi.org/10.1111/jam.15631
Asim, M., & Nageswara Rao, K. (2021). Assessment of heavy metal pollution in Yamuna River, Delhi-NCR, using Heavy Metal Pollution Index and GIS. Environmental Monitoring and Assessment, 193(2). https://doi.org/10.1007/s10661-021-08886-6
Bhardwaj, R., Gupta, A., & Garg, J. (2017). Evaluation of Heavy Metal Contamination using environmetrics and indexing approach for river Yamuna, Delhi Stretch, India. Water Science, 31(1), 52–66. https://doi.org/10.1016/j.wsj.2017.02.002
Biswas, P., & Vellanki, B. P. (2021). Occurrence of emerging contaminants in highly anthropogenically influenced river Yamuna in India. Science of The Total Environment, 782, 146741. https://doi.org/10.1016/j.scitotenv.2021.146741
Bowes, M. J., Read, D. S., Joshi, H., Sinha, R., Ansari, A., Hazra, M., Simon, M., Vishwakarma, R., Armstrong, L. K., Nicholls, D. J., Wickham, H. D., Ward, J., Carvalho, L. R., & Rees, H. G. (2020). Nutrient and microbial water quality of the Upper Ganga River, India: Identification of Pollution Sources. Environmental Monitoring and Assessment, 192(8). https://doi.org/10.1007/s10661-020-08456-2
Brief on the Yamuna: WHAT AILS THE YAMUNA?. (2021). Retrieved 19 October 2022, from https://www.cseindia.org/brief-on-the-yamuna-what-ails-the-yamuna--11059
Bureau of Indian Standards (BIS). (1991). Specification for drinking water 1S:10500: Bureau of Indian Standards, New Delhi
Central Pollution Control Board. (2020). Alternative treatment technologies for wastewater treatment in drains In Compliance to Direction of Hon’ble NGT in the Matter of OA No. 06/2012 Titled Manoj Mishra Vs Union of India & ORS.
Chaturvedi, V., & Kumar, A. (2011). Diversity of culturable sodium dodecyl sulfate (SDS) degrading bacteria isolated from detergent contaminated ponds situated in Varanasi City, India. International Biodeterioration & Biodegradation, 65(7), 961–971. https://doi.org/10.1016/j.ibiod.2011.07.005
Chauhan, A., & Jindal, T. (2020). Microbiological methods for environment, food and pharmaceutical analysis. Springer Nature.
Chauhan, A., Bharti, P., Goyal, P., Varma, A., & Jindal, T. (2015). Psychrophilic pseudomonas in antarctic freshwater lake at stornes peninsula, larsemann hills over east Antarctica. Springerplus, 4(1). https://doi.org/10.1186/s40064-015-1354-3
CPCB. (1980–1981). The Ganga River—Part I—The Yamuna basin, ADSORBS/2. Delhi: Central Pollution Control Board.
Dehghani, M. H., Zarei, A., & Yousefi, M. (2019). Efficiency of ultrasound for degradation of an anionic surfactant from water: Surfactant determination using methylene blue active substances method. MethodsX, 6, 805–814. https://doi.org/10.1016/j.mex.2019.03.028
Fedeila, M., Hachaïchi-Sadouk, Z., Bautista, L. F., Simarro, R., & Nateche, F. (2018). Biodegradation of anionic surfactants by alcaligenes faecalis, Enterobacter cloacae and serratia marcescens strains isolated from industrial wastewater. Ecotoxicology and Environmental Safety, 163, 629–635. https://doi.org/10.1016/j.ecoenv.2018.07.123
Gentile, G., Bonsignore, M., Santisi, S., Catalfamo, M., Giuliano, L., Genovese, L., Yakimov, M. M., Denaro, R., Genovese, M., & Cappello, S. (2016). Biodegradation potentiality of psychrophilic bacterial strain Oleispira antarctica RB-8 T. Marine Pollution Bulletin, 105(1), 125–130. https://doi.org/10.1016/j.marpolbul.2016.02.041
Ghose, N. C., Saha, D., & Gupta, A. (2009). Synthetic detergents (Surfactants) and organochlorine pesticide signatures in surface water and groundwater of greater kolkata, india. Journal of Water Resource and Protection, 1(4), 290–298. https://doi.org/10.4236/jwarp.2009.14036
Govarthanan, M., Ameen, F., Kamala-Kannan, S., Selvankumar, T., Almansob, A., Alwakeel, S. S., & Kim, W. (2020). Rapid biodegradation of chlorpyrifos by plant growth-promoting psychrophilic Shewanella sp. BT05: An eco-friendly approach to clean up pesticide-contaminated environment. Chemosphere, 247, 125948. https://doi.org/10.1016/j.chemosphere.2020.125948
Halmi, M. I. E., Hussin, W. S. W., Aqlima, A., Syed, M. A., Ruberto, L. A. M., MacCormack, W. P., & Yukor, M. Y. (2013). Characterization of a sodium dodecyl sulphate-degrading Pseudomonas sp. strain DRY15 from Antarctic soil.
ISO 7875‐1. (1996). Water quality—determination of surfactants—part 1: determination of anionic surfactants by measurement of the methylene blue index (MBAS).
Ivon, E. A., Ubi, G. M., Etangetuk, N. A., Anyanwu, C. O., Nkang, A. N., & Ekanem, A. P. (2020). Toxic Potentials of Nittol Detergent on Haematological Parameters of African Catfish (Clarias gariepinus) in Nigeria. Annual Research & Review in Biology, 35(4), 53–67.
Jaiswal, M., Hussain, J., Gupta, S. K., Nasr, M., & Nema, A. K. (2019). Comprehensive evaluation of water quality status for entire stretch of Yamuna River, India. Environmental Monitoring and Assessment, 191(4), 1–17.
John, E. M., Rebello, S., Asok, A. K., & Jisha, M. S. (2015). Pseudomonas plecoglossicida S5, a novel nonpathogenic isolate for sodium dodecyl sulfate degradation. Environmental Chemistry Letters, 13(1), 117–123.
Kaczerewska, O., Martins, R., Figueiredo, J., Loureiro, S., & Tedim, J. (2020). Environmental behaviour and ecotoxicity of cationic surfactants towards marine organisms. Journal of Hazardous Materials, 392, 122299. https://doi.org/10.1016/j.jhazmat.2020.122299
Khan, A. H., Aziz, H. A., Khan, N. A., Dhingra, A., Ahmed, S., & Naushad, M. (2021). Effect of seasonal variation on the occurrences of high-risk pharmaceutical in drain-laden surface water: A risk analysis of Yamuna River. Science of The Total Environment, 794, 148484. https://doi.org/10.1016/j.scitotenv.2021.148484
Khleifat, K. M. (2006). Biodegradation of sodium lauryl ether sulfate (Sles) by two different bacterial consortia. Current Microbiology, 53(5), 444–448. https://doi.org/10.1007/s00284-006-0266-4
Dziewit, L., & Bartosik, D. (2014). Plasmids of psychrophilic and psychrotolerant bacteria and their role in adaptation to cold environments. Frontiers in Microbiology, 5. https://doi.org/10.3389/fmicb.2014.00596
Morita, R. Y. (1975). Psychrophilic bacteria. Bacteriological reviews, 39(2), 144–167.
Kishore, S., Malik, S., Shah, M. P., Bora, J., Chaudhary, V., Kumar, L., … Ranjan, A. (2022). A comprehensive review on removal of pollutants from wastewater through microbial nanobiotechnology -based solutions. Biotechnology and Genetic Engineering Reviews. https://doi.org/10.1080/02648725.2022.2106014
Kolsal, F., et al. (2017). Hydrocarbon degradation abilities of psychrotolerant bacillus strains. AIMS Microbiology, 3(3), 467–482. https://doi.org/10.3934/microbiol.2017.3.467
Kumar, S., Stecher, G., Li, M., Knyaz, C., & Tamura, K. (2018). MEGA X: Molecular Evolutionary Genetics Analysis across computing platforms. Molecular Biology and Evolution, 35, 1547–1549.
Kumar, M., Sharif, M., & Ahmed, S. (2019). Impact of urbanization on the river Yamuna Basin. International Journal of River Basin Management, 18(4), 461–475. https://doi.org/10.1080/15715124.2019.1613412
Kumar, A., Ranjan, A., Gulati, K., Thakur, S., & Jindal, T. (2016). Assessment of chemical and microbial contamination in groundwater through leaching of sewage waste in Delhi, India. Environmental Earth Sciences, 75(3). https://doi.org/10.1007/s12665-015-5016-0
Kumar, V., Sahu, P., K. Singh, P., K. Shukla, N., Mishra, D. P., & Markandeya. (2021). Evaluation and quantification of anionic surfactant in the gomti river at lucknow city, india. In I. Ahmed Moujdin & J. Kevin Summers (Eds.), Promising Techniques for Wastewater Treatment and Water Quality Assessment. IntechOpen. https://doi.org/10.5772/intechopen.93517
Kumari, A., Rajput, V. D., Mandzhieva, S. S., Rajput, S., Minkina, T., Kaur, R., … Glinushkin, A. P. (2022). Microplastic pollution: An emerging threat to terrestrial plants and insights into Its remediation strategies. Plants, 11(3). https://doi.org/10.3390/plants11030340
Li, Y., Hu, H., & Wu, Q. (2007). Isolation and characterization of psychrotrophic nitrobenzene-degrading strains from river sediments. Bulletin of Environmental Contamination and Toxicology, 79(3), 340–344. https://doi.org/10.1007/s00128-007-9239-y
M. Abd-Elnaby, H., M. Abou-Elela, G., Hussein, H., A. Ghozlan, H., & A. Sabry, S. (2019). Characterization and Bioremediation potential of marine psychrotolerant Pseudomonas spp. isolated from the Mediterranean Sea, Egypt. Egyptian Journal Of Aquatic Biology And Fisheries, 23(4), 669–683. https://doi.org/10.21608/ejabf.2019.63537
Malik, D., Singh, S., Thakur, J., Singh, R. K., Kaur, A., & Nijhawan, S. (2014). Heavy metal pollution of the Yamuna River: An introspection. International Journal of Current Microbiology and Applied Sciences, 3(10), 856–863.
Markúsdóttir, M., Heiðmarsson, S., Eyþórsdóttir, A., Magnússon, K. P., & Vilhelmsson, O. (2013). The natural and anthropogenic microbiota of Glerá, a sub-arctic river in northeastern Iceland. International Biodeterioration & Biodegradation, 84, 192–203. https://doi.org/10.1016/j.ibiod.2012.04.001
Miri, S., Rasooli, A., Brar, S. K., Rouissi, T., & Martel, R. (2022). Biodegradation of p-xylene—A comparison of three psychrophilic Pseudomonas strains through the lens of gene expression. Environmental Science and Pollution Research, 29(15), 21465–21479. https://doi.org/10.1007/s11356-021-17387-5
Misra, A. K. (2010). A river about to die: Yamuna. Journal of water resource and protection, 2(5), 489.
Okpokwasili, G. C., & Olisa, A. O. (1991). River-water biodegradation of surfactants in liquid detergents and shampoos. Water Research, 25(11), 1425–1429. https://doi.org/10.1016/0043-1354(91)90121-6
Pandey, P., Khillare, P., & Kumar, K. (2011). Assessment of organochlorine pesticide residues in the surface sediments of river Yamuna in Delhi, India. Journal of Environmental Protection, 02(05), 511–524. https://doi.org/10.4236/jep.2011.25059
Parween, M., Ramanathan, A., & Raju, N. J. (2021). Assessment of toxicity and potential health risk from persistent pesticides and heavy metals along the Delhi stretch of river Yamuna. Environmental Research, 202, 111780. https://doi.org/10.1016/j.envres.2021.111780
Patel, R., & Patel, K. S. (1998). Flow injection determination of anionic surfactants with cationic dyes in water bodies of central India. The Analyst, 123(8), 1691–1695. https://doi.org/10.1039/a802945h
Rai, R. K., Upadhyay, A., Ojha, C. S., & Singh, V. P. (2011). Water pollution. Water Science and Technology Library, 245–275. https://doi.org/10.1007/978-94-007-2001-5_9
Ramanjulu, C., Naidu, N. V., Kanchi, S., & Bisetty, K. (2015). Seasonal variation and distribution of anionic surfactants in and around Tirupati: a famous pilgrim centre in South India. Asian Journal of Chemistry.
Rathour, R., Gupta, J., Tyagi, B., Kumari, T., & Thakur, I. S. (2018). Biodegradation of pyrene in soil microcosm by Shewanella sp. ISTPL2, a psychrophilic, alkalophilic and halophilic bacterium. Bioresource Technology Reports, 4, 129–136. https://doi.org/10.1016/j.biteb.2018.10.004
Said, S., & Hussain, A. (2019). Pollution mapping of Yamuna River segment passing through Delhi using high-resolution geoeye-2 imagery. Applied Water Science, 9(3). https://doi.org/10.1007/s13201-019-0923-y
Sepehr, S., Shahnavaz, B., Asoodeh, A., & Karrabi, M. (2019). Biodegradation of phenol by cold-tolerant bacteria isolated from alpine soils of Binaloud Mountains in Iran. Journal of Environmental Science and Health, Part A, 54(4), 367–379. https://doi.org/10.1080/10934529.2018.1553818
Seth, R., Singh, P., Mohan, M., Singh, R., & Aswal, R. S. (2013). Monitoring of phenolic compounds and surfactants in water of Ganga Canal, Haridwar (India). Applied Water Science, 3(4), 717–720. https://doi.org/10.1007/s13201-013-0116-z
Shahbazi, R., Kasra-Kermanshahi, R., Gharavi, S., Moosavi-Nejad, Z., & Borzooee, F. (2013). Screening of SDS-degrading bacteria from car wash wastewater and study of the alkylsulfatase enzyme activity. Iranian Journal of Microbiology, 5(2), 153.
Sharma, M. P., Singal, S. K., & Patra, S. (2009). Water quality profile of Yamuna River, India. Hydro Nepal: Journal of Water, Energy and Environment, 3, 19–24. https://doi.org/10.3126/hn.v3i0.1914
Sharma, R., Kumar, R., Satapathy, S. C., Al-Ansari, N., Singh, K. K., Mahapatra, R. P., . . . Pham, B. T. (2020). Analysis of water pollution using different physicochemical parameters: A study of Yamuna River. Frontiers in Environmental Science, 8. https://doi.org/10.3389/fenvs.2020.581591
Siddiqui, Z. H., Wattal, R. K., Batchu, H., & Abbas, Z. K. (2020). Assessment of cytotoxic and genotoxic effects of Yamuna River water pollutants in an urban metropolis, Delhi (India). Water Supply, 20(7), 2682–2697. https://doi.org/10.2166/ws.2020.165
Singh, K. K., & Vaishya, R. C. (2016). Isolation of bacterial isolates from municipal wastewater for bioremediation of anionic surfactants. International Journal of Scientific Progress & Research, 23(3), 181–185.
Sundararaman, S., Kumar, J. A., Deivasigamani, P., & Devarajan, Y. (2022). Emerging pharma residue contaminants: Occurrence, monitoring, risk and fate assessment–A challenge to water resource management. Science of the Total Environment, 825, 153897.
Thathola, P., Agnihotri, V., Pandey, A., & Upadhyay, S. K. (2022). Biodegradation of bisphenol A using psychrotolerant bacterial strain Pseudomonas palleroniana GBPI_508. Archives of Microbiology, 204(5), 272. https://doi.org/10.1007/s00203-022-02885-y
Verma, S., & Chatterjee, S. (2021). Biodegradation of profenofos, an acetylcholine esterase inhibitor by a psychrotolerant strain Rahnella sp PFF2 and degradation pathway analysis. International Biodeterioration & Biodegradation, 158, 105169. https://doi.org/10.1016/j.ibiod.2020.105169
Verma, S., Singh, D., & Chatterjee, S. (2021). Malathion biodegradation by a psychrotolerant bacteria Ochrobactrum sp. M1D and metabolic pathway analysis. Letters in Applied Microbiology, 73(3), 326–335. https://doi.org/10.1111/lam.13517
Yeldho, D., Rebello, S., & Jisha, M. (2010). Plasmid-Mediated Biodegradation of the Anionic Surfactant Sodium Dodecyl Sulphate, by Pseudomonas aeruginosa S7. Bulletin Of Environmental Contamination And Toxicology, 86(1), 110–113. https://doi.org/10.1007/s00128-010-0162-2
Yilmaz, F., & Icgen, B. (2014). Characterization of SDS-degrading Delftia acidovorans and in situ monitoring of its temporal succession in SDS-contaminated surface waters. Environmental Science and Pollution Research, 21(12), 7413–7424. https://doi.org/10.1007/s11356-014-2653-x
Zargar, A.R. (2019). Hindu festival in India marred by a river of toxic foam and a blanket of killer smog. CBS News, 5th November. Available at: https://www.cbsnews.com/news/yamuna-rivers-toxic-foam-and-delhi-air-pollution-greet-india-hindu-devotees-for-chhath-puja-festival
Zhu, X., Wang, Z., Sun, Y., Gu, L., Zhang, L., Wang, J., Huang, Y., & Yang, Z. (2020). Surfactants at environmentally relevant concentrations interfere the inducible defense of scenedesmus obliquus and the implications for ecological risk assessment. Environmental Pollution, 261, 114131. https://doi.org/10.1016/j.envpol.2020.114131
Acknowledgements
The authors are grateful to the Founder President of Amity University, Dr. Ashok K Chauhan for his constant support and encouragement. The research was supported by the Strategic Academic Leadership Program of the Southern Federal University ("Priority 2030").
Author information
Authors and Affiliations
Contributions
JA, AC, AR: Conceptualization; JA, AR, AC: Methodology; JA, AC, AR, SJ, RP: Formal analysis; AC, TJ: Resources; JA, AC, AR: Data curation; JA: Writing-original draft preparation; AB, AR, AC, RB, SJ, RP: Writing-review and editing; VR, SS, EP, TM, SJ, RP: Critical review and editing; AC, AR, RP, TJ: Supervision. All the authors read and approved the final version of the manuscript.
Corresponding authors
Ethics declarations
Ethical approval
This article does not contain any studies with human participants or animals.
Consent to Participate
All authors agree mutually with the participation and publication of this work and declare that this is original research.
Consent to publish
All authors agree mutually to publication of this work.
Conflict of Interest
The authors report no financial or any other conflicts of interest in this work.
Additional information
Publisher's Note
Springer Nature remains neutral with regard to jurisdictional claims in published maps and institutional affiliations.
Rights and permissions
Springer Nature or its licensor (e.g. a society or other partner) holds exclusive rights to this article under a publishing agreement with the author(s) or other rightsholder(s); author self-archiving of the accepted manuscript version of this article is solely governed by the terms of such publishing agreement and applicable law.
About this article
Cite this article
Arora, J., Ranjan, A., Chauhan, A. et al. A Novel Study on Anionic Surfactant Degradation Potential of Psychrophillic and Psychrotolerant Pseudomonas spp. Identified from Surfactant-contaminated River Water. Appl Biochem Biotechnol 196, 2612–2629 (2024). https://doi.org/10.1007/s12010-023-04647-y
Accepted:
Published:
Issue Date:
DOI: https://doi.org/10.1007/s12010-023-04647-y