Abstract
Gentiana straminea Maxim. (MahuaQinjiao) is an important Chinese medicinal herb that is rich in secoiridoids. To increase the production of this plant, we have developed an efficient tissue culture system for high-frequency plant regeneration. We have also obtained plants with high secoiridoid monomer production through tissue culture and somatic embryogenesis. We have found that the MB medium is better than the B5 medium for callus induction. Calluses induced from immature seeds are superior to those from hypocotyls or young leaves in regeneration via somatic embryogenesis. We have also demonstrated that 2,4-dichlorophenoxyacetic acid is efficient for both callus induction and embryogenesis, indole-3-acetic acid is suitable for embryogenic callus proliferation, and N6-(benzyl)-adenine promotes both embryo development and the accumulation of gentiopicroside in the cultures. Regenerated plants have been selected for high gentiopicroside content. One plant contains 5.82% of gentiopicroside, which is higher than the control plants (1.20–3.73%). The regenerated plants are genetically more stable than the calluses based on both cytological and random amplified polymorphic DNA analyses.
Similar content being viewed by others
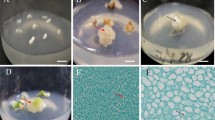
Avoid common mistakes on your manuscript.
Introduction
Gentiana straminea Maxim., a member of the Gentianaceae family, is an important medicinal plant in China. It grows in the wild on the Qinghai-Tibet plateau at an altitude of 2,500–4,700 m. It is a rich source of secoiridoid glucosides (Inouye and Nakamura 1971), including gentiopicroside, qinjiaoside, harpagoside, gentianine, gentianidine, gentianol, and swertiamarin (Liu et al. 2000; Wang and Zhou 2001; Ma et al. 2003), which are monomers possessing unique medicinal properties. Gentiopicroside is used to treat hepatitis by inhibiting the production of tumor necrosis, and it has a depressive effect on the central nervous system (Kondo et al. 1994). It also has anti-ulcerogenic properties and inhibitory effects on gastric secretion (Yamammura et al. 1978). Gentianine has been produced from the plant and sold as capsules for the treatment of these diseases.
Due to an increasing demand of plant-derived medicines, the supply of raw materials is inadequate. Recently, some of the wild G. straminea in the Qinghai-Tibet plateau has been destroyed by excavation. G. straminea’s seeds have a very low germination rate when they are introduced into low altitude regions. Thus, it is important to establish an efficient tissue culture system for high-frequency plant regeneration for the production of gentianine.
Somatic embryogenesis is considered to be an effective technique for plant regeneration because of its high-regeneration potential and low frequency of mutations. In the molecular and cellular development of somatic embryogenesis, plant growth regulators such as auxins and cytokinins have been shown to play significant roles (Dodeman et al. 1997; Charriere and Hahne 1998). Somatic embryogenesis has been described in some species of Gentiana, including Gentiana pneumonanthe L. (Bach and Pawlowska 2003), Gentiana kurroo Royle (Fiuk et al. 2003), Gentiana punctata L. (Mikuła et al. 2004), Gentiana cruciata L. (Mikuła et al. 2005a, b) Gentiana pannonica Scop. and Gentiana tibetica King (Mikuła and Rybczynski 2001), but the accumulation of effective components in them has not been reported to date. Additionally, only a few reports have described successful component production in regenerated plants from Saussurea involucrata Kar. et Kir. and Scrophularia nodosa L. (Guo et al. 2007; Sesterhenn et al. 2007). In this study, we report the effects of auxins and cytokinins on high-frequency somatic embryogenesis and plantlet regeneration of calluses induced from immature seeds of G. straminea and efficient production of a secoiridoid gentiopicroside from the embryonic calluses and regenerated plants.
Materials and Methods
Plant materials.
Immature capsules were collected from one G. straminea Maxim. plant growing in Yushu county of Qinhai Province, China. Voucher specimens were deposited in Qinhai Normal University. Immature seeds in the capsules were surface-sterilized in 70% (v/v) ethanol for 30 s followed by 0.1% aqueous mercuric chloride for 10 min. The seeds were rinsed with autoclaved distilled water four to five times. The young leaves and hypocotyls were germinated from the immature seeds, which were cultured on MB medium without hormone using the method described previously (Xiang et al. 1999).
Culture media and culture conditions.
Basal MB medium consisted of Murashige and Skoog (1962) inorganic salts, B5 vitamins (Gamborg et al. 1968), 3% (w/v) sucrose, 2 mg l−1 glycin, and 200 mg l−1 hydrolyzed casein as reported by Xia and Chen (1996). Various concentrations of 2,4-dichlorophenoxyacetic acid (2,4-D), indole-3-acetic acid (IAA), α-naphthaleneacetic acid (NAA), kinetin (KT), and N 6-(benzyl)-adenine (BA; Sigma-Aldrich Company, Shanghai, China) were used throughout these experiments. The response of the material on B5 medium was also examined. The different media combinations for induction, proliferation, and differentiation of the calluses were tested and shown in Tables 1 and 2. The pH of all the media was adjusted to 5.8 before autoclaving at 121°C at a pressure of 1.06 kg cm−2 for 20 min. All of the cultures were incubated in the dark or in a chamber with a 10–12-h photoperiod. A light intensity of 18–20 μmol m−2 s−1 was provided by cool-white fluorescent lamps.
Induction, proliferation, and differentiation of the calluses.
Young leaves and hypocotyls derived from the immature seeds were cut into pieces about 3 mm in length. The pieces mentioned above and some immature seeds were cultured on various induction media (Table 1). After 1 mo of culture, the induced calluses were transferred to proliferation medium (Table 2) for another 1–2 mo of subculture. Every experiment had at least three replicates and each experiment contained 20–25 explants. The embryogenic calluses were removed and transferred to differentiation medium (Table 2). After 1 mo of culture, the somatic embryos could germinate into plantlets. Regenerated plantlets of 3–5 cm in height were transferred to seedling-strengthening MB basal medium without hormones. Healthy plantlets were transplanted into soil.
Histological examination.
For histological observations, somatic embryos at different stages of development were fixed by FAA (50% ethanol/acetic acid/formaldehyde = 90:5:5), dehydrated in a gradient of ethyl alcohol, and then embedded in paraffin. The samples were cut into 8-μm sections with a rotary microtome (AO, Houston, TX) and the sections were stained with safraninine and fast green.
High-performance liquid chromatography analysis of gentiopicroside production. Yellow-green calluses and green embryogenic calluses were collected after 15 d of subculture on various media and ten regenerated plants had their gentiopicroside content measured. Regenerated plantlets, which were germinated from somatic embryos on differentiation medium containing 4.44 μM BA with the same growth status (vegetative state about 5 cm in height) were collected after 6 wk of subculture on differentiation medium. As controls, five wild plants were collected and analyzed. The plants were shade-dried for 1 wk and powdered. Three milliliters of methanol were added to 30 mg of the samples, and then the mixtures were sonicated for 1 h at room temperature. The extracts were filtered through a 0.22-μm membrane filter. An aliquot of 10 μl of each sample was injected for high-performance liquid chromatography (HPLC) analysis. The column was an Ultrasphere C18 column (150 mm × 4.6 mm i.d., 5 μm, Phenomenex, Torrance, CA). The mobile phase was water and methanol (70:30). The effluent was monitored at 270 nm and the flow rate was maintained at 1.0 ml min−1. The peak area of gentiopicroside was integrated by Class-vp5.0 system, with external standard. A standard calibration curve was plotted by using various concentrations of gentiopicroside (20–1,000 μg ml−1). Standard gentiopicroside was provided by the National Institute for the Control of Pharmaceutical and Biological Products (Beijing, China).
Cytological examination.
The embryogenic calluses and root tips of the regenerated plantlets were collected and pretreated in water (4°C) for more than 24 h. These samples were then fixed in acetic acid and ethyl alcohol (3:1) for at least 12 h. After rinsing in water for 20 min, the calluses and root tips were incubated in an enzyme mixture (1% cellulose Onozuka RS, 0.3% pectolyase Y-23, 0.6 M mannitol, 5 mM CaCl2, pH 5.8) at 37°C for 30 min, followed by gentle rinsing in water for 30 min. The pretreated material was then transferred to a clean slide and squashed to spread the chromosomes. The chromosome spreads were stained by standard aceto carmine methods (Xiang et al. 2003).
Random amplified polymorphic DNA analysis.
Genomic DNAs from the calluses and 23 regenerated plantlets were isolated by the CTAB method according to Doyle and Doyle (1990). Thirty-five random amplified polymorphic DNA (RAPD) primers (Operon Technology, Huntsville, AL; OPA1–10, OPJ1–10, OPH1–10 and OPF1–5) were used to amplify the DNA using the protocol described by Xia et al. (1998). Polymerase chain reaction (PCR) was performed with the following conditions: 45 cycles of 94°C for 10 s, 36°C for 30 s, and 72°C for 50 s. PCR products were separated on a 1.5% (w/v) agarose gel and detected by ethidium bromide staining.
Statistical analysis.
Data in each treatment were derived from the means of at least three replicates for growth rate analysis and inducing frequency of somatic embryogenesis analysis. All data were analyzed using a one-way analysis of variance (ANOVA) and comparisons between the mean values of treatments were made by the least significant difference test at the 5% level of probability using the software STATISTICA 6.0 (2001).
Results and Discussions
Effects of growth regulators, origin of explants, and culture media on embryogenic calluses.
Indirect in vitro somatic embryogenesis and plant regeneration depends mainly on the growth regulators, the origin of the explants, and the culture media. The immature seeds, hypocotyls, and young leaves could all produce calluses on either MB or B5 medium supplemented with different levels of 2,4-D (4.52–13.57) after 25 d of culture (Table 1; Fig. 1 a). Among the tested concentrations of 2,4-D, 13.57 μM was the best for callus induction. The frequencies of callus formation from immature seeds in MB and B5 were 92.3% and 68.9%, respectively. The frequencies of calluses formation from hypocotyls in MB and B5 media were 100% and 88.3%, respectively. The frequencies from young leaves were only 28.3% in MB and 20.0% in B5 media. These results indicated that MB was better than B5 for callus induction, and that the hypocotyls had the highest frequency of callus induction among the three kinds of explants. Calluses induced from both hypocotyls and young leaves were pale gray, fragile, and loose. They grew slowly and did not show embryogenesis. However, the calluses which originated from immature seeds appeared to be pale-yellow and had friable nodular structures (Fig. 1 b).
The growth rates of the embryogenic calluses were affected by the type of media, growth regulator, and the types of calluses (Table 2). The yellow-green calluses (proembryogenic calluses) and green embryogenic calluses proliferated at different rates on MB and B5 media with different growth regulators. Their fresh weights at the end of a 20-d subculture period are listed in Table 2. Among the different conditions we tested, the best condition for embryonic callus growth was 11.42 μM IAA in MB. The yellow-green calluses increased 10.52 ± 0.03 times, and the green calluses increased 3.31 ± 0.49 times by their fresh weight on this medium in 20 d. The yellow-green calluses were able to grow more vigorously than the green calluses on the same medium.
Effects of auxins and cytokinins on embryogenesis.
To investigate the effects of auxins and cytokinins on embryogenesis, the green and nodular calluses were separated and subcultured on fresh MB medium supplemented with different growth regulators at various concentrations either alone or in combinations (Table 3). The frequency of somatic embryos was affected by the concentrations of 2,4-D. Numerous somatic embryos developed on the surface of the embryogenic calluses on media # 2 and 3 (see Table 3). The highest frequency (88.4%) of somatic embryos on MB medium was obtained with 13.57 μM 2,4-D (Table 3, medium 3). It was reported that somatic embryogenesis needed a high concentration of auxins and cytokinins in early stages of embryo induction and development (Sharma et al. 2005). However, in this experiment, there was less embryogenesis on 2,4-D-containing medium with KT than without. Calluses became brown in the presence of KT (Table 3, media 4, 5, 6, and 7). IAA and NAA also induced embryogenesis when the calluses were subcultured for 2 wk, but they were less efficient than 2,4-D (Table 3, media 8, 9, 10, and 11). When the calluses were subcultured for 4 wk, IAA and NAA promoted maturation of the somatic embryos.
The exogenous auxins (2,4-D, NAA, and IAA) and cytokinins (KT and BA) are the main plant growth regulators used in plant tissue culture, but their influence on somatic embryogenesis is usually genotype-dependent. In general, auxins play a determinative role in the induction of embryonic cells. However, high concentrations of auxin restrain their further development (Ammirato 1983; Sunandakumari et al. 2005). Cytokinins, especially thidiazuron, could also induce somatic embryogenesis and multiple shoots (Gill and Ozias-Akins 1999; Mithila et al. 2003; Su et al. 2005). However, sometimes they were not effective in embryogenesis inductions (Sharma et al. 2005). The balance between auxins and cytokinins can also affect somatic embryogenesis (Ahloowalia 1987; Eapen and George 1993; Fulzele and Satdeve 2003; Sharma et al. 2005). We have shown that embryogenic calluses proliferation was affected by the type of explants. The most responsive explants were hypocotyls. We have also found that 13.57 μM 2,4-D was the most effective for promoting somatic embryogenesis among the different concentrations of 2,4-D, NAA, and IAA tested. Similar results were reported on gentian somatic embryogenesis (Ørnstrup et al. 1993; Tabira 1994). It is known that auxins (such as 2,4-D) are efficient for inducing cell division, while hormone-free medium or cytokinins (such as KT or BA) promotes development of embryos. 2,4-D and KT have been found to be efficient for the formation of embryos, and 2,4-D by itself is sufficient to initiate cell division and embryonic development up to the cotyledon stage (Ørnstrup et al. 1993; Tabira 1994). In our research, we noted that auxin (2,4-D) in combination with cytokinin (KT) decreased the frequency of induction of G. straminea embryos. Thus, somatic embryogenesis required auxin, while the cytokinin or auxin/cytokinin combination for the somatic embryo induction may depend on the species and the kinds of explants. Our results indicated that somatic embryo induction might be influenced by the concentrations of endogenous auxin or cytokinin of G. straminea.
Histological examination of the embryonic calluses.
Histological examination of the embryogenic calluses indicated that the somatic embryos were initiated either from the cortex or inside of the calluses by a single cell (Fig. 2 a–c). The process of induction of somatic embryos from calluses of G. straminea was very similar to that of other species in gentian (Cruz et al. 1990; Canhoto et al. 1996; Puigderrajols et al. 2001; Verdeil et al. 2001). Embryogenic calluses formed meristematic zones constructed by cells with a rich accumulation of starch in amyloplasts. The formation of somatic embryos began from single cells isolated from the surface meristematic layer. While this physical and physiological isolation of a single competent cell has a thick wall and lacks plasmodesmata (Yeung 1995), the cells acquiring ability to form proembryo could be observed in the meristematic zones (Fig. 2 a) at the surface or inner layer of calluses. It divided transversely to form a two-cell proembryo (Fig. 2 b), which divided vertically to form a three- or four-celled globular proembryo (Fig. 2 c). This was different from some related Getianaceae plants in which the zygotic embryo divides transversely and forms a four-celled linear proembryo (Li and Wang 1994; He et al. 2000). The division we observed was different from normal zygotic embryo division. The proembryo developed to globular embryo (Fig. 2 d), then to an intact embryo with both cotyledon and radicle (Fig. 3 e).
Histological section of embryogenic calluses. (a) Meristematic zones at the inner layer of calluses; MZ meristematic zones. (b) Two-cell proembryo on surface of calluses; Wp two-cell proembryo. (c) Three- or four-cell proembryo on the surface of the calluses; Tp three-cell proembryo; Fp four-cell proembryo. (d) Somatic embryo at globular shape. GE globular embryo. Bar = 50 μm.
Indirect somatic embryogenesis of G. straminea. (a) Proembryos on the surface of the green nodular structure after 7 d of subculture on 2# medium (see Table 2). (b) Proembryos on the surface of yellow-green embryogenic calluses after 7 d of subculture on 2# medium. (c) Heart-shaped embryo and multiple torpedo embryos on 14# medium after 5 d of subculture. (d) Germinating embryo with secondary global embryos on its surface after 15 d of subculture on 14# medium. (e) Germinating plantlets with normal cotyledons and radicle after 15 d of subculture on 14# medium. (f) Abnormal plantlet after 4 wk of subculture on 10# medium showing cluster roots and small buds. (g) Multiple embryos with abnormal cotyledons on 15# medium after 15 d of subculture. (h) Plantlets on 14# medium after 6 wk of subculture. Arrow, the globular-shaped proembryos. Bar = 3 mm.
Effects of auxins and cytokinins on the maturation and germination of the young embryos.
The somatic embryos gradually became pale when they were maintained on the 13.57-μM 2,4-D-containing MB medium. After transfer to MB medium with 9.05 μM 2,4-D, the young embryos in most of the cultures only developed to proembryos (Fig. 3 a, b). The proembryos grew and developed to maturity when they were subcultured on hormone-free MB medium or supplemented with BA (Fig. 3 c–e). The addition of 2.22 μM BA was the most effective for the maturation and germination of the embryos. However, some of the cotyledons from the mature plantlets were abnormal (Fig. 3 g). This might be a result of the high concentrations of hormone, as reported in Cucumis melo L. (Mohiuddin et al. 1998) and Dicentra spectabilis (L.) Lem. (Lee and Lee 2003). IAA and NAA treatment also caused embryos to mature when they were subcultured for 4 wk, but all of the embryos were abnormal with clustered roots and small buds (Fig. 3 f). In general, somatic embryogenesis of G. straminea required a high concentration of 2,4-D, but further growth and maturation of the embryos required the presence of BA or low levels of IAA and NAA.
Effects of auxins and cytokinins on gentiopicroside production by the embryogenic calluses and plantlets.
The amount of gentiopicroside produced by the yellow-green and green embryogenic calluses that were subcultured on different media was measured by RP-HPLC analysis (Table 4; Fig. 4 a–c). The concentration of gentiopicroside was higher in the green calluses than in the yellow-green calluses on MB medium with different concentrations of IAA and NAA. The highest production was 1.68% in green calluses on MB with 4.44 μM BA, and 0.03% in yellow-green proembryogenic calluses on MB with 11.42 μM IAA (Table 4). This revealed that BA treatment was more beneficial to gentiopicroside formation than NAA, IAA, or 2,4-D. This might be because auxins, especially 2,4-D, have a lower capacity for inducing callus differentiation than cytokinins. It was confirmed that differentiation of the calluses was favorable for the accumulation of gentiopicroside in this assay. In summary, a two-step method was suitable for gentiopicroside accumulation: (1) embryonic calluses were cultured on medium supplemented with 11.42 μM IAA for rapid growth, and (2) abundant calluses were transferred to medium supplemented with 4.44 μM BA for gentiopicroside generation.
The amounts of gentiopicroside were measured in parts of the regenerated plantlets (Table 5), and there were changes in different regenerated plantlets. The highest was 5.82%, which was about 20-fold higher than the lowest amount and much higher than the average reported for wild plants (0.75–3.49%; Miyakawa et al. 1997; Ma et al. 2003) and was also higher than the control plants (1.20–3.73%). The average amount was 1.69%, which was similar to what was produced by the green calluses on BA-containing media.
The variations in the amounts of efficient components among different plants of one population were also reported on iridiod content of S. nodosa L. by Sesterhenn et al. (2007). It was due to that the accumulation of a secondary metabolite depends on the equilibrium between the biosynthesis, transport, storage, and degradation processes and that they can therefore change in ways that are specific to tissues, organs, or developments (Wink 2003).
Somaclonal variation of the embryogenic calluses and regenerated plantlets.
Somaclonal variation commonly occurs during in vitro culture, and this can lead to the phenotypic variation due to elimination and recombination of the chromosome/DNA (Lee and Phillips 1988; Evans 1989; Doğramaci-Altuntepe et al. 2001). In this experiment, the chromosomes of the embryogenic calluses and the root tips of regenerated plants were analyzed. Most of the cells in the embryogenic calluses contained 20–26 chromosomes (Fig. 5 a, b), while 90% of the cells in the root tip of the regenerated plants had 25–26 chromosomes (Fig. 5 c; Table 6). These results indicated that chromosome variation occurs in the embryogenic calluses and regenerated plantlets, and the chromosome number present in the cells from regenerated plants was more stable than those from the embryonic calluses. However, most of the cells were stable with regard to chromosome number.
Analysis of the stability of the embryogenic calluses and plantlets. (a) Chromosomes from the green embryogenic callus no. 2 (2n = 26). (b) Chromosomes from a plantlet root tip of no. 3 (2n = 26). (c) Chromosomes from the yellow-green embryonic callus (2n = 25). (d, e) RAPD patterns of different regenerated plantlets, calluses, and wild plants using primers OPF5 (CCGAATTCCC) and OPH1(GGTCGGAGAA). M marker λEcoRI/HindIII, W wild plant, C yellow-green embryogenic calluses, 1–12 different regenerated plantlets from nos. 1 to 12. Bar = 3 μm.
RAPD profiles were also used to check genomic variation (Fig. 5 d, e). Four of the 35 primers we used revealed that all of the plantlets had the same bands, while the embryonic calluses retained about 95% of the RAPD patterns of their parent. Therefore, 5% of the loci in the embryonic calluses showed varying degrees of DNA recombination or exclusion. This was consistent with our results from chromosome analysis.
In conclusion, our major purpose was to compare the effects of different auxins and cytokinins on high-frequency plant regeneration via somatic embryogenesis from suitable explants and to promote gentiopicroside accumulation in the embryonic calluses and regenerated plantlets. The results of this work showed that BA alone in MB medium both promoted embryo development and gentiopicroside accumulation (Tables 2 and 3). Through this research, we have developed a simple and effective system for plant regeneration with high content gentiopicroside, via high-frequency somatic embryogenesis. Large numbers of plantlets were transferred to soil and eventually grown to maturity. It will be important for the production of gentianine.
References
Ahloowalia B. S. Somatic embryogenesis and plant regeneration in Eruca sativa. Crop Sci. 27: 813–814; 1987.
Ammirato P. V. Embryogenesis. In: Evans D. V.; Sharp W. R.; Ammirato P. V.; Yamada Y. (eds) Handbook of plant cell culture, techniques for propagation and breeding, vol 1. Macmillan, New York, pp 82–83; 1983.
Bach A.; Pawlowska B. Somatic embryogenesis in Gentiana pneumonanthe L. Acta Biol. Crac., Ser. Bot. 45: 79–86; 2003.
Canhoto J. M.; Mesquita J. F.; Cruz G. S. Ultrastructural changes in cotyledons of pineapple guava (Myrtaceae) during somatic embryogenesis. Ann. Bot. 78: 513–521; 1996. doi:10.1006/anbo.1996.0149.
Charriere F.; Hahne G. Induction of embryogenesis versus caulogenesis on in vitro cultured sunflower (Halianthus annuum L.) immature zygotic embryo: role of plant growth regulators. Plant Sci. 137: 63–71; 1998. doi:10.1016/S0168-9452(98)00128-9.
Cruz G. S.; Canhoto J. M.; Abreu M. A. V. Somatic embryogenesis and plant regeneration from zygotic embryos of Feijoa sellowiana Berg. Plant Sci. 66: 263–270; 1990. doi:10.1016/0168-9452(90)90212–7.
Dodeman V. L.; Ducreux G.; Kreis M. Zygotic embryogenesis versus somatic embryogenesis. J. Exp. Bot. 48: 1493–1509; 1997.
Doğramaci-Altuntepe M.; Peterson T. S.; Jauhar P. P. Anther culture-derived regenerants of durum wheat and their cytological characterization. J. Heredity 92: 56–64; 2001. doi:10.1093/jhered/92.1.56.
Doyle J. J.; Doyle J. I. Isolation of plant DNA from fresh tissue. Focus 12: 13–15; 1990.
Eapen S.; George L. Somatic embryogenesis in peanut: influence of growth regulators and sugars. Plant Cell Tissue Organ Cult. 35: 151–156; 1993. doi:10.1007/BF00032964.
Evans D. A. Somaclonal variation-genetic basis and breeding applications. Trends Genet. 5: 46–50; 1989. doi:10.1016/0168-9525(89)90021-8.
Fiuk A.; Rajkewicz M.; Rybczynski J. J. In vitro culture of Gentiana kurroo Royle. Biotechnologia 3: 267–274; 2003.
Fulzele D. P.; Satdeve R. K. Somatic embryogenesis, plant regeneration, and evaluation of camptochecin content in Nothapodytes foetida. In Vitro Cell. Dev. Biol. Plant 39: 212–216; 2003. doi:10.1079/IVP2002368.
Gamborg O. L.; Miller R. A.; Ojina K. Nutritional requirements of suspension cultures of soybean root cells. Exp. Cell Res. 50: 151–158; 1968. doi:10.1016/0014-4827(68)90403-5.
Gill R.; Ozias-Akins P. Thidiazuron-induced highly morphogenic calli and high frequency regeneration of fertile peanut (Arachis hypogaea L.) plants. In Vitro Cell. Dev. Biol. Plant 35: 445–450; 1999. doi:10.1007/s11627-999-0066-1.
Guo B.; Gao M.; Liu C. Z. In vitro propagation of an endangered medicinal plant Saussurea involucrata Kar. et Kir. Plant Cell. Rep. 26: 261–265; 2007. doi:10.1007/s00299-006-0230-6.
He T. N.; Chen S. L.; Liu J. Q.; Hong D. Y. Embryology of Gentiana striata (Gentianaceae). Acta Botanica Boreali-Occidentalla Sinica 20: 960–967; 2000.
Inouye H.; Nakamura Y. Studies on monoterpene glucosides and related natural products. XVI. Occurrence of secoiridoid glucosides in gentianaceous plants especially in the genera Gentiana and Swertia. Yakugaku Zasshi 91: 755–759; 1971.
Kondo Y.; Takano F.; Hojo H. Suppression of chemically and immunologically induced hepatic injuries by gentiopicroside in mice. Planta Med. 60: 414; 1994. doi:10.1055/s-2006-959521.
Lee K. P.; Lee D. W. Somatic embryogenesis and plant regeneration from seeds of wild Dicentra spectabilis (L.) Lem. Plant Cell. Rep. 22: 105–109; 2003. doi:10.1007/s00299-003-0642-5.
Lee M.; Phillips R. L. The chromosomal basis of somaclonal variation. Annu. Rev. Plant Physiol. Plant Mol. Biol. 39: 413–437; 1988. doi:10.1146/annurev.pp.39.060188.002213.
Li H.; Wang Y. Z. Embryological studies in Gentiana macrophylla. Acta Botanica Boreali-Occidentalla Sinica 14: 243–248; 1994.
Liu H.; Wang K. T.; Zhao Y. K.; Zhang H. Y.; Chen X. G.; Hu Z. D. Identification and determination of active components in Gentiana rigescens Franch. by micellar electrokinetic chromatography. J. High Resolut. Chromatogr. 23: 697–698; 2000. doi:10.1002/1521-4168(20001201)23:12<697::AID-JHRC697>3.0.CO;2-D.
Ma X.; Chen X. G.; Hu Z. D. Gentiopicroside content comparative of eight species of Qinjiao produced in Gansu province. Journal of Chinese Medical Materials 26: 85–86; 2003.
Miku; a A.; Fiuk A.; Rybczynski J. J. Induction, maintenance and preservation of embryogenic competence of Gentiana cruciata L. cultures. Acta Biol. Crac. 47: 227–236; 2005a.
Miku; a A.; Rybczynski J. J. Somatic embryogenesis of Gentiana genus I. The effect of the preculture treatment and primary explant origin on somatic embryogenesis of Gentiana cruciate L., G. pannonica Scop., and G tibetica King. Acta Physiol. Plant 23: 15–25; 2001. doi:10.1007/s11738-001-0017-x.
Miku; a A.; Tykarska T.; Mieczyslaw K.; Rybczynski J. J. Somatic embryogenesis of Gentiana cruciata L.: histological and ultrastructural changes in seedling hypocotyls explant. In Vitro Cell. Dev. Biol. Plant 41: 686–694; 2005b. doi:10.1079/IVP2005678.
Miku; a A.; Tykarska T.; Zielin S.; Kuras M.; Rybczynski J. J. Ultrastructural changes in zygotic embryos of Gentiana punctata L. during callus formation and somatic embryogenesis. Acta Biol. Crac., Ser. Bot. 46: 1–12; 2004.
Mithila J.; Hall J. C.; Victor J. M. R.; Saxena P. K. Thidiazuron induces shoot organogenesis at low concentrations and somatic embryogenesis at high concentrations on leaf and petiole explants of African violet (Saintpaulia ionantha Wendl.). Plant Cell Rep. 21: 408–414; 2003.
Miyakawa T.; Osnima T.; Hirayama H.; Sayara K.; Ma L.; Wu F.; Song W. Z. Determination of two main bitter secoiridoid glycosides in Gentianaceae. Chinese Journal of Pharmaceutical Analysis 17: 241; 1997.
Mohiuddin A. K. M.; Chowdhury M. K. U.; Abdullah Z. C.; Harikrishna K.; Napis S. Factors affecting in vitro adventitious shoot regeneration of muskmelon (Cucumis melo L.) cv birdie. Sci. Int. 10: 143–146; 1998.
Murashige T.; Skoog F. A revised medium for rapid growth and bioassays fro tobacco tissue cultures. Physiol. Plant 15: 473–497; 1962. doi:10.1111/j.1399-3054.1962.tb08052.x.
Ørnstrup H.; Mrlgaard J. P.; Farestveit B. Somatic embryogenesis and plant regeneration from cell suspensions of Exacum affine. Plant Cell Tissue Organ Cult. 35: 37–41; 1993. doi:10.1007/BF00043937.
Puigderrajols P.; Mir G.; Molinas M. Ultrastructure of early secondary embryogenesis by multicellular and unicellular pathways in Cork Oak (Quercus suber L.). Ann. Bot. 87: 179–189; 2001. doi:10.1006/anbo.2000.1317.
Sesterhenn K.; Distl M.; Wink M. Occurrence of iridoid glycosides in in vitro cultures and intact plants of Scrophularia nodosa L. Plant Cell Rep. 26: 365–371; 2007. doi:10.1007/s00299-006-0233-3.
Sharma P.; Koche V.; Quraishi A.; Mishra S. K. Somatic embryogenesis in Buchanania lanzan spreng. In Vitro Cell. Dev. Biol. Plant 41: 645–647; 2005. doi:10.1079/IVP2005680.
Su Y. J.; Chen J. T.; Chang W. C. Efficient and repetitive production of leaf-derived somatic embryos of Oncidium. Biol. Plant 50: 107–110; 2005. doi:10.1007/s10535-005-0081-y.
Sunandakumari C.; Zhang C. L.; Martin K. P.; Slater A.; Madhusoodanan P. V. Effect of auxins on indirect in vitro morphogenesis and expression of gusA transgene in a lectinaceous medicinal plant, Euphorbia nivulia Buch-Ham. In Vitro Cell. Dev. Biol. Plant 41: 695–699; 2005. doi:10.1079/IVP2005651.
Tabira H. Development of micropropagation system of Gentiana triflora. International Horticultural Congress, Kyoto, pp 186–187; 1994.
Verdeil J. L.; Hocher V.; Huet C.; Grosdemange F.; Escoute J.; Ferriere N.; Nicole M. Ultrastructural changes in coconut calli associated with the acquisition of embryogenic competence. Ann. Bot. 88: 9–18; 2001. doi:10.1006/anbo.2001.1408.
Wang N.; Zhou L. General situation of chemical components studying of Gentiana genus. Shangxi For. Sci. Technol. 3: 71–78; 2001.
Wink M. Evolution of secondary metabolites from an ecological and molecular phylogenetic perspective. Phytochemistry 64: 3–19; 2003. doi:10.1016/S0031-9422(03)00300-5.
Xia G. M.; Chen H. M. Plant regeneration from intergeneric somatic hybridization between Triticum aestivum and Leymus chinensis. Plant Sci. 120: 197–203; 1996. doi:10.1016/S0168-9452(96)04492-5.
Xia G. M.; Li Z. Y.; Wang S. L.; Xiang F. N.; Liu J. Y.; Chen P. D.; Liu D. J. Asymmetric somatic hybridization between haploid wheat and UV irradiated Haynaldia villosa. Plant Sci. 37: 217–223; 1998.
Xiang F. N.; Xia G. M.; Chen H. M. Effect of UV dosage on somatic hybridization between common wheat (Triticum aestivum L.) and Avena sativa L. Plant Sci. 164: 697–707; 2003. doi:10.1016/S0168-9452(03)00021-9.
Xiang F. N.; Xing M. Q.; Xia G. M.; Chen H. M. Tissue culture and antihepatitis constituent in calli of Swertia franchetiana H. Smith. Bulletin Bot. Res. 19: 256–259; 1999.
Yamammura J.; Konishima T.; Sawada T. Biologically active principles of crude drugs, pharmacological actions of Swertia japonica extracts, sweriamarin and gentianine. Yakugaku Zasshi 98: 1446; 1978.
Yeung E. C. Structural and developmental patterns in somatic embryogenesis. In: Thorpe T. A. (ed) In vitro embryogenesis in plants. Kluwer Academic, Dordrecht, pp 205–247; 1995.
Acknowledgments
This study was supported by the National Basic Research Program of China, No. 2007CB948203; the National Natural Science Foundation of China, Nos. 30470154 and 30771116; New Century Training Program Foundation for Talents by the Ministry of Education, No. NCET-05-0581; and the Doctor Station Foundation Fellowship Chinese Natural Education Ministry, No. 20050422015. The authors are grateful to Roberta Greenwood and Weichang Yu for editing this manuscript.
Author information
Authors and Affiliations
Corresponding authors
Additional information
Editor: Neftali Ochoa-Alejo
Rights and permissions
About this article
Cite this article
Cai, Y., Liu, Y., Liu, Z. et al. High-frequency embryogenesis and regeneration of plants with high content of gentiopicroside from the Chinese medicinal plant Gentiana straminea Maxim.. In Vitro Cell.Dev.Biol.-Plant 45, 730–739 (2009). https://doi.org/10.1007/s11627-009-9225-7
Received:
Accepted:
Published:
Issue Date:
DOI: https://doi.org/10.1007/s11627-009-9225-7