Abstract
The current study determined, for the first time, the levels of titanium (Ti), strontium (Sr), barium (Ba), vanadium (V), gallium (Ga), tin (Sn), thallium (Tl), and antinomy (Sb), in deposited dust, and human hair collected from general population of different geographical areas of Pakistan. All the samples were prepared by microwave digestion and measured by ICP-MS. The results showed that on deposited dust samples, the detected elements followed the descending trend as: Ti > Sr > Ba > V > Ga > Sn > Tl > Sb similar to the upper continental crust. The deposited dust samples from low elevation areas exhibited highest levels of all studied elements (except antimony which was higher in soil samples from mountainous areas), followed by rive plains, mountainous areas, and highland valleys. In contrast, on human hair samples, the elements followed the descending trend as: Sr > Ba > Ti > Ga > V > Sn > Sb > Tl respectively. Ba, Ga, and V concentrations were higher in soil samples from lower elevation Indus plain, and Sr, Tl, Sb, and Ti were higher in samples from mountainous areas. The bioaccumulation trend of all studied elements was in descending order as follows: Sb, Ga, Sn, Ba, Sr, Ti, V, Tl, respectively. Principal component analysis (PCA) and correlation matrix evidenced both geological influences and anthropogenic activities as potential sources of these studied elements. On the other hand, the risk estimation (HI > 1) concluded that population were at higher health risk (non-carcinogenic) for Ga and Ti. All other studied rare elements were within safe limit for humans from all zones.
Similar content being viewed by others
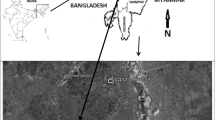
Explore related subjects
Discover the latest articles, news and stories from top researchers in related subjects.Avoid common mistakes on your manuscript.
Introduction
Human health risk caused by environmental exposure to heavy metals, metalloids, and rare earth elements has obtained more and more attention in the last decades (Rodriguez et al. 2004; Mohmand et al. 2015, Alamdar et al. 2016). Trace elements occur in the environment naturally and are also released as a result of anthropogenic activities (Zheng et al. 2013; Huang et al. 2014). Soil contamination results for example from human-related activities such as mining (Moreno et al. 2013), soil (Yoshinaga et al. 2014), traffic exhaust (Kousoulidou et al. 2008), combustion of heavy fuel oil (Sanchez de la Campa et al. 2011) Pandolfi et al. 2011), fireworks, industrial and sewage emission, agriculture activities (Amato et al. 2009; Eqani et al. 2016), electronics waste, and plastic toys (Guney and Zagury 2013; Korfali et al. 2013).
Due to its small particle size, re-suspension in wind, rapid and frequently cross continental boundaries and tendency to accumulate in downward areas (Pelletier 2006; Okorie et al. 2012), soil dust can enter the body and affect human health directly by ingestion, inhalation, and dermal contact (Eqani et al. 2016). The non-degradable and persistent nature of the metal ions results in longer exposure and accumulation of these elements (Mohmand et al. 2015). However, bioaccumulation of different toxic elements into human body mostly depends on different routes, frequency and source of exposure, bioavailability, physical and chemical properties, particle size, and solubility (ATSDR 2012; Mohmand et al. 2015). Trace elements biomonitoring is a well-accepted tool for the study of human exposure (i.e., occupational, residential) and environmental safety assessment from different areas of the world. Among the possible biomarkers in biological media, hair is an attractive choice, because it is easily and non-invasively collected, inexpensive, and easily stored and transported to the laboratory for the analysis (Klevay et al. 2004; Mohmand et al. 2015). Hair is composed of a protein, consisting of cysteine sulphydyl (thiol) groups, which can bind various elements (McLean et al. 2009; Oliveira et al. 2010; Noguchi et al. 2012). Moreover, human hair grows at a rate of approximately 1 cm/month, allowing the long-term monitoring of long and recent contaminant exposure (Mohmand et al. 2015).
The investigation of trace elements in soil dust samples in Pakistan is of great interest due to intensive industrial and agricultural activities and also because soil contamination can affect groundwater and surface water sources, plants, animals, and humans. For this reason, it is fundamental to evaluate the level of soil contamination and the determination of its potential health risk for children and adults. Although some studies have addressed the heavy metal contamination in dust and hair samples in Pakistan in the last few years (Ashraf and Jaffar 1997; Khalique et al. 2005; Khalique et al. 2006; Faiz et al. 2009; Bukhari et al. 2012), no study has dealt with the investigation of the levels of some rare elements but still under certain circumstances potentially toxic, such as antimony, barium, gallium, strontium, thallium, titanium, tin, and vanadium in the same matrices. In addition, all of these elements are also present in the continental crust (Rudnick and Gao 2003). In our pilot country wide monitoring project, we reported the levels of selected heavy metals into dust, water, and human samples (Mohmand et al. 2015; Eqani et al. 2016; Alamdar et al. 2016), and these studies reflected the severe environmental degradation into different areas of Pakistan. Attributed to both anthropogenic activities and natural sources into different areas of Pakistan, the scenario stresses the need for comprehensive reporting of missing documented elements (i.e., antimony, barium, gallium, strontium, thallium, titanium, tin, and vanadium) at country level.
Thus, the main objectives of this study were (a) to characterize the occurrence and levels of the aforementioned rarely reported trace elements in soil dust (deposited) samples and hair samples of residents living within four different geographical areas of Pakistan and (b) to evaluate the human health risk exposed to deposited dust borne trace elements.
Materials and methods
Study area description
Pakistan is located between the latitudes of 24° and 37° North and longitudes of 61° and 75° East, with a total area of 796,095 km2, of which 1600 km from North to South and 885 km from East to West. Pakistan is characterized by a diverse climatic condition, topography, and geography. The geography of Pakistan is a profound blend of landscapes, varying from agricultural plains to desert, forests, hills, and plateaus, with different climatic conditions. In the current study, the different regions of Pakistan were categorized into four major zones on the basis of their geographical characteristics and altitudes: Frozen Mountain Zone (FMZ), Lower Himalayan Wet Mountainous zone (WMZ), Riverine Delta Zone (RDZ), and Low Lying Zone (LLZ) (see Fig. 1). More details about the study area and sampling sites are given elsewhere (Alamdar et al. 2016; Eqani et al. 2016).
Sample collection
Deposited soil dust (n = 110) and hair samples (n = 180) were collected, between May and June 2013, from selected (n = 21) site stations throughout the country. In our study, we collected dust samples in a room with four ways cross window at each site and a wooded table with mirror on top had placed inside each room. The outdoor dust that had settled on the mirror surface was periodically brushed off and collected in the sampling bags. Deposited soil dust sampling details are given elsewhere (Alamdar et al. 2016; Eqani et al. 2016). Hair samples were collected using non-metallic scissor, from both genders. Hair samples (~ 3 cm) were taken from occipital region or from the front hair-line (Mohmand et al. 2015). Hair samples were collected from volunteers (60 females and 120 males), aged between 20 and 50 years, living in the four selected Pakistani areas. After sampling, all hair and dust samples were stored in air tight sealed plastic bags and transferred to Institute of Urban Environment, Chinese Academy of Science (China). The study protocol was approved by the Ethical Committee of Biosciences Department, COMSATS Institute of Information and Technology, Islamabad, Pakistan. Under the informed written consent, all participants filled a questionnaire including basic personal information (such as gender, date of birth, occupation), lifestyle (including smoking and drinking habits), and information regarding spraying, dying, and other treatments to the hairs.
Sample analysis
All the reagents were of analytical reagent grade: nitric acid GR grade 65% (CNW Corporation, Shanghai, China); perchloric acid (HCLO4); hydrogen peroxide (H2O2, 30% v/v) GR grade (Sinopharm Chemical Reagent Co., Ltd., Beijing, China); and Ultrapure water obtained from a Milli-Q system (Millipore Corporation, Billerica, MA, USA). All the glassware was washed with mild detergent and soaked in 10% (v/v) HNO3 overnight and rinsed three times with Ultra-pure water before use. Moreover, the microwave digestion tubes were cleaned with HNO3, in order to ensure the cleanliness and to avoid cross-contamination.
For digestion of dust, accurately weighed portions (about 0.1 ± 0.02 g) were treated with 2 mL HNO3: HCLO4 (v/v = 1/1) overnight and then 1 mL of H2O2 was added after 24 h. For the hair samples, 2 mL HNO3 (overnight) and then 1 mL of H2O2 were used for the digestion. Samples were digested in an accelerated microwave digestion system (Mars CEM, CEM Corporation, Matthews NC, USA) at 800 W, 120 °C for 10 min and then 800 W, 170 °C for 30 min. All digested samples were filtered by 0.22 μm Nylon membrane syringe filters, and final volume was adjusted to 50 ml using ultra-pure water (Mohmand et al. 2015). Inductively coupled plasma with mass spectroscopy (Agilent 7500cx ICP-MS, Agilent Technologies, Santa Clara, CA, USA) was used for analysis of titanium (Ti, mass = 47.867), vanadium (V, mass = 50.9415), gallium (Ga, mass = 69.723), strontium (Sr, mass = 87.62), tin (Sn, mass = 118.71), antimony (Sb, mass = 121.76), barium (Ba, mass = 137.327), and thallium (Tl, mass = 204.3833). The operating parameters of ICP-MS were set as follows: RF power 1510 W, flow rate of carrier, makeup, and helium gases were 1.1, 0.10, and 3.5 mL/min, respectively. The nebulizer pump was set at 0.1 rps. In order to check the instrumental sensitivity and stability, mixed internal standard (1 mg/L) was added to the extract before ICP-MS analysis (Agilent, ICP-MS Internal Standard Mix: Sc, Ge, Rh, Tb). The intensity of Sc, Ge, Rh, and Tb was steady throughout the experiment (RSD < 5%) suggested the sensibility and stability of signal. Moreover, polyatomic interferences having the same relationship (m/z) of the analytes of interest were controlled by using octopole set up with reaction mode “ON” and helium gas (4 mL/min).
QA/QC
Quality control (QC) procedure was carried out using a control sample prepared by mixing aliquots of each sample. After running of every 10 samples, the QC sample was injected to assess the instrumental stability. Spiked samples were also prepared by using the same method. The spiked sample concentration was at the two final levels, 10 and 20 ng mL −1. In the spiked samples before digestion, all elements Ti, V, Ga, Sr, Sn, Sb, Ba, and Tl were incorporated to measure the recovery percentage. Moreover, GSD-9 certified reference material (Chinese stream sediment - Trace elements, GBW07309) was also analyzed to confirm analytical performance and good precision [RSD < 18%]. The method recovery was assessed using the both spiked samples, and CRM was ranged between 90 and 105%. The diluting solution for working standards preparation was a mixture of HNO3 (65%), HCLO4, H2O2 (30%), and H2O (v/v/v/v) in a ratio of 1:1:1:3, respectively and was prepared daily. The working dilutions of different concentration were prepared from standard stock solution (100 μg/mL, GSB 04-1767-2004) of each element. All the samples were analyzed in duplicate. The relative difference for replicate analysis was < 5%. All samples were run in a randomized fashion to reduce the uncertainties from artifacts-related injection order and instrumental sensitivity changes during the entire sequence.
Estimation of daily intake and risk assessment
The estimation of chemical/element daily intake of selected elements through ingestion, inhalation, and dermal contact was calculated by following our earlier published report (Eqani et al. 2016).
Statistical analysis
Statistical analysis was conducted using SPSS software package v 20.0 (SPSS Inc., Chicago, IL, USA). A level of p < 0.05 was considered statistically significant for multiple comparisons and one-way ANOVA followed by Tukey’s HSD post hoc test was employed to study the multiple comparisons among different studied elements and different land-use types. Principal component analysis (PCA) was applied on zone-wise studied elements data, to identify the sources. Correlation matrix using Pearson’s moment correlation coefficient was also used to identify correlations between studied elements.
Results and discussion
Levels of rarely studied elements in deposited dust and human hair
The concentration (ppm) of the investigated elements (Ti, V, Ga, Sr, Sn, Ba, Tl, and Sb) in deposited dust samples are reported in Table S-1 and Fig. 2. According to Fig. 2, average concentrations in deposited soil dust samples were in the following order: Ti > Sr > Ba > V > Ga > Sn > Tl > Sb. In general, for all the trace elements, except for Sb and Tl, concentration levels showed values higher than 1 ppm. Considering the spatial patterns of the investigated elements, the highest concentrations were measured in the deposited soil dust samples collected from LLZ and WMZ. Ti was the predominant element in dust samples and was significantly (p < 0.05) the highest element in dust samples collected in LLZ (785 ppm) compared to those collected in WMZ (457 ppm), RDZ (321 ppm), and FMZ (304 ppm). Similarly, Sr showed significantly (p < 0.05) highest concentration levels in dust from LLZ (671 ppm) followed by WMZ (290 ppm), RDZ (263 ppm), and FMZ (158 ppm). Ba concentrations were highest in dust samples from LLZ (213 ppm), followed by WMZ (199 ppm), FMZ (171 ppm), and RDZ (135 ppm). However, not a significant difference (p > 0.05) for Ba was noted among the different zones. Vanadium (V) level was significantly (p < 0.05) higher from LLZ (41 ppm), followed in descending order by WMZ (21 ppm), RDZ (17 ppm), and FMZ (10 ppm). Ga showed the (p < 0.05) highest concentration from LLZ (15 ppm) as compared with those from WMZ (9 ppm), RDZ (7 ppm), and FMZ (0.8 ppm). Tin (Sn) was higher from LLZ (5 ppm) followed in decreasing order by RDZ (2.3 ppm), WMZ (2.1 ppm), and FMZ (1.6 ppm). Thallium (Tl) levels (ppm) were higher from LLZ (0.26), followed in descending order by FMZ (0.25), WMZ (0.25), and RDZ (0.18), respectively. Antimony (Sb) concentration were lower than other studied elements, though was highest from WMZ (0.14 ppm) and descended as LLZ (0.118 ppm), and FMZ (0.104 ppm), and finally from RDZ (0.09 ppm).
The comparison of the mean concentrations of rarely reported elements in dust samples found in the present study with those reported in different parts of the world are reported in Table 1 and are in the same order as present in the upper continental crust (Rudnick and Gao 2003). Our results showed that Ti, Sr, Ba, V, and Ga levels (ppm) were higher than those measured in developed countries (Fergusson et al. 1986; Rasmussen et al. 2001; Valotto et al. 2015) but similar to other Asian countries including China (Hu et al. 2011) and Japan (Yoshinaga et al. 2014). Nevertheless, Sb, Tl, and Sn concentration values were much lower than those determined elsewhere (Table 1).
The statistical concentrations hair data are reported in Table S-1 and Figs. 3 and S-3. Ba, Sr, Ti, and Ga were the most abundant elements observed in the human hair samples, with mean concentration levels > 1 ppm. The distribution of the studied elements followed the trend; Sr > Ba > Ti > Ga > V > Sn > Sb > Tl. Sr showed relatively higher levels (ppm) in human hair samples from RDZ (32.7), followed by LLZ (29.9), WMZ (15.8), and FMZ (9.5). The significantly (p < 0.05) highest concentrations of Tl were from RDZ (0.0048 ppm), followed by WMZ (0.0046 ppm), FMZ (0.004 ppm), and LLZ (0.002). Vanadium was highest in the hair sample collected from WMZ (0.8 ppm), followed in descending by FMZ (0.7 ppm), RDZ (0.5 ppm), and LLZ (0.4), respectively. Likewise, Ba and Ga concentrations (ppm) were highest in hair samples from WMZ (43, 6.9, respectively), followed in descending order by LLZ (8.9, 1.3, respectively), RDZ (5.4, 0.8, respectively), and FMZ (4.1, 0.7, respectively).
The distribution pattern of these elements is completely different from those observed in the soil dust samples. In contrast, Ti, Sn, and Sb levels (ppm) was significantly (p < 0.05) higher from FMZ (12, 0.52, 0.16), followed by WMZ (10, 0.37, 0.13), RDZ (8.6, 0.12, 0.05), and lowest from LLZ (3.0, 0.09, 0.04), respectively.
The comparison of current dataset with other global and regional studies (Table 1) have shown that the mean concentration of Ti, Ba, Ga, Sr, and Tl were relatively higher than those reported elsewhere (Table 1). However, V, Sb, and Sn were lower and/or comparable than those determined in Turkey (Cihan et al. 2011), China (Hu et al. 2011), and Poland (Chojnacka et al. 2006). In contrast, Sn levels from this study were lower than other reported for studies across the world.
Source identification of rarely studied elements
PCA has been used for the source identification and indication of the extent of pollution of studied elements into deposited dust of different zones (Fig. 4). PCA biplots (PC-1 and PC-2 explaining ~ 90% of variance) clearly highlighted the loading of all studied elements toward RDZ and LLZ with few exceptions of urban samples from WMZ reflecting the major contribution of anthropogenic sources in these areas. However, the majority of samples collected from FMZ and WMZ pointed toward the natural leaching process, which contributed toward dust contamination of studied elements (Eqani et al. 2016). RDZ includes important urban districts of the Punjab province (Pakistan) (i.e., Lahore, Gujaranwala, Sargodha, Bhakkar, Mainwali, Layyah, and Dera Ghazi Khan), which are facing severe environmental degradation problems due to their rapid urbanization (Eqani et al. 2016). Moreover, all this area is characterized by widespread agricultural activities, as well as industrial productions in the areas of Lahore and Gujranwala. Similarly, LLZ includes the Indus plain and the most important agricultural areas of Pakistan, which are characterized by hot arid/semi-arid climate, low rain falls, and frequent wind storms in summer. Moreover, LLZ contains urban areas, such as Hyderabad city, which are influenced by several anthropogenic activities, in particular industrial activities and heavy urban traffic, which may be important anthropogenic trace metals emission sources (Eqani et al. 2016). Nearly 100 million people of low socioeconomic status live in these areas, and they are potentially exposed to different environmental toxins during their rural farming livelihood and occupation.
A Pearson correlation was also used to confirm the possible existence of a relationship among analyzed elements into dust as well as their source of origin indicated by PCA. Table S-2 shows the strong correlation (p < 0.01) between the studied elements and sources, including natural and anthropogenic activities. According to literature studies, the presence of titanium in soil dust samples may be due to the contribution of automotive exhausts and industrial activities (i.e., steel, pharmaceutical, cosmetic, and alloy industries) (Rodriguez et al. 2004). Moreover, Ti is relatively abundant, ranking 8th in the list of chemical compounds occurring in the earth’s crust; thus, its presence could also be related to weathering and corrosion of mineral rocks and soil (de Miguel et al. 1997; Nava et al. 2012; Moreno et al. 2013). Sr is released into the atmosphere primarily as a result of natural sources, such as entertainment of dust particles, wind erosion, and soil re-suspension. Moreover, fertilizers and pesticide use may also contribute to the release of Sr in soil (ATSDR 2004; Perry 1999). Barium is a naturally occurring component of minerals that are found in small but widely distributed in the earth’s crust. It enters the environment naturally through the weathering of rocks (de Miguel et al. 1997), whereas anthropogenic releases are associated with industrial processes including mining, refining, coal ash, fuel combustion, diesel engines and vehicles exhaust, and paint and rubber industries, because it is used as smoke suppressant (Sternbeck et al. 2002; ATSDR 2004). Vanadium is the 22nd most abundant element in the earth’s crust, and it is released naturally to the atmosphere by the formation of continental dust, marine aerosols, and volcanic emissions. It has also crustal origin and thus enters into environment from weathering of rocks and soil erosion (Moreno et al. 2013). The most important anthropogenic sources of vanadium include the combustion of fossil fuels, particularly residual fuel oils, which constitute to the single largest overall release of vanadium to the atmosphere (Pandolfi et al. 2011). Pharmaceutical waste, fertilizers application, municipal sewage sludge, and mining effluents are further anthropogenic vanadium sources (Sanchez de la Campa et al. 2011; Moreno et al. 2013). The main sources of Ga in soil dust include electronics waste, recycling of elements raw material, and incineration of industrial waste (Chen et al. 2007; IMS Research 2012). Sn is released into atmosphere from both anthropogenic and natural sources. It can be released via road construction activities, agricultural activities, or via forceful windstorms. Moreover, effluents from smelting, refining, tin-industrial waste incineration and combustion of fossil fuels, textile mills also contributed toward Sn contamination in these areas (Senesi et al. 1999; ATSDR 2005). However, the levels did not significantly (p < 0.05) vary among the different investigated zones. Tl is fairly mobilized into environment, because it is reported to be present via adsorption on sludge and ultimately cause dust borne contamination into associated human and wildlife (Al-Najar et al. 2003). The main sources of thallium are insecticides (as thallium sulfate is used in rat poisons), electronic industry waste from solar cells, light sensitive crystals, IR detectors and medical imaging devices, coal mining, lead smelting, artificial jewelry, paints, fireworks, and cement factories (Wang et al. 2013; Turner and Furniss 2012; Rusyniak et al. 2002). The major contributor of antimony contamination released via anthropogenic activities (e.g., coal burning, smelting of antimony containing ores, abrasion from brakes, tires, and vehicles exhaust) (Adachi and Tainosho 2004; Krachler et al. 1999). Given the lower water solubility, it can be expected that antimony can be adsorbed onto soil particles and re-suspended into atmosphere as particulate materials from road dust and traffic vehicles (Quiroz et al. 2013).
The bioaccumulation factor of rarely studied elements
The bioaccumulation factors (BAF) of selected elements, calculated as the ratio between their concentration in soil dust and hair (Mohmand et al. 2015), are reported in Table 2. The BAF pattern of studied elements in hair is shown in Fig. 3 and follows the trend: Sb > Ga > Sn > Ba > Sr > V > Ti > Tl (Fig. 3). Sb and Ga (with few expectations from LLZ) have highest BAF values (0.55–1.5, 0.12–0.78, respectively). While BAF for Sn (0.32~FMZ and 0.18~WMZ), Sr (0.12~RDZ), and Ba (0.22~WMZ) were also higher at several described instances. BAF values for V, Ti, and Tl ranged between 0.01 and 0.05, and showed lower potential bioaccumulation. The different patterns of studied elements in hair bioaccumulation may be due to distinct factors such as geographical conditions, race, occupation, lifestyle, level of awareness, diet, socioeconomic conditions, and extent of anthropogenic activities of each particular region (Mikulewicz et al. 2013). Our results are also in agreement with those reported in a study conducted in Nigeria (Akan et al. 2012). The bioaccumualtion patterns of the studied elements showed that low socioeconomic status of general population and lack of awareness throughout the country may be mainly contributed toward higher bioaccumulation rates of these elements via different sources including contaminated food, water, and/or dust. However, bioaccumulation of studied elements may be mainly related to several industrial activities (i.e., glass, paint, and ceramics), construction, and renovation as well as occupational exposure in the studied areas. Moreover, other important sources as cement, smelting, insecticides, and fossil fuel combustion exhaust (Rusyniak et al. 2002; Turner and Furniss 2012) may play a key role.
Sb showed higher potential for bioaccumulation and followed by Ga, Sn, and Sr. Exposure to Sb has been reported to be associated with inflammation of lungs, bronchospasm, GIT ulcer, and blood pressure variation (Ross et al. 2009). Gallium was the second element having the highest BAF values, and it may lead to immune suppression, liver problems, pulmonary inflammation, and abnormalities in blood cell counts (Betoulle et al. 2002). The major health issues due to Sn are respiratory depression, cough, kidney damage, neurological disorders, and deafness (Saary and House 2002). Similarly, Sr exposure may cause anaphylactic reaction, extreme tachycardia, and lung cancer (ATSDR 2004).
On the other hand, the impact of barium exposure on human health is associated with silicosis, pulmonary lesions, elevation in blood pressure, and ECG abnormalities (ATSDR 2007). The higher levels of Vanadium in some cases may cause different health problems including alteration in erythrocytes and reticulocyte level, increased blood pressure, eye irritation, and conjunctivitis (ATSDR 2012). Titanium exposure may lead to irritation of mucous membrane of skin, eye, and respiratory tract, bronchitis and pneumonia, increase in neutrophils count, and decreased in lymphocytes (ATSDR 1997). Based on results from this study, thallium had the least tendency to bioaccumulate, although the health effects from thallium exposure are alopecia (hair loss), lethargy, ataxia, abdominal pain or vomiting, back pain, neuropathy, muscle weakness, coma, convulsion, abnormal vision, and sometime death (Riyaz et al. 2013; Lu et al. 2007).
In the current study, the studied elements bioaccumulation patterns showed that hair is a promising biomonitoring tool. Nevertheless, few limitations including application of cosmetic products such as shampoo, hair dyes, soaps, and others actually compete with the complexing ability of toxic elements to these reactive sites, thus leading to a significant leaching of elements from the shaft bulk of hair (Senofonte et al. 2000). Other factors such as age, gender, occupation, diet, and time of exposure to rare elements also influence on bioaccumulation in hair (Akan et al. 2012). However, human hair can also be used for diagnostic purpose to determine the metabolic disorders, environmental exposure, and nutritional status (Nowak and Chmielnicka 2002).
Human health risk assessment
Direct human exposure to contaminants in soil and dust can occur by different pathways such as unintentional inhalation, accidental ingestion, and/or via dermal contact. The human health risk assessment is an effective approach to provide a quantitative determination for future risk management and environmental monitoring of different environmental contaminants, including currently studied rare elements in dust. In order to assess both carcinogenic and non-carcinogenic risk for humans from soil dust ingestion, inhalation, and dermal contact, the chronic daily intake (CDI) and hazard quotients (HQs) of rarely studied elements were determined. The CDI sequence showed that the higher health exposure risk, both for humans, was through dust ingestion, followed by dermal contact and inhalation (Table S-4). In general, trends of CDIingestion > CDIdermal contact > CDIinhalation, and HQ and hazard index (HI) values lower than safe limit (HI < 1) are consistent with other studies conducted in China (Zheng et al. 2013; Huang et al. 2014), Japan (Yoshinaga et al. 2014) and Luanda (Ferreira-Baptista and De Miguel 2005).
The HQs, which were estimated by dividing the CDI values for each element by the oral reference dose (RfDo), are reported in Table S-5. The RfDo values for each element are listed in Table S-3. The HI, estimated as the sum of the HQs from different exposure pathways (such as HQingestion, HQD-contact, and HQ inhalation), is reported in Table 3. HQ of all elements except Ga (HQing and HQD-contact) from all exposure pathways (ingestion, dermal contact, inhalation) from all zones was within the safe limit (HQ < 1) for humans. The HI for individual elements, which is the sum of each hazard quotient from different exposure pathways, such as HQingestion, HQD-contact, and HQinhalation, are reported in Table 3 for all the investigated areas. The HI values observed for Ga and Ti for humans were always below the safe limit (HI < 1), except for the HI values of Ga for adults from LLZ (4.4), WMZ (2.5), and RDZ (1.9) and for children from LLZ (8), WMZ (6), RDZ (5), and FMZ (1.1). The HI for Ti was above safe limit (HI > 1) only for children from LLZ (1.2). Nevertheless, the HI values for the other elements, for both adults and children, in all the investigated areas, was below the safe limit (HI < 1), indicating that there is little adverse health risk. Adult health risk due to these elements was consistently lower than that of child. This trend may be due not only to the lower tolerance of the most sensitive sub-population to toxin and their less body weight (Acosta et al. 2009) but also to their hand to mouth activity, whereby contaminated dust can be readily ingested (Karim and Qureshi 2013).
Conclusions
The findings of the present study confirm the need to act and implement strategies to reduce trace element emissions aimed to protect exposed populations and the ecosystem. The human health risk assessment is an effective approach to provide a quantitative determination for future risk management and environmental monitoring of heavy metals, metalloids, and rare earth elements contamination in dust. The results of the current study showed that the potential health risk from the other studied elements for humans through dust could not be overlooked and should be investigated along with other sources of contamination (water and foods) into detail in future. The current study provided first detailed human biomonitoring baseline data along with the dust-borne contaminations, which would be very useful toward the environmental management of rarely reported elements from Pakistan.
References
Acosta JA, Cano AF, Arocena JM, Debela F, Martinez-Martinez S (2009) Distribution of metals in soil particle size fractions and its implication to risk assessment of playground in Murcia City (Spain). Geoderma 149:101–109
Adachi K, Tainosho Y (2004) Characterization of heavy metal particles embedded in tire dust. Environ Int 30:1009–1017
Akan JC, Chellube ZM, Abdulrahman FI, Waziri M (2012) Hair and nail heavy metal levels in residents from Maiduguri, Borno State, Nigeria: Influence of gender, age, occupationally exposure and smoking habit. Can J Pure Appl Sci 6(3):2143–2151
Alamdar A, Eqani SAMAS, Ali SW, Sohail M, Bhowmik AK, Subhani M, Ghaffar B, Ullah R, Cincinelli A, Huang Q, Shen H (2016) Human Arsenic exposure via dust across different ecological zones throughout the Pakistan. Ecotoxicol Environ Saf 126:219–227
Al-Najar H, Schulz R, Römheld V (2003) Plant availability of thallium in the rhizosphere of hyperaccumulator plants: a key factor for assessment of phytoextraction. Plant Soil 249(1):97–105
Amato F, Pandolfi M, Viana M, Querol X, Alastuey A, Moreno T (2009) Spatial and chemical patterns of PM10 in road dust deposited in urban environment. Atmos Environ 43:1650–1659
Ashraf W, Jaffar M (1997) Concentration of selected metals in scalp hair of an occupationally exposed population segment of Pakistan. Int J Environ Stud 51:313–321
ATSDR (Agency for Toxic Substances and Disease Registry) (1997) Toxicological profile for titanium. U.S. Department of Health and Human Services, Public Health Service. https://www.atsdr.cdc.gov/toxprofiles/tp.asp?id=495&tid=88
ATSDR (Agency for Toxic Substances and Disease Registry) (2004) Toxicological profile for strontium. U.S. Department of Health and Human Services, Public Health Service. https://www.atsdr.cdc.gov/toxprofiles/TP.asp?id=656&tid=120
ATSDR (Agency for Toxic Substances and Disease Registry) (2005) Toxicological profile for tin U.S. Department of Health and Human Services, Public Health Service. https://www.atsdr.cdc.gov/ToxProfiles/tp.asp?id=543&tid=98
ATSDR (Agency for Toxic Substances and Disease Registry) (2007) Toxicological profile for barium. U.S. Department of Health and Human Services, Public Health Service. https://www.atsdr.cdc.gov/toxprofiles/tp.asp?id=327&tid=57
ATSDR (Agency for Toxic Substances and Disease Registry) (2012) Toxicological profile for vanadium. U.S. Department of Health and Human Services, Public Health Service. https://www.atsdr.cdc.gov/toxprofiles/tp.asp?id=276&tid=50
Betoulle S, Etienne JC, Vernet G (2002) Acute immunotoxicity of gallium to carp (Cyprinuscarpio L.) Bull Environ Contam Toxicol 68:817–823
Blaurock-Busch E, Busch YM, Friedle A, Buerner H, Parkash C, Kaur A (2014) Comparing the metal concentration in the hair of cancer patients and healthy people living in the Malwa region of Punjab, India. Clin Med Insights Oncol Open Access 8:1–13
Bukhari IH, Mehmood MH, Naqvi SAR, Sarwar MJ, Latif Z (2012) Studies of some heavy metals profile in hair, nail and plasma samples of normal and allergy patients of Sargodha Zone. J Chem Soc Pak 34(4):881–884
Chen D, Frezza M, Shakya R, Cui QC, Milacic V, Verani CN, Dou QP (2007) Inhibition of the proteasome activity by gallium (III) complexes contributes to their antiprostate tumor effects. Cancer Res 67:9258–9265
Chojnacka K, Górecka H, Górecki H (2006) The effect of age, sex, smoking habit and hair color on the composition of hair. Environ Toxicol Pharm 22:52–57
Cihan YB, Sözen S, Yıldırım SÖ (2011) Trace elements and heavy metals in hair of stage iii breast cancer patients. Biol Trace Elem Res 144:360–379
da Silva Carneiro C, Mársico ET, de Jesus EFO, de Oliveira Resende Ribeiro R, de Faria Barbosa R (2011) Trace elements in fish and oysters from Sepetiba Bay (Rio de Janeiro-Brazil) determined by total reflection x-ray fluorescence using synchrotron radiation. Chem Ecol 27:1–8
de Miguel E, Llamas JF, Chacon E, Berg T, Larssen S, Royset O, Vadset M (1997) Origin and patterns of distribution of trace elements in street dust: unleaded petrol and urban lead. Atmos Environ 31(17):2733–2740
Eqani SAMAS, Kanwal A, Ali SM, Sohail M, Bhowmik AK, Ambreen A, Ali N, Fasola M, Shen H (2016) Spatial distribution of dust–bound trace metals from Pakistan and its implications for human exposure. Environ Pollut 213:213–222
Faiz Y, Tufail M, Tayyeb Javed M, Chaudhry MM, Siddique N (2009) Road dust pollution of Cd, Cu, Ni, Pb and Zn along Islamabad Expressway, Pakistan. Microchem J 92:186–192
Fergusson JE, Forbes EA, Schroeder RJ, Ryan DE (1986) The elemental composition and sources of house dust and street dust. Sci Total Environ 50:217–221
Ferreira-Baptista L, De Miguel E (2005) Geochemistry and risk assessment of street dust in Luanda, Angola: a tropical urban environment. Atmos Environ 39:4501–4512
Guney M, Zagury GJ (2013) Contamination of ten harmful elements in toys and children’s jewelry bought on the North American market. Environ Sci Technol 47:5921–5930
Hu X, Zhang Y, Luo J, Wang T, Lian H, Ding Z (2011) Bioaccessibility and health risk of arsenic, mercury and other metals in urban street dusts from a mega-city, Nanjing, China. Environ Pollut 159(5):1215–1221
Huang M, Chen X, Shao D, Zhao Y, Wang W, Wong MH (2014) Risk assessment of arsenic and other metals via atmospheric particles, and effects of atmospheric exposure and other demographic factors on their accumulations in human scalp hair in urban area of Guangzhou, China. Ecotoxicol Environ Saf 102:84–92
IMS Research Ltd. (2012) IMS Research expects 2011 packaged GaN LED revenues to decline 6% to $8 billion on weakness in backlighting—LED growth expected from 2012–2015 as lighting accelerates. IMS Research Ltd. Press, Wellingborough
Karim Z, Qureshi BA (2013) Health risk assessment of heavy metals in urban soil of Karachi, Pakistan. Hum Ecol Risk Assess 20:658–667
Khalique A, Shah MH, Jaffar M, Shaheen N, Manzoor S, Tariq SR (2005) Status of selected heavy metal distribution in scalp hair of traffic control personnel exposed to vehicular emissions. Hum Ecol Risk Assess 11:1065–1075
Khalique A, Shah MH, Jaffar M, Shaheen N, Tariq SR, Manzoor S (2006) Scalp hair metal analysis in the assessment of the occupational exposure of arc welders. Toxicol Environ Chem 88(4):697–704
Klevay LM, Christopherson DM, Shuler TR (2004) Hair as a biopsy material: trace element data on one man over two decades. Eur J Clin Nutr 58:1359–1364
Korfali SI, Sabra R, Jurdi M, Taleb RI (2013) Assessment of toxic metals and phthalates in children’s toys and clays. Arch Environ Contam Toxicol 65:368–381
Kousoulidou M, Ntziachristos L, Mellios G, Samaras Z (2008) Road-transport emission projections to 2020 in European urban environments. Atmos Environ 42:7465–7475
Krachler M, Burow M, Emaons H (1999) Biomonitoring of antimony in environmental matrices from terrestrial and limnic ecosystems. J Environ Monit 1(5):477–481
Lu CI, Huang CC, Chang YC, Tsai YT, Kuo HC, Chuang YH (2007) Short-term thallium intoxication: dermatological findings correlated with thallium concentration. Arch Dermatol 143(1):93–98
Luo R, Zhuo X, Ma. D (2014) Determination of 33 elements in scalp hair samples from inhabitants of a mountain village of Tonglu city, China. Ecotoxicol Environ Saf 104:215–219
Manno E, Varrica D, Dongarrà G (2006) Metal distribution in road dust samples collected in an urban area close to a petrochemical plant at Gela, Sicily. Atmos Environ 40:5929–5941
McLean CM, Koller CE, Rodger JC, MacFarlane GR (2009) Mammalian hair as an accumulative bio indicator of metal bioavailability in Australian terrestrial environments. Sci Total Environ 407:3588–3596
Meza-Figueroa D, De la O-Villanueva M, De la Parra ML (2007) Heavy metal distribution in dust from elementary schools in Hermosillo, Sonora, México. Atmos Environ 41:276–288
Miekeley N, Carneiro MTWD, da Silveira CLP (1998) How reliable are human hair reference intervals for trace elements? Sci Total Environ 218:9–17
Mikulewicz M, Chojnacka K, Gedrange T, Górecki H (2013) Reference values of elements in human hair: a systematic review. Environ Toxicol Pharmacol 36:1077–1086
Mohmand J, Eqani SAMAS, Fasola M, Alamdar A, Mustafad I, Ali N, Liu L, Peng S, Shen H (2015) Human exposure to toxic metals via contaminated dust: bio-accumulation trends and their potential risk estimation. Chemosphere 132:142–151
Moreno T, Karanasiou A, Amato F, Lucarelli F, Navac S, Calzolai G, Chiari M, Coz E, Artíñano B, Lumbreras J, Borge R, Boldo E, Linares C, Alastuey A, Querol X, Gibbons W (2013) Daily and hourly sourcing of metallic and mineral dust in urban air contaminated by traffic and coal-burning emissions. Atmos Environ 68:33–44
Nava S, Becagli S, Calzolai G, Chiari M, Lucarelli F, Prati P, Traversi R, Udisti R, Valli G, Vecchi R (2012) Saharan dust impact in central Italy: an overview on three years elemental data Records. Atmos Environ 60:444–452
Noguchi T, Itai T, Kawaguchi M, Takahashi S, Tanabe S (2012) Applicability of human hair as a bioindicator for trace elements exposure. In: Kawaguchi M, Misaki K, Sato H, Yokokawa T, Itai T, Nguyen TM, Ono J, Tanabe S (eds) Interdisciplinary studies on environmental chemistry—environmental pollution and ecotoxicology. pp. 73–77. https://www.terrapub.co.jp/onlineproceedings/ec/06/pdf/PR610.pdf
Nowak B, Chmielnicka J (2002) Relationship of lead and cadmium to essential elements in hair, teeth, and nails of environmentally exposed people. Ecotoxicol Environ Saf 46(3):265–274
Okorie A, Entwistle J, Dean JR (2012) Estimation of daily intake of potentially toxic elements from urban street dust and the role of oral bioaccessibility testing. Chemosphere 86:460–467
Oliveira KCC, Faturi C, Garcia AR, Nahúm BS, LourençoJúnior JB, Joele MRSP (2010) Supplemental feeding for buffaloes with agroindustry by-products on silvopastoral system in Brazilian eastern Amazon. Rev Vet 21(Suppl. 1):802–804
Pandolfi M, Gonzalez-Castanedo Y, Alastuey A, de la Rosa JD, Mantilla E, Sánchez de la Campa A, Querol X, Pey J, Amato F, Moreno T (2011) Source apportionment of PM(10) and PM(2.5) at multiple sites in the strait of Gibraltar by PMF: impact of shipping emissions. Environ Sci Pollut Res Int 18(2):260–269
Pelletier JD (2006) Sensitivity of playa windblown-dust emissions to climatic and anthropogenic change. J Arid Environ 66:62–75
Perry KD (1999) Effects of outdoor pyrotechnic displays on the regional air quality of Western Washington State. J Air Waste Manag Assoc 49(2):146–155
Quiroz W, Cortés M, Astudillo F, Bravo M, Cereceda F, Vidal V, Lobos MG (2013) Antimony speciation in road dust and urban particulate matter in Valparaiso, Chile: Analytical and environmental considerations. Microchem J 110:266–272
Rasmussen PE, Subramanian KS, Jessiman BJ (2001) A multi-element profile of housedust in relation to exterior dust and soils in the city of Ottawa, Canada. Sci Total Environ 267:125–140
Riyaz R, Pandalai SL, Schwartz M, Kazzi ZN (2013) A fatal case of thallium toxicity: challenges in management. J Med Toxicol 9(1):75–78
Rodrıguez S, Xavier Q, Alastuey A, Viana M, Alarco’n M, Mantilla E, Ruiz CR (2004) Comparative PM10–PM25 source contribution study at rural, urban and industrial sites PM episodes in Eastern Spain. Sci Total Environ 328:95–113
Rodushkin I, Engström E, Sörlin D, Baxter D, Hörnfeldt B, Nyholm E, Ecke F (2011) Uptake and accumulation of anthropogenic Os in free-living bank voles (Myodes glareolus). Water Air Soil Pollut 218:603–610
Ross G, Cooper, Harrison AP (2009) The exposure to and health effects of antimony. Indian J Occup Environ Med 13(1):3–10
Rudnick RL, Gao S (2003) Composition of the continental crust. Treatise Geochem 3-9:1–64
Rusyniak DE, Furbee RB, Kirk MA (2002) Thallium and arsenic poisoning in a small mid western town. Ann Emerg Med 39(3):307–311
Saary MJ, House RA (2002) Preventable exposure to trimethyl tin chloride: a case report. Occup Med 52(4):227–230
Sanchez de la Campa AM, Moreno T, De la Rosa J, Alastuey A, Querol X (2011) Size distribution and chemical composition of metalliferous stack emissions in the San Roque petroleum refinery complex, southern Spain. J Hazard Mater 190:713–722
Savabieasfahani M, Hoseiny M, Goodarzi S (2012) Toxic and essential trace metals in first baby haircuts and mother hair from imam hossein hospital Tehran, Iran. Bull Environ Contam Toxicol 88:140–144
Senesi GS, Baldassarre G, Senesi N (1999) Trace element inputs into soils by anthropogenic activities and implications for human health. Chemosphere 39(2):343–377
Senofonte O, Violante N, Caroli S (2000) Assessment of reference values for elements in human hair of urban schoolboys. J Trace Elem Med Biol 14(1):6–13
Sternbeck J, Sjodin A, Andreasson K (2002) Metal emissions from road traffic and the influence of resuspension—results from two tunnel studies. Atmos Environ 36:4735–4744
Tamburo E, Varrica D, Dongarrà G, Grimaldi LME (2015) Trace elements in scalp hair samples from patients with relapsing-remitting multiple sclerosis. PLoS ONE 10:e0122142
Turner A, Furniss O (2012) An evaluation of the toxicity and bioaccumulation of thallium in the coastal marine environment using the macroalga, Ulva lactuca. Mar Pollut Bull 64(12):2720–2724
Valotto G, Rampazzo G, Visin F, Gonella F, Cattaruzza E, Glisenti A, Formenton G, Tieppo P (2015) Environmental and traffic-related parameters affecting road dust composition: a multi-technique approach applied to Venice area (Italy). Atmos Environ 122:596–608
Wang C, Chen Y, Liu J, Wang J, Li X, Zhang Y (2013) Health risks of thallium in contaminated arable soils and food crops irrigated with wastewater from a sulfuric acid plant in western Guangdong province. China Ecotoxicol Environ Saf 90:76–81
Yoshinaga J, Yamasaki K, Yonemura A, Ishibashi Y, Kaido T, Mizuno K, Takagi M, Tanaka A (2014) Lead and other elements in house dust of Japanese residences—source of lead and health risks due to metal exposure. Environ Pollut 189:223–228
Zheng J, Chen K, Yan X, Chen S, Hu G, Peng X, Yuan J, Mai B, Yang Z (2013) Heavy metals in food, house dust, and water from an e-waste recycling area in South China and the potential risk to human health. Ecotoxicol Environ Saf 96:205–212
Acknowledgments
The authors wish to thank the NSFC, China (The Research Fellowship for International Young Scientists: NSFC# 21450110419) and President’s International Fellowship for Postdoctoral Researchers (Grant No. 2016PB009) for the financial support.
Author information
Authors and Affiliations
Corresponding authors
Additional information
Responsible editor: Philippe Garrigues
Highlights
• First data of rarely studied trace elements from Pakistan
• Human hair was used to evaluate human exposure to the studied elements
• Ti, Sr, Ba, V, Ga, Sn, Tl, and Sb levels were assessed in paired dust and human hair
• Sb, Ga, and Sn from mountain zones and Sr from Indus plain areas showed higher BAF values
• HI for Ti and Ga reflected non-carcinogenic human health risk
Electronic supplementary material
ESM 1
(DOC 203 kb).
Rights and permissions
About this article
Cite this article
Eqani, S.A.M.A.S., Tanveer, Z.I., Qiaoqiao, C. et al. Occurrence of selected elements (Ti, Sr, Ba, V, Ga, Sn, Tl, and Sb) in deposited dust and human hair samples: implications for human health in Pakistan. Environ Sci Pollut Res 25, 12234–12245 (2018). https://doi.org/10.1007/s11356-017-0346-y
Received:
Accepted:
Published:
Issue Date:
DOI: https://doi.org/10.1007/s11356-017-0346-y