Abstract
In this study, the determination of heavy metal concentrations (Fe, Mn, Ni, Cd, Cr, Cu, Co, Zn) in dust samples collected from different socioeconomic households in the urban area of Dhanbad city was investigated. Inductively Coupled Plasma-Optical Emission Spectroscopy (ICP-OES) was utilized to detect the heavy metal content of the dust samples after digestion and filtration. The mean concentrations of Fe found 19,983.25 mg kg−1 followed by Mn (313.03 mg kg−1), Zn (296.05 mg kg−1), Cu (62.16 mg kg−1), Cr (37.87 mg kg−1), Ni (33.67 mg kg−1), Co (11.66 mg kg−1), and Cd (5.65 mg kg−1). Contamination Factor (CF) for elements Fe, Mn, Ni, and Co obtained values less than 1 signifying low contamination, CF values for element Cu ranged between 1 to 3 signifying moderate contamination whereas Zn comes under considerable contamination. CF values for elements Cd signify high contamination in all types of households. Cd was found to be highly enriched with a mean value of EF greater than 20 followed by Zn, Cu, Cr, Ni, Co, and Mn. The hazard index (HI) of selected heavy metals was less than 1 and the carcinogenic risk (CR) values for Cd, Cr, and Ni were found below safe levels (1E-06 to 1E-04) for both adults as well as children. The HI value for children was around five times that of adults, suggesting that children are at a greater risk of exposure to heavy metals in indoor dust from all socioeconomic type households.
Similar content being viewed by others
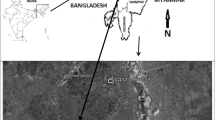
Explore related subjects
Discover the latest articles, news and stories from top researchers in related subjects.Avoid common mistakes on your manuscript.
Introduction
Household dust is a primary environmental carrier that requires considerable attention due to the increasing amount of toxicants to which humans are regularly exposed (Pal et al. 2021). Settled dust has the potential to act as a worldwide indication of residential pollution (Kumar and Jain 2022; Li et al. 2019; Neupane et al. 2020). Humans spend the majority of their time indoors which results in a link between household dust and their health (Cheng et al. 2018; Sahu et al. 2018; Shi and Wang 2021; Yaparla et al. 2019). Indoor dust is made up of suspended particles that can act as a carrier for a wide range of organic and inorganic substances, which include biological components, different heavy metals, polychlorinated biphenyls, and harmful polycyclic aromatic hydrocarbons (PAHs), all of which are absorbable by humans (Arar et al. 2019; Melymuk et al. 2020; Živančev et al. 2022). Various studies show that household dust from various places has a wide range of sources, compositions, and particle size distributions (Bhardwaj et al. 2017; Gustafsson et al. 2018; Zhao et al. 2021). As indoor dust has a diverse mixture of natural and anthropogenic origins, the common components of that have include soil, settled particulate matter, human and animal allergens, insect pieces, domestic effluents, and other debris of different forms, density, and porosity (Bora et al. 2021; Deng et al. 2021; Wan et al. 2019). Pollutant concentrations in household dust are likely to be unevenly distributed, especially if particulates and/or pollutants came from multiple sources (eg, indoor activities, air intrusion, or track-ins) (Cao et al. 2014).
To enable thorough monitoring of our everyday environment, reliable measuring methods for determining the basic composition of household dust are necessary. The sources of heavy metals in household dust are known to be diverse and depend on various factors like household conditions and their location, indoor activities as well as outdoor sources (Boisa and Odagwe 2019; Cheng et al. 2018; Liu et al. 2021; Tao et al. 2021; Underhill et al. 2015). The concentration of heavy metals in household dust is greatly influenced by the surrounding environment. Heavy metals may be transferred through ventilation and by occupants moving from outside to inside a residence, which contributes to the accumulation of heavy metals in the dusty environment transported in from outside (Cao et al. 2022; Saraga et al. 2017; Zhao et al. 2021). The re-suspended particles have adhered to the surfaces of food, skin, toys, and furniture, where humans, particularly children, consume them (Han et al. 2020; Huang et al. 2014).
Moreover, heavy metals in household dust have been linked to different day-to-day activities (smoking, cleaning frequency, the everyday burning of fuel for domestic cooking, and the frequency of cooking) and the use of air conditioning, various paints, and rubber carpet products (Cheng et al. 2018; Shi and Wang 2021; Yaparla et al. 2019; Zhao et al. 2021). Heavy metals have numerous negative health impacts, including reduced bone density, renal dysfunction, nervous system injury, and disruption of typical brain development (Chen et al. 2019; Men et al. 2018; Zhao et al. 2021). Heavy metals tend to accumulate in tissue, resulting in biological magnification over time. The trace elements such as Co, Ni, Mn, Fe, Cu, and Cd have been linked to a variety of acute and chronic health effects such as asthma, cognitive impairment, irritability, weariness, dermatitis, impaired vision, throat or nose irritation, lung cancer in humans, among other environmental effects (Bahloul 2020; Hou et al. 2019; Men et al. 2018; Rahman et al. 2021a).
The results demonstrated that dust with particle sizes of < 63 um may be easily re-suspended into the environment and has a higher potential to absorb by people via ingestion, inhalation, and dermal adsorption than dust with other particle sizes (El-Zahhar et al. 2021; Rahman et al. 2021b; Sahu et al. 2016). Additionally, fine particles of dust have a very large surface area and were shown to contain greater quantities of heavy metals (Cheng et al. 2018; Hassan et al. 2015). As a result, the focus of heavy metals in household dust studies is on fine particles of dust. Various studies show that outdoor aerosols have a substantial contribution to indoor pollution, with street dust accounting for 20–95 percent of residential dust (Rasmussen et al. 2018; Torres-Sánchez et al. 2017). Moreover, the number of storeys and building height, the frequency and timing of window openings, pets in the home, and the number of residents and their activities (cooking, sweeping), also influence the concentrations of heavy metals in household dust (Gul et al. 2022; Kitagawa et al. 2022; Shi and Wang 2021). Indoor activities and penetration of outside aerosols indoors are the principal sources of heavy metals in the indoor environment. Indoor activities such as cooking, cleaning, smoking, and dust particle resuspension all contribute to household dust pollution (Ali 2019). Heavy metals can enter the indoor environment by suspended particles in the outdoor air (Rasmussen et al. 2018), high vehicular traffic outside (Pipal et al. 2021; Rohra et al. 2018), and dirt that sticks to footwear (Ali 2019; Cheng et al. 2018; Kelepertzis et al. 2019).
The possible health consequences of household dust’s heavy metals exposure by oral ingestion, inhalation, and dermal contact have dependent on the size of the dust particles, the rate of deposition, and the chemical constitution of the dust (Blanchard et al. 2014; Li et al. 2019; Zhao et al. 2015). Recently, health risk assessment has been utilized for diverse environmental media such as playground dirt, school dust, road dust, and indoor dust to measure the toxicity of pollutants via multiple exposure routes such as ingestion, inhalation, and dermal contact (Agarwal et al. 2019; Hou et al. 2019; Rahman et al. 2021a). Children, in particular, are more impacted by dust contamination because of their hand-to-mouth activity (Bahloul 2020; Huang et al. 2014; Moghtaderi et al. 2020). It also interferes with the child's brain development, which is at its peak throughout their growing phase and makes them more vulnerable to heavy metals (Muhamad-Darus et al. 2017; Parhizkar et al. 2021). Heavy metals, in particular, nickel, cadmium, and chromium are not biodegradable and can have a severe effect on public health varying from allergic responses and intoxication to lethal cancer disorders (Cao et al. 2022; Mostafaii et al. 2021; Safiur Rahman et al. 2019; Shi and Wang 2021; Ying et al. 2016).
Many studies in various cities across the globe have examined the health risks of exposure to heavy metals in indoor dust such as in Chengdu (Cheng et al. 2018), and Guangzhou (Huang et al. 2014) in China, Telangana (Yaparla et al. 2019), and Asansol (Pal et al. 2021) in India, Dhaka city, Bangladesh (Rahman et al. 2021b), Istanbul, Turkey (Gul et al. 2022), Malesia (Latif et al. 2014), Athens, Greece (Kelepertzis et al. 2019), Huelva, Spain (Torres-Sánchez et al. 2017), and Iran (Moghtaderi et al. 2020). However, there have been limited studies that provide extensive information and a database on the heavy metals in various household dust and on the health concerns of the resident's actual situation (socio-economical status, household activities, exposure frequency, and body weight). Therefore, this study aimed to investigate the heavy metal contamination in the different household dust samples located in Dhanbad city. The primary objectives of this study were i) Determination of heavy metal concentrations (Mn, Ni, Cu, Co, Zn, Cd, Fe, and Cr) in different households, ii) To assess the health risk of household dust in different socioeconomic residences.
Material and methods
Description of the study area
Dhanbad (23.7957°N and 86.4304°E) is popularly known as the coal capital of India, located in the Jharkhand state of eastern India. Dhanbad has a significant population of 1.16 M due to the vast resources of energy from having the Jharia coalfield range. Various households in the most populated regions of Dhanbad with different socioeconomic statuses were selected for this study. Passive sampling for heavy metal contamination was performed inside the houses of different socioeconomic statuses in Dhanbad city. Figure 1 shows the sampling locations in the study area.
Household classification and questionnaire survey
Man's socioeconomic standing influences his manner of life, the type of house he lives in, the location of his dwelling, and his access to different services and resources. According to income earned (Chandramouli and General 2011), low, middle, and high–income group dwellings in Dhanbad city were chosen for dust sampling. A questionnaire survey was carried out in order to classify and quantify the households based on their socioeconomic position. The questionnaire questions were prepared following a peer evaluation of the literature and their suitability in the area of study (Bird 2009; Kumar and Samadder 2017). In our questionnaire, we employed the modified BG Prasad scale and the Kuppuswamy scale to assess socioeconomic status (SES) (Bird 2009; Khan et al. 2016). The survey questions pertained to a general overview of basic attributes (family income and educational qualification), the size of the family, housing structures, floor level, kitchen structure/conditions, cooking fuel used, indoor conditions (floors, walls, ventilation, air conditioning, etc.), and residing time in various locations within the house (Kesavachandran et al. 2015; Prabhu et al. 2019). The information was gathered at the house through a direct interview and question-and-answer session. We gave them our blank questionnaire (SI-1) and requested them to fill it out and return it to us. Some basic questions were like "How many family members are there in the house?", "How much time do they spend in the house?", “What type of fuel do you usually use for cooking?”, "How old your house is?". Finally, we chose those houses for the observation that provided suitable responses in the form of 'Yes,' and they were subjected to our examination.
34 households were chosen for the dust sampling from the study region. 4 of these did not support or authorize sampling. So, after estimating the monthly income earned by each household member, we ended up with 30 houses in our count. The households were denoted as H1 to H30 for households 1 to 30. 10 (H1-H10) of the 30 households are from the Low-Income Groups (LIG: monthly earning INR < 5000) and are largely in slum areas with 1 or 2-room houses/hut-type structures. 10 (H21-H30) were High Income Groups (HIG: monthly earnings INR > 60,000), while (H11-H20) the rest were Mid Income Groups (MIG: monthly earnings INR < 60,000), primarily detached residences, often 1 or 2-storey structures (Singh et al. 2014). In LIG and MIG dwellings, slum areas, and detached houses, there was no exterior ventilation/exhaust system. Natural ventilation has been provided via windows and doors in those houses. HIG was equipped with air-conditioning system. In LIG houses, coal was utilized in a homemade cook stove for cooking purposes. In the rest groups, LPG cylinders and a few electric cookstoves were utilized for cooking. There was no sufficient ventilation/windows in the numerous LIG households, and there were also no separate kitchens within the home.
Analytical methods
Sampling was conducted between February and March 2022 at selected households with a temperature range of 16–35 °C and an average relative humidity of 45% (Table SI-1). Less windy and dry days without precipitation were chosen as sampling days for less indoor-outdoor interaction during the sampling procedure. The household dust samples were collected using clean plastic brushes from the windowsill, ground surface, slabs, etc., in the dry vicinity of everyday activities. Three sub-samples according to the equal quantity principle in one household on the same day were collected. Three sampling locations, at least one meter apart, were chosen from each site for sample collection (Ugwu and Ofomatah 2021). All samples collected from each sampling point in each household were mixed together to get a single composite sample for one house. Each household dust sample was stored in zipper bags after being wrapped with aluminium foil and then transported to the laboratory. The dust samples were passed through a 100 μm sieve of nylon mess (IS:460, Universal soil equipers, New Delhi, India) to remove excess materials such as paving stones, unwanted rubber, stumps, mortars, bricks, and other extraneous particle (Cheng et al. 2018; El-Zahhar et al. 2021; Kumar and Jain 2022). These dust samples were kept at 105 °C in the hot air oven, dried to a consistent weight, and finally sieved through a nylon mesh of 63 μm size for chemical analysis. Dried materials were weighed (1 g) and mixed with 20 ml of mixing solution (HNO3-HCl) in a 3:1 ratio before being digested. The samples were placed at room temperature for 60 min and then heated on a hot plate at 105 °C for 2 h to complete digestion and obtain a clear liquid (Naimabadi et al. 2022). Inductively Coupled Plasma-Optical Emission Spectroscopy (Make: Thermo Fisher Scientific, USA, Model: iCAP 7400 Duo) was used to determine the metal content of the extracts after digestion and filtration (42 Whatman filter paper). The extracts had also been diluted to 50 ml with Mili-Qwater in the volumetric flask before the determination of the heavy metal concentration (Co, Cr, Cd, Cu, Fe, Mn, Ni, and Zn). The blank experiment was conducted by repeating the sample preparation procedure without the sample. The elemental composition of heavy metals in the household dust was determined by comparing the composition of the blank and sample solutions. The detailed Quality Control (QA/QC) procedure has been explained in Supplementary Information (SI-2).
Pollution assessment
The heavy metal concentrations have been measured using pollution various indicators/indices in different household dust of Dhanbad city. The utilized pollution indices are given below.
Enrichment factor (EF)
The enrichment factor (EF) was used to determine the severity of metal contamination as well as potential natural and anthropogenic sources. The following equation is used to define EF mathematically (Liu et al. 2021; Rout et al. 2015):
where [Cx/Cref]Sample denotes the concentration ratio of a measured metal and the reference metal in the indoor dust samples and [Bx/Bref]Background denotes the concentration ratio of the same for background material (Taylor and Mclennan 1995). Fe was selected as the study's reference metal because it is a prominent part of clay minerals and has been utilized effectively by various studies. EF can also help in distinguishing between an anthropogenic and a natural source. The EF was divided into five groups (Li et al. 2018; Victoria et al. 2014), which are detailed in the supplemental material (Table SI-2).
Contamination factor (CF)
To evaluate metal pollution in settling dust, Hakanson (1980) developed the contamination factor (CF) index (Hakanson 1980). Many scientists have utilized such indices to assess heavy metal contamination in dust samples (Gope et al. 2018; Pobi et al. 2020; Tomlinson et al. 1980). The contamination factor (Cf) has been expressed as the following equations.
where Cfi denotes the contamination factor, Cm sample denotes the metal concentration in the dust, Cref denotes the metal concentration in the reference or background sample, and n denotes the number of metals analyzed in the study (Taylor and Mclennan 1995). The metal enhancement in the samples is reflected in the Cfi (Table SI-3).
Geo-accumulation index (Igeo)
Mueller (1979) developed the Igeo, which has been used to assess the degree of heavy metal contamination in the dust (Mueller 1979). The Igeo was calculated using the following equation:
where Cm Sample denotes the metal concentration in the dust samples, Cref denotes the metal concentration in the reference or background sample, and 1.5 denotes the background matrix correction factor owing to lithogenic influences. Table SI-4 summarizes Mueller (1979) suggested Igeo categorization.
Pollution load index (PLI)
The following equation, developed by Tomlinson et al. (1980) was used to calculate the pollution load index (PLI) for the dust samples (Tomlinson et al. 1980).
where n and CF are the number of metals in the sample and the contamination factor of each metal respectively. Table SI-5 has more information about the categorization.
Exposure and toxicity assessment
The US Environmental Protection Agency's (EPA) model has been used in several studies to estimate residents' exposure to potentially harmful heavy metals found in indoor dust (Rahman et al. 2021a; Shi and Wang 2021; Zhou et al. 2019). Residents (adults and children) may be exposed through three distinct routes, including ingestion, inhalation, and dermal contact.
Exposure dose
The daily dose intake of each metal computed for each exposure pathway is given below.
Ingestion of dust particles (ADDing) via the mouth
Inhalation of dust particles via mouth or nose (ADDinh)
Dermal contact/adsorption (ADDderm) via skin
where ADD represents the daily dose (mg kg−1 day−1) for each metal and exposure route (ing: ingestion, inh: inhalation, derm: dermal), C is the concentration of heavy elements in household dust (mg kg−1), IngR is Ingestion Rate (mg/d), EF is Exposure Frequency (d/yr), ED is Exposure Duration (yr), BW is Body weight (kg), AT is Averaging time (d), InhR is Inhalation Rate (m3/d), PEF is Particle emission factor (m3 kg−1), SA is Surface Area Exposed (cm2), SL is Skin adherence factor (mg cm−2 day−1), and ABS is Dermal Absorption Fraction (unitless).
Non-carcinogenic health risk assessment
To quantify non-carcinogenic health risks from toxic heavy metals in dust particles, the hazard quotient (HQ) and hazard index (HI) are used. The following equation can be used to calculate HQ.
where ADD represents the daily dose (mg kg−1 day−1) for each metal and exposure route and Ref represents the reference dosage (mg kg−1 day−1) for particular heavy metals. HI is the total of the HQ exposure pathways of inhalation, ingestion, and dermal absorption (HI = HQinh + HQing + HQdermal).
If HI < 1, there should be no non-carcinogenic risk. If HI > 1, it is assumed that there would be a danger of non-carcinogenic consequences, with the chance increasing as HI rises.
Carcinogenic health risk assessment
Three elements (Cr, Cd, and Ni) were classified as carcinogenic. Cancer risks (CRs) have been calculated as the likelihood of a single individual developing cancer as a result of overall exposure to the possible carcinogen over a lifetime. The following equation can be used to determine the cancer risk (CR)
where ADDinh is a daily dose (mg kg−1 day−1) for inhalation exposure and SF is slope factor (mg kg−1 day−1)−1. There is most likely a significant risk of serious carcinogenic consequences if CR > 1 × 10–4, whereas a range of 1 × 10−6 to 1 × 10−4 can be considered as acceptable/tolerable risk (USEPA 2001). Health risk parameters for each exposure route have been mentioned in Table SI-6.
Statistical analysis
The software programs SPSS 20.0 and Excel 2013 were used to run statistical analyses on the data. Pearson's correlation coefficient analysis was employed to investigate the relationships between household environmental characteristics and heavy metal concentrations. The link between 8 metals in home dust and their likely origins was discovered using principal component analysis (PCA). PCA has been routinely employed in dust-containing heavy metals in recent years (Pal et al. 2021; Siddiqui et al. 2020; Zhou et al. 2020). To make the findings more interpretable, PCA was performed using the varimax rotation of Kaiser normalization. The PCA loadings were displayed to examine the source similarities in this study.
Result and discussion
Heavy metal analysis
Table 1 shows the mean heavy metal contamination (Mn, Ni, Cu, Co, Zn, Cd, Fe, and Cr) in different samples collected from the sampled houses (in mg kg−1). Variation of metal concentration at LIG households was Fe > Mn > Zn > Cu > Cr > Ni > Co > Cd, at MIG households was Fe > Zn > Mn > Cu > Cr > Ni > Co > Cd whereas HIG households were Fe > Zn > Mn > Cu > Cr > Ni > Co > Cd (Table SI-7). Overall, the trend of concentration in different households was found as Fe > Mn > Zn > Cu > Cr > Ni > Co > Cd. Fe was found to have the highest concentration with an average value of 19,983.25 ± 6155.31 mg kg−1 followed by Mn (313.03 ± 89.04 mg kg−1), Zn (296.05 ± 125.69 mg kg−1), Cu (62.16 ± 25.19 mg kg−1), Cr (37.87 ± 11.07 mg kg−1), Ni (33.67 ± 13.35 mg kg−1), Co (11.66 ± 5.12 mg kg−1), and Cd (5.65 ± 8.74 mg kg−1). There were substantial differences in Fe concentrations in various households between the study locations (p < 0.05). Fe concentrations across the samples ranged from 8837.43 mg kg−1(H17) to 35,116.14 mg kg−1(H19). It was discovered that the dust samples collected in this study had a lower average Fe content than the dust samples from Asansol, India (Pal et al. 2021), Dhaka, Bangladesh (Rahman et al. 2021a), Doha, Qatar (Mahfouz et al. 2019) whereas higher than Chennai, India (Agarwal et al. 2019), Malaysia (Latif et al. 2014), and Athens, Greece (Kelepertzis et al. 2019). Simultaneously, changes in Fe concentrations in household dust recorded at different houses were computed to account for the influence of outside dust entering the inside environment through occupants' movement as well as the impact of wind blowing from the outside. It has been reported that the primary sources of Fe in the selected households are natural, which is consistent with some previous findings (Rahman et al. 2021a).
Mn concentrations across the samples ranged from 139.84 mg kg−1(H29) to 492.78 mg kg−1(H7). Major sources of Mn in household dust could be the use of household washing agents, and outdoor sources for automobile emissions (Iwegbue et al. 2018). Mn is the main raw material used in steel plants and is the main source of Mn contamination in an outdoor environment (Gope et al. 2018), and Mn-contaminated dust enters indoors from the outdoor environment. Mn is the primary raw material used in steel plants and the primary source of Mn pollution in an outdoor environment (Gope et al. 2018), and Mn-polluted dust enters the interior environment from the outdoors. Mn concentrations are mostly from natural soil sources that are carried or blown into homes, where they accumulate (Isley et al. 2022). This is corroborated by greater Mn concentrations in detached houses, which have a larger potential for track-in due to outside soil areas and shorter track-in lengths than apartments/multy storey buildings. Mn concentrations were greatest in metal-framed homes, most likely because Mn is an addition to steel (Kaar et al. 2018). Additionally, increased Mn concentrations in indoor dust samples were linked to the use of wood fuels for heating and indoor smoking. Manganese is also used in a broad range of consumer items, including fireworks, dry-cell batteries, fertilizer, paints, medical imaging agents, cosmetics, and so on (Isley et al. 2022; Kraepiel et al. 2015).
Zn concentrations ranged from 133.26 mg kg−1(H17) to 703.56 mg kg−1(H5), with considerable differences across locations (p < 0.05). H5, a home in the LIG households had the highest concentration of Zn, whereas H17, a residential complex belonging to HIG households had the lowest concentration. People are generally exposed to Zn through food however, oral intake might be excessive due to non-dietary causes. Unquestionable levels of Zn may disrupt the equilibrium of other essential components (Rahman et al. 2021b). Household dust can include Zn from the flooring of rubber/vinyl/leather, galvanized iron roofs, and carpets (Rahman et al. 2021a). Depreciation of vulcanized rubber tyres, breakdown of galvanized vehicle parts, and lubricating fluids all release Zn into the environment, which may be tracked back to the indoor environment via outdoor particulates (Hou et al. 2019; Muhamad-Darus et al. 2017). Furthermore, Zn contamination in household dust is caused by various domestic brass and bronze utensils, dry cell batteries, wall and furniture paints, rubber toys, and ceramic materials (Iwegbue et al. 2017; Pal et al. 2021; Pobi et al. 2020).
Average Cu concentrations were 61.08 ± 28.44, 72.80 ± 26.66, and 52.58 ± 13.33 mg kg−1 in LIG, MIG, and HIG households respectively (Table SI-7). The sources of Cu contamination in households are the use of wood preservatives and bactericides, which contain Cu (copper arsenate, copper sulfate, etc.) (Iwegbue et al. 2017). Outside vehicular emissions and the use of coal as a fuel indoors could enhance Cu concentration outdoor as well as in households of Dhanbad (Gope et al. 2018). The mean Cu concentration in different household dust samples was equivalent to the previous studies in the global range (Rahman et al. 2021a).
Cd levels ranged from 1.19 mg kg−1(H17) to 39.86 mg kg−1(H27), with significant differences between sample locations (p < 0.05). When compared to other households, a house (H10), which is located in a quiet neighborhood distant from main roadways, has an extremely low Cd level. In contrast, the values for the remaining stations were nearly equal to the highest concentration recorded at H9. Cr contents in different dust samples ranged from 16.61 mg kg−1(H17) to 71.49 mg kg−1(H5). The lowest concentrations of Cr, like Cd, were found in the H10 household. The high concentration of Cd in household dust is caused by the influence of outdoor dust which comes from a variety of activities such as fossil fuel combustion, engine oil, batteries, and tire wear (Matt et al. 2020; Pal et al. 2021). The main indoor source for the contamination of indoor dust with heavy metals was ornamental items. According to several studies Zn, Cu, and Cd may accumulate as a result of wall paint and coating materials (Bao et al. 2019; Cheng et al. 2018; Zhang et al. 2022). Additionally, the most important element for the contamination of indoor dust with heavy metals is the color of the wall paint. In contrast to purple and green paint, which have been documented to contain significant amounts of Zn and Cu, yellow paint is frequently linked to contamination with Zn, Cu, and Cd (Liu et al. 2021; Wang et al. 2023). Additionally, waste incineration, chemical engineering, electroplating, printing, and dyeing are the main sources of Cd and Cr emissions (He et al. 2017; Zhou et al. 2022).
Ni levels ranged from 7.61 mg kg−1(H17) to 66.88 mg kg−1(H5), with significant differences between sample locations (p < 0.05). Mean Ni concentrations (33.67 mg kg−1) in the current study were found to be higher than those reported in the Toronto study (23 mg kg−1) (Hejami et al. 2020), but lower than levels reported (43.2 mg kg−1) in dormitory buildings of Lanzhou City, China (Bao et al. 2019). Indoor Ni pollution is mostly caused by coal combustion, which is widely utilized as a home cooking fuel in Dhanbad (Gope et al. 2018; Li et al. 2014).
The concentrations of Co in dust from different households ranged from 3.44 mg kg−1(H17) to 21.61 mg kg−1(H7). Elevated levels of Co were observed in dust from a MIG household nearby roads (H14: 20.01 mg kg−1) and a LIG household (H3: 17.47 mg kg−1). Co is widely utilized in alloys, coatings, paints, and pigments. Because of their high gloss, flawless color, and aesthetic effect, Co containing construction materials are frequently employed in modern structures (Alotaibi et al. 2022). Cobalt is utilized in glazes as well as the production of pottery, ceramics, and even roof tiles. It is used to make a variety of alloys, including stainless steel. The average value of Co (11.66 mg kg−1) is significantly higher than that reported by Patel and Jain (2021) for the outdoor dust samples of Dhanbad (7.50 mg kg−1) (Patel and Jain 2021). The primary contributing factors of Co in indoor dust were building construction or remodelling, as well as weathering and corrosion of the building elements (Zhao et al. 2015).
Assessment of heavy metal pollution
Enrichment factor (EF)
As Fe is a significant component of surface soil, it can affect Fe levels in interior dust. Figure 2 shows the enrichment factor (EF) in various socio-economical households. Most of the heavy metals investigated in all types of households had an enrichment factor of more than 1.0, suggesting that they came from coal and other linked sources. In the data collected, Cd has the highest EF value, followed by Zn, Cu, Cr, Ni, Co, and Mn. According to these findings, Cd is more abundant in indoor dust than the other heavy elements investigated. Because there is no major industry near the sample sites, the Cd levels might be generated by the burning of oil and coal/LPG during cooking, incense and candle burning, lubricating fluids, and/or worn tyres outside roads (Hassan 2012; Kulshrestha et al. 2014; Liu et al. 2021). Outdoor road dust is likely to have impacted the level of Cd in the interior dust gathered from the selected households. Significant enrichment was found for the element Cd (EF > 20) in all types of households uncovering that these components in Dhanbad household dust are primarily derived from anthropogenic sources. Moreover, Cu signifying moderate enrichment as their mean values of EF were found between 2 to 5, which are reported to be primarily derived from natural sources, for example, outdoor dust, and soil mineral dust carried by the wind. Zn comes under the significant enrichment category which can be caused by various domestic brass and bronze utensils, leather, and carpets. The EF value showed the following diminishing pattern in LIG households: Cd > Zn > Cu > Cr > Co > Ni > Mn, whereas Cd > Zn > Cu > Cr > Ni > Mn > Co for MIG and HIG households.
Contamination factor (CF)
The contamination factor (CF), geo-accumulation index (Igeo), and pollution load index (PLI) were among the pollution measuring indicators utilized to analyze dust in the study region. Figure 3 displays the contamination factor (CF) calculated values. The results reveal that the elements Fe, Mn, Ni, Cr, and Co obtained values less than 1 signifying low contamination from anthropogenic sources in different households. CF values for element Cu ranged between 1 to 3 signifying moderate contamination in all types of households whereas Zn comes under considerable contamination. CF values for elements Cd signify high contamination in all types of households. A similar study conducted in the Kumasi metropolis of Ghana found that in dust samples, the CFs for heavy metals varied from low contamination (CF < 1) for Co to moderate contamination (1 < CF < 3) for Cr. However, indoor dust samples from the export processing zone (EPZ) region of Dhaka City (Bangladesh), showed considerable pollution (1 < CF < 3) with Zn and Cu (Rahman et al. 2021a). The LIG households carry a significant amount of coal inside the house for cooking. The fine coal ash from domestic stove combustion is typically dumped in residential neighborhoods. Depending on the ash content in the coal used, the fly ash has a 5–10 fold more enrichment in heavy metals (Rout et al. 2015). Higher dust pollution is a result of particulate matter emissions from domestic stoves burning coal and the resuspension of residual coal ash, in LIG and MIG households utilizing coal as cooking fuel.
Geo-accumulation index (Igeo)
Igeo has been widely employed in various heavy metal studies and it is a key method for determining the amounts of heavy metal pollution in dust samples from households. The average Igeo values for various metals in dust samples collected from various households are displayed in Fig. 4. Dust samples from LIG households' Igeo values showed that Cr, Zn, and Cu fell into the unpolluted to moderately polluted category, Cd into the moderately to highly contaminated category, and Fe, Mn, Ni, and Co into the unpolluted category. After that, Igeo values for samples of home dust from HIG households showed that Mn and Cu were in the unpolluted to the moderately polluted category, Cr and Zn were in the moderately polluted category, Cd was in the highly contaminated category, and Fe, Ni, and Co were in the unpolluted category. Similar results for Igeo were found in previous studies (Rahman et al. 2021a).
Pollution load index (PLI)
To compare whether the different types of households are contaminated or not, the pollution load index was calculated. The PLI aims to quantify the total level of contamination at a sample location. According to the findings, the eight metals are overall contaminated in the following order: LIG > HIG > MIG (Fig. 5). Regardless of the systematic contamination, the PLI value for all of the dust samples (n = 30) was between 0.5 and 1.9, suggesting that only baseline concentrations of pollutants are present. In a similar study in the Kumasi metropolis of Ghana, PLI values were found in the range of baseline levels of pollutants (Nkansah et al. 2015).
Probable source identification
Pearson correlation analysis
Pearson's correlation coefficient was utilized to evaluate the link between heavy metal concentrations in dust samples, and the findings are provided in Fig. 6. The Shapiro-Wilk normality test was used to determine whether the data were suitable for correlation analysis at first. Fe, Mn, Co, Ni, and Cr values were found to be greater than the threshold value (0.005), indicating that their data is normal, but Cu, Zn, and Cd values were found to be below the threshold, indicating that their data is not normal. Box-Cox was found to be the best normalizing transformation approach for Cu, Cd, and Zn data, while Arcsinh was found to be the best for Fe data after the investigation. The correlation coefficient was calculated using converted findings with normality distribution in the data. Figure 6 shows the relationship between heavy metals in various substrates. Zn with Cu (r = 0.835, p < 0.01) and Ni (r = 0.726) for example, showed a substantial significant association between the metals in various homes. This suggests that Zn and Cu may come from the same contamination sources. Some of the heavy metals in residential dust are strongly connected, for example, a very significant association was discovered in indoor dust between Ni and Zn (r = 0.726, p < 0.01). Cr and Zn also exhibit a positive correlation with other heavy metals (p < 0.01). The correlations were weaker in the case of Cd and Fe (r = 0.272), which suggests that the quantity of Fe in the households may not be connected to the sources of Cd in the household dust. The other metals, on the other hand, were not connected. According to Suresh et al. (2011), a positive correlation coefficient between heavy metals indicates their shared origin, whereas a negative correlation reveals a variety of variables, which might include a mixture of geochemical processes and/or dominating lithological sources, as well as their mixed connection (Suresh et al. 2011).
Principal component analysis
PCA is a multivariate approach that separates meaningful data from a dataset by processing numerous intercorrelated quantitative dependent variables (He et al. 2019; Liang et al. 2020). The data were examined to determine whether they were appropriate for the PCA using the Kaiser-Meyer-Olkin (KMO) and Bartlett's sphericity tests. By estimating the proportion of variation across variables that may have a common variance, the KMO test determines how much data is acceptable for factor analysis. The KMO test result (0.66) indicated that the data on trace metal contamination is suitable for analysis (Park 2021; Siddiqui et al. 2020). Similarly, in this study, Bartlett's test level was close to 0. Principal component analysis (PCA) was performed to establish possible factors that contribute towards the metal concentrations and source apportionment. All data set was subjected to factor analysis (FA). The number of significant principal components (PC) was selected based on Varimax orthogonal rotation with Kaiser normalisation with an eigenvalue greater than 1. The rotated component matrix is given in Table 2. Table 2 shows the % of overall variance as well as the heavy metals component matrix. The PCA was used to assess the associations between eight heavy metals in all household dust samples, and the results indicated that the three components (PC1, 38.8%; PC2, 22.1%; PC3, 16.3%) accounted for 77.2% of the total variance. Zn, Cu, Cr, and Ni were significantly weighted in Factor 1; Fe, Mn, and Co dominated in Factor 2; and Cd dominated in Factor 3 (Table 2). The statistical data and EF values can be used to determine the most likely emission sources. Factor 1's heavy metals profile corresponded to the emission characteristics of the Cu, Zn, and Ni composition from indoor cooking/smoking and indoor activities. This implies that the first group elements Cu, Ni, Co, Cr, and Zn share a common source; the coal burning for cooking, indoor facilities with their conditions, and their anthropogenic origins.
The second groups show the natural elements of outside soil and dust inside. The main sources of Fe include soils and resuspended dust, as well as vehicle exhaust (Rahman et al. 2021a; Rout et al. 2015). Crustal elements Mn and Co have also been found in the second group implying that it was a component of the combined source of atmospheric deposition and wind-borne dust, suggesting a mixed origin (natural/anthropogenic). For the third group, the presence of Cd in household dust is caused by a variety of factors, including the burning of oil and coal/LPG during cooking, and candle/incense burning which may also contribute to Cd deposition in indoor dust (Shi and Wang 2021). In this investigation, potential Cu sources included fuel and oil leaks. Cd and Cu may also accumulate as a result of wall paint (Iwegbue et al. 2017). Latex underlays, carpets, and galvanized steel roofs are all thought to be significant Zn sources in residential spaces (Marinho Reis et al. 2018). As a result, factor 1 appeared to be derived in part from indoor activities sources and in part from latex paint chemicals. Cd was shown to be an independent cluster with no connection to other metals in the PCA. Invertors and any other batteries may be the sources of Cd. Cd is utilized as a catalyst, binder, or coloring agent in interior plastic construction materials and plastic components (Turner 2019), so old plastic items in the home can be a potential source of Cd in household dust. Furthermore, older homes typically have worn and warped windows and doors, as well as fissures in the flooring, which can allow heavy metals to build in household dust (Rasmussen et al. 2018; Zeng et al. 2020). Particles rich in Cd, Fe, Mn, and Zn are produced by combustion sources like cooking, smoking, and candle burning, whereas Cd, Cu, Fe, and Ni are released into the home's microenvironment by sources like personal care products, cleaning agents, and soil resuspension from human activities (Shi and Wang 2021).
Health risk assessment
Assessment of cancer and non-cancer hazards might be beneficial in determining the health risks faced by residents at various households who may be exposed to harmful heavy metals. Cr, Ni, and Cd have been designated as probable human carcinogens by the International Agency for Research on Cancer (IARC) by the USEPA (USEPA 2001). The findings of a carcinogenic and non-carcinogenic health risk assessment of exposure to household dust are shown in Table SI-8 to Table SI-12. Figure 7 has shown that Fe had the greatest ADDing value of 8.21E-03 and 6.56E-02, while Cd had the lowest value of 2.32E-06 and 1.86E-05 for adults and children respectively. It was discovered that residents of low socioeconomic status (households nearby the road) had a greater chance of having non-cancer hazards. The presence of heavy metals in dust from roadside vehicular movement might be the cause. Socioeconomic status greatly influences ADD values. The quantification based on different socio-economical houses shows a variation in different metals-related ADD. The HIG houses were ADDing values (child) for Fe ranges from 4.15E-02 to 1.02E-01 whereas for the LIG houses it varies from 4.05E-02 to 8.49E-02 showing the variation of Fe there (Table SI-9). There is a little bit of difference in MIG and LIG houses ADDing values for Cd. The ADDing for Cu and Zn for adults as well as children shows the maximum for MIG among them showing the disproportionality between different houses.
Non-carcinogen risk
Different residents in Dhanbad City, India, were evaluated for their exposure to household dust to identify non-cancer causing hazards for exposure to both children and adults. The observed results declared that the non-cancer risk (HQs) of Ni, Co, and Zn for ingestion was significantly greater than for inhalation and dermal adsorption (Fig. 8). The findings of this research are consistent with prior studies reported in the literature (Pal et al. 2021; Rahman et al. 2021a). The greatest HQing value for children while taking into account the non-cancer risk of ingestion was determined to be 1.28E-01 for Co, and the lowest was discovered for Zn (3.24E-03), following in the order of Co > Fe > Mn > Cr > Cd > Ni > Cu > Zn. Descriptive characteristics of the overall hazard quotient (HQ) for adults and Children of each metal in various socio-economical households have been provided in the supplementary file as Table SI-12.
The hazard index (HI) value has been determined using the non-cancer risk (HQ) values for the defined three exposure routes (ingestion, inhalation, and dermal adsorption) (Agarwal et al. 2019; Rahman et al. 2021b). Except for Fe, Cr, and Cd, exposure pathways for both children and adults decreased in the following order: ingestion, dermal contact, and inhalation, suggesting that the primary exposure route to impair human health is household dust ingestion. HI values in the household's dust for the studied heavy metals decrease in the order Cr > Cd > Fe > Co > Mn > Ni > Cu > Zn. Oral consumption has already been established as the primary human exposure route for dust particles (Cheng et al. 2018; Gope et al. 2018). It might be accounted for by the fact that bigger size particles are difficult to resuspend inside and that residents' exposure to heavy metals through home dust is probably more dependent on hand-to-mouth contact (especially by small children) than on inhalation and dermal contact. Moreover, HQing had a greater impact on HI in children than in adults. In contrast to ingestion and inhalation, dermal contact had higher HQs for Cr and Cd. The outcome may be partially attributed to the kids' unique behavioral tendencies, such as their frequent mouth-to-hand or finger contact (Bahloul 2020; Wang et al. 2022). All of the metals examined in this study had HI values under 1, which shows that none of them pose non-carcinogenic dangers for kids and adults. According to Fig. 9, which was determined to be the critical value of noncarcinogenic effects (USEPA 2001), the overall HI values for both adults and children were less than 1, suggesting that no significant noncarcinogenic effects would be experienced by residents. The HI value for kids was approximately fifth fold than adults, indicating that kids are more likely to be exposed to heavy metals in indoor dust from all types of families, regardless of socioeconomic status.
Cancerous health risk
The heavy metals Cd, Cr, and Ni have been classified as carcinogenic by the IARC. According to the cancer risk assessment (Table 3), Cr (adult: (5.34E-08 – 2.15E-07), child: (5.62E-07 – 2.26E-06)) has the highest cancer risk, followed by Cd (adult: (5.75E-10 – 1.92E-08), child: (6.05E-09 – 2.02E-07), and Ni (adult:(4.89E-10 – 4.38E-09), child: (5.14E-09 – 4.52E-08) which are consistent with previous studies. Children are substantially more vulnerable to the cancer health effects of household dust since their carcinogenic risk is larger than that of adults (Gope et al. 2018).
The CR values for Cd, Cr, and Ni were found below safe levels (1E-06 to 1E-04). Therefore, Cd, Cr, and Ni in dust samples do not pose a cancer risk to the residents. Nevertheless, since those harmful metals influence several human organs, a random evaluation of cancer risk in children and adults should be conducted.
Conclusion
This study presents an analysis of selected heavy metals (Mn, Ni, Cu, Co, Zn, Cd, Fe, and Cr) and their health risks found in indoor dust in different socioeconomic residents of Dhanbad city. Fe had the highest average concentration of the chosen heavy metals in the household dust followed by Mn > Zn > Cu > Cr > Ni > Co > Cd. The inherent heterogeneity of the different urban areas was reflected by the considerable variations in total concentrations of heavy metals.
The major route of heavy metals exposure to humans, according to health hazard assessment, is ingestion, followed by dermal absorption and inhalation. HI values in the household’s dust for the studied heavy metals decrease in the order Cr > Cd > Fe > Co > Mn > Ni > Cu > Zn for the child, whereas for adults the sequence is Fe > Cr > Cd > Co > Cu > Mn > Ni > Zn. Various indoor sources (cooking/smoking/ personal care/domestic appliances) have caused to increase in Cr, Ni, Cu, and Co levels and their enrichment. HI also indicates that household dust poses a greater risk to human health, particularly for young children. The overall CR values for Co, Cr, and Ni were found below Tolerance levels (10–4—10–6). The CR values were observed in the decreasing order of Cr > Cd > Ni. It was discovered that residents of low socioeconomic status (households nearby the road) had a greater chance of having non-cancer hazards. The results of the PCA complemented and supported the findings of the Pearson Correlation Analysis adequately. The coal burned for cooking and various indoor facilities served as the common source for the elements Cu, Ni, Cr, and Zn, although Cd was influenced by a variety of sources. This study has demonstrated that residents are exposed to heavy metals from a variety of indoor and outdoor sources. However, due to the abundance of Cd, Cr, and Ni in the study area, children are at a considerably higher carcinogenic risk than adults. Although the hazards associated with many metals are within acceptable limits for adults, they shouldn't be disregarded because their concentration might soon be lethal to adults' health.
Data availability
Supplementary data is attached with this manuscript. The data presented in this study are also available on request from the corresponding authors.
References
Agarwal N, Shiva Nagendra SM, Peter AE, Yarpala D (2019) Microanalysis of the floor dust particles in the classrooms of tropical urban environment. J Inst Eng Ser A 100:447–458. https://doi.org/10.1007/s40030-019-00362-2
Ali N (2019) Polycyclic aromatic hydrocarbons (PAHs) in indoor air and dust samples of different Saudi microenvironments; health and carcinogenic risk assessment for the general population. Sci Total Environ 696:133995. https://doi.org/10.1016/j.scitotenv.2019.133995
Alotaibi MO, Albedair LA, Alotaibi NM, Elobeid MM, Al-Swadi HA, Alasmary Z, Ahmad M (2022) Pollution indexing and health risk assessment of heavy-metals-laden indoor and outdoor dust in elementary school environments in Riyadh, Saudi Arabia. Atmosphere (Basel) 13:464. https://doi.org/10.3390/atmos13030464
Arar S, Al-Hunaiti A, Masad MH, Maragkidou A, Wraith D, Hussein T (2019) Elemental contamination in indoor floor dust and its correlation with PAHs, fungi, and gram+/- bacteria. Int J Environ Res Public Health 16:3552. https://doi.org/10.3390/ijerph16193552
Bahloul M (2020) Pollution characteristics and health risk assessment of heavy metals in dry atmospheric deposits from Sfax solar saltern area in southeast of Tunisia 11 Medical and Health Sciences 1117 Public Health and Health Services. J Environ Heal Sci Eng 17:1085–1105. https://doi.org/10.1007/s40201-019-00423-5
Bao L, Wang S, Sun H, Huang W, Wang G, Nan Z (2019) Assessment of source and health risk of metal(loid)s in indoor/outdoor dust of university dormitory in Lanzhou City, China. Environ Sci Pollut Res 26:32333–32344. https://doi.org/10.1007/s11356-019-06365-7
Bhardwaj P, Singh B, Pandey A, Jain V, Kumar K (2017) Characterization and morphological analysis of summer and wintertime PM2. 5 aerosols over urban-rural locations in Delhi-NCR. Int J Appl Environ Sci 12:1009–1030
Bird DK (2009) The use of questionnaires for acquiring information on public perception of natural hazards and risk mitigation - A review of current knowledge and practice. Nat Hazards Earth Syst Sci 9:1307–1325. https://doi.org/10.5194/nhess-9-1307-2009
Blanchard O, Glorennec P, Mercier F, Bonvallot N, Chevrier C, Ramalho O, Mandin C, Bot BL (2014) Semivolatile organic compounds in indoor air and settled dust in 30 French dwellings. Environ Sci Technol 48:3959–3969. https://doi.org/10.1021/es405269q
Boisa N, Odagwe BU (2019) Indoor dust-based pollution status and risk assessment for a rural town, ebedei in nigeria hosting gas flare facility. J Environ Prot (Irvine,. Calif) 10:208–220. https://doi.org/10.4236/jep.2019.102012
Bora J, Deka P, Bhuyan P, Sarma KP, Hoque RR (2021) Morphology and mineralogy of ambient particulate matter over mid-Brahmaputra Valley: application of SEM–EDX, XRD, and FTIR techniques. SN Appl Sci 3:1–15. https://doi.org/10.1007/s42452-020-04117-8
Cao Z, Xu F, Covaci A, Wu M, Yu G, Wang B, Deng S, Huang J (2014) Differences in the seasonal variation of brominated and phosphorus flame retardants in office dust. Environ Int 65:100–106. https://doi.org/10.1016/j.envint.2013.12.011
Cao S, Wen D, Chen X, Duan X, Zhang L, Wang B, Qin N, Wei F (2022) Source identification of pollution and health risks to metals in household indoor and outdoor dust: a cross-sectional study in a typical mining town, China. Environ Pollut 293:118551. https://doi.org/10.1016/j.envpol.2021.118551
Chandramouli C, General R (2011) Census of India 2011. Provisional population totals. New Delhi: Government of India, 409–413
Chen CH, Wu CD, Chiang HC, Chu D, Lee KY, Lin WY, Yeh JI, Tsai KW, Guo YLL (2019) The effects of fine and coarse particulate matter on lung function among the elderly. Sci Rep 9:1–8. https://doi.org/10.1038/s41598-019-51307-5
Cheng Z, Chen LJ, Li HH, Lin JQ, Yang ZB, Yang YX, Xu XX, Xian JR, Shao JR, Zhu XM (2018) Characteristics and health risk assessment of heavy metals exposure via household dust from urban area in Chengdu, China. Sci Total Environ 619–620:621–629. https://doi.org/10.1016/j.scitotenv.2017.11.144
Deng M, Han X, Ge J, Liang X, Du B, Li J, Zeng L (2021) Prevalence of phthalate alternatives and monoesters alongside traditional phthalates in indoor dust from a typical e-waste recycling area: source elucidation and co-exposure risk. J Hazard Mater 413:125322. https://doi.org/10.1016/j.jhazmat.2021.125322
El-Zahhar AA, Idris AM, Fawy KF, Arshad M (2021) SEM, SEM-EDX, µ-ATR-FTIR and XRD for urban street dust characterisation. Int J Environ Anal Chem 101:988–1006. https://doi.org/10.1080/03067319.2019.1674849
Gope M, Masto RE, George J, Balachandran S (2018) Tracing source, distribution and health risk of potentially harmful elements (PHEs) in street dust of Durgapur, India. Ecotoxicol Environ Saf 154:280–293. https://doi.org/10.1016/j.ecoenv.2018.02.042
Gul HK, Gullu G, Babaei P, Nikravan A, Kurt-Karakus PB, Salihoglu G (2022) Assessment of house dust trace elements and human exposure in Ankara, Turkey. Environ Sci Pollut Res: 7718–7735. https://doi.org/10.1007/s11356-022-22700-x
Gustafsson Å, Krais AM, Gorzsás A, Lundh T, Gerde P (2018) Isolation and characterization of a respirable particle fraction from residential house-dust. Environ Res 161:284–290. https://doi.org/10.1016/j.envres.2017.10.049
Han Q, Wang M, Cao J, Gui C, Liu Y, He X, He Y, Liu Y (2020) Health risk assessment and bioaccessibilities of heavy metals for children in soil and dust from urban parks and schools of Jiaozuo, China. Ecotoxicol Environ Saf 191:110157. https://doi.org/10.1016/j.ecoenv.2019.110157
Hakanson L (1980) An ecological risk index for aquatic pollution control. A sedimentological approach. Water Res 14(8):975–1001. https://doi.org/10.1016/0043-1354(80)90143-8
Hassan SKM (2012) Metal concentrations and distribution in the household, stairs and entryway dust of some Egyptian homes. Atmos Environ 54:207–215. https://doi.org/10.1016/j.atmosenv.2012.02.013
Hassan SK, El-Abssawy AA, Khoder MI (2015) Chemical composition, characterization and factors affecting household dust (<20 & #181;m) in Greater Cairo, Egypt. Open J Air Pollut 04:184–197. https://doi.org/10.4236/ojap.2015.44016
He CT, Zheng XB, Yan X, Zheng J, Wang MH, Tan X, Qiao L, Chen SJ, Yang ZY, Mai BX (2017) Organic contaminants and heavy metals in indoor dust from e-waste recycling, rural, and urban areas in South China: spatial characteristics and implications for human exposure. Ecotoxicol Environ Saf 140:109–115. https://doi.org/10.1016/j.ecoenv.2017.02.041
He KQ, Yuan CG, Yin LQ, Zhang KG, Xu P yao, Xie JJ, Shen YW (2019) A comparative study on arsenic fractions in indoor/outdoor particulate matters: a case in Baoding, China. Environ. Monit Assess 191. https://doi.org/10.1007/s10661-019-7643-5
Hejami AA, Davis M, Prete D, Lu J, Wang S (2020) Heavy metals in indoor settled dusts in Toronto, Canada. Sci Total Environ 703:134895. https://doi.org/10.1016/j.scitotenv.2019.134895
Hou S, Zheng N, Tang L, Ji X, Li Y, Hua X (2019) Pollution characteristics, sources, and health risk assessment of human exposure to Cu, Zn, Cd and Pb pollution in urban street dust across China between 2009 and 2018. Environ Int 128:430–437. https://doi.org/10.1016/j.envint.2019.04.046
Huang M, Wang W, Chan CY, Cheung KC, Man YB, Wang X, Wong MH (2014) Contamination and risk assessment (based on bioaccessibility via ingestion and inhalation) of metal(loid)s in outdoor and indoor particles from urban centers of Guangzhou, China. Sci Total Environ 479–480:117–124. https://doi.org/10.1016/j.scitotenv.2014.01.115
Isley CF, Fry KL, Liu X, Filippelli GM, Entwistle JA, Martin AP, Kah M, Meza-Figueroa D, Shukle JT, Jabeen K, Famuyiwa AO, Wu L, Sharifi-Soltani N, Doyi INY, Argyraki A, Ho KF, Dong C, Gunkel-Grillon P, Aelion CM, Taylor MP (2022) International analysis of sources and human health risk associated with trace metal contaminants in residential indoor dust. Environ Sci Technol 56:1053–1068. https://doi.org/10.1021/acs.est.1c04494
Iwegbue CMA, Oliseyenum EC, Martincigh BS (2017) Spatio-temporal distribution of metals in household dust from rural, semi-urban and urban environments in the Niger Delta, Nigeria. Environ Sci Pollut Res. https://doi.org/10.1007/s11356-017-8609-1
Iwegbue CMA, Obi G, Emoyan OO, Odali EW, Egobueze FE, Tesi GO, Nwajei GE, Martincigh BS (2018) Characterization of metals in indoor dusts from electronic workshops, cybercafés and offices in southern Nigeria: implications for on-site human exposure. Ecotoxicol Environ Saf 159:342–353. https://doi.org/10.1016/j.ecoenv.2018.04.070
Kaar S, Krizan D, Schwabe J, Hofmann H, Hebesberger T, Commenda C, Samek L (2018) Influence of the Al and Mn content on the structure-property relationship in density reduced TRIP-assisted sheet steels. Mater Sci Eng A 735:475–486. https://doi.org/10.1016/j.msea.2018.08.066
Kelepertzis E, Argyraki A, Botsou F, Aidona E, Szabó Á, Szabó C (2019) Tracking the occurrence of anthropogenic magnetic particles and potentially toxic elements (PTEs) in house dust using magnetic and geochemical analyses. Environ Pollut 245:909–920. https://doi.org/10.1016/j.envpol.2018.11.072
Kesavachandran CN, Kamal R, Bihari V, Pathak MK, Singh A (2015) Particulate matter in ambient air and its association with alterations in lung functions and respiratory health problems among outdoor exercisers in national capital region, India. Atmos Pollut Res 6:618–625. https://doi.org/10.5094/APR.2015.070
Khan D, Kumar A, Samadder SR (2016) Impact of socioeconomic status on municipal solid waste generation rate. Waste Manag 49:15–25. https://doi.org/10.1016/j.wasman.2016.01.019
Kitagawa YKL, Kumar P, Galvão ES, Santos JM, Reis NC, Nascimento EGS, Moreira DM (2022) Exposure and dose assessment of school children to air pollutants in a tropical coastal-urban area. Sci Total Environ 803:149747. https://doi.org/10.1016/j.scitotenv.2021.149747
Kraepiel AML, Dere AL, Herndon EM, Brantley SL (2015) Natural and anthropogenic processes contributing to metal enrichment in surface soils of central Pennsylvania. Biogeochemistry 123:265–283. https://doi.org/10.1007/s10533-015-0068-5
Kulshrestha A, Massey DD, Masih J, Taneja A (2014) Source characterization of trace elements in indoor environments at urban, rural and roadside sites in a Semi Arid Region of India. Aerosol Air Qual Res 14:1738–1751. https://doi.org/10.4209/aaqr.2013.05.0147
Kumar S, Jain MK (2022) Applied Geochemistry Characterization and morphometric study of household settled dust : a case study in Dhanbad, the coal capital of India. Appl Geochemistry 144:105398. https://doi.org/10.1016/j.apgeochem.2022.105398
Kumar A, Samadder SR (2017) An empirical model for prediction of household solid waste generation rate – A case study of Dhanbad, India. Waste Manag 68:3–15. https://doi.org/10.1016/j.wasman.2017.07.034
Latif MT, Yong SM, Saad A, Mohamad N, Baharudin NH, Mokhtar MB, Tahir NM (2014) Composition of heavy metals in indoor dust and their possible exposure: a case study of preschool children in Malaysia. Air Qual Atmos Heal 7:181–193. https://doi.org/10.1007/s11869-013-0224-9
Li X, Zhang S, Yang M (2014) Accumulation and risk assessment of heavy metals in dust in main living areas of Guiyang City, Southwest China. Chinese J Geochemistry 33:272–276. https://doi.org/10.1007/s11631-014-0687-x
Li N, Han W, Tang J, Bian J, Sun S, Song T (2018) Pollution characteristics and human health risks of elements in road dust in Changchun, China. Int J Environ Res Public Health 15. https://doi.org/10.3390/ijerph15091843
Li L, Qiu Y, Gustafsson Å, Krais AM, Weiss JM, Lundh T, Bergman Å (2019) Characterization of residential household dust from Shanghai by particle size and analysis of organophosphorus flame retardants and metals. Environ Sci Eur 31. https://doi.org/10.1186/s12302-019-0279-9
Liang CS, Wu H, Li HY, Zhang Q, Li Z, He KB (2020) Efficient data preprocessing, episode classification, and source apportionment of particle number concentrations. Sci Total Environ 744:1–17. https://doi.org/10.1016/j.scitotenv.2020.140923
Liu B, Huang F, Yu Y, Li X, He Y, Gao L, Hu X (2021) Heavy metals in indoor dust across China: occurrence, sources and health risk assessment. Arch Environ Contam Toxicol 81:67–76. https://doi.org/10.1007/s00244-021-00849-9
Mahfouz MM, Yigiterhan O, Elnaiem AE, Hassan HM, Alfoldy B (2019) Elemental compositions of particulate matter retained on air condition unit’s filters at Greater Doha, Qatar. Environ Geochem Health 41:2533–2548. https://doi.org/10.1007/s10653-019-00304-8
Marinho Reis AP, Cave M, Sousa AJ, Wragg J, Rangel MJ, Oliveira AR, Patinha C, Rocha F, Orsiere T, Noack Y (2018) Lead and zinc concentrations in household dust and toenails of the residents (Estarreja, Portugal): a source-pathway-fate model. Environ Sci Process Impacts 20:1210–1224. https://doi.org/10.1039/c8em00211h
Matt GE, Quintana PJE, Hoh E, Zakarian JM, Dodder NG, Record RA, Hovell MF, Mahabee-Gittens EM, Padilla S, Markman L, Watanabe K, Novotny TE (2020) Persistent tobacco smoke residue in multiunit housing: legacy of permissive indoor smoking policies and challenges in the implementation of smoking bans. Prev Med Reports 18:101088. https://doi.org/10.1016/j.pmedr.2020.101088
Melymuk L, Demirtepe H, Jílková SR (2020) Indoor dust and associated chemical exposures. Curr Opin Environ Sci Heal 15:1–6. https://doi.org/10.1016/j.coesh.2020.01.005
Men C, Liu R, Xu F, Wang Q, Guo L, Shen Z (2018) Pollution characteristics, risk assessment, and source apportionment of heavy metals in road dust in Beijing, China. Sci Total Environ 612:138–147. https://doi.org/10.1016/j.scitotenv.2017.08.123
Moghtaderi M, Ashraf MA, Moghtaderi T, Teshnizi SH, Nabavizadeh SH (2020) Heavy metal concentration in classroom dust samples and its relationship with childhood asthma: a study from islamic Republic of Iran. East Mediterr Heal J 26:594–601. https://doi.org/10.26719/emhj.19.072
Mostafaii GR, Bakhtyari Z, Atoof F, Baziar M, Fouladi-Fard R, Rezaali M, Mirzaei N (2021) Health risk assessment and source apportionment of heavy metals in atmospheric dustfall in a city of Khuzestan Province, Iran. J Environ Heal Sci Eng 19:585–601. https://doi.org/10.1007/s40201-021-00630-z
Mueller G (1979) Schwermetalle in Den Sedimenten Des Rheins - Veranderungen Seit 1971. Umsch Wissensch Techn 79:778–783
Muhamad-Darus F, Nasir RA, Sumari SM, Ismail ZS, Omar NA (2017) Nursery schools: characterization of heavy metal content in indoor dust. Asian J Environ Stud 2:63–70. https://doi.org/10.21834/aje-bs.v2i5.223
Naimabadi A, Ghasemi A, Mohtashami M, Saeidi J, Bakaeian M, Haddad Mashadrizeh A, Azimi-Nezhad M, Mohammadi AA (2022) Heavy metal analysis in of indoor and outdoor dust extracts and cytotoxicity evaluation and inflammation factors on lung, gastric and skin cell lines. Heliyon 8:e12414. https://doi.org/10.1016/j.heliyon.2022.e12414
Neupane BB, Sharma A, Giri B, Joshi MK (2020) Characterization of airborne dust samples collected from core areas of Kathmandu Valley. Heliyon 6:e03791. https://doi.org/10.1016/j.heliyon.2020.e03791
Nkansah MA, Fianko JR, Mensah S, Debrah M, Francis GW (2015) Determination of heavy metals in dust from selected nursery and kindergarten classrooms within the Kumasi metropolis of Ghana. Cogent Chem 1:1119005. https://doi.org/10.1080/23312009.2015.1119005
Pal M, Gope M, Basu A, Laha T, Masto RE, Labar R, Kundu TK, Hoque RR, Khillare PS, Balachandran S (2021) Indoor quality of residential homes and schools of an industrial area in asansol: characterization, bioaccessibility and health risk assessment of potentially toxic elements. Nat Environ Pollut Technol 20:13–28. https://doi.org/10.46488/NEPT.2021.V20I01.002
Parhizkar G, Doroodzani AK, Dobaradaran S, Ramavandi B, Hashemi SE, Raeisi A, Nabipour I, Keshmiri S, Darabi AH, Afrashte S, Khamisipour G, Keshtkar M (2021) Childhood exposure to metal(loid)s in industrial and urban areas along the Persian Gulf using toenail tissue as a biomarker. Environ Pollut 291:118090. https://doi.org/10.1016/j.envpol.2021.118090
Park DI (2021) Development and validation of a knowledge, attitudes and practices questionnaire on covid-19 (Kap covid-19). Int J Environ Res Public Health 18. https://doi.org/10.3390/ijerph18147493
Patel DK, Jain MK (2021) Health risk assessment of trace element in the ambient air along the roadside in Dhanbad, India. Int J Environ Sci Technol 19(5):4107–4122. https://doi.org/10.1007/s13762-021-03266-6
Pipal AS, Rohra H, Tiwari R, Taneja A (2021) Particle size distribution, morphometric study and mixing structure of accumulation and ultrafine aerosols emitted from indoor activities in different socioeconomic micro-environment. Atmos Pollut Res 12:101–111. https://doi.org/10.1016/j.apr.2021.02.015
Pobi KK, Nayek S, Gope M, Rai AK, Saha R (2020) Sources evaluation, ecological and health risk assessment of potential toxic metals (PTMs) in surface soils of an industrial area, India. Environ Geochem Health 42:4159–4180. https://doi.org/10.1007/s10653-020-00517-2
Prabhu V, Gupta SK, Madhwal S, Shridhar V (2019) Exposure to atmospheric particulates and associated respirable deposition dose to street vendors at the residential and commercial sites in Dehradun City. Saf Health Work 10:237–244. https://doi.org/10.1016/j.shaw.2019.01.005
Rahman MS, Jolly YN, Akter S, Kamal NA, Rahman R, Choudhury TR, Begum BA (2021a) Sources of toxic elements in indoor dust sample at export processing zone (EPZ) area: Dhaka, Bangladesh; and their impact on human health. Environ Sci Pollut Res. https://doi.org/10.1007/s11356-021-13167-3
Rahman MS, Kumar P, Ullah M, Jolly YN, Akhter S, Kabir J, Begum BA, Salam A (2021b) Elemental analysis in surface soil and dust of roadside academic institutions in Dhaka city, Bangladesh and their impact on human health. Environ Chem Ecotoxicol 3:197–208. https://doi.org/10.1016/j.enceco.2021.06.001
Rasmussen PE, Levesque C, Chénier M, Gardner HD (2018) Contribution of metals in resuspended dust to indoor and personal inhalation exposures: relationships between PM10 and settled dust. Build Environ 143:513–522. https://doi.org/10.1016/j.buildenv.2018.07.044
Rohra H, Tiwari R, Khandelwal N, Taneja A (2018) Mass distribution and health risk assessment of size segregated particulate in varied indoor microenvironments of Agra, India - A case study. Urban Clim 24:139–152. https://doi.org/10.1016/j.uclim.2018.01.002
Rout TK, Masto RE, Padhy PK, Ram LC, George J, Joshi G (2015) Heavy metals in dusts from commercial and residential areas of Jharia coal mining town. Environ Earth Sci 73:347–359. https://doi.org/10.1007/s12665-014-3429-9
Safiur Rahman M, Khan MDH, Jolly YN, Kabir J, Akter S, Salam A (2019) Assessing risk to human health for heavy metal contamination through street dust in the Southeast Asian Megacity: Dhaka, Bangladesh. Sci Total Environ 660:1610–1622. https://doi.org/10.1016/j.scitotenv.2018.12.425
Sahu D, Ramteke S, Dahariya NS, Sahu BL, Patel KS, Matini L, Nicolas J, Yubero E, Hoinkis J (2016) Assessment of road dust contamination in India. Atmos Clim Sci 06:77–88. https://doi.org/10.4236/acs.2016.61006
Sahu V, Elumalai SP, Gautam S, Singh NK, Singh P (2018) Characterization of indoor settled dust and investigation of indoor air quality in different micro-environments. Int J Environ Health Res 28:419–431. https://doi.org/10.1080/09603123.2018.1481498
Saraga D, Maggos T, Sadoun E, Fthenou E, Hassan H, Tsiouri V, Karavoltsos S, Sakellari A, Vasilakos C, Kakosimos K (2017) Chemical characterization of indoor and outdoor particulate matter (PM2.5, PM10) in Doha, Qatar. Aerosol Air Qual Res 17:1156–1168. https://doi.org/10.4209/aaqr.2016.05.0198
Shi T, Wang Y (2021) Heavy metals in indoor dust: Spatial distribution, influencing factors, and potential health risks. Sci Total Environ 755:142367. https://doi.org/10.1016/j.scitotenv.2020.142367
Siddiqui AU, Jain MK, Masto RE (2020) Pollution evaluation, spatial distribution, and source apportionment of trace metals around coal mines soil: the case study of eastern India. Environ Sci Pollut Res 27:10822–10834. https://doi.org/10.1007/s11356-019-06915-z
Singh P, Saini R, Taneja A (2014) Physicochemical characteristics of PM2.5: low, middle, and high-income group homes in Agra, India-a case study. Atmos Pollut Res 5:352–360. https://doi.org/10.5094/APR.2014.041
Suresh G, Ramasamy V, Meenakshisundaram V, Venkatachalapathy R, Ponnusamy V (2011) Influence of mineralogical and heavy metal composition on natural radionuclide concentrations in the river sediments. Appl Radiat Isot 69:1466–1474. https://doi.org/10.1016/j.apradiso.2011.05.020
Tao Y, Yuan Y, Cui Y, Zhu L, Zhao Z, Ma S, Ye Z, Ge X (2021) Comparative analysis of the chemical characteristics and sources of fine atmospheric particulate matter (PM2.5) at two sites in Changzhou, China. Atmos Pollut Res 12(8):101124. https://doi.org/10.1016/j.apr.2021.101124
Taylor SR, McLennan SM (1995) The geochemical evolution of the continental crust. Rev Geophys 33(2):241–265. https://doi.org/10.1029/95RG00262
Tomlinson DL, Wilson JG, Harris CR, Jeffrey DW (1980) Problems in the assessment of heavy-metal levels in estuaries and the formation of a pollution index. Helgoländer Meeresuntersuchungen 33:566–575. https://doi.org/10.1007/BF02414780
Torres-Sánchez R, de la Campa AMS, Beltrán M, Sánchez-Rodas D, de la Rosa JD (2017) Geochemical anomalies of household dust in an industrialized city (Huelva, SW Spain). Sci Total Environ 587–588:473–481. https://doi.org/10.1016/j.scitotenv.2017.02.167
Turner A (2019) Cadmium pigments in consumer products and their health risks. Sci Total Environ 657:1409–1418. https://doi.org/10.1016/j.scitotenv.2018.12.096
Ugwu KE, Ofomatah AC (2021) Concentration and risk assessment of toxic metals in indoor dust in selected schools in Southeast, Nigeria. SN Appl Sci 3:1–13. https://doi.org/10.1007/s42452-020-04099-7
Underhill LJ, Bose S, Williams DL, Romero KM, Malpartida G, Breysse PN, Klasen EM, Combe JM, Checkley W, Hansel NN (2015) Association of roadway proximity with indoor air pollution in a Peri-urban community in Lima, Peru. Int J Environ Res Public Health 12:13466–13481. https://doi.org/10.3390/ijerph121013466
USEPA (2001) Risk assessment guidance for superfund (RAGS) volume III - Part A: process for conducting probabilistic risk assessment, appendix B. Off Emerg Remedial Response US Environ Prot Agency III:1–385
Victoria A, Dampare S, Cobbina SJ, Duwiejuah AB (2014) Heavy metals concentration in road dust in the Bolgatanga Municipality, Ghana heavy metals concentration in road dust in the Bolgatanga Municipality, Ghana. J Environ Pollut Hum Heal 2:74–80. https://doi.org/10.12691/jephh-2-4-1
Wan X, Kang S, Rupakheti M, Zhang Q, Tripathee L, Guo J, Chen P, Rupakheti D, Panday AK, Lawrence MG, Kawamura K, Cong Z (2019) Molecular characterization of organic aerosols in the Kathmandu Valley, Nepal: insights into primary and secondary sources. Atmos Chem Phys 19:2725–2747. https://doi.org/10.5194/acp-19-2725-2019
Wang B, Gao F, Li Y, Lin C, Cheng H, Duan X (2022) Assessment of children’s metal exposure via hand wipe, outdoor soil and indoor dust and their associations with blood biomarkers. Int J Environ Res Public Health 19:14614. https://doi.org/10.3390/ijerph192114614
Wang M, Lv Y, Lv X, Wang Q, Li Y, Lu P, Yu H, Wei P, Cao Z, An T (2023) Distribution, sources and health risks of heavy metals in indoor dust across China. Chemosphere 313:137595. https://doi.org/10.1016/j.chemosphere.2022.137595
Yaparla D, Nagendra SMS, Gummadi SN (2019) Characterization and health risk assessment of indoor dust in biomass and LPG-based households of rural Telangana, India. J Air Waste Manag Assoc. https://doi.org/10.1080/10962247.2019.1668874
Ying L, Shaogang L, Xiaoyang C (2016) Assessment of heavy metal pollution and human health risk in urban soils of a coal mining city in East China. Hum Ecol Risk Assess 22:1359–1374. https://doi.org/10.1080/10807039.2016.1174924
Zeng L, Du B, Lv L, Gao J, Zhang C, Tong L, Liu G (2020) Occupant exposure and ventilation conditions in Chinese residential kitchens: Site survey and measurement for an old residential community in Shanghai. J Build Eng 31:101406. https://doi.org/10.1016/j.jobe.2020.101406
Zhang Y, Zhao B, Jiang Y, Xing J, Sahu SK, Zheng H, Ding D, Cao S, Han L, Yan C, Duan X, Hu J, Wang S, Hao J (2022) Non-negligible contributions to human health from increased household air pollution exposure during the COVID-19 lockdown in China. Environ Int 158:106918. https://doi.org/10.1016/j.envint.2021.106918
Zhao N, Lu X, Chao S, Xu X (2015) Multivariate statistical analysis of heavy metals in less than 100 μm particles of street dust from Xining, China. Environ Earth Sci 73:2319–2327. https://doi.org/10.1007/s12665-014-3578-x
Zhao X, Li Z, Wang D, Tao Y, Qiao F, Lei L, Huang J, Ting Z (2021) Characteristics, source apportionment and health risk assessment of heavy metals exposure via household dust from six cities in China. Sci Total Environ 762:143126. https://doi.org/10.1016/j.scitotenv.2020.143126
Zhou L, Liu G, Shen M, Hu R, Sun M, Liu Y (2019) Characteristics and health risk assessment of heavy metals in indoor dust from different functional areas in Hefei, China. Environ Pollut 251:839–849. https://doi.org/10.1016/j.envpol.2019.05.058
Zhou L, Liu G, Shen M, Hu R, Liu Y (2020) Source identification of heavy metals and stable carbon isotope in indoor dust from different functional areas in Hefei, China. Sci Total Environ 710:135599. https://doi.org/10.1016/j.scitotenv.2019.135599
Zhou L, Liu G, Shen M, Liu Y (2022) Potential ecological and health risks of heavy metals for indoor and corresponding outdoor dust in Hefei, Central China. Chemosphere 302:134864. https://doi.org/10.1016/j.chemosphere.2022.134864
Živančev J, Antić I, Buljovčić M, Đurišić-Mladenović N (2022) A case study on the occurrence of polycyclic aromatic hydrocarbons in indoor dust of Serbian households: distribution, source apportionment and health risk assessment. Chemosphere 295. https://doi.org/10.1016/j.chemosphere.2022.133856
Funding
This research did not receive any specific grant from funding agencies in the public, commercial, or not-for-profit sectors.
Author information
Authors and Affiliations
Contributions
Shravan Kumar: Writing- Original draft preparation, Methodology, Formal analysis, Data curation, Visualization. Manish Kumar Jain: Conceptualization, Supervision, Writing Reviewing, and Editing.
Corresponding author
Ethics declarations
Ethics approval and consent to participate
Not applicable.
Consent for publication
Informed consent was obtained from all subjects involved in the study.
Competing interests
The authors declare that they have no competing interests.
Additional information
Publisher's Note
Springer Nature remains neutral with regard to jurisdictional claims in published maps and institutional affiliations.
Supplementary Information
Below is the link to the electronic supplementary material.
Rights and permissions
Springer Nature or its licensor (e.g. a society or other partner) holds exclusive rights to this article under a publishing agreement with the author(s) or other rightsholder(s); author self-archiving of the accepted manuscript version of this article is solely governed by the terms of such publishing agreement and applicable law.
About this article
Cite this article
Kumar, S., Jain, M.K. A comparative study of health risks of selected potentially toxic metals in household dust from different socio-economical houses of Dhanbad city, India. Air Qual Atmos Health 17, 1547–1564 (2024). https://doi.org/10.1007/s11869-024-01527-0
Received:
Accepted:
Published:
Issue Date:
DOI: https://doi.org/10.1007/s11869-024-01527-0