Abstract
Purpose
Myocardial uptake can hamper visualization of lung tumors, atherosclerotic plaques, and inflammatory diseases in 2-deoxy-2-[18F]fluoro-D-glucose ([18F]FDG) studies because it leads to spillover in adjacent structures. Several preparatory pre-imaging protocols (including dietary restrictions and drugs) have been proposed to decrease physiological [18F]FDG uptake by the heart, although their effect on tumor glucose metabolism remains largely unknown. The objective of this study was to assess the effects of a ketogenic diet (as an alternative protocol to fasting) on tumor glucose metabolism assessed by [18F]FDG positron emission tomography (PET) in a mouse model of lung cancer.
Procedures
PET scans were performed 60 min after injection of 18.5 MBq of [18F]FDG. PET data were collected for 45 min, and an x-ray computed tomograph (CT) image was acquired after the PET scan. A PET/CT study was obtained for each mouse after fasting and after the ketogenic diet. Quantitative data were obtained from regions of interest in the left ventricular myocardium and lung tumor.
Results
Three days on a ketogenic diet decreased mean standard uptake value (SUVmean) in the myocardium (SUVmean 0.95 ± 0.36) more than one night of fasting (SUVmean 1.64 ± 0.93). Tumor uptake did not change under either dietary condition.
Conclusions
These results show that 3 days on high-fat diets prior to [18F]FDG-PET imaging does not change tumor glucose metabolism compared with one night of fasting, although high-fat diets suppress myocardial [18F]FDG uptake better than fasting.
Similar content being viewed by others
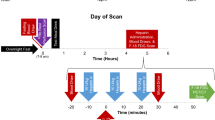
Explore related subjects
Discover the latest articles, news and stories from top researchers in related subjects.Avoid common mistakes on your manuscript.
Introduction
Positron emission tomography (PET) with 2-deoxy-2- [18F]fluoro-D-glucose ([18F]FDG) is a highly sensitive and quantitative technique that has many clinical applications in the field of molecular imaging, most notably in oncology [1]. Tumor cells, in the same way as many other cells (macrophages, lymphocytes, granulocytes, and activated fibroblasts), avidly take up [18F]FDG, thus enabling PET imaging to detect cancer and inflammatory and infectious processes [2, 3]. However, regular uptake of [18F]FDG results in well-known problems of detectability in several applications [4, 5], mostly where the target is close to an area of high uptake. The high [18F]FDG uptake in the absence of disease observed in some organs, such as the brain and heart, hampers visualization and diagnosis of neighboring lesions.
Several preparatory pre-imaging protocols (including dietary restrictions and drugs) have been proposed to decrease physiological [18F]FDG uptake by the heart [6,7,8,9,10], thus may improve the detectability of lung and myocardial lesions and reducing the number of false-positive readings. We previously compared two methods for reducing myocardial uptake of [18F]FDG in healthy animals [11] by demonstrating that a ketogenic diet prior to [18F]FDG-PET imaging suppresses myocardial uptake in mice better than blocking glucose transport into cardiomyocytes with verapamil [8]. Our findings were in agreement with those of previous studies, which proposed diets as a powerful alternative to fasting to reduce myocardial uptake of [18F]FDG in both clinical scenarios [10, 12, 13] and preclinical scenarios [9]. We also validated a ketogenic diet in a murine lung inflammation model (tracheal Escherichia coli lipopolysaccharide challenge), although, given the severe pulmonary distress induced, the efficiency of the ketogenic diet was slightly poorer than in healthy mice [11].
Nebeling, et al. [14] reported reduced tumor [18F]FDG uptake in two pediatric cancer patients after a long period on a high-fat diet. Furthermore, in vitro experiments showed a reduction in tumor viability due to the inability of cancer cells (gliomas, astrocytomas, and neuroblastomas) to metabolize ketogenic bodies [15]. These findings are supported by several preclinical studies [16,17,18,19] that suggest a potential anticancer effect of long periods on a ketogenic diet (from 13 days [16] to 104 days [17]). Although the effect of dietary preparation (diets or fasting) before administration of [18F]FDG lasts only a few hours [10, 11, 20, 21], this brief application is enough to enhance [18F]FDG uptake by carotid plaques, lung tumors, and pulmonary or cardiac inflammation/infection [11, 22].
Even though fasting remains the most common preparatory protocol for [18F]FDG-PET studies, in the last decade, diets have been proposed as a better and more powerful alternative for suppressing uptake of [18F]FDG by the heart [10, 13, 21], both in research applications and clinical applications related to various heart conditions (e.g., endocarditis [22], coronary plaque [21]). To our knowledge, no attempt has been made to study the effect of high-fat diets on tumor uptake. Therefore, we hypothesized that high-fat diets can also be a suitable protocol for improving the diagnosis of lung tumors with no negative effects on tumor [18F]FDG uptake. We performed a longitudinal [18F]FDG-PET study to compare the effects of a ketogenic diet on tumor glucose metabolism with those of a conventional fasting protocol in a mouse model of lung cancer.
Material and Methods
Experimental Procedures
The study was performed on 14 lung tumor–bearing K-RasLSLG12Vgeo; p53lox/lox compound mice in a pure C57/BL6J background. The adenocarcinomas were induced in anesthetized 8- to 10-week-old mice (intraperitoneal injection of ketamine 75 mg/kg, xylazine 12 mg/kg) by intranasal instillation of a single dose of 106 pfu of Adeno-Cre virus (Gene Transfer Vector Core, University of Iowa). The animals were included in the study when the tumors could be detected by computed tomography (mean latency of 5 months). Mice were housed in cages under standard conditions and allowed food and water ad libitum. Each animal underwent [18F]FDG PET/CT under two different conditions (3 days were left between PET scans): after one night of fasting (mice were deprived of food for 15–18 h before [18F]FDG injection) and after 3 days on a ketogenic diet (TD 96355, Harlan).
Blood glucose levels (BGLs) were measured before administration of [18F]FDG using a glucose meter (Glucocard TM G+ meter, A. Menarini, Spain) on conscious animals. BGL was measured to guarantee that the animals reach [18F]FDG administration under similar glucose conditions. The control group comprised ten additional C57/BL6J healthy animals that received a regular diet (A04, SAFE).
Image Acquisition
All the studies were performed with a small-animal PET/CT scanner (Argus, SEDECAL, Spain [23, 24]). PET studies were acquired 60 min after intraperitoneal administration of 19.4 ± 1.02 MBq of [18F]FDG. During uptake, the animals were kept awake and warm under infrared light to prevent brown fat uptake. PET data were collected for 45 min with the mice anesthetized using 1.5 % sevoflurane in oxygen at 3 l/min and reconstructed using OSEM-2D with 50 subsets and 3 iterations. The voxel size of the reconstructed images was 0.388 mm in the transaxial plane and 0.775 mm in the axial plane. After the PET scan, a CT study was acquired using an X-ray beam current of 240 μA and a tube voltage of 40 kVp and reconstructed using an FDK algorithm [23]. These CT scans were used as anatomical templates.
Standardized Uptake Value
PET/CT images were analyzed using Multimodality Workstation software [25] (MMWKS, Spain). On each CT image, regions of interest (ROIs) were delimited over all identifiable tumor lesions, brown fat, skeletal muscle (triceps), and the heart (20 circular ROIs measuring 2.5 mm in diameter). These ROIs were applied to the automatically coregistered PET images to measure their corresponding standard uptake value (SUV, mean, and maximum).
Histopathology
The lungs of nine animals (fasting n = 3 and ketogenic diet = 6) were harvested after the last PET/CT image to study the possible influence of dietary conditions on tumor histology. The histology protocol consisted of fixation of the lung lobes in 10 % buffered formalin (Sigma) and embedding in paraffin, followed by staining with hematoxylin-eosin and immunostaining against glyceraldehyde-3-phosphate dehydrogenase (GAPDH, Millipore, MAB374). Microscopic images were scanned and photos exported into the software application Zen 2012 (ZEISS, Germany) for quantification. GAPDH was assessed by scoring the intensity of staining of the tumor cells as “low,” “mild,” and “intense” expression [26]. Tumors were also graded as adenoma or adenocarcinoma by an expert pathologist based on hematoxylin-eosin staining.
Statistical Analysis
The Wilcoxon test was used for paired comparisons (differences in tumor uptake, tumor size, weight, heart uptake, brown fat uptake, skeletal muscle uptake, and BGL), and the Mann-Whitney U test was used for nonpaired data (histopathology, tumor uptake) and also to compare myocardial uptake and BGL with the control group. Finally, relationships between BGL, histopathology (intensity of GAPDH staining), and tumor [18F]FDG uptake were assessed using the Spearman’s correlation analysis. Nonparametric tests were selected because of the reduced sample size. Data are reported as mean (± standard deviation), and statistical significance was set at p < 0.05.
Ethics Statement
All animal procedures were approved by the Animal Experimentation Ethics Committee of Hospital General Universitario Gregorio Marañón (ES280790000087) and the Ethics Committees of CNIO and the Carlos III Health Institute, Madrid, and performed according to European regulations (2010/63/UE) and National regulations (RD 53/2013).
Results
Quantification of myocardial SUVmean showed that, compared with control group (SUVmean 2.03 ± 1.21), the greatest reduction in [18F]FDG uptake was achieved with the ketogenic diet (SUVmean 0.95 ± 0.36; p = 0.004), whereas fasting produced no significant reduction in myocardial uptake (SUVmean 1.64 ± 0.93; p = NS). Besides, a longitudinal comparison between the same animals in both diet conditions shows that 3 days on ketogenic diet decrease [18F]FDG myocardium uptake better than one night on fasting (p = 0.013). Fig. 1 shows an example of [18F]FDG-PET images from three different animals after both dietary conditions. The myocardium is completely suppressed after ketogenic feeding in most cases improving tumor visualization, whereas one night of fasting induced heterogenic suppression, from complete to non-suppression. Brown fat uptake (ketogenic diet 0.83 ± 0.15, fasting 2.17 ± 2.83, p = 0.167) and skeletal muscle uptake (ketogenic diet 0.57 ± 0.25, fasting 0.57 ± 0.28, p = 0.660) did not showed statistically significant differences between both dietary conditions.
Figure 2 shows tumor tissue and myocardial uptake values after each dietary condition. Tumor SUVmax did not showed significant differences after both dietary conditions (ketogenic diet 4.04 ± 1.46, and fasting 3.37 ± 1.48, p = 0.074). No significant differences were observed on tumor size between scans (fasting 0.112 ± 0.121 cm3 and ketogenic diet 0.139 ± 0.156 cm3, p = 0.397). Our basis for using SUVmax instead of SUVmean was that it is the most common marker in oncological applications.
Table 1 shows BGL before administration of [18F]FDG for each dietary condition. BGL was lower in both cases (ketogenic diet, 70.5 ± 27.29 mg/dl, and fasting, 72.14 ± 21.15 mg/dl, p = 0.002) than in control animals (no fasting 110.7 ± 21.3 mg/dl). Differences in BGL between fasting and the ketogenic diet did not reach statistical significance. No significant relationship was found between BGL and tumor uptake or myocardial uptake. On average, the ketogenic diet increased the animals’ weight (24.96 ± 3.60 g) by 1 g compared with the data collected after one night of fasting (23.96 ± 3.85 g, p = 0.009).
Figure 3a shows representative immunohistochemical GAPDH staining on non-small cell lung cancer (adenomas and adenocarcinomas). Adenocarcinomas had a higher SUVmax than adenomas (2.71 ± 1.52 vs. 1.54 ± 0.96, p = 0.036), and tumors with intense GAPDH activity had a higher SUVmax than low-mild GAPDH expression (2.98 ± 1.59 vs. 1.46 ± 0.38, p = 0.024). Our results showed a significant positive association between tumor 18F-FDG uptake and GAPDH expression (rho = 0.5, p = 0.02, Spearman test).
Discussion
[18F]FDG PET/CT is an essential tool for the study of tumor progression when staging cancer patients [27] and also in research applications [20], where, for instance, it provides a rapid platform for the preclinical evaluation of treatments in rodent models [28]. Consequently, it is important to consider any possible anticancer effect of ketogenic diets, since this can interfere with the assessment of new therapeutic strategies.
The aim of this study was to explore the possibilities of a ketogenic diet (as an alternative protocol to fasting) to improve detection of lung cancer in a mouse model by analyzing tumor and myocardial [18F]FDG uptake and the expression of glucose metabolism enzymes. We demonstrated that 3 days on a ketogenic diet before PET/CT studies significantly reduced cardiac uptake and did not alter tumor [18F]FDG uptake or cell viability, thus validating the proposed pre-imaging protocol as an effective alternative to fasting.
Lung histopathology revealed that our animal model simultaneously presented adenomas and adenocarcinomas at different stages. In addition, we observed a positive relationship between expression of GAPDH and uptake of [18F]FDG by tumor tissue.
It is well known that food deprivation improves the visualization of [18F]FDG-PET target areas, such as tumor tissue [29,30,31] and inflammatory/infectious responses [32, 33]. This is the standard protocol for the study of brain glucose metabolism [34, 35] in animal models. On the other hand, fasting is known to switch myocardial metabolism from glucose to free fatty acids (Randle cycle) [22, 36], thus reducing myocardial uptake of [18F]FDG.
Nowadays, several clinical studies [10, 12, 13, 37] and preclinical studies [9, 11] suggest that diets (low-carbohydrate or low-carbohydrate plus high-fat diets) are more effective than fasting for suppression of myocardial uptake. Myocardial suppression is particularly important in lung imaging [11, 20] and when diagnosing heart diseases [22]. In addition, it has been reported that diets do not reduce uptake in inflamed areas (osteoarthritis, tendinitis, dental inflammation) compared with fasting [10]. To our knowledge, ours is the first study to demonstrate that short high-fat diets do not alter tumor glucose metabolism, at least in the model we applied. Although in average tumor uptake difference did not reach statistical significance, this value is higher under ketogenic diet than under fasting in 9 out of 14 tumors (Fig. 2). We cannot rule out that a larger sample could render statistical significance. This possible increase in uptake could depend on a higher [18F]FDG availability because of the lower myocardial uptake.
In agreement with López-Ríos et al. [38], we found a positive relationship between expression of GAPDH and SUV. To our knowledge, our study is the first to explore this relationship in a murine lung cancer model. Several proteomic parameters (β-F1-ATPase, Hsp60, GAPDH, COX, IF1, and their combinations) comprising what is known as “the bioenergetics signature” [26, 39, 40] have been assessed in order to predict tumor progression, thus supporting the hypothesis of Warburg [41], who postulated that cancer cells undergo abnormally high aerobic glycolysis owing to impaired mitochondrial bioenergetics activity. Previous studies evaluated the relationship between the tumor bioenergetics signature and [18F]FDG uptake. For example, Huebbers et al. [26] demonstrated that [18F]FDG uptake correlates with high levels of β-F1-ATPase but found no association with Hsp60, GAPDH, or other proteins in patients with head and neck carcinoma. López-Ríos et al. [38] studied a cohort of lung carcinoma patients and found a linear association between SUV and expression of GAPDH and a negative association between SUV and β-F1-ATPase.
It is well known that hyperglycemia has an effect on uptake of [18F]FDG by tumor tissue [29, 42] and by brain [43]; consequently, patients are instructed to fast for at least 6 h before [18F]FDG-PET studies. In the present study, both dietary conditions decreased BGL (within the safety limit of 63–176 mg/dl for mice), as expected [9, 11].
Our study is limited by the heterogenic nature of the lung tumors, which hampers the interpretation of the results. Animal experiments were not randomized, diets were always tested following the same order (fasting and ketogenic diet). Although it is true that we cannot totally rule out any residual or carry-over effect between diets, as the optimal withdrawal period is unknown, FFA metabolism is reported to be suppressed when insulin levels increase after a regular meal [44]. Although dynamic PET studies might increase the robustness of [18F]FDG uptake by using kinetic models, we did not follow this approach, because dynamic acquisition requires the animals to be anesthetized during the uptake period, thus altering the distribution of [18F]FDG [45, 46]. No attempt was made to increase fasting time, because, according to our experience, this is a risk factor in our transgenic tumor animal model. Finally, the difference in body weight observed in our study may be attributable to fasting [47]. Another limitation of our longitudinal study is that we did not acquire a baseline [18F]FDG before starting dietary protocols. This was because we did not consider it advisable to perform so many studies on the same animals. Nevertheless, we included a diet-free control group to assess myocardial [18F]FDG uptake values and BGL.
In conclusion, our results show that 3 days on a high-fat diet prior to [18F]FDG-PET imaging did not change tumor [18F]FDG uptake compared with one night of fasting, although high-fat diets suppressed myocardial 18F-FDG uptake better than fasting. Our findings also demonstrate that [18F]FDG uptake is increased in tumors with higher GAPDH expression and that [18F]FDG uptake is more pronounced in adenocarcinomas than in adenomas. In summary, the ketogenic diet seems to be a potentially good alternative to fasting for [18F]FDG-PET studies in animal cancer models.
References
Bertagna F, Biasiotto G, Giubbini R (2013) The role of F-18-fluorothymidine PET in oncology. Clin Transl Imaging 1:77–97
Hoh CK (2007) Clinical use of FDG PET. Nucl Med Biol 34:737–742
Sathekge M, Maes A, Van de Wiele C (2013) FDG-PET imaging in HIV infection and tuberculosis. Semin Nucl Med 43:349–366
Fallahi B, Moasses-Ghafari B, Fard-Esfahani A et al (2017) Factors influencing the pattern and intensity of myocardial 18F-FDG uptake in oncologic PET-CT imaging. Iranian J Nucl Med 25:52–61
Maurer AH, Burshteyn M, Adler LP, Steiner RM (2011) How to differentiate benign versus malignant cardiac and paracardiac 18F FDG uptake at oncologic PET/CT. Radiographics 31:1287–1305
Lum DP, Wandell S, Ko J, Coel MN (2002) Reduction of myocardial 2-deoxy-2-[18F]fluoro-D-glucose uptake artifacts in positron emission tomography using dietary carbohydrate restriction. Mol Imaging Biol 4:232–237
Shreve PD, Anzai Y, Wahl RL (1999) Pitfalls in oncologic diagnosis with FDG PET imaging: physiologic and benign variants. Radiographics 19:61–77 quiz 150-151
Gaeta C, Fernandez Y, Pavia J et al (2011) Reduced myocardial 18F-FDG uptake after calcium channel blocker administration. Initial observation for a potential new method to improve plaque detection. Eur J Nucl Med Mol Imaging 38:2018–2024
Tupper T, Wang Y, McDonagh E, et al. (2011) Utilizing Ketogenic Diet as an Alternative to Fasting in Preclinical 18F-FDG PET. In World Mol Imaging Congress (WMIC): 2011 Program Book
Williams G, Kolodny GM (2008) Suppression of myocardial 18F-FDG uptake by preparing patients with a high-fat, low-carbohydrate diet. AJR Am J Roentgenol 190:W151–W156
Cussó L, Vaquero JJ, Bacharach S, Desco M (2014) Comparison of methods to reduce myocardial 18F-FDG uptake in mice: Calcium Channel blockers versus high-fat diets. PLoS One 9:e107999
Cheng VY, Slomka PJ, Ahlen M, Thomson LEJ, Waxman AD, Berman DS (2010) Impact of carbohydrate restriction with and without fatty acid loading on myocardial 18F-FDG uptake during PET: a randomized controlled trial. J Nucl Cardiol 17:286–291
Kobayashi Y, Kumita S-i, Fukushima Y et al (2013) Significant suppression of myocardial 18F-fluorodeoxyglucose uptake using 24-h carbohydrate restriction and a low-carbohydrate, high-fat diet. J Cardiol 62:314–319
Nebeling LC, Miraldi F, Shurin SB, Lerner E (1995) Effects of a ketogenic diet on tumor metabolism and nutritional status in pediatric oncology patients: two case reports. J Am Coll Nutr 14:202–208
Skinner R, Trujillo A, Ma XJ, Beierle EA (2009) Ketone bodies inhibit the viability of human neuroblastoma cells. J Pediatr Surg 44:212–216
Seyfried TN, Sanderson TM, El-Abbadi MM et al (2003) Role of glucose and ketone bodies in the metabolic control of experimental brain cancer. Br J Cancer 89:1375–1382
Abdelwahab MG, Fenton KE, Preul MC, Rho JM, Lynch A, Stafford P, Scheck AC (2012) The ketogenic diet is an effective adjuvant to radiation therapy for the treatment of malignant glioma. PLoS One 7:e36197
Caso J, Masko EM, Thomas JA et al (2013) The effect of carbohydrate restriction on prostate cancer tumor growth in a castrate mouse xenograft model. Prostate 73:449–454
Poff AM, Ari C, Seyfried TN, D’Agostino DP (2013) The ketogenic diet and hyperbaric oxygen therapy prolong survival in mice with systemic metastatic cancer. PLoS One 8:e65522
Ambrogio C, Cámara JA, Nieto P et al (2015) Analysis of murine lung tumors by micro PET-CT imaging. In Bio-protocol:e1692
Wykrzykowska J, Lehman S, Williams G, Parker JA, Palmer MR, Varkey S, Kolodny G, Laham R (2009) Imaging of inflamed and vulnerable plaque in coronary arteries with 18F-FDG PET/CT in patients with suppression of myocardial uptake using a low-carbohydrate, high-fat preparation. J Nucl Med 50:563–568
Bertagna F, Bisleri G, Motta F, Merli G, Cossalter E, Lucchini S, Biasiotto G, Bosio G, Terzi A, Muneretto C, Giubbini R (2012) Possible role of F18-FDG-PET/CT in the diagnosis of endocarditis: preliminary evidence from a review of the literature. Int J Cardiovasc Imaging 28:1417–1425
Abella M, Vaquero JJ, Sisniega A, Pascau J, Udías A, García V, Vidal I, Desco M (2012) Software architecture for multi-bed FDK-based reconstruction in X-ray CT scanners. Comput Meth Prog Bio 107:218–232
Wang Y, Seidel J, Tsui BMW, Vaquero JJ, Pomper MG (2006) Performance evaluation of the GE Healthcare eXplore VISTA dual-ring small-animal PET scanner. J Nucl Med 47:1891–1900
Woo S-K, Lee TS, Kim KM, Kim JY, Jung JH, Kang JH, Cheon GJ, Choi CW, Lim SM (2008) Anesthesia condition for 18F-FDG imaging of lung metastasis tumors using small animal PET. Nucl Med Biol 35:143–150
Huebbers CU, Adam AC, Preuss SF, Schiffer T, Schilder S, Guntinas-Lichius O, Schmidt M, Klussmann JP, Wiesner RJ (2015) High glucose uptake unexpectedly is accompanied by high levels of the mitochondrial β-F1-ATPase subunit in head and neck squamous cell carcinoma. Oncotarget 6:36172–36184
Guckenberger M, Rudofsky L, Andratschke N (2015) FDG-PET imaging for advanced radiotherapy treatment of non-small-cell lung Cancer. In: Hodler J, von Schulthess GK, Kubik-Huch RA, Zollikofer CL (eds) Diseases of the chest and heart 2015–2018. Springer Milan, pp 177–182
García-Beccaria M, Martínez P, Méndez-Pertuz M et al (2015) Therapeutic inhibition of TRF1 impairs the growth of p53-deficient K-Ras(G12V)-induced lung cancer by induction of telomeric DNA damage. EMBO Mol Med 7:930–949
Lindholm P, Minn H, Leskinen-Kallio S, Bergman J, Ruotsalainen U, Joensuu H (1993) Influence of the blood glucose concentration on FDG uptake in Cancer—a PET study. J Nucl Med 34:1–6
Rubello D, Gordien P, Morliere C et al (2015) Variability of hepatic 18F-FDG uptake at interim PET in patients with Hodgkin lymphoma. Clin Nucl Med 40:e405–e410
Wahl RL, Henry CA, Ethier SP (1992) Serum glucose: effects on tumor and normal tissue accumulation of 2-[F-18]-fluoro-2-deoxy-D-glucose in rodents with mammary carcinoma. Radiology 183:643–647
Hindryckx P, Staelens S, Devisscher L, Deleye S, de Vos F, Delrue L, Peeters H, Laukens D, de Vos M (2011) Longitudinal quantification of inflammation in the murine dextran sodium sulfate-induced colitis model using muPET/CT. Inflamm Bowel Dis 17:2058–2064
Kestler M, Muñoz P, Rodríguez-Créixems M et al (2014) Role of 18F-FDG PET in patients with infectious endocarditis. J Nucl Med 55:1093–1098
Ravasi L, Shimoji K, Soto-Montenegro ML, Esaki T, Seidel J, Sokoloff L, Schmidt K (2011) Use of [18F]fluorodeoxyglucose and the ATLAS small animal PET scanner to examine cerebral functional activation by whisker stimulation in unanesthetized rats. Nucl Med Commun 32:336–342
Thanos PK, Michaelides M, Gispert JD, Pascau J, Soto-Montenegro ML, Desco M, Wang R, Wang GJ, Volkow ND (2008) Differences in response to food stimuli in a rat model of obesity: in-vivo assessment of brain glucose metabolism. Int J Obes 32:1171–1179
Stanley WC, Recchia FA, Lopaschuk GD (2005) Myocardial substrate metabolism in the normal and failing heart. Physiol Rev 85:1093–1129
Harisankar C, Mittal B, Agrawal K, Abrar M et al (2011) Utility of high fat and low carbohydrate diet in suppressing myocardial FDG uptake. J Nucl Cardiol 18:926–936
López-Ríos F, Sánchez-Aragó M, García-García E, Ortega ÁD, Berrendero JR, Pozo-Rodríguez F, López-Encuentra Á, Ballestín C, Cuezva JM (2007) Loss of the mitochondrial bioenergetic capacity underlies the glucose avidity of carcinomas. Cancer Res 67:9013–9017
Cuezva JM, Chen G, Alonso AM, Isidoro A, Misek DE, Hanash SM, Beer DG (2004) The bioenergetic signature of lung adenocarcinomas is a molecular marker of cancer diagnosis and prognosis. Carcinogenesis 25:1157–1163
Sánchez-Aragó M, Formentini L, Cuezva JM (2013) Mitochondria-mediated energy adaption in Cancer: the H(+)-ATP synthase-geared switch of metabolism in human tumors. Antioxid Redox Signal 19:285–298
Warburg O (1956) On respiratory impairment in cancer cells. Science 124:269–270
Langen KJ, Braun U, Rota Kops E, Herzog H, Kuwert T, Nebeling B, Feinendegen LE (1993) The influence of plasma glucose levels on fluorine-18-fluorodeoxyglucose uptake in bronchial carcinomas. J Nucl Med 34:355–359
Wong KP, Sha W, Zhang X, Huang SC (2011) Effects of administration route, dietary condition, and blood glucose level on kinetics and uptake of 18F-FDG in mice. J Nucl Med 52:800–807
Stanley WC (2001) Changes in cardiac metabolism: a critical step from stable angina to ischaemic cardiomyopathy. Eur Heart J Suppl 3:O2–O7
Fueger BJ, Czernin J, Hildebrandt I, Tran C, Halpern BS, Stout D, Phelps ME, Weber WA (2006) Impact of animal handling on the results of 18FDG PET studies in mice. J Nucl Med 47:999–1006
Toyama H, Ichise M, Liow JS, Vines DC, Seneca NM, Modell KJ, Seidel J, Green MV, Innis RB (2004) Evaluation of anesthesia effects on [18F]FDG uptake in mouse brain and heart using small animal PET. Nucl Med Biol 31:251–256
Jensen T, Kiersgaard M, Sørensen D, Mikkelsen LF (2013) Fasting of mice: a review. Lab Anim 47:225–240
Acknowledgments
The authors thank Alexandra de Francisco and Yolanda Sierra for their excellent work with animal preparation and imaging protocols.
Funding
This work was partially supported by Comunidad de Madrid (S2017/BMD-3867 RENIM-CM) and cofinanciado con Fondos Estructurales de la Unión Europea. The CNIC is supported by the Ministerio de Ciencia, Innovación y Universidades and the Pro CNIC Foundation, and is a Severo Ochoa Center of Excellence (SEV-2015-0505).
Author information
Authors and Affiliations
Corresponding author
Ethics declarations
Ethics Statement
All animal procedures were approved by the Animal Experimentation Ethics Committee of Hospital General Universitario Gregorio Marañón (ES280790000087) and the Ethics Committees of CNIO and the Carlos III Health Institute, Madrid, and performed according to European regulations (2010/63/UE) and National regulations (RD 53/2013).
Conflict of Interest
The authors declare that they have no conflicts of interest.
Rights and permissions
About this article
Cite this article
Cussó, L., Musteanu, M., Mulero, F. et al. Effects of a Ketogenic Diet on [18F]FDG-PET Imaging in a Mouse Model of Lung Cancer. Mol Imaging Biol 21, 279–285 (2019). https://doi.org/10.1007/s11307-018-1233-8
Published:
Issue Date:
DOI: https://doi.org/10.1007/s11307-018-1233-8