Abstract
A clean, efficient and eco-friendly procedure has been developed using nano-copper chromite (nano-CuCr2O4) as catalyst for the synthesis of biscoumarin and dihydropyrano[c]chromene derivatives. This method provides several advantages such as mild reaction conditions, simple work-up procedure and is environment friendly. In addition, water is chosen as a green solvent.
Similar content being viewed by others
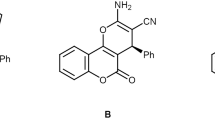
Explore related subjects
Discover the latest articles, news and stories from top researchers in related subjects.Avoid common mistakes on your manuscript.
Introduction
It is known that coumarin derivatives are very useful compounds. They have been widely used as medicine intermediates due to their useful biological and pharmacological properties, such as anticoagulant, spasmolytic, diuretic, and anticancer [1–4]. Biscoumarin can be employed as a urease inhibitor material [5]. Furthermore, dihydropyrano[3,2-c]chromenes and their derivatives are of considerable interest as they possess a wide range of biological properties, such as anti-Alzheimer’s disease, anti-AIDS-associated dementia and anti-schizophrenia [6]. Therefore, their synthesis has attracted much attention over the past decades. Numerous methods have been adapted to synthesize dihydropyrano[c]chromenes [6–14] and biscoumarin [15–19].
The use of water as a green solvent is emphasized in green chemical processes, because of the special properties of water, such as abundance, inexpensive, environmentally benign, hydrogen bonding in the transition state and high cohesive energy density, as well as negative activation volume. In addition, work-up and purification can be carried out by simple phase separation techniques [20]. For these reasons, the synthesis of heterocyclic compounds using multi-component protocols in water has been much investigated in recent years [21].
Mixed oxides with spinel structure (AB2O4) are important inorganic metalloid materials which are non-toxic, inexpensive, and very stable materials with strong resistance to acids and alkalis, while their nanoparticles possess relatively high surface areas. These properties make them suitable for use as solid heterogeneous catalysts for organic transformations [22]. One of them, copper chromite nanoparticles (CuCr2O4), has been used as a versatile catalyst in numerous reactions, for example, the oxyamination of benzene to aniline [23], the hydroxylation of benzene to phenol [24] and the synthesis of pyrazol derivatives [25].
In view of our ongoing efforts to explore newer reactions for the synthesis of heterocyclic systems [26–28], we would like to report here the one-pot synthesis of biscoumarin and 3,4-dihydropyrano[c]chromene derivatives by a three-component reaction in aqueous media.
Experimental
All reagents were purchased from Merck, Fluka and Aldrich with high-grade quality, and used without any purification. Copper chromite nanoparticles were successfully synthesized by coprecipitation according to the reported method [29].
X-ray diffraction (XRD) analyses of the samples were carried out with a Philips Xpert Pro using Cu Kα (λ = 1.5406 Å). Scanning electron microscopy (SEM) microstructure analyses were carried out with a Vega 435 Vpi linked to an energy dispersive X-ray microanalysis system.
Representative procedure for the synthesis of dihydropyrano[3,2-c]chromene derivatives
A solution of aromatic aldehyde (1 mmol), malononitrile (1.2 mmol), 4-hydroxycoumarin (1 mmol), and nano-CuCr2O4 (10 mol%) in 5.0 mL water was stirred at room temperature for the appropriate times (Table 1). Completion of the reaction was monitored by TLC. The solid was filtered off and washed with water (2 × 20 mL) and purified by recrystallization from ethanol. The product was dissolved in acetone and the catalyst was separated by filtration (Tables 2, 3). The physical data (mp, IR, NMR) of known compounds were found to be identical with those reported in the literature.
Representative procedure for the synthesis of α, α′-benzylidene bis(4-hydroxycoumarin) derivatives
A solution of aromatic aldehyde (1 mmol), 4-hydroxycoumarin (2 mmol), and nano-CuCr2O4 (10 mol%) in 5.0 mL water was stirred at room temperature for the appropriate times (Table 4). Completion of the reaction was monitored by TLC. The solid was filtered off and washed with water (2 × 20 mL) and purified by recrystallization from ethanol. The product was dissolved in acetone and the catalyst was separated by filtration.
The structures of all products were confirmed by IR, 1HNMR, 13CNMR, and elemental analysis.
Spectral data of products
2-Amino-4-phenyl-4, 5-dihydro-5-oxopyrano[3,2-c]chromene-3-carbonitrile ( 4a )
1H NMR (400 MHz, DMSO-d6): δ = 4.46 (1H, s, CH), 7.25 (2H, d, J = 8 Hz, ArH), 7.28 (1H, s, ArH), 7.33 (2H, t, J = 8 Hz, ArH), 7.43 (2H, s, NH2), 7.46–7.52 (2H, m, ArH), 7.72 (1H, t, J = 8 Hz, ArH), 7.91 (1H, d, J = 8 Hz, ArH) ppm. IR(KBr): 3380, 3291, 2196, 1705, 1671, 1599, 1527, 1374 cm−1.
2-Amino-4-(4-fluorophenyl)-4, 5-dihydro-5-oxopyrano[3,2-c]chromene-3-carbonitrile ( 4c )
1H NMR (400 MHz, DMSO-d6): δ = 4.49 (1H, s, CH), 7.14 (2H, t, J = 8 Hz, ArH), 7.33 (2H, t, J = 8 Hz, ArH), 7.44 (2H, s, NH2), 7.45 (1H, d, J = 8 Hz, ArH), 7.48 (1H, t, J = 8 Hz, ArH), 7.70 (1H, t, J = 8 Hz, ArH), 7.90 (1H, d, J = 8 Hz, ArH) ppm. IR(KBr): 3380, 3293, 2194, 1717, 1677, 1605, 1506, 1379 cm−1.
2-Amino-4-(4-nitrophenyl)-4, 5-dihydro-5-oxopyrano[3,2-c]chromene-3-carbonitrile ( 4g )
1H NMR (250 MHz, DMSO-d6): δ = 4.68 (1H, s, CH), 7.22–7.59 (5H, m, ArH), 7.62 (2H, s, NH2), 7.71–7.77 (1H, m, ArH), 7.90–7.93 (1H, m, ArH), 8.18 (2H, d, J = 8 Hz, ArH) ppm. IR(KBr): 3400, 3300, 2198, 1707, 1670, 1596, 1491, 1373 cm−1.
2-Amino-4-(4-cholorophenyl)-4, 5-dihydro-5-oxopyrano[3,2-c]chromene-3-carbonitrile ( 4h )
1H NMR (250 MHz, DMSO-d6): δ = 4.46 (1H, s, CH), 7.27–7.36 (4H, m, ArH), 7.44 (2H, s, NH2), 7.49 (2H, s, ArH), 7.70 (1H, t, J = 7.5 Hz, ArH), 7.88 (1H, d, J = 7.5 Hz, ArH) ppm. IR(KBr): 3390, 3300, 2199, 1710, 1670, 1603, 1512, 1371 cm−1.
2-Amino-4-(2, 3-dichlorophenyl)-4, 5-dihydro-5-oxopyrano[3,2-c]chromene-3-carbonitrile ( 4j )
1H NMR (400 MHz, DMSO-d6): δ = 5.09 (1H, s, CH), 7.28–7.35 (3H, m, ArH), 7.49 (1H, d, J = 8 Hz, ArH), 7.52 (2H, s, NH2), 7.55 (1H, d, J = 8 ArH), 7.74 (1H, t, J = 8 Hz, ArH), 7.92 (1H, d, J = 8 Hz, ArH) ppm. IR(KBr): 3380, 3291, 2196, 1705, 1671, 1599, 1527, 1374 cm−1.
3,3′-(4-Methoxybenzylidene)-bis-(4-hydroxycoumarin) ( 5c )
1H NMR (400 MHz, DMSO-d6) δ: 3.71 (3H, s, CH3O), 6.31 (1H, s, CH), 6.80–7.93 (12H, m, ArH), 8.16–8.78 (2H, m, OH) ppm. IR(KBr): 3443, 2926, 1668, 1606, 1563, 1510, 1452, 1352, 767 cm−1.
3,3′-(3-Nitrobenzylidene)-bis-(4-hydroxycoumarin) ( 5h )
1H NMR (400 MHz, DMSO-d6) δ: 6.39 (1H, s, CH), 7.28–8.04 (12H, m, ArH), 8.04–9.52 (2H, m, OH) ppm. IR(KBr): 3424, 2925, 1655, 1616, 1564, 1494, 1450, 1347, 762 cm−1.
3,3′-(4-Cholorobenzylidene)-bis-(4-hydroxycoumarin) ( 5i )
1H NMR (400 MHz, DMSO-d6) δ: 6.63 (1H, s, CH), 7.16–7.90 (12H, m, ArH), 7.90–9 (2H, m, OH) ppm. IR(KBr): 3420, 2923, 1668, 1606, 1563, 1490, 1451, 1351, 765 cm−1.
3,3′-(4-Choloro 3-nitrobenzylidene)-bis-(4-hydroxycoumarin) ( 5j )
1H NMR (400 MHz, DMSO-d6) δ: 6.28 (1H, s, CH), 7.26–7.85 (11H, m, ArH), 8.15–8.56 (2H, m, OH); 13C NMR (DMSO-d6) δ: 36.42, 103.23, 116.24, 119.31, 121.99, 123.81, 124.03, 124.51, 131.39, 132.07, 132.89, 143.87, 147.94, 152.90, 164.70, 167.34 ppm. IR(KBr): 3423, 2920, 1665, 1613, 1558, 1536, 1450, 1348, 765 cm−1. Anal. Calcd For C25H16ClNO8: C, 60.80; H, 3.27; N, 2.84; Found: C, 60.89; H, 3.25; N, 2.90.
Results and discussion
In the preliminary experiments, copper chromite nanoparticles were successfully synthesized by the coprecipitation method and were characterized by XRD analysis and SEM. X-ray diffraction patterns of the sample synthesized at Cu/Cr = 0.5 and pH values of 9 are presented in Fig. 1. The main peak is at 2θ = 35.4°, which is referred to the plane, and clearly indicates the formation of CuCr2O4. The results indicated that the samples have been composed of CuCr2O4 spinel and CuO phases. The wide diffraction peaks are indicative of a very fine particle size of the sample.
The samples were analyzed by X-ray dispersive analysis (EDX) and the results are shown in Fig. 2. It can be seen that all the samples exhibit assignments of Cu, Cr and O.
The SEM micrograph of the nanocatalyst is shown in Fig. 3. The micrograph of the sample exhibits particles of irregular shape and sizes of particles varying from 20 to 30 nm. Large aggregates of nanoparticles are clearly present in the sample, because of the very small size of the particles.
In the first instance, the reaction of 4-chlorobenzaldehyde, malononitrile and 4-hydroxycoumarin was explored using copper chromite (nano-CuCr2O4) as catalyst to afford the corresponding dihydropyranochromene product. The best results were obtained by carrying out the reaction at room temperature for 2 min with 10 mol% nano-copper chromite as a catalyst in aqueous media and a molar ratio of 4-chlorobenzaldehyde/malononitrile/4-hydroxycoumarin of 1:1.2:1.
In addition, we also studied the influence of the amount of nano-CuCr2O4 on the reaction yield. It was found that the nano-CuCr2O4 plays a crucial role in the success of the reaction in terms of the rate and the yields. For example, the reaction could be carried out in the absence of nano-CuCr2O4 in water at room temperature for 10 h, but it produced a very poor yield (Table 1, entry 1). The best catalytic activity of nano-CuCr2O4 was optimized to be 10 mol% (Table 1, entry 4). The excessive amounts of catalyst do not increase the yield significantly. Furthermore, to elucidate the role of the nanoparticle size effect, the CuCr2O4 nanoparticle catalyst was replaced by a CuCr2O4 catalyst with larger particle size at the optimum reaction conditions (Table 1, entry 2). In this experiment, the yield of the product was very modest even with a prolonged reaction time, indicating that the particle size has a considerable influence on the catalyst reactivity.
These results promoted us to investigate the scope and generality of this three-component protocol using various aldehydes under optimized conditions (Scheme 1). The results are summarized in Table 2. In all these cases, the corresponding dihydropyranochromene derivatives were obtained in good yields.
As shown in Table 2, we can find a series of aromatic aldehyde were reacted with malononitrile, and 4-hydroxycoumarin in the presence of nano-CuCr2O4 in water at room temperature, the reaction proceeded smoothly to afford the corresponding products 4 in good yields. All aromatic aldehydes containing electron-withdrawing groups (such as the nitro group, halides) or electron-donating groups (such as the alkyl group) were employed and reacted well to give the corresponding product 4 in good to excellent yields under these reaction conditions, so we conclude that no obvious effect of electrons or the nature of the substituents on the aromatic ring were observed. We also found that the reaction did not proceed in the case in which aliphatic aldehyde was used. The reason we think is that the activity of aliphatic aldehydes is less than that of aromatic aldehydes.
Some of the reported results for this reaction are summarized in Table 3 to compare with our results. The data indicated that the nano-CuCr2O4 is the most suitable for this reaction.
Under the same reaction conditions, nano-CuCr2O4 was also found to efficiently catalyze the one-pot condensation of 4-hydroxycoumarin and aromatic aldehydes in neat water at room temperature, high yields of products 5 were found (Scheme 2). The results are summarized in Table 4.
Benzaldehyde and other aromatic aldehydes containing electron-with drawing groups (such as the nitro group, halides) and 4-hydroxycoumarin were employed under this reaction condition to afford the corresponding products with good yields (84–98 %) in Table 4. The reactions of aromatic aldehydes containing electron-donating groups (such as the alkyl group) with 4-hydroxycoumarin can also give the corresponding products in good yields (84–88 %). The property of substituents on the aromatic ring did not show strongly obvious effects in terms of yields under this reaction condition.
Table 5 gives the details about the catalytic activity of some other reported protocols in the synthesis of α,α′-benzylidenebis(4-hydroxycoumarin) derivatives. Table 6 demonstrates that nano-CuCr2O4 can act as an effective catalyst in comparison with other catalysts.
It was apparent that this method can accelerate this reaction using nano-CuCr2O4 as a catalyst under solvent-free conditions in neat water at room temperature.
It is noteworthy that the catalyst is recyclable and could be reused without any significant loss of activity. In the case of the model reaction in water, after five runs, the catalytic activity of the catalyst was almost the same as that of the freshly used catalyst (Table 6).
Conclusion
In conclusion, nano-CuCr2O4 was found to be a novel alternative catalyst for the synthesis of benzylidene bis(4-hydroxycoumarin) and dihydropyrano[c]chromene derivatives in water at room temperature. The simple experimental procedure, environmentally friendly process, short reaction times, excellent yields and reusability of the catalyst make this procedure facile, practical and sustainable.
References
I. Kostava, I. Manolov, I. Nicolova, S. Konstantonov, M. Karaivanova, Eur. J. Med. Chem. 36, 339 (2001)
Z.H. Chohan, A.U. Shaikh, A. Rauf, C.T.J. Supuran, Enzym. Inhib. Med. Chem. 21, 741 (2006)
G.R. Green, J.M. Evans, A.K. Vong, A. in Comprehensive Heterocyclic Chemistry II, vol. 5, ed. By A.R. Katritzky, C.W. Rees, E.F.V. Scriven (Pergamon, Oxford, 1995), p. 469
L. Bonsignore, G. Loy, D. Secci, A. Calignano, Eur. J. Med. Chem. 28, 517 (1993)
K.M. Khan, S. Iqbal, M.A. Lodhi, G.M. Maharvi, Z. Ullah, M.I. Choudhary, A. Rahman, S. Perveen, Bioorg. Med. Chem. 12, 1963 (2004)
S. Abdolmohammadi, S. Balalaie, Tetrahedron Lett. 48, 3299 (2007)
M.M. Heravi, B.A. Jani, F. Derikvand, F.F. Bamoharram, H.A. Oskooie, Catal. Commun. 10, 272 (2008)
H. Mehrabi, H. Abusaidi, J. Iran. Chem. Soc. 7, 890 (2010)
H.R. Shaterian, M. Honarmand, Synth. Commun. 41, 3573 (2011)
G.M. Ziarani, A. Badiei, M. Azizi, P. Zarabadi, Iran. J. Chem. Eng. 30, 59 (2011)
N. Hazeri, M.T. Maghsoodlou, F. Mir, M. Kangani, H. Saravani, E. Molashahi, Chin. J. Catal. 35, 391 (2014)
M.E. Sedaghat, M.R. Booshehri, M.R. Nazarifar, F. Farhadi, Appl. Clay Sci. 95, 55 (2014)
M. Esmaeilpour, J. Javidi, F. Dehghani, F.N. Dodeji, RSC Adv. 5, 26625 (2015)
H. Kiyani, F. Ghorbani, Res. Chem. Intermed. 41, 4031 (2015)
G.-X. Gong, J.-F. Zhou, L.-T. An, X.-L. Duan, S.-J. Ji, Synth. Commun. 39, 497 (2009)
K. Tabatabaeian, H. Heidari, A. Khorshidi, M. Mamaghani, N.O. Mahmoodi, J. Serbian Chem. Soc. 76, 1 (2011)
N. Tavakoli-Hoseini, M.M. Heravi, F.F. Bamoharram, A. Davoodnia, M. Ghassemzadeh, J. Mol. Liq. 163, 122 (2011)
J.M. Khurana, S. Kumar, Monatsh. Chem. 141, 561 (2010)
R. Rezaei, M.R. Sheikhi, Res. Chem. Intermed. 41, 1283 (2015)
M.-O. Simon, C.-J. Li, Chem. Soc. Rev. 41, 1415 (2012)
A. Rahmati, N. Pashmforoush, J. Iran. Chem. Soc. 12, 993 (2015)
G. Fortunato, H.R. Oswald, A. Reller, J. Mater. Chem. 11, 905 (2001)
S.S. Acharyya, S. Ghosh, R. Bal, Chem. Commun. 50, 13311 (2014)
S.S. Acharyya, S. Ghosh, R. Bal, A.C.S. Appl, Mater. Interfaces 6, 14451 (2014)
J. Safaei-Ghomi, B. Khojastehbakht-Koopaei, S. Zahedi, Chem. Heterocycl. Compd. 51, 34 (2015)
Z. Karimi-Jaberi, A. Jaafarizadeh, Res. Chem. Intermed. 41, 4913 (2015)
Z. Karimi-Jaberi, B. Pooladian, Synth. Commun. 43, 1188 (2013)
Z. Karimi-Jaberi, M.R. Nazarifar, B. Pooladian, Chin. Chem. Lett. 23, 781 (2012)
M. Edrissi, S.A. Hosseini, M. Soleymani, Micro Nano Lett. 6, 836 (2011)
Author information
Authors and Affiliations
Corresponding author
Rights and permissions
About this article
Cite this article
Karimi-Jaberi, Z., Moaddeli, M.S., Setoodehkhah, M. et al. Nano-copper chromite (nano-CuCr2O4): a novel and efficient catalyst for the synthesis of biscoumarin and pyrano[c]chromene derivatives in water at room temperature. Res Chem Intermed 42, 4641–4650 (2016). https://doi.org/10.1007/s11164-015-2305-x
Received:
Accepted:
Published:
Issue Date:
DOI: https://doi.org/10.1007/s11164-015-2305-x