Abstract
Metal assembly to a dendrimer can provide various functionalities based on the branched structure. Here, we researched assembly phenomena of bismuth salts in the phenylazomethine dendrimer and achieved enhancement of emission intensity per metal unit by using Bi(OTf)3. This enhancement suggested increasing of Bi–N coordination bonds derived from the bismuth units in the dendrimer.
Similar content being viewed by others
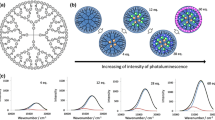
Explore related subjects
Discover the latest articles, news and stories from top researchers in related subjects.Avoid common mistakes on your manuscript.
Introduction
The precise arrangement of metals in organic polymers or macromolecules is an important objective considering natural materials, such as photosynthesis that control the direction and ratio of electron transfer. Various polymer-metal complexes have been reported, but the fine control of the nano-sized structure and the metal position is not available in conventional polymer-metal complexes due to the length distribution of the polymers (Ciardelli et al. 1995; Pittman et al. 1996).
Dendrimers, uniform three-dimensional branched polymers, are some of the most advantageous materials for fabricating controlled polymer-metal complexes (Tomalia et al. 1985; Newkome et al. 1985; Bosman et al. 1999; Grayson and Frechet 2001; Astruc and Chardac 2001). Various functional derivatives which contain metal complexes in the core and/or branches have been investigated (Newkome et al. 1995; Balzani et al. 1998). For example, introduction of metal ions or complexes in the core was utilized for organic light-emitting materials (Kawa and Frechet 1998; Hwang et al. 2008; Vicinelli et al. 2002; McClenaghan et al. 2003; Baudin et al. 2002). Catalytic and redox active metal complexes were also adopted for the functionalities (Bhyrappa et al. 1996; Knapen et al. 1994; Twyman et al. 2002; Nlate et al. 2000; Wang et al. 2014; Nijhuis et al. 2004; Suzuki et al. 2006). In addition, metal complexes at the periphery of the branches can be used for dendritic networks leading to the fabrication of macromolecules (Newkome et al. 1995, 1998).
The metal units of these metallodendrimers described above are incorporated into the skeletons. They have a merit of the high stability and can be novel building blocks for supramolecular structures. On the other hand, the assembly of the metal units into the dendrimer is another attractive strategy for metallodendrimers. Polyamidoamine (PAMAM) dendrimers can be metal complex dendrimers by the coordination of metal salts (Tomalia et al. 1985; 25 Esfand and Tomalia 2001; Lee et al. 2005; Tomalia et al. 2007; Lee et al. 2003). Such metal assembled dendrimers have been used as a nano-sized flask for the fabrication of size-controlled metal nanoparticles (Crooks et al. 2001; Zhao et al. 1998; Zhao and Crooks 1999). In this case, the shell-effect works for protection of the fabricated nanoparticles from the outer environment.
Among these dendrimers, we focused on dendritic polyphenylazomethines (DPAs) as a platform for metal assembly (Higuchi et al. 2001; Yamamoto et al. 2002; Takanashi et al. 2004; Enoki et al. 2006; Imaoka et al. 2013). The DPAs have imine-based coordination ligands in their branches. In addition, the π-conjugated skeleton produces an electronic potential gradient from the core to the periphery sites. It creates a different basicity of the imine sites and enables the stepwise controlled assembly of Lewis acidic units (e.g., metal salts, boron units, and organic molecules) (Yamamoto and Imaoka 2014; Yamamoto et al. 2005, 2009; Nakajima et al. 2004; Imaoka et al. 2005, 2006; Takanashi et al. 2007; Kambe et al. 2016a, b; Albrecht et al. 2013, 2016). The DPAs are also used for fabrication of metal particles and clusters (Yamamoto and Imaoka 2014; Yamamoto et al. 2009; Takahashi et al. 2017; Kambe et al. 2017; Inomata et al. 2018; Satoh et al. 2008).
Recently, we reported a luminous dendrimer based on the stepwise 1:1 complexation of BiCl3 into the 4th generation DPA (DPAG4). This bismuth-assembled dendrimer showed orange-colored luminescence (broad emission peak centered around 600 nm). The stepwise 1:1 assembly enabled the fine intensity tuning of the photoluminescence. In addition, the photoluminescence was maintained even in the solid state whereas the corresponding mononuclear complex was quenched under the same condition. These specific features demonstrated effective shell protection of the DPAG4. Such a shell effect was also utilized for optical switching based on the reversible complexation (Kambe et al. 2016a). In this study, we used bismuth salts different from BiCl3 considering the effect of the coordination mode and achieved an increasing emission intensity per the assembled metal unit using the complexation behavior of Bi(OTf)3 (OTf: trifluoromethanesulfonate). The OTf ligand has excellent leaving group properties (Dixon et al. 1990). Therefore, it dissociates from the bismuth atom in a solvent and enables increasing coordination bonds between the Bi(III) and phenylazomethine units. We demonstrated the utility of this multiple coordination behavior for decreasing of the amount of the metal units which generated photoluminescence through an assembly process.
Experimental section
Materials
Bi(OTf)3, (Strem Chemicals), BiCl3 ultra dry (Alfa Aesar), and bismuth 2-ethylhexanoate (Alfa Aesar) were purchased and used without any further purification. Dehydrated acetonitrile and triethylamine were obtained from Kanto Chemicals. Chloroform was obtained from Wako Pure Chemical Industries, Ltd. The DPAG4 was synthesized according to the literature (Enoki et al. 2006).
Characterization
The UV-vis spectra were recorded using Shimadzu UV-3600 and UV-3100PC spectrometer with a quartz cell having a 1-cm optical length at 20 °C. The photoluminescence spectra were obtained by a Jasco FP-8300 at room temperature.
Complexation between bismuth salts and DPAG4
In an Ar-filled glove box, an acetonitrile solution of Bi(OTf)3 (3.19 mM), or BiCl3 (2.21 mM), and an acetonitrile/chloroform (1/1) mixed solution of the DPAG4 (0.64 μM) were prepared. Then, the appropriate amount of the bismuth salt solution was added to the DPAG4 solution (3.0 mL) and the solution was vigorously stirred. The reactions were monitored by using UV-vis absorption and photoluminescence spectroscopy.
In the case of bismuth 2-ethylhexanoate, an acetonitrile solution of bismuth 2-ethylhexanoate (2.56 mM), and an acetonitrile/chloroform (1:1) mixed solution of the DPAG4 (0.47 μM) were used.
Removal of Bi(OTf)3 from the DPAG4
This experiment was conducted by reference to the method in the case of BiCl3 described in the previous literature (Kambe et al. 2016a). Trietylamine (1.0 μL) was added to the DPAG4 with Bi(OTf)3 solution (0.64 μM, 3.0 mL; acetonitrile/chloroform = 1/1).
Results and discussion
Assembly of bismuth salts
A certain amount of the acetonitrile solution of Bi(OTf)3 (3.19 mM) was stepwisely added to the mixed solution (acetonitrile/chloroform) of the DPAG4 (0.64 μM). The spectral change was monitored by UV-vis spectroscopy. The absorption band around 410 nm increased during the addition of the Bi(OTf)3 (from 1 to 20 equivalents). The peak shape and position were almost the same in the case of other metal units including BiCl3 (Yamamoto and Imaoka 2014; Kambe et al. 2016a), suggesting similar coordination interaction between metals and the DPAG4. On the other hand, the amount of the absorption change was different. This spectral change was saturated around 20 equivalents of Bi(OTf)3 whereas the DPAG4 possessed 60 phenylazomethine sites. After the saturation, the absorption peak shifted to the longer wavelength region when the Bi(OTf)3 was continuously added to the solution containing the DPAG4 with 20Bi(OTf)3 (Fig. S1). This spectral change is considered to be derived from decomposition of the azomethine parts in the DPAG4. These results suggest that the complexation between Bi(OTf)3 and the phenylazomethine ligands in the DPAG4 was multidentate, and the number of bismuth-coordinating phenylazomethine ligands per bismuth unit is greater than that in the case of BiCl3 which behave in a 1:1 coordinating fashion (Kambe et al. 2016a). In contrast, bismuth 2-ethylhexanoate that has carboxylate ligands was found not to be coordinated by the phenylazomethine ligands because the absorption spectra did not change. This means that the assembly feature could be changed by choice of the initial ligands on the bismuth atom (Fig. 1).
Schematic illustration of assembly process of bismuth units into the DPAG4. BiCl3 has a 1:1 coordination with the phenylazomethine part. On the other hand, bismuth trifluoromethanesulfonate shows increasing metal-imine complexes, while bismuth 2-ethylhexanoate does not react. Graphs show the UV-vis titration results. The complexation of Bi(OTf)3 demonstrated saturation of the spectral change at the point of 20 equivalent bismuth units (middle up). No spectral change was observed in the case of bismuth 2-ethylhexanoate (right up)
Photoluminescence properties
Assembly of Bi(OTf)3 into the DPAG4 induced photoluminescence, and the emission intensity increased with the addition of the Bi(OTf)3. The observed emission and excitation peaks were found to be similar to those of BiCl3 (Fig. 2a). This suggested that the photoluminescence properties were maintained versus the change in the coordinating ligands. However, the emission peak was shifted to the shorter wavelength region after 4 equivalents of Bi(OTf)3 (Fig. 2b). The reason is considered to be a multiple complexation among the dendrimers.
a Excitation (dotted line; λem = 600 nm) and emission (bold line; λex = 450 nm) spectra of 4Bi(OTf)3-DPAG4. The solvent is a 1:1 mixed solution of acetonitrile and chloroform. b Emission spectral change during the addition of Bi(OTf)3. Dotted and bold black lines correspond to the DPAG4 with 4 and 20 equivalents of Bi(OTf)3, respectively
Reversible complexation
The complexation between Bi(OTf)3 and the phenylazomethines is labile. Therefore, dissociation of the coordination bond using a base reagent is expected (Fig. 3a). Figure 3b and c shows the absorption and emission spectral change of the 20Bi(OTf)3-DPAG4 solution with the addition of triethylamine which is a stronger base, respectively. We observed that the increased photoluminescence and absorption by the complexation with Bi(OTf)3 was completely back to the initial state. The optical and chemical features that show the reversible complexation with photoluminescence are characteristic of the optical dendrimer based on the DPAG4.
Photoluminescence intensities
The use of Bi(OTf)3 units enabled enhancement of the photoluminescence per bismuth unit (Fig. 4a). Figure 4b shows the emission spectra of 4Bi(OTf)3-DPAG4 and 4BiCl3-DPAG4 at the same concentration (0.64 μM). The higher intensity was clearly observed in the case of Bi(OTf)3. Figure 4c shows the intensity change during the addition of the bismuth units. The intensity was normalized by the peak area from BiCl3-DPAG4. We found that the photoluminescence intensity was enhanced in the case of Bi(OTf)3 at the low equivalents of the bismuth units. This result showed successful cut-down of the metal units that trigger the optical emission from the dendrimer. Table 1 shows a comparison of the parameters corresponding to the photoluminescence intensity (F) and quantum yield (F/A) (F: area of the emission spectra, and A: absorption at the excitation wavelength). Though 4Bi(OTf)3-DPAG4 has high photoluminescence intensity, the F/A is smaller than that of 4BiCl3-DPAG4. These results suggest that the increasing absorption affects the enhancement of the photoluminescence.
a The complexation of Bi(OTf)3 can increase number of the emissive bismuth complexes. b Photoluminescence spectra of the DPAG4 with 4 equivalents of Bi(OTf)3 (blue bold line) and BiCl3 (black dotted line). The excitation wavelength is 450 nm. c Change in photoluminescence intensity during addition of the bismuth salts normalized by the peak area of BiCl3-DPAG4. The blue squares and black circles correspond to Bi(OTf)3 and BiCl3, respectively
Conclusions
In conclusion, we revealed the coordination reactions of various kinds of bismuth units to the DPAG4 and achieved successful enhancement of the photoluminescence from the bismuth-coordinating dendrimers by utilizing the coordination fashion of Bi(OTf)3. This complexation was revealed by the UV-vis absorption and photoluminescence spectra including the monitoring of the bond formation and dissociation reaction, suggesting multiple coordination bonds. We also revealed that this photoluminescence enhancement was affected by the increasing absorption from the metal-imine complexes in the DPAG4. These results demonstrate a certain application of this coordination behavior for the enhancement of the complex properties in the dendrimer.
References
Albrecht K, Kasai Y, Kuramoto Y, Yamamoto K (2013) A fourth-generation carbazole–phenylazomethine dendrimer as a size-selective host for fullerenes. Chem Commun 49:865–867. https://doi.org/10.1039/c2cc36451d
Albrecht K, Hirabayashi Y, Otake M, Mendori S, Tobari Y, Azuma Y, Majima Y, Yamamoto K (2016) Polymerization of a divalent/tetravalent metal-storing atom-mimicking dendrimer. Sci Adv 2:e1601414. https://doi.org/10.1126/sciadv.1601414
Astruc D, Chardac F (2001) Dendritic catalysts and dendrimers in catalysis. Chem Rev 101:2991–3024. https://doi.org/10.1021/cr010323t
Balzani V, Campagna S, Denti G, Juris A, Serroni S, Venturi M (1998) Designing dendrimers based on transition-metal complexes. Light-harvesting properties and predetermined redox patterns. Acc Chem Res 31:26–34. https://doi.org/10.1021/ar950202d
Baudin HB, Davidsson J, Serroni S, Juris A, Balzani V, Campagna S, Hammarström L (2002) Ultrafast energy transfer in binuclear ruthenium−osmium complexes as models for light-harvesting antennas. J Phys Chem A 106:4312–4319. https://doi.org/10.1021/jp012770i
Bhyrappa P, Young JK, Moore JS, Suslick KS (1996) Dendrimer-metalloporphyrins: synthesis and catalysis. J Am Chem Soc 118:5708–5711. https://doi.org/10.1021/ja953474k
Bosman AW, Janssen HM, Meijer EW (1999) About dendrimers: structure, physical properties, and applications. Chem Rev 99:1665–1688. https://doi.org/10.1021/cr970069y
Ciardelli F, Tsuchida E, Worle D (eds) (1995) Macromolecular metal complexes. Springer-Verlag, Berlin
Crooks RM, Zhao M, Sun L, Chechik V, Yeung LK (2001) Dendrimer-encapsulated metal nanoparticles: synthesis, characterization, and applications to catalysis. Acc Chem Res 34:181–190. https://doi.org/10.1021/ar000110a
Dixon NE, Lawrance GA, Lay PA, Sargeson AM, Taube H (1990) Trifluoromethanesulfonates and trifluoromethanesulfonato-O complexes. Inorg Synth 28:70–76. https://doi.org/10.1002/9780470132593.ch16
Enoki O, Katoh H, Yamamoto K (2006) Synthesis and properties of a novel phenylazomethine dendrimer with a tetraphenylmethane core. Org Lett 5:569–571. https://doi.org/10.1021/ol052673y
Esfand R, Tomalia DA (2001) Poly(amidoamine) (PAMAM) dendrimers: from biomimicry to drug delivery and biomedical applications. Drug Discov Today 6:427–436. https://doi.org/10.1016/S1359-6446(01)01757-3
Grayson SM, Frechet JMJ (2001) Convergent dendrons and dendrimers: from synthesis to applications. Chem Rev 101:3819–3868. https://doi.org/10.1021/cr990116h
Higuchi M, Shiki S, Ariga K, Yamamoto K (2001) First synthesis of phenylazomethine dendrimer ligands and structural studies. J Am Chem Soc 123:4414–4420. https://doi.org/10.1021/ja004239r
Hwang SH, Moorefield CN, Newkome GR (2008) Dendritic macromolecules for organic light-emitting diodes. Chem Soc Rev 37:2543–2557. https://doi.org/10.1039/b803932c
Imaoka T, Tanaka R, Arimoto S, Sakai M, Fujii M, Yamamoto K (2005) Probing stepwise complexation in phenylazomethine dendrimers by a metallo-porphyrin core. J Am Chem Soc 127:13896–13905. https://doi.org/10.1021/ja0524797
Imaoka T, Tanaka R, Yamamoto K (2006) Investigation of a molecular morphology effect on polyphenylazomethine dendrimers; physical properties and metal-assembling processes. Chem Eur J 12:7328–7336. https://doi.org/10.1002/chem.200600511
Imaoka T, Kawana Y, Kurokawa T, Yamamoto K (2013) Macromolecular semi-rigid nanocavities for cooperative recognition of specific large molecular shapes. Nat Commun 4:2581. https://doi.org/10.1038/ncomms3581
Inomata Y, Albrecht K, Yamamoto K (2018) Size dependent oxidation state and CO oxidation activity of tin oxide clusters. ACS Catal 8:451–456. https://doi.org/10.1021/acscatal.7b02981
Kambe T, Imaoka T, Yamamoto K (2016a) Reducing capsule based on electron programming: versatile synthesizer for size-controlled ultra-small metal clusters. Chem Eur J 22:16406–16409. https://doi.org/10.1002/chem.201603229
Kambe T, Watanabe A, Imaoka T, Yamamoto K (2016b) Bismuth complexes in phenylazomethine dendrimers: controllable luminescence and emission in the solid state. Angew Chem Int Ed 55:13151–13154. https://doi.org/10.1002/anie.201607396
Kambe T, Haruta N, Imaoka T, Yamamoto K (2017) Solution-phase synthesis of Al13 − using a dendrimer template. Nat Commun 8:2046. https://doi.org/10.1038/s41467-017-02250-4
Kawa M, Frechet JMJ (1998) Self-assembled lanthanide-cored dendrimer complexes: enhancement of the luminescence properties of lanthanide ions through site-isolation and antenna effects. Chem Mater 10:286–296. https://doi.org/10.1021/cm970441q
Knapen JWJ, van der Made AW, de Wilde JC, van Leeuwen PWNM, Wijkens P, Grove DM, van Koten G (1994) Homogeneous catalysts based on silane dendrimers functionalized with arylnickel(II) complexes. Nature 372:659–663. https://doi.org/10.1038/372659a0
Lee JH, Lim YB, Choi JS, Lee Y, Kim TI, Kim HJ, Yoon JK, Kim K, Park JS (2003) Polyplexes assembled with internally quaternized PAMAM-OH dendrimer and plasmid DNA have a neutral surface and gene delivery potency. Bioconjug Chem 14:1214–1221. https://doi.org/10.1021/bc034095g
Lee CC, MacKay JA, Fréchet JMJ, Szoka FC (2005) Designing dendrimers for biological applications. Nat Biotechnol 23:1517–1526. https://doi.org/10.1038/nbt1171
McClenaghan ND et al (2003) Ruthenium(II) dendrimers containing carbazole-based chromophores as branches. J Am Chem Soc 125:5356–5365. https://doi.org/10.1021/ja021373y
Nakajima R, Tsuruta M, Higuchi M, Yamamoto K (2004) Fine control of the release and encapsulation of Fe ions in dendrimers through ferritin-like redox switching. J Am Chem Soc 126:1630–1631. https://doi.org/10.1021/ja037480p
Newkome GR, Yao ZQ, Baker GR, Gupta VK (1985) Cascade molecules: a new approach to micelles. A [27]-arborol. J Org Chem 50:2003–2004. https://doi.org/10.1021/jo00211a052
Newkome GR, Guther R, Moorefield CN, Cardullo F, Echegoyen L, Perez-Cordero E, Luftmann H (1995) Routes to dendritic networks: Bis-dendrimers by coupling of cascade macromolecules through metal centers. Angew Chem Int Ed 34:2023–2026. https://doi.org/10.1002/anie.199520231
Newkome GR, He E, Godinez LA (1998) Construction of dendritic assemblies: a tailored approach to isomeric metallomacromolecules by means of Bis(2,2′:6′,2″-terpyridine)ruthenium(II) connectivity. Macromolecules 31:4382–4386. https://doi.org/10.1021/ma980280r
Nijhuis CA, Huskens J, Reinhoudt DN (2004) Binding control and stoichiometry of ferrocenyl dendrimers at a molecular printboard. J Am Chem Soc 126:12266–12267. https://doi.org/10.1021/ja048271n
Nlate S, Ruiz J, Blais JC, Astruc D (2000) Ferrocenylsilylation of dendrons: a fast convergent route to redox-stable ferrocene dendrimers. Chem Commun 417–418. https://doi.org/10.1039/a908791e
Pittman CU, Cullberston BM, Sheet JE (eds) (1996) Metal containing polymeric materials. ACS Symposium Series; Plenum, New York
Satoh N, Nakashima T, Kamikura K, Yamamoto K (2008) Quantum size effect in TiO2 nanoparticles prepared by finely controlled metal assembly on dendrimer templates. Nature Nanotechnol 3:106–111. http://www.nature.com/nnano/journal/v3/n2/abs/nnano.2008.2.html
Suzuki M, Nakajima R, Tsuruta M, Higuchi M, Einaga Y, Yamamoto K (2006) Synthesis of ferrocene-modified phenylazomethine dendrimers possessing redox switching. Macromolecules 39:64–69. https://doi.org/10.1021/ma052268m
Takahashi M, Koizumi H, Chun WJ, Kori M, Imaoka T, Yamamoto K (2017) Finely controlled multimetallic nanocluster catalysts for solvent-free aerobic oxidation of hydrocarbons. Sci Adv 3:e1700101. https://doi.org/10.1126/sciadv.1700101
Takanashi K, Chiba H, Higuchi M, Yamamoto K (2004) Efficient synthesis of poly(phenylazomethine) dendrons allowing access to higher generation dendrimers. Org Lett 6:1709–1712. https://doi.org/10.1021/ol049656d
Takanashi K, Fujii A, Nakajima R, Chiba H, Higuchi M, Einaga Y, Yamamoto K (2007) Heterometal assembly in dendritic polyphenylazomethines. Bull Chem Soc Jpn 80:1563–1572. https://doi.org/10.1246/bcsj.80.1563
Tomalia DA, Baker H, Dewald J, Hall M, Kallos G, Martin S, Roeck J, Ryder J, Smith P (1985) A new class of polymers: starburst-dendritic macromolecules. Polym J 17:117–132. https://doi.org/10.1295/polymj.17.117
Tomalia DA, Reyna LA, Svenson S (2007) Dendrimers as multi-purpose nanodevices for oncology drug delivery and diagnostic imaging. Biochem Soc Trans 35:61–67. https://doi.org/10.1042/bst0350061
Twyman LJ, King ASH, Martin IK (2002) Catalysis inside dendrimers. Chem Soc Rev 31:69–82. https://doi.org/10.1039/b107812g
Vicinelli V, Ceroni P, Maestri M, Balzani V, Gorka M, Vögtle F (2002) Luminescent lanthanide ions hosted in a fluorescent polylysin dendrimer. Antenna-like sensitization of visible and near-infrared emission. J Am Chem Soc 124:6461–6468. https://doi.org/10.1021/ja017672p
Wang Y, Salmon L, Ruiz J, Astruc D (2014) Metallodendrimers in three oxidation states with electronically interacting metals and stabilization of size-selected gold nanoparticles. Nat Commun 5:3489. https://doi.org/10.1038/ncomms4489
Yamamoto K, Imaoka T (2014) Precision synthesis of subnanoparticles using dendrimers as a superatom synthesizer. Acc Chem Res 47:1127–1136. https://doi.org/10.1021/ar400257s
Yamamoto K, Higuchi M, Shiki S, Tsuruta M, Chiba H (2002) Stepwise radial complexation of imine groups in phenylazomethine dendrimers. Nature 415:509–511. https://doi.org/10.1038/415509a
Yamamoto K, Higuchi M, Kimoto A, Imaoka T, Masachika K (2005) Novel functional groups with fine-controlled metal assembling function. Bull Chem Soc Jpn 78:349–355. https://doi.org/10.1246/bcsj.78.349
Yamamoto K, Imaoka T, Chun WJ, Enoki O, Katoh H, Takenaga M, Sonoi A (2009) Size-specific catalytic activity of platinum clusters enhances oxygen reduction reactions. Nat Chem 1:397–402. https://doi.org/10.1038/nchem.288
Zhao M, Crooks RM (1999) Dendrimer-encapsulated Pt nanoparticles: synthesis, characterization, and applications to catalysis. Adv Mater 11:217–220. https://doi.org/10.1002/(SICI)1521-4095(199903)11:3<217::AID-ADMA217>3.0.CO;2-7
Zhao M, Sun L, Crooks RM (1998) Preparation of Cu nanoclusters within dendrimer templates. J Am Chem Soc 120:4877–4878. https://doi.org/10.1021/ja980438n
Acknowledgments
We thank Suzukakedai Materials Analysis Division, Technical Department, Tokyo Institute of Technology, and NIMS microstructural characterization platform as a program of “Nanotechnology Platform” and advanced characterization nanotechnology platform of the University of Tokyo supported by the “Nanotechnology Platform” of the Ministry of Education, Culture, Sports, Science and Technology (MEXT), Japan.
Funding
This study was supported by JSPS KAKENHI Grant Numbers 17K05804 and 15H05757, the ERATO program of the Japan Science and Technology (JST) Agency (JPMJER1503), and the cooperative research program of the Network Joint Research Center for Materials and Devices of Japan.
Author information
Authors and Affiliations
Contributions
The manuscript was written through the contributions of all authors. All authors have given approval to the final version of the manuscript.
Corresponding author
Ethics declarations
Conflict of interest
The authors declare that they have no conflict of interest.
Additional information
This article is part of the topical collection: Unifying Concepts for Nanoscience and Nanosystems: 20th Anniversary Issue
Donald Tomalia, Paolo Milani and Kenneth Dawson, co-editors
Electronic supplementary material
ESM 1
(PDF 101 kb)
Rights and permissions
About this article
Cite this article
Kambe, T., Imaoka, S., Imaoka, T. et al. Build-up enhancement of photoluminescence from phenylazomethine bismuth dendrimer using Bi(OTf)3. J Nanopart Res 20, 118 (2018). https://doi.org/10.1007/s11051-018-4222-6
Received:
Accepted:
Published:
DOI: https://doi.org/10.1007/s11051-018-4222-6