Abstract
Unregulated rivers provide unobstructed corridors for the dispersal of both native and invasive species. We sought to evaluate range size and habitat use of an invasive species (Silver Carp, Hypophthalmichthys molitrix) in an unimpounded river reach (Wabash River, IN), to provide insights into the dispersal of invasive species and their potential overlap with native species. We hypothesized that range size would increase with fish length, be similar among sexes, and vary annually while habitats used would be deeper, warmer, lower velocity, and of finer substrate. Silver Carp habitat use supported our hypotheses but range size did not vary with sex or length. 75% home range varied annually, suggesting that core areas occupied by individuals may change relative to climate-based factors (e.g., water levels), whereas broader estimates of range size remained constant across years. Ranges were often centered on landscape features such as tributaries and backwaters. Results of this study indicate habitat and landscape features as potential areas where Silver Carp impacts on native ecosystems may be the greatest. Observed distribution of range sizes indicates the presence of sedentary and mobile individuals within the population. Mobile individuals may be of particular importance as they drive the spread of the invasive species into new habitats.
Similar content being viewed by others
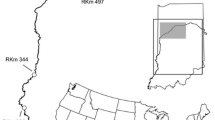
Avoid common mistakes on your manuscript.
Introduction
Silver Carp (Hypophthalmichthys molitrix [Valenciennes, 1844]) are global invaders, occurring in >70 countries and territories (Kolar et al., 2007). Multiple characteristics have contributed to the invasion success of Silver Carp, including plasticity in life history traits, rapid growth, high fecundity, and rapid dispersal (Kolar et al., 2007; DeGrandchamp et al., 2008; Coulter et al., 2013). The North American invasion of this fish provides an excellent context for ecological study of fish beyond their native distributions because they have invaded several rivers of divergent hydrogeomorphology. Most North American rivers with high densities of Silver Carp are impounded, with lock-and-dam systems interspersed with pool habitats (Chick & Pegg, 2001; MacNamara et al., 2016). However, Silver Carp are also found in unimpounded rivers and river reaches in North America, including many in the Great Lakes, upper Mississippi River, and Ohio River Basins which are at risk of future invasion (USACE, 2010; Cudmore et al., 2012). Environmental conditions differ between impounded and unimpounded river reaches, primarily with respect to flow regime and slack-water habitat availability. Unimpounded river reaches function as corridors for dispersal and could provide better conditions for Silver Carp spawning due to a more natural flow regime with spikes in discharge to trigger spawning, fewer zones for drifting eggs and larvae to settle (Abdusamadov, 1987; Kocovsky et al., 2012), and no dams to inhibit movement to spawning sites. Biological and ecological traits of Silver Carp have been observed to vary across ecosystems and among populations (e.g., Deters et al., 2013; Coulter et al., 2013; Jayasinghe et al., 2015), and it is likely that these traits also diverge between invaded unimpounded and impounded river reaches (e.g., Stuck et al., 2015).
Among the suite of characteristics that can influence invasion success, movement and habitat use are of particular interest because movement can contribute to increased propagule pressure that facilitates invasions (Lockwood et al., 2005), and habitat use can provide targets for monitoring to detect new invasions and controlling established populations (Peterson, 2003; Guisan & Thuiller, 2005). One metric that can be influenced by both movement and habitat use is range size. Range size is a concept in biogeography and animal ecology that expresses the spatial extent over which an individual occurs (Burt, 1943; Gerking, 1950, 1953, 1959). Range sizes in fishes can be influenced by habitat selection for a variety of factors, including flow rate, water temperature (Linfield, 1985; Winemiller & Jepsen, 1998), water depth (Winemiller & Jepsen, 1998), food resource availability (Tyler & Gilliam, 1995), ontogenetic changes, and life history events (Crook, 2004a). In some species, individuals with greater dispersal distances may also occupy larger range sizes (Lester et al., 2007). Therefore, range measures may vary with changes in ontogenetic traits (e.g., fish length and maturation) and the occurrence of specific life history events (e.g., spawning) as well as habitat availability. Mature Silver Carp within their native distributions live primarily in lentic or lentic-like habitats and move to very specific lotic sites for spawning (Chen et al., 2007). As a result, ranges within the species’ native distributions should include both lentic residential and lotic spawning sites. However, invasive Silver Carp in North America inhabit primarily lotic habitats, and observations suggest that spawning site selection may be less specific or have different requirements (Schrank et al., 2001; Coulter et al., 2013; Deters et al., 2013; Coulter et al., 2016b) that could produce differences in observed ranges and habitat use.
Individual variation in movements and range is often an overlooked component of fish behavior (Magurran, 1986) but can have a great impact on dispersal patterns exhibited by a population. Dispersal patterns are also under great selection pressure (Baguette et al., 2013) and may differ among and within populations (Travis & Dytham, 2002; Wright et al., 2003; Hughes et al., 2007; Phillips & Dudík, 2008). Despite this variation, some generalities in dispersal have emerged. For some species, individuals may be classified as mobile or sedentary (Funk, 1955; Kennedy, 1981; Aparicio & De Sostoa, 1999; Skalski & Gilliam, 2000) and the classification of an individual may change through time. Mobile individuals may function to reduce inbreeding and connect populations (Bengsston, 1978; Waldman & McKinnon, 1993; Jackson et al., 2001) and these individuals may be more fit than sedentary counterparts (Fraser et al., 2001). However, cyprinids, especially large cyprinids, are underrepresented in the movement literature as much movement theory is derived from studies of salmonids (e.g., Gowan et al., 1994; Rodríguez, 2002; but see Goforth & Foltz, 1999; Crook, 2004a). Thus, it is difficult to hypothesize dispersal patterns for large cyprinids, including Silver Carp, without additional research.
Habitat use differences in Silver Carp are likely based on availability within invaded riverine ecosystems and individual life stage. For example, observations from regulated rivers indicate that lentic-like habitats, such as backwaters and other lower velocity areas, are preferred habitats for Silver Carp (Kolar et al., 2007; DeGrandchamp et al., 2008; Calkins et al., 2012). However, such habitats are not readily available in all rivers supporting established Silver Carp populations (e.g., Wabash River). Thus, there is great need to examine range size and habitat use of Silver Carp in unregulated rivers to support management efforts while also offering insights into the ecology and behavior of Silver Carp.
The goal of this study was to examine range size and habitat use of invasive Silver Carp in an invaded, unimpounded river reach which can be used to refine predictive models (e.g., range expansion and invasion success), evaluate habitat overlap with native species, target areas for removal efforts, and provide general insights into invasion ecology and invasive species management. Using four different estimates of range size, we examined potential variation in range size among individuals, between sexes, in relation to total length, and among years. Habitat selection by Silver Carp was also evaluated based on river substrate, water depth, velocity, and temperature. We hypothesized that Silver Carp range size estimates would (1) increase with increasing length as larger fish often have greater resource requirements (Hobbs et al., 2005; Downs & Horner, 2007), (2) be similar between sexes since movements were previously found to be similar (Coulter et al., 2016a), and (3) range size would vary annually as yearly differences in water levels restrict or allow access to different habitats. We also hypothesized that Silver Carp would utilize deeper, lower velocity, and warmer habitats that are more lentic-like (Chen et al., 2007; Kolar et al., 2007; DeGrandchamp et al., 2008; Calkins et al., 2012) and that Silver Carp would prefer small particle substrates that are associated with lower velocity habitats.
Materials and methods
Range sizes and habitat use were calculated using existing telemetry infrastructure and data (Coulter et al., 2016a) in the Wabash River, Indiana (USA), collected from 2011 to 2013. The data were collected from 232 Silver Carp tagged with acoustic telemetry tags (Vemco V16-4H, Bedford, Nova Scotia, Canada), 17 stationary Vemco VR2W receivers deployed over an approximately 400 km reach of the Wabash River, and active tracking conducted using a Vemco VR100 receiver during the same period over an approximately 100-km portion (Fig. 1). Tagged individuals were released following recovery of equilibrium within 2 km of their capture location (full surgery details in Coulter et al., 2016a). Active tracking was performed once a week, and a single telemetry detection was used for each individual on each day from either active tracking or the stationary receivers to reduce potential autocorrelation (e.g., Fieberg, 2007). If multiple detections of the same individual occurred within the same day, the last detection of the day was used. Additionally, no detections collected within 48 h of tag implantation were used for range estimation. During tagging in 2012 and 2013, fin clips were taken from tagged individuals, and DNA extracted from the clips was used to classify individuals as Silver Carp, Bighead Carp [Hypophthalmichthys nobilis (J. Richardson, 1845)], or hybrids based on three established primers (Mia et al., 2005; Coulter et al., 2016a) using polymerase chain reaction (PCR) as detailed in Lenaerts et al. (2015). No fin clips were taken in 2011, so external morphology (keel, gill rakers, head size, coloration) was used to classify individuals as Silver Carp, Bighead Carp, or hybrids for that year. Results from genetic analysis indicated a 10% chance of misclassification of these fishes based on external morphology, with the majority of these misclassifications coming from hybrids misclassified as Silver Carp. Only data from individuals visually (2011, n = 84) or genetically (2012, n = 40; 2013, n = 108) determined to be Silver Carp were included in this study. Sex was determined during tagging if eggs or milt were visible during surgery or could be expressed with light pressure (males, n = 12, females, n = 77). Total length of tagged Silver Carp averaged 698.5 mm (±4.30 SE, range 374–940 mm, n = 232).
Area of the Wabash River, Indiana, where the Silver Carp telemetry study took place from 2011 to 2013. Active tracking occurred over approximately 100 river kilometers (rkm). The locations of stationary receivers (triangles) and the stream gaging station (star, USGS streamflow gaging station number 03336000) are indicated on the map. Acoustic Doppler current profiler (ADCP) was used over approximately 13 rkm in 2012 and 2013 to measure water velocity, depth, and temperature. Substrate data were mapped in 2014 over 10 rkm
Estimation of range sizes
Ranges encompass the habitats and resources needed to complete life-history activities over a time period of interest. Overall total range was calculated as the maximum displacement (i.e., the linear river distance between the most upstream and downstream telemetry detections) of an individual over the entire study (similar to Clapp et al., 1990). Overall total range values take into account the maximum extent to which an individual Silver Carp may move to better incorporate the influence of large spawning migrations these individuals may exhibit (e.g., DeGrandchamp et al., 2008; Coulter et al., 2016a). Ranges are linear, streamwise distances that do not take into account lateral movements across the width of the river in part due to the linear placement of stationary receivers. Detections from the telemetry study were imported into ArcMap (V. 10.2, ESRI, Redlands, CA, USA), separated by individual fish, and overlaid with the flowline for the Wabash River. Overall total range was only calculated for each individual with >5 detections over the 3-year study period. Individuals with <5 detections were often detected moving downstream and never moved upstream or only had one or two detections shortly after tagging. These detection histories indicate possible mortalities, and thus ranges from these individuals were not included in analyses. Because individuals were tagged and released in multiple years, overall total ranges were derived from telemetry data collected over different time periods, which could influence range sizes. Therefore, overall total ranges were first compared among individuals with overall total ranges derived from 1, 2, or 3 year of data using a Kruskal–Wallis test so that range estimates could be combined when possible. If significant differences existed, pairwise comparisons were done with Mann–Whitney U tests (Bonferroni corrected α = 0.025 used in with Mann–Whitney tests). Additionally, numbers of detections could potentially influence total range estimates and a Spearman rank correlation was used to test for any relationships.
Additional comparisons were made using Silver Carp overall total ranges estimated from 2 or 3 years of data that were shown to not be significantly different from each other. A frequency distribution of Silver Carp overall total range size was created to visualize distributions of range size. A Spearman rank correlation was used to determine if total length of the Silver Carp was associated with a change in total range size. Also, Silver Carp male and female overall total ranges were compared using a Mann–Whitney U test.
Some individuals tagged in the initial year of the study had >5 detections from each study year (2011, 2012, and 2013), which allowed for comparison of how an individual’s range, and the mean river kilometer (rkm) of each range varied through time. Annual total range was calculated as maximum displacement during a particular year, while annual home ranges (both 95 and 75% isopleths) were calculated using the kernel density estimator tool in ArcMap. Using kernel density to estimate a home range considers the frequency of use of different habitats, thereby giving less weight to short migrations and providing an estimate of the area individuals used most of the time. The kernels were generated and then river lengths contained within those kernels were considered the annual home range size. Mean rkm of annual total range and home ranges were determined based on rkm listed on maps in Hoggatt (1975). All range size estimates and mean rkm were not normally distributed and multiple transformations did not achieve normality. Therefore, non-parametric tests were employed for all statistics related to range size. The effect of numbers of detections on annual total range and home range estimates was tested using Spearman rank correlations. Friedman rank sum tests (non-parametric repeated measures analysis of variance equivalent) were used to evaluate whether the annual total range and home range sizes and mean rkm varied for individual Silver Carp among years followed by Wilcoxon–Nemenyi–McDonald–Thompson post hoc tests (Hollander & Wolfe, 1999) if significant. The study period encompassed a drought year (2012), and wet year (2013), and a more typical year (2011) which could contribute to differences in range sizes.
Substrate
Substrate data were obtained using a Humminbird 898c SI side-scan sonar (Johnson Outdoors Marine Electronics, Eufaula, AL, USA) for a 10-km reach of the Wabash River near Lafayette, IN, in 2014. Four passes were made through the 10-km reach to thoroughly cover the entire river bottom. Raw sonar data were converted to raster files usable in a GIS with DrDepth 5.0.20 software (DrDepthPC Version 5.0.20 by Per Pelin, 2012). GIS layers of sonar data were characterized into five substrate categories: sand, gravel, cobble, boulder, and bedrock. Substrates were visually interpreted based on texture, bottom hardness, and image quality guidelines developed by the Georgia Department of Natural Resources (Kaeser & Litts, 2010), and the proportion of each substrate within the 10-km reach of the river was determined. In 2012, the substrate classifications were groundtruthed to assess accuracy of these techniques. Groundtruthing consisted of ninty-five randomly selected points which were probed using the copper pole method to determine substrate type (Pritchett & Pyron, 2011). Correct substrate characterization occurred in 87 out of 95 points. Incorrect characterizations were likely caused by image distortion from shallow water and woody debris. The groundtruthed 2012 data were collected using only a single pass during the sonar survey, whereas an additional survey in 2014 used multiple passes to survey the entire river bottom. Therefore, the 2014 survey was used to match with telemetry data.
Data from active tracking of telemetered Silver Carp (all years) were overlaid with the available substrate data to determine the substrate type for each detection that was wholly within a single substrate polygon. Active tracking detections were not used if they were found to be on a line between two substrate types. Bedrock and boulder substrates composed <1% of available substrate (Boulder 0%, Bedrock 0.003%) and no Silver Carp occurred over these substrates; therefore, these substrates were not included in analyses. Only one detection, per individual, per week was utilized for analysis to minimize autocorrelation of consecutive detections. For each Silver Carp, proportions of detections over each substrate type were compared to the proportions of all available substrates using a Chi-squared test (χ 2). The resource selection index (W) for each non-zero bin (bins in this test were substrate type) was then calculated as a measure of preference or avoidance. Percent of active tracking detections in a given bin divided by the percent of available habitat in a given bin (W overlapping with 1 indicates neutral selection, W < 1 indicates habitat avoidance, W > 1 indicates habitat preference; Manly et al., 2002; Harrison et al., 2016). Bonferroni 95% confidence intervals (CI) were calculated around each W value (Thomas & Taylor, 1990; Manly et al., 2002; DeGrandchamp et al., 2008) to evaluate wether W values were significantly different than 1 which would indicate preference or avoidance (Hobbs & Bowden, 1982).
Depth, velocity, and temperature
Velocity (cm/s), depth (m), and water temperature (°C) were collected using an acoustic Doppler current profiler (ADCP) that measured three-dimensional water velocity profiles over the water column (with the exception of near-surface and near-bed areas due to instrument limitations; Jackson, 2016). The ADCP data were collected by the U.S. Geological Survey (USGS) to examine bigheaded carps’ spawning in the Wabash River, and so ADCP data were only collected for a specific river reach. The boat-mounted ADCP was used to measure along the thalweg of the Wabash River over a two-day period covering approximately 13 km near West Lafayette, IN, during summer 2012 (26-Jun to 27-Jun) and again in 2013 (16-Jun to 17-Jun). In 2012, a 2 MHz TRDI StreamPro ADCP (Teledyne RD Instruments, Poway, CA, USA) was used. In 2013, a 1200 kHz Rio Grande ADCP (Teledyne RD Instruments, Poway, CA, USA) was used. The StreamPro is capable of measuring in shallow water (<0.5 m) and was well suited to the low flows in the Wabash River during the 2012 drought. In both years, the ADCP data were georeferenced using a Hemisphere Crescent A100 differential GPS receiver with sub-meter accuracy (Hemisphere GPS, Calgary, AB, Canada). The ADCP collected a velocity profile approximately every second (depends on instrument and configuration) and these data were processed using the Velocity Mapping Toolbox (Parsons et al., 2013). Depth-averaged velocities (averaged over the measured portion of the water column) for each profile were temporally averaged over 30-s windows to reduce the noise in the data and provide mean values of velocity, depth, and temperature for short reaches. The location of each reach-averaged mean parameter was assigned the mean of latitude and longitude of the positions of the 1-s data within each 30-s window. Water temperature was measured at the head of the ADCP, which was typically submerged <10 cm below the water surface.
Active tracking detections from the 13 km reach collected within a maximum of two weeks of each ADCP data collection event were used to assess possible environmental preferences. This time period was selected to minimize potential variation in flow and temperature that could occur with changes in river discharge (USGS) streamflow gaging station number 03336000: Wabash River at Covington, IN; Fig. 2). Silver Carp active tracking detections were overlaid with the ADCP 30-s mean data points. The measurements from the ADCP point closest to each telemetry detection were considered to be the values of velocity, depth, and temperature used by the telemetered fish. Only one detection, per individual in each year, was used. All 30-s ADCP points were considered the available habitat within the river reach examined. Available environmental conditions were binned as follows: velocity (10 cm/s bins), depth (0.5 m bins), and temperature (0.5°C bins in 2012; 0.2°C bins in 2013; e.g., Manly et al., 2002; Harrison et al., 2016). Bin size was selected to minimize zeros in available habitat. Manly’s habitat selection index (W) and Bonferroni 95% CIs were calculated and evaluated for each non-zero bin as described for substrate (Manly et al., 2002; Harrison et al., 2016). All statistics for this study were conducted in R (V. 3.1.2) with an α = 0.05 unless otherwise indicated.
Dates of acoustic Doppler current profiler (ACDP) sampling and dates of corresponding active tracking detections from Silver Carp in the Wabash River, Indiana, displayed with hydrographs of streamflow at Wabash River at Covington, IN (USGS streamflow gaging station number 03336000). Vertical-dashed lines indicate the first day of ADCP sampling which lasted for 2 days total. In 2012, active tracking detections of Silver Carp occurred from 6-Jun to 11-Jul. In 2013, active tracking detections occurred from 19-Jun to 25-Jun. Gray-shaded areas indicate the time period from which active tracking detections were used to match with ADCP points (within 2 weeks of ADCP sampling and without a major change in river discharge)
Results
Overall total range
Silver Carp 1-year total ranges (19.4 km [24.3, 14.6 95% CI], n = 104) were significantly smaller than 2- (67.5 km [104.1, 30.8 CI], n = 41) and 3-year total ranges (67.8 [85.5, 50.1 CI], n = 60; Kruskal–Wallis: χ 2 = 57.1, df = 2, P < 0.0001; Mann–Whitney: 1–2-year, W = 1711, P = 0.02, 1–3-year, W = 1192, P < 0.0001), although the latter two were not significantly different from each other (Mann–Whitney: 2–3-year, W = 802, P = 0.04; Table 1). Therefore, individuals with total ranges derived from 2 or 3 years were combined. Numbers of detections were not significantly correlated with overall total range (Spearman rank correlation: ρ = 0.09, P = 0.18). Frequency distributions of Silver Carp overall total range sizes indicate that many individuals had either relatively small (<26 km, n = 40) or very large ranges (>102 km, n = 17), with an additional subset of individuals exhibiting intermediately sized ranges (Fig. 3). There was no apparent relationship between fish total length and overall total range size (Spearman rank correlation: ρ = 0.10, P = 0.32) and no significant difference in range size between sexes (males: 94.9 km [140.4, 49.4 CI], female: 75.9 km [132.2, 19.5 CI]; Mann–Whitney: W = 75, P = 0.89, n males = 6, n females = 24).
Annual total and home ranges
Number of detections was not significantly correlated with total or home range estimates (Total range: [2011] ρ = 0.02, P = 0.86, [2012] ρ = 0.05, P = 0.61, [2013] ρ = −0.09, P = 0.19; 95% home range: [2011] ρ = 0.29, P = 0.21, [2012] ρ = −0.04, P = 0.87, [2013] ρ = 0.18, P = 0.50; 75% home range: [2011] ρ = -0.34, P = 0.15, [2012] ρ = −0.25, P = 0.29, [2013] ρ = 0.18, P = 0.44). For Silver Carp with telemetry data from all three study years, annual total range did not vary among years (Table 2; 35.8 km [43.3, 28.3 CI]; Friedman rank sum: χ 2 = 2.4, df = 2, n = 60, P = 0.304; Fig. 4), and this was also true of kernel density annual home range estimates at the 95% isopleth (31.2 km [37.7, 24.7]; Friedman rank sum: χ 2 = 3.4, P = 0.19). However, kernel density annual home range estimates at the 75% isopleth were significantly different among years (Friedman rank sum: χ 2 = 15.3, P = 0.0005), and post hoc testing indicated significant differences between 2011 (2.1 km [2.6, 1.6 CI]) and 2013 (4.2 km [5.1, 3.3 CI]; P = 0.0003), 2012 (2.7 km [3.5, 1.9 CI]) and 2013 (P = 0.03), but not between 2011 and 2012 (P = 0.72). Mean rkm of annual total (Friedman rank sum: χ 2 = 13.4, P = 0.001), 95% isopleth (Friedman rank sum: χ 2 = 7.03, P = 0.03), and 75% isopleth (Friedman rank sum: χ 2 = 11.78, P = 0.003) home range all showed differences among years. Mean rkm of annual total ranges were more upstream in 2011 (523.9 [538.9, 509.0 CI]) than in 2012 (560.1 [520.7, 492.5 CI], P = 0.001) and 2013 (561.7 [522.0, 497.0 CI], P = 0.02) while 2012 and 2013 were not different from each other (P = 0.74). Mean rkm of 95% annual home ranges were more upstream in 2011 (539.7 [555.0, 524.4 CI]) than in 2013 (512.3 [521.1, 497.1 CI], P = 0.02), but were not different between 2011 and 2012 (522.0 [533.4, 510.6 CI], P = 0.31) or between 2012 and 2013 (P = 0.46). Mean rkm of 75% annual home ranges were more upstream in 2011 (523.4 [538.6, 508.3 CI]) than 2012 (506.5 [520.7, 492.4 CI], P = 0.005) and 2013 (509.6 [522.1, 497.1 CI], P = 0.01) while 2012 and 2013 were not different (P = 0.94).
Habitat use
All but two of 261 total Silver Carp detections from 2011 to 2013 that overlaid the 10-km reach where substrate was mapped occurred wholly within a single substrate polygon. Silver Carp were detected in areas of each substrate type in a manner inconsistent with substrate availability in the study reach (Chi-square: χ 2 = 85.6, n = 259, df = 2, P = 0.04; Fig. 5), occurring most often over sand. Manly’s selection index indicated that Silver Carp showed selection for cobble (W = 4.00 [1.31, 6.63 CI]) and neutral preferences for gravel (W = 1.33 [0.12, 2.53 CI]) and sand (W = 0.67 [-0.14, 1.48 CI]). For both years, only one of all possible water velocity bins were positively selected by Silver Carp (Figs. 6, 7), 20–30 cm/s in 2012 and 91–100 cm/s (lowest velocity measured in 2013). Other available water velocities were avoided by Silver Carp. Silver Carp showed positive selection for greater water depths, >1 m in 2012 and >5 m in 2013, and they avoided areas with depths ≤1 m. Warmer temperatures were also positively selected by Silver Carp, >26°C in 2012 and >22.6°C in 2013. Silver Carp avoided lower water temperatures in both years, ≤26°C in 2012 and ≤22.4°C in 2013.
Mean water velocity, depth, and temperature measured by an acoustic Doppler current profiler (ADCP) and integrated over 30-s intervals from a reach of the Wabash River, Indiana, from river 521 to 508 km. Silver Carp (hollow bars) values represent the mean (±95% CI) of ADCP values associated with active tracking detections while ADCP points (black bars) are those not associated with active tracking detections
Resource selection index values (W) and Bonferroni 95% confidence intervals for Silver Carp in the Wabash River, Indiana. Available environmental conditions, as measured by acoustic Doppler current profiler (ADCP), were binned and then W was calculated for all non-zero bins as the percent Silver Carp detections within a habitat bin divided by the percent of available habitat in the habitat bin. W ± 95% Bonferroni CI values that overlap with 1 indicate neutral selection for that habitat bin while W values <1 indicate avoidance (–), and W values >1 indicate selection (+). X-axes of all figure parts were adjusted to show the range of available habitat measured by the ADCP (e.g., all values measured are within the highest and lowest values shown on an axis). X-axes tick marks indicate the size of the habitat bins, and tick mark labels are the upper values for each bin (e.g., tick mark label of 20 cm/s means that bin contains values between 10 and 20 cm/s)
Discussion
The bimodal distribution and variation in ranges we identified for invasive Silver Carp in the Wabash River provide evidence that this species exhibits individual-based movement tendencies. Specifically, we observed both relatively sedentary and highly mobile individuals for which maximum river extent used was consistently high over a period 3 years. Such variation in movement behavior among tagged individuals of the same species have been observed for many fish species (e.g., Brett, 1965; Gallaugher et al., 1995; Kolok, 1999; Roy et al., 2013). Because movements are known to vary at an individual level, it seems logical that range sizes would be similarly variable (e.g., Fish & Savitz, 1982; Baguette et al., 2013). Individual differences in movements may serve to link populations and prevent inbreeding (Bennett, 1987; Cooke et al., 2005) as well as promote colonization of new habitats (Biro & Stamps, 2008). Similar individual variation in range size may have a great impact on species invasion and establishment in novel environments, and thus the observed highly mobile individuals may be an important factor in the invasion success of Silver Carp.
Although most telemetered Silver Carp exhibited total range sizes ≤26 or >102 km, a third, smaller group of Silver Carp displayed intermediate-sized total ranges. Similar trichotomies have also been observed in the movements of both small cyprinids (e.g., Belica & Rahel, 2008) and other fish species (Rodríguez, 2002; Crook, 2004b; Radinger & Wolter, 2014) but the potential causes of these trichotomies are uncertain. Although tagged Silver Carp did not fit a strict dichotomy of sedentary versus mobile tendencies, the general propensity of most individuals to have either relatively small or very large total ranges could provide the basis for control efforts. Future control efforts that target more mobile individuals in Silver Carp or other invasive fish populations could reduce or prevent further spread of these fishes. For example, removal efforts could be timed to coincide with periods of greatest activity by mobile individuals or when individuals are more stationary depending on the removal method employed. Future research should focus on determining the characteristics specific to mobile individuals, including the genetic basis for fish movements, and the proportion at which these individuals occur in Silver Carp populations.
Sedentary and mobile movement tendencies may vary temporally for some fishes (Booth et al., 2014), and Silver Carp movement patterns have previously been shown to vary seasonally (DeGrandchamp et al., 2008; Coulter et al., 2016a). Annual climatic variation is also known to produce differences in fish movements and ranges (Winemiller & Jepsen, 1998; Jackson et al., 2001). We therefore expected growth of individuals over the 3-year study and annual differences in climate (e.g., extreme drought in 2012) would yield year-to-year variation in size and location of annual total and home ranges of tagged Silver Carp. However, annual total range and the 95% kernel density home range for Silver Carp showed no annual variation. The methods used to derive annual total and home range at the 95% isopleth were more influenced by short-term movements (i.e., spawning runs) than 75% home range sizes. Lack of variation in the sizes of these estimates indicates that individuals may be utilizing the same spawning site over multiple years and thus must travel the same distance each year. On the other hand, annual home range estimated using the 75% isopleth did vary annually and were larger in 2013 than in 2011. Fishes are known to increase their core home range as they grow (Hobbs et al., 2005; Downs & Horner, 2007). Additionally, discharge and water levels could have influenced annual home range size, although 2012 was a drought year and 75% isopleth range estimates were not different in this year. Overall, it appears that total river lengths utilized by Silver Carp did not change annually and were potentially driven by spawning site fidelity; however, their core range may still vary with changing resource needs or climate.
Silver Carp have spread into the Wabash River via the Ohio and Mississippi rivers and are expanding their range in an upstream direction (Kolar et al., 2007; USACE, 2010). This upstream expansion could cause mean rkm of individual ranges to increase if fish are moving in the upstream direction but we did not observe this in the current study. Mean rkm of annual total and home ranges were furthest upstream during the initial year of the study. Instead, mean rkm values were focused around landscape features. One of the few backwaters on the Wabash River that is consistently connected to the main channel is located at approximately rkm 508 (Coulter et al., 2016a) and Silver Carp are known to prefer low velocity and backwater habitats (DeGrandchamp et al., 2008). The presence of this backwater may attract Silver Carp to this location and mean rkms in 2012 and 2013 are very close to the location of this backwater. Additionally, several major bridges cross the Wabash River upstream and downstream from the backwater (~rkm 504, 510, 515) which correspond to several deeper pools and low velocity habitats which may also attract Silver Carp. Annual total range and 75% home ranges in 2011 were centered near the confluence of the Wabash River with one of its major tributaries, the Tippecanoe River (~ rkm 520). Tributaries may offer varied habitat and resources compared to the main channel. Other ranges were centered upstream of rkm 530 which is approximately where the Wabash River shifts from sand dominated substrate to a higher prevalence of cobble and bedrock which may be favoriable spawning habitat. Our results indicate that the tagged Silver Carp may not be advancing upstream in the Wabash River but that fish instead focus on specific habitat features. While the specific features selected may vary with annual differences in the environment, these features could be used to target removal and control efforts.
We expected total length of Silver Carp to influence variation in total range size and that sex would not, but neither were found to be significant. Other studies have also reported no difference in movements of male and female fishes (Moser & Ross, 1995; Skalski & Gilliam, 2000); however, some species do exhibit sex-based bias in range sizes (Hutchings & Gerber, 2002). There has been previous evidence that movement distances of Silver Carp are not correlated with sex (Coulter et al., 2016a), and our findings support this assertion. The similar ranges exhibited by male and female Silver Carp suggest that resource or habitat requirements are similar between sexes. However, it is important to note that our sample size for male carp was small, and this may have masked our ability to detect sex-specific differences in range size. Additionally, there was no relationship between range size and fish length. In other fish species, larger individuals have larger home ranges (Peters, 1983; Minns, 1995), but a suite of abiotic and biotic factors can alter this expectation (e.g., McLoughlin & Ferguson, 2000; Hansen & Closs, 2005; Woolnough et al., 2009). We speculate that food resources utilized by Silver Carp in our study area may be comparatively less patchy and potentially more abundant than other high trophic level diet items, and/or distributed by flow to the extent that food availability is not a factor that influences Silver Carp range size. However, individuals tagged in this study were likely all mature given their lengths and would thus all make spawning migrations. Additional study is needed to definitively assess the potential for fish total length, especially in immature fishes (e.g., Bowering, 1983), to influence range size in Silver Carp. Immature individuals that do not participate in spawning migrations may exhibit smaller ranges and so may make good targets for removal efforts, removing individuals from the population prior to reproduction and reducing future recruitment.
River substrates may be characterized by differences in availability of other resources, such as macroinvertebrates, which can influence use of specific substrates by fishes (e.g., Grossman et al., 1987; Garman, 1991; Fischer, 2000). Silver Carp were found most often over sand, which is the dominant available substrate in the river segment examined. Sand substrate is sometimes associated with lower velocities (Wetzel, 2001) and areas of deposition that Silver Carp seem to prefer (e.g., DeGrandchamp et al., 2008). However, Manly’s habitat selection index indicated that Silver Carp were positively selecting cobble substrate. Cobble substrates may increase turbulent flow (Vogel, 1996), and Silver Carp tend to spawn in more turbulent water (Kolar et al., 2007). Spawning was detected concurrent to most of the active tracking detections that were used to match with substrate mapping (Coulter et al., 2016b), and so spawning behavior may have been driving some of the observed substrate selection.
Silver Carp exhibited differential habitat use when environmental conditions associated with telemetry detections were compared to available habitat in the study segment. Observed differences supported the hypothesis that Silver Carp would occupy areas that were warmer, deeper, and had lower water velocities. Silver Carp have previously been found in lower velocity habitats (Kolar et al., 2007; DeGrandchamp et al., 2008), and it was not surprising that telemetered individuals preferred lower velocities, although they also avoided velocities ≤20 cm/s. Occupying lower velocity waters likely decreases energy requirements for fishes maintaining position in lotic ecosystems (Silva et al., 2012), and habitats of varied velocities may also have differences in food availability that drive fish distributions (Calkins et al., 2012). The lowest measured velocities in 2012 that were avoided by Silver Carp may have been concurrent with shallower depths or reduced food availability. Telemetered Silver Carp tended to occupy areas of deeper water, although the actual depth at which the individuals were located was not quantified. During the 2012 drought, selection for areas with greater water depths was stronger than in 2013. Future drought events may serve to concentrate Silver Carp in deeper pools, thus providing opportunities for more efficient removal. Thermal optima for Silver Carp are higher than water temperatures measured via ADCP in the Wabash River (Adults: 29°C, Kolar et al., 2007), although Wabash River water temperatures likely increased into July and August (Mean monthly temperature increase June–August 2012: +6°C, 2013: +3°C; USGS streamflow gaging station number 03323500 Wabash River at Huntington, IN). Therefore, telemetered Silver Carp preference for warmer water temperatures likely reflected thermal selection to maximize growth in habitat that was closest to the species’ thermal optima.
Our findings expand the current literature on fish movements by demonstrating that individuals in a population of large-bodied cyprinids exhibit relatively sedentary or highly mobile tendencies. Results from our study can be applied to research involving other aquatic invasive species, incorporated into management strategies for Silver Carp, and used as baselines for comparing and modeling Silver Carp movement, habitat use, and future range expansion. For example, habitat and range information could be used to set harvest goals to reduce abundances or improve modeling efforts by incorporating behavior (e.g., Guisan & Thuiller, 2005; Rhodes et al., 2005). Range estimates can aid in determining the distances over which Silver Carp populations may expand into new habitats and aid in delineating population boundaries. Additionally, information on habitats used by Silver Carp could be used to target removal efforts and improve early detection (e.g., eDNA) in environments where Silver Carp may invade or occur in low densities. Our results indicate that removal efforts should target deeper, warmer, and lower velocity habitats. If individuals are removed from these preferred habitats, additional Silver Carp may replace the harvested individuals and thus these same locations could be harvested on multiple occasions potentially creating population sinks. As observed in 2012, droughts could serve to concentrate Silver Carp into very specific habitats, further increasing harvest efficiency when water levels remain sufficient for successfully deploying fishery gears.
Change history
24 August 2017
An erratum to this article has been published.
References
Abdusamadov, A. S., 1987. Biology of white amur (Ctenopharyngodon idella), silver carp (Hypophthalmichthys molitrix) and bighead (Aristichthys nobilis), acclimatized in the Terek Region of the Caspian Basin. Journal of Ichthyology 26: 41–49.
Aparicio, E. & A. De Sostoa, 1999. Pattern of movements of adult Barbus haasi in a small Mediterranean stream. Journal of Fish Biology 55: 1086–1095.
Baguette, M., S. Blanchet, D. Legrand, V. M. Stevens & C. Turlure, 2013. Individual dispersal, landscape connectivity and ecological networks. Biological Reviews 88: 310–326.
Belica, L. A. T. & F. J. Rahel, 2008. Movements of creek chubs, Semotilus atromaculatus, among habitat patches in a plains stream. Ecology of Freshwater Fish 17: 258–272.
Bengsston, B. O., 1978. Avoiding inbreeding: at what cost? Journal of Theoretical Biology 73: 439–444.
Bennett, A. F., 1987. Interindividual variability: an underutilized resource. In Feder, M. E., A. F. Bennett, W. W. Burggren & R. B. Huey (eds), New Directions in Ecological Physiology. Cambridge University Press, Cambridge: 147–169.
Biro, P. A. & J. A. Stamps, 2008. Are animal personality traits linked to life-history productivity? Trends in Ecology and Evolution 23: 361–368.
Booth, M. T., A. S. Flecker & N. G. Hairston Jr., 2014. Is mobility a fixed trait? Summer movement patterns of Catostomids using PIT telemetry. Transactions of the American Fisheries Society 143: 1098–1111.
Bowering, W. R., 1983. Age, growth, and sexual maturity of Greenland halibut, Reinhardtius hippoglossoides (Walbaum), in the Canadian Northwest Atlantic. Fishery Bulletin 81: 599–611.
Brett, J. R., 1965. The relation of size to rate of oxygen consumption and sustained swimming speed of sockeye salmon (Oncorhynchus nerka). Journal of the Fisheries Board of Canada 22: 1491–1501.
Burt, W. H., 1943. Territoriality and home range concepts as applied to mammals. Journal of Mammology 24: 346–352.
Calkins, H. A., S. J. Tripp & J. E. Garvey, 2012. Linking silver carp habitat selection to flow and phytoplankton in the Mississippi River. Biological Invasions 14: 949–958.
Chen, P., E. O. Wiley & K. M. Mcnyset, 2007. Ecological niche modeling as a predictive tool: silver and bighead carps in North America. Biological Invasions 9: 43–51.
Chick, J. H. & M. A. Pegg, 2001. Invasive carp in the Mississippi River basin. Science 292: 2250–2251.
Clapp, D. F., R. D. Clark Jr. & J. S. Diana, 1990. Range, activity, and habitat of large, free-ranging brown trout in a Michigan stream. Transactions of the American Fisheries Society 119: 1022–1034.
Cooke, S. J., G. T. Crossin, D. A. Patterson, K. K. English, S. G. Hinch, J. L. Young, R. F. Alexander, M. C. Healey, G. Van Der Kraak & A. P. Farrell, 2005. Coupling non-invasive physiological assessments with telemetry to understand inter-individual variation in behavior and survivorship of sockeye salmon: development and validation of a technique. Journal of Fish Biology 67: 1342–1358.
Coulter, A. A., D. Keller, J. J. Amberg, E. J. Bailey & R. R. Goforth, 2013. Phenotypic plasticity in the spawning traits of bigheaded carp (Hypophthalmichthys spp.) in novel ecosystems. Freshwater Biology 58: 1029–1037.
Coulter, A. A., E. J. Bailey, D. Keller & R. R. Goforth, 2016a. Invasive Silver Carp movement patterns in the predominantly free-flowing Wabash River (Indiana, USA). Biological Invasions 18: 471–485.
Coulter, A. A., D. Keller, E. J. Bailey & R. R. Goforth, 2016b. Predictors of bigheaded carp drifting egg density and spawning activity in an invaded, free-flowing river. Journal of Great Lakes Research 42: 83–89.
Crook, D. A., 2004a. Is the home range concept compatible with the movements of two species of lowland river fish? Journal of Animal Ecology 73: 353–366.
Crook, D. A., 2004b. Movements associated with home-range establishment by two species of lowland river fish. Canadian Journal of Fisheries and Aquatic Sciences 61: 2183–2193.
Cudmore, B., N. E. Mandrak, J. M. Dettmers, D. C. Chapman & C. S. Kolar, 2012. Binational ecological risk assessment of bigheaded carps (Hypophthalmichthys spp.) for the Great Lakes Basin. Department of Fisheries and Oceans, Ottawa, Ontario, Canada, No. 2011/114.
DeGrandchamp, K. L., J. E. Garvey & R. E. Colombo, 2008. Movement and habitat selection by invasive Asian carps in a large river. Transactions of the American Fisheries Society 137: 45–56.
Deters, J. E., D. C. Chapman & B. McElroy, 2013. Location and timing of Asian carp spawning in the Lower Missouri River. Environmental Biology of Fishes 96: 617–629.
Downs, J. A. & M. W. Horner, 2007. Network-based kernel density estimation of home range analysis. Proceedings of the Ninth International Conference on Geocomputation, Maynooth, Ireland.
Fieberg, J., 2007. Kernel density estimators of home range: smoothing and the autocorrelation red herring. Ecology 88: 1059–1066.
Fischer, P., 2000. An experimental test of metabolic and behavioural responses of benthic fish species to different types of substrate. Canadian Journal of Fisheries and Aquatic Sciences 57: 2336–2344.
Fish, P. A. & J. Savitz, 1982. Variation in home ranges of largemouth bass, yellow perch, bluegills, and pumpkinseeds in an Illinois lake. Transactions of the American Fisheries Society 112: 147–153.
Fraser, D. F., J. F. Gilliam, M. J. Daley, A. N. Le & G. T. Skalski, 2001. Explaining leptokurtic movement distributions: intrapopulation variation in boldness and exploration. The American Naturalist 158: 124–135.
Funk, J. L., 1955. Movement of stream fishes in Missouri. Transactions of the American Fisheries Society 85: 39–57.
Gallaugher, P., H. Thorarensen & A. P. Farrell, 1995. Hematocrit in oxygen transport and swimming in rainbow trout (Oncorhynchus mykiss). Respiration Physiology 102: 279–292.
Garman, G. C., 1991. Use of terrestrial arthropod prey by a stream-dwelling cyprinid fish. Environmental Biology of Fishes 30: 325–331.
Gerking, S. D., 1950. Stability of a stream fish population. Journal of Wildlife Management 14: 193–202.
Gerking, S. D., 1953. Evidence for the concepts of home range and territory in stream fishes. Ecology 34: 347–365.
Gerking, S. D., 1959. The restricted movement of fish populations. Biological Reviews 34: 221–242.
Goforth, R. R. & J. W. Foltz, 1999. Movements of the yellowfin shiner, Notropis lutipinnis. Ecology of Freshwater Fish 7: 49–55.
Gowan, C., M. K. Young, K. D. Fausch & S. C. Riley, 1994. Restricted movement in resident stream salmonids: a paradigm lost? Canadian Journal of Fisheries and Aquatic Sciences 51: 2626–2637.
Grossman, G. D., A. De Sostoa, M. C. Freeman & J. Lobon-Cervía, 1987. Microhabitat use in a Mediterranean riverine fish assemblage. Oecologia 73: 501–512.
Guisan, A. & W. Thuiller, 2005. Predicting species distribution: offering more than simple habitat models. Ecology Letters 8: 993–1009.
Hansen, E. A. & G. P. Closs, 2005. Diel activity and home range size in relation to food supply in a drift-feeding stream fish. Behavioral Ecology 16: 640–648.
Harrison, P. M., L. F. G. Gutowsky, E. G. Martins, D. A. Patterson, S. J. Cooke & M. Power, 2016. Temporal plasticity in thermal-habitat selections of burbot Lota lota a diel-migrating winter-specialist. Journal of Fish Biology 88: 2111–2129.
Hobbs, N. T. & D. C. Bowden, 1982. Confidence intervals of food preference indices. Journal of Wildlife Management 46: 505–507.
Hobbs, R. C., K. L. Laidre, D. J. Vos, B. A. Mahoney & M. Eagleton, 2005. Movements and area use of belugas, Delphinapterus lencas, in a subarctic Alaskan estuary. Arctic 58: 331–340.
Hoggatt, R. E., 1975. Drainage areas of Indiana streams. U.S. Department of the Interior Geological Survey, Water Resources Division, Indianapolis.
Hollander, M. & D. A. Wolfe, 1999. Nonparametric Statistical Methods, 2nd ed. Wiley, New York.
Hughes, C. L., C. Dytham & J. K. Hill, 2007. Modelling and analysing evolution of dispersal in populations at expanding range boundaries. Ecological Entomology 32: 437–445.
Hutchings, J. A. & L. Gerber, 2002. Sex-biases dispersal in a salmonid fish. Proceedings of the Royal Society of London B: Biological Sciences 269: 2487–2493.
Jackson, D. A., P. R. Peres-Neto & J. D. Olden, 2001. What controls who is where in freshwater fish communities – the roles of biotic, abiotic, and spatial factors. Canadian Journal of Fisheries and Aquatic Sciences 58: 157–170.
Jackson, P. R., 2016. Spatial distribution of depth-averaged velocity measured in the Wabash River near West Lafayette, IN (June 26-27, 2012 and June 17-18, 2013): U.S. Geological Survey data release, http://dx.doi.org/10.5066/F78W3BDQ.
Jayasinghe, U. A. D., E. G. Berthou, Z. Li, W. Li, T. Zhang & J. Liu, 2015. Co-occurring bighead and silver carps show similar food preferences but different isotopic niche overlap in different lakes. Environmental Biology of Fishes 98: 1185–1199.
Kaeser, A. J. & T. L. Litts, 2010. A novel technique for mapping habitat in navigable streams using low-cost side scan sonar. Fisheries 35: 163–174.
Kennedy, G. J. A., 1981. Individual variation in homing tendency in the European minnow, Phoxinus phoxinus (L). Animal Behavior 29: 621–625.
Kocovsky, P. M., D. C. Chapman & J. E. McKenna, 2012. Thermal and hydrologic suitability of Lake Erie and its major tributaries for spawning of Asian carps. Journal of Great Lakes Research 38: 159–166.
Kolar, C.S., D. C. Chapman, W. R. Courtenay Jr., C. M. Housel, J. D. Williams & D. P. Jennings, 2007. Bigheaded carps: a biological synopsis and environmental risk assessment. American Fisheries Society Special Publication 33, Bethesda.
Kolok, A. S., 1999. Interindividual variation in the prolonged locomotor performance of ectothermic vertebrates: a comparison of fish and herpetofaunal methodologies and a brief review of the recent fish literature. Canadian Journal of Fisheries and Aquatic Sciences 56: 700–710.
Lenaerts, A. W., A. A. Coulter, Z. F. Feiner & R. R. Goforth, 2015. Egg size variability in an establishing population of invasive silver carp Hypophthalmichthys molitrix (Valenciennes 1844). Aquatic Invasions 10.
Lester, S. E., B. I. Ruttenberg, S. D. Gaines & B. P. Kinlan, 2007. The relationship between Dispersal ability and geographic range size. Ecology Letters 10: 745–758.
Linfield, R. S. J., 1985. An alternative concept to home range theory with respect to populations of cyprinids in major river systems. Journal of Fish Biology 27: 187–196.
Lockwood, J. L., P. Cassey & T. Blackburn, 2005. The role of propagule pressure in explaining species invasions. Trends in Ecology and Evolution 20: 223–228.
MacNamara, R., D. Glover, J. Garvey, W. Bouska & K. Irons, 2016. Bigheaded carps (Hypophthalmichthys spp.) at the edge of their invaded range: using hydroacoustics to assess population parameters and the efficacy of harvest as a control strategy in a large North American river. Biological Invasions 18: 3293–3307.
Magurran, A. E., 1986. Individual difference in fish behaviour. In Pitcher, T. J. (ed.), The Behaviour of Teleost Fish. Croom Helm, London: 338–365.
Manly, B. F. J., L. L. McDonald, D. Thomas, T. L. McDonald & W. P. Erickson, 2002. Resource Selection by Animals: Statistical Design and Analysis for Field Studies, 2nd ed. Kluwer, London.
McLoughlin, P. D. & S. H. Ferguson, 2000. A hierarchical pattern of limiting factors helps explain variation in home range size. Écoscience 7: 123–130.
Mia, M. Y., J. B. Taggart, A. E. Gilmour, A. A. Gheyas, T. K. Das, K. H. M. Kohinoor, M. A. Rahman, M. A. Sattar, M. G. Hussain, M. A. Mazid, D. J. Penman & B. J. McAndrew, 2005. Detection of hybridization between Chinese carp species (Hypopthalmichthys molitrix and Aristichthys nobilis) in hatchery broodstock in Bangladesh, using DNA microsatellite loci. Aquaculture 247: 267–273.
Minns, C. K., 1995. Allometry of home range size in lake and river fishes. Canadian Journal of Fisheries and Aquatic Sciences 52: 1499–1508.
Moser, M. L. & S. W. Ross, 1995. Habitat use and movements of shortnose and Atlantic sturgeons in the lower Cape Fear River, North Carolina. Transactions of the American Fisheries Society 124: 225–234.
Parsons, D. R., P. R. Jackson, J. A. Czuba, F. L. Engel, B. L. Rhoads, K. A. Oberg, J. L. Best, D. S. Mueller, K. K. Johnson & J. D. Riley, 2013. Velocity mapping toolbox (VMT): a processing and visualization suite for moving-vessel ADCP measurements. Earth Surface Processes and Landforms 38: 1244–1260.
Peters, R. H., 1983. The Ecological Implications of Body Size. Cambridge Press, Toronto.
Peterson, A. T., 2003. Predicting the geography of species’ invasions via ecological niche modelling. The Quarterly Review of Biology 78: 419–433.
Phillips, S. J. & M. Dudík, 2008. Modeling of species distributions with Maxent: new extensions and a comprehensive evaluation. Ecography 31: 161–175.
Pritchett, J. & M. Pyron, 2011. Predicting fish assemblages form substrate variation in a turbid river: grab samples compared to pole probing. North American Journal of Fisheries Management 31: 574–579.
Radinger, J. & C. Wolter, 2014. Patterns and predictors of fish dispersal in rivers. Fish and Fisheries 15: 456–473.
Rhodes, J. R., C. A. McAlpine, D. Lunney & H. P. Possingham, 2005. A Spatially explicit habitat selection model incorporating home range behaviour. Ecology 86: 1199–1205.
Rodríguez, M. A., 2002. Restricted movement in stream fish: the paradigm is incomplete, not lost. Ecology 83: 1–13.
Roy, M. L., A. G. Roy, J. W. A. Grant & N. E. Bergeron, 2013. Individual variability in the movement behavior of juvenile Atlantic salmon. Canadian Journal of Fisheries and Aquatic Sciences 70: 339–347.
Schrank, S. J., P. J. Braaten & C. S. Guy, 2001. Spatiotemporal variation in density of larval bighead carp in the lower Missouri River. Transactions of the American Fisheries Society 130: 809–814.
Silva, A. T., C. Katopodis, J. M. Santos, M. T. Ferreira & A. N. Pinheiro, 2012. Cyprinid swimming behaviour in response to turbulent flow. Ecological Engineering 44: 314–328.
Skalski, G. T. & J. F. Gilliam, 2000. Modeling diffusive spread in a heterogeneous population: a movement study with stream fish. Ecology 81: 1685–1700.
Stuck, J. G., A. P. Porreca, D. H. Wahl & R. E. Columbo, 2015. Contrasting population demographics of invasive Silver Carp between an impounded and free-flowing river. North American Journal of Fisheries Management 35: 114–122.
Thomas, D. L. & E. J. Taylor, 1990. Study designs and tests for comparing resource use and availability. Journal of Wildlife Management 54: 322–330.
Travis, J. M. & C. Dytham, 2002. Dispersal evolution during invasions. Evolutionary Ecology Research 4: 1119–1129.
Tyler, J. A. & J. F. Gilliam, 1995. Ideal free distributions of stream fish: a model and test with minnows Rhinicthys atratulus. Ecology 76(5): 80–592.
USACE (United State Army Corps of Engineers), 2010. Great lakes and Mississippi river interbasin study: other pathways preliminary risk characterization. U.S. Army Engineer District, Louisville, Kentucky. http://glmris.anl.gov/documents/docs/Other_Pathways_Risk.pdf. Accessed 19 December 2014.
Vogel, S., 1996. Life in Moving Fluids: The Physical Biology of Flow. Princeton University Press, Princeton.
Waldman, B. & J. S. McKinnon, 1993. Inbreeding and outbreeding in fishes, amphibians, and reptiles. In Thornhill, N. W. (ed.), The Natural History of Inbreeding and Outbreeding: Theoretical and Empirical Perspectives. University of Chicago Press, Chicago: 250–282.
Wetzel, R. G., 2001. Limnology: Lake and River Ecosystems, 3rd ed. Academic Press, San Diego.
Winemiller, K. O. & D. B. Jepsen, 1998. Effects of seasonality and fish movement on tropical river food webs. Journal of Fish Biology 53: 267–296
Woolnough, D. A., J. A. Downing & T. J. Newton, 2009. Fish movement and habitat use depends on water body size and shape. Ecology of Freshwater Fish 18: 83–91
Wright, D., L. B. Rimmer, V. L. Pritchard, R. K. Butlin & J. Krause, 2003. Inter and intra-population variation in shoaling and boldness in the zebrafish (Danio rerio). Journal of Fish Biology 63: 258–259.
Acknowledgements
Funding for the telemetry portion of this study was provided by the Indiana Department of Natural Resources (EDS E2-1-D639) and the Great Lakes Restoration Initiative. We thank Doug Keller, Tom Stefanavage, and Craig Jansen for assisting in the execution of this project and Zachary Feiner for coding help. We also thank the many Goforth lab students and technicians at Purdue University as well as United States Geological Survey Indiana-Kentucky Water Science Center personnel for assistance in the field. We appreciate the feedback and guidance provided by anonymous reviewers of this manuscript and E. Murphy. A. Coulter was supported by a Graduate Assistance in Areas of National Need fellowship and a Purdue University Department of Forestry and Natural Resources Ross Fellowship during the completion of this work. Funding from the National Institute of Food and Agriculture also supported R. Goforth and the Goforth lab during this study. Any use of trade, firm, or product names is for descriptive purposes only and does not imply endorsement by the U.S. Government.
Author information
Authors and Affiliations
Corresponding author
Additional information
Handling editor: Andrew Dzialowski
An erratum to this article is available at https://doi.org/10.1007/s10750-017-3348-3.
Rights and permissions
About this article
Cite this article
Prechtel, A.R., Coulter, A.A., Etchison, L. et al. Range estimates and habitat use of invasive Silver Carp (Hypophthalmichthys molitrix): evidence of sedentary and mobile individuals. Hydrobiologia 805, 203–218 (2018). https://doi.org/10.1007/s10750-017-3296-y
Received:
Revised:
Accepted:
Published:
Issue Date:
DOI: https://doi.org/10.1007/s10750-017-3296-y