Abstract
This study investigates the characteristic of heavy metals (Pb, Zn, Cu, Cd, Cr, Ni and As) in biochar derived from sewage sludge at different pyrolysis temperatures (300, 400, 500, 600 and 700 °C). The heavy metal concentrations, chemical speciation distribution, leaching toxicity, and bio-available contents were investigated using ICP-OES after microwave digestion, a sequential extraction procedure recommended by the Community Bureau of Reference (BCR), an improved nitric acid–sulphuric acid method, and diethylenetriamine pentaacetic acid (DTPA) extraction method, respectively. The results showed that a great percentage of the heavy metals remained in biochar, the concentrations of heavy metals in biochar (except Cd in B7) were higher than that in sludge, and the enrichment of the heavy metals in biochar enhanced with the pyrolysis temperature. Although the effect of pyrolysis temperature on the chemical speciation distribution, the leaching toxicity and the bio-available contents of heavy metals in biochar was inconsistent, the potential risk of biochar on soil and groundwater contamination was lower than sewage sludge.
Similar content being viewed by others
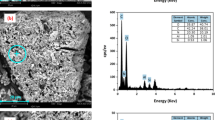
Explore related subjects
Discover the latest articles, news and stories from top researchers in related subjects.Avoid common mistakes on your manuscript.
Introduction
The sewage sludge is a by-product generated in the sewage treatment process, containing rich organic material and nutrient elements such as nitrogen, phosphorus and potassium, and lots of toxic and harmful substances like germs, ova and heavy metals [1]. With the greatly increasing demands for resources, the purpose of sewage sludge treatment was converted from volume and mass reductions to energy and resource utilization [2, 3]. Although farm application effectively re-utilized nutrient elements contained in sewage sludge, there are potential environment risks, germs, ova and heavy metals originally contained in sewage sludge may result into soil and groundwater contamination, which greatly restricted large-scale farm application of sewage sludge as soil conditioner. Pyrolysis treatment, transferring the chemical energy from sewage sludge to syngas and bio-oil, is a relatively clean energy utilization method, but pyrolysis solid residual has long not been given enough attention to its further utilization [4].
Recently, the pyrolysis solid residual, biochar, has attracted great attention because of its carbon sequestration and soil amendment benefits [5, 6]. Because of its good biological and chemical stability, biochar can exist in the soil for more than a thousand years, thus reduce carbon emission effectively [7]. In addition, biochar will help to improve the soil through raising the soil organic matter content, nutrient content, enhancing the water and nutrient holding capacity, aeration etc. [7, 8]. Compared to biomass feedstocks, sewage sludge is rich in nutrient elements (N, P and K) and minerals; therefore, sewage sludge biochar has inherent advantage of improving the soil fertility. However, the majority of heavy metals (except the volatile elements Hg and Cd) originally contained in sewage sludge still remained in biochar [9–11], thus almost all the researches about sewage sludge biochar includes the investigation on heavy metal migratory behaviors and its potential risk on soil and groundwater contamination. For example, Hossain et al. [12] reported the accumulation of heavy metal in biochar, and an obvious decline in available heavy metal content. Jin et al. [4] also found that all heavy metals in sewage sludge were kept in biochar except As, and their contents were enriched 2.5–3.5 times, whereas fast pyrolysis significantly suppressed heavy metals leaching from biochar. Moreover, Agrafioti et al. [3] found pyrolysis suppressed heavy metal release in acetic acid extraction with pH of 5.9 and 6.0. Additionally, the results from leaching experiments also showed that the leaching contents of heavy metals in biochar were significantly lower than those in sewage sludge [13, 14]. He, Hwang and Kistler et al. [15–17] also concluded that heavy metals are immobile and stable in the biochar and that pyrolysis is able to reduce their potential release.
Although extensive studies were conducted, the specific and comprehensive investigation on heavy metals in sewage sludge biochar was still scarce. The main objective of the present work is to study the characteristic of heavy metals in biochar derived from sewage sludge. Hence, heavy metals contents in biochar produced at various temperatures were investigated first, then their leaching contents were investigated to reflect their leaching toxicity. Extraction solvents with pH of 5.0 and 6.0 were used here to better simulate the actual acid soil environment. Finally, their chemical speciation distributions and bio-available contents were investigated to reflect their eco-toxicity and bioavailability, respectively.
Materials and methods
Experimental materials
The entire sewage sludge sample used in experiments was collected from Guangzhou Liede sewage treatment plant, with the treatment process of anaerobic–anoxic–aerobic. Dewatering of sewage sludge was conducted using a belt filter press and conditioning by cationic polymeric flocculants. The sewage sludge sample was first separated from other impurities, such as glass fragments and plastic bags, and then dried in a convection laboratory oven at room temperature until it was qualified for pulverization. The sewage sludge sample was then ground to 1- to 2-mm fine particles, stirred and mixed in mortar to ensure that the collected sewage sludge sample was homogenous and representative for subsequent experiment. Finally, the sewage sludge sample was dried again overnight at temperature of 105 °C in a convection laboratory oven and was stored in sealed plastic bags until subsequent experiment. The ultimate analysis and proximate analysis of sewage sludge sample are shown in Table 1.
Experimental device and process
The experimental device (showed in Fig. 1) for biochar preparation was horizontal quartz tube pyrolysis furnace, with a temperature range of room temperature and 1000 °C, and a temperature precision of 1 °C. The quartz tube, with 600 mm length and 50 mm inner diameter, had two detachable rubber plugs on both sides, respectively, connected with inlet and outlet pipes. Purified nitrogen (99.999 %) was provided by nitrogen cylinder to ensure that the whole pyrolysis process was under the oxygen-free atmosphere. The flow meter 1, connected with the inlet pipe, was used to measure the nitrogen flow to maintain the purging flow at 1000 mL min−1. The first flask, loaded with ice water mixture, was used to condense and collect the bio-oil and water vapor. The second flask, loaded with sodium hydroxide solution, was used to absorb acidic gases. The flowmeter 2, connected with the outlet pipe, was used to measure the flow of nitrogen and syngas.
Experimental process was described below. 100 ± 1 g sewage sludge sample was loaded into the middle of the quartz tube, then the quartz tube was sealed with two rubber plugs, and the nitrogen flow was opened to purge the quartz tube for 30 min. Meanwhile, the pyrolysis furnace was heated with a heat rate of 10 °C per minute. The quartz tube was placed into the pyrolysis furnace when it reached the desired temperature (300, 400, 500, 600 and 700 °C). During pyrolysis, the gas flow rate was measured by flowmeter 2, which gradually increased and then decreased, when it was consistent with that measured by flowmeter 1, indicating the pyrolysis process completed (no syngas emitting). Then, the pyrolysis furnace was turned off and the quartz tube was removed from the pyrolysis furnace quickly. When the quartz tube naturally was cooled to room temperature, the purified nitrogen flow was stopped, the biochar was recovered and kept in the sealed bags for further experimental analysis. In the following, B3, B4, B5, B6 and B7 represented the biochar produced at the temperatures of 300, 400, 500, 600 and 700 °C, respectively.
Analytical method
The ultimate analysis and proximate analysis of sewage sludge sample were analyzed in triplicate according to Ultimate Analysis of Coal (GB/T476-2001) and Proximate Analysis of Coal (GB/T212-2001). Heavy metals were measured using ICP-AES (USA Thermo Jarrell Ash Corporation), after 0.1 g of the sewage sludge sample, biochar and bio-oil was dissolved in a mixture of HCI (2.25 mL, 5 %), HNO3 (0.75 mL, 5 %) and HCIO4 (2 mL, 5 %), respectively (CJ221-2005). To better simulate the actual soil environment, an improved nitric acid–sulphuric acid method (HJ/T299-2007), the pH of extraction solvent was 5.0 and 6.0, respectively, was used to investigate the leaching toxicity of heavy metals in sewage sludge and biochar. A sequential extraction procedure, recommended by BCR [18], was used to determine the heavy metals chemical speciation distribution, and DTPA method (NY/T 890-2004) was chosen to determine the bio-available heavy metals contents in sewage sludge sample and biochar.
Statistic analysis
ANOVA was used to test ultimate analysis and proximate analysis results of sewage sludge, the heavy metals contents, bio-available heavy metals contents in sewage sludge and biochar, heavy metals concentrations in leachate of sewage sludge and biochar. The least significant difference (p < 0.05) test was applied to assess the differences among the means of three replications.
Result and analysis
Heavy metals contents in sewage sludge and biochar
As shown in Table 2, the contents of heavy metals in sewage sludge varied greatly, the sequence was Pb > Zn > Cu > Cd > Cr > Ni > As, among which the content of Pb reached 3740 mg kg−1, while the content of As was only 26 mg kg−1. It was obvious that domestic wastewater generally contained low heavy metals contents, whereas the discharge of industrial wastewater into urban wastewater treatment plants would dramatically increase the content of specific heavy metal in sewage sludge. Although the contents of heavy metals in various biochar were varying, which were still higher than that in sewage sludge, additionally, the tendency was the contents of heavy metals increased with the pyrolysis temperature (except Cd in B7). Previous researches also reported heavy metals accumulation in the biochar with the increase of pyrolytic temperature [10, 19]. Although Zn, Cu, Cr, Ni, and As contained in biochar met the control standards for agricultural use (CJ/T309-2009), the excessive quantities of Pb and Cd limited the biochar application as soil amendment.
The retention rate was defined as the ratio of heavy metal quantities in biochar and sewage sludge, the result showed that 90.4–98.3 % of Pb, 96.4–99.5 % of Zn, 92.5–99.3 % of Ni, 85.5–92.5 % of As, 81.5–94.5 % of Cu, and 70.0–87.5 % of Cr remained in biochar. Kistler et al. [17] reported the similar result, almost all the Cr, Ni, Cu and Zn remained in solid residues (biochar) over the temperature range of 350–750 °C. The heavy metals may exist as various mineral salts (carbonate, sulfate, chlorate, phosphate, etc.), sulfides, hydroxide and oxide in sewage sludge, among which mineral salts and hydroxide are generally converted into oxide or sulfides with better thermostability during reductive pyrolytic condition, thus a great proportion of the heavy metals remained in biochar. Correspondingly, 0.5–8.6 % of Pb, 0–3.1 % of Zn, 0–6.8 % of Ni, 6.1–12.4 % of As, 4.1–17.5 % of Cu, and 11.1–28.2 % of Cr were found in bio-oil. Similar contents of heavy metals were also detected in bio-oil from pyrolysis of sewage sludge in the previous research [20]. Thus, the recovery rates (the rate of the amount of each heavy metal detected in both of biochar and bio-oil, and that in sewage sludge) of Pb, Zn, Ni, As, Cu, and Cr was 98.8–99.0, 99.0–99.5, 99.0–99.3, 97.9–98.6, 98.6–99.0, and 98.2–98.6 %, respectively. Still a very small fraction of volatilized heavy metals was thought to remain in the cold surface of quartz tube and the first flask, therefore, syngas was regarded as heavy metals free.
Chemical speciation of heavy metals in sewage sludge and biochar
Heavy metals could be divided into exchangeable fraction (F1), reducible fraction (F2), oxidizable fraction (F3), and residual fraction (F4) according to BCR sequential extraction procedure. F1 has good mobility, which has direct eco-toxicity and bioavailability to environment. F2 refers to Fe and Mn oxides and hydrous oxide, F3 mainly refers to organic matter and sulphide, both of them have potentially eco-toxicity and bioavailability. F4 refers to minerals as quartz, feldspars, etc., which is stable in various environment, and has no eco-toxicity and bioavailability [18].
As shown in Fig. 2, for Cu, F3 and F4 fractions were the main forms, F1 and F2 fractions only accounted for small proportions, respectively, in sewage sludge. In B3, F1 disappeared, F2 and F4 were almost the same as that in sewage sludge, while F3 increased. In B4–B7, Cu existed as F3 and F4, F1 and F2 disappeared. Yuan et al. [21] also found that Cu existed as F1 and F2 was reduced in biochar, the high percentages of Cu existed as F3 was attributed the fact that the preference of Cu for organic matters. For Cr, The distribution was similar to Cu in sewage sludge, while that was different in biochar. In B3–B7, F1 disappeared, F3 and F4 were the main forms, while F2 still accounted for a very small fraction. Walter et al. [22] also showed over 95 % of Cr in sewage sludge existed in F3 and F4, because most of Cr was in the organic and residual phases [23]. Zn followed the order of F2 > F3 > F1 > F4 in sewage sludge. Although pyrolysis leaded to decrease of F1 fraction, still 1.4–11.2 % of Zn existed as F1, thus Zn in biochar had a direct eco-toxicity and bioavailability. Additionally, F2 fraction decreased while F3 fraction increased, therefore, Zn in biochar also had a potential eco-toxicity and bioavailability, especially in the oxidizing environment. Walter et al. also found high percentage of F3 fraction owing to the affinity of Zn for entering organic complexes [22]. As followed the order of F1 > F3 > F4 > F2 in sewage sludge. With the increase of pyrolysis temperature, F1 fraction in biochar gradually dropped off, thus the direct eco-toxicity and bioavailability of As declined, whereas As still had potential eco-toxicity and bioavailability, especially in reducing environment, because F2 fraction gradually rose, although F3 fraction decreased with pyrolysis temperature. Ni existed mainly as F3 form in sewage sludge. Compared with sewage sludge, F2 fraction dropped sharply, F3 fraction increased, while F1 and F4 fractions changed little in biochar. According to these results, it may be concluded that Ni in biochar had higher potential eco-toxicity and bioavailability in oxidizing environment than in reducing environment. Almost all Pb and Cd existed as F4 fraction in sewage sludge and biochar, which indicated that Pb and Cd were few eco-toxicity and bioavailability although Pb and Cd concentrations in sewage sludge and biochar exceeded Chinese sludge control standard for agricultural use (CJ/T309-2009). The above result showed that the chemical speciation distribution of heavy metals in biochar was largely determined by their distribution in sewage sludge. However, the eco-toxicity and bioavailability of heavy metal in biochar were relieved, as the loss of the mobile and easily available heavy metal, and the transformation of them into relatively stable forms during the pyrolysis process [21, 24].
Leaching toxicity of heavy metals in sewage sludge and biochar
Heavy metals concentrations (Table 3) in both leachate followed the order of Zn > Cu > Cr > Pb > Ni > As > Cd, which was different from the order of heavy metals concentrations in sewage sludge and biochar, the result indicated that the leaching toxicity was inconsistent with the content of heavy metal. Moreover, the heavy metals concentrations in biochar leachate were lower than that of sewage sludge leachate, owing to the transform of some heavy metals from easy-to-leach forms to hard-to-dissolve forms through pyrolysis [21, 24], and some loss of the mobile and easily available heavy metals during the pyrolysis process. The concentrations of heavy metals in leachate with pH of 5 and 6 were very low and similar, illustrating that heavy metals contained in biochar had similar immobility in acidic soil with pH of 5 and 6. The reason might be the flourishing pore structure and high BET surface area of biochar enhanced its ability to immobilize heavy metals [3, 15]. It has also been reported that compounds and functional groups of biochar might suppress the release of heavy metal through the formation of organometallic complex [4, 13, 16]. Hence, the leaching toxicity of heavy metals in biochar was lower than that in sewage sludge, which corresponded to the reduction of the eco-toxicity and bioavailability of heavy metal in biochar resulted from BCR sequential extraction procedure. However, the effect of pyrolysis temperature was limited, there was no significant difference among the concentrations of heavy metals in leachate of biochar produced at different temperatures except at 300 °C, because the temperature was not high enough to stabilize heavy metals through melting like the high temperature incineration [25, 26].
The leaching rate, defined as the ratio of the leachable heavy metal to the total content of each heavy metal, was employed to investigate the proportion of leachable heavy metal. The results are shown in Table 4. Obviously, the leaching rates of heavy metals in sewage sludge were much lower than that of biochar, which indicates converting sewage sludge into biochar significantly reduces heavy metals leaching toxicity. However, pyrolysis temperature exerted various effect on different heavy metal leaching rate. Specifically, For Pb, Ni, Cd and Cr, their respective leaching rates had no significant difference during the range of 300 and 700 °C. For Zn, the leaching rate had no significant difference during the range of 400 and 700 °C, however, it was lower than that of biochar produced at 300 °C. For As and Cu, both their leaching rates decreased with the pyrolysis temperature rising, which may ascribed to more transformation of As and Cu from easy-to-leach forms to hard-to-dissolve forms, and more loss of the mobile and easily available As and Cu with the increasing pyrolysis temperature, as discussed above.
Bio-available heavy metals in sewage sludge and biochar
Bio-available heavy metal was the heavy metal fraction which could be directly absorbed by plants, the DTPA extractable fraction has been widely used to estimate bioavailability of heavy metals in sludge [12, 13], due to its capacity to chelate a wide range of metallic elements. The bio-available heavy metal contents in sewage sludge and biochar are shown in Table 5, the changes of bio-available heavy metals contents reflected the transformations of chemical speciation of heavy metals during the pyrolysis process. The contents of bio-available Pb, Cd, Ni and Cr in biochar produced at different temperatures were similar (p < 0.05), respectively, the contents of bio-available Zn and As peaked in B3, and the content of bio-available Cu peaked in B6; hence, the pyrolysis temperature had inconsistent effect on the contents of bio-available heavy metals in biochar, which was similar to the result obtained by Hossain et al. [12]. However, it was consistent and obvious that biochar had lower contents of bio-available heavy metals than sewage sludge, indicating that pyrolysis process could repress the release of heavy metal in DTPA extractant, which was similar to the decrease of the leaching concentrations of heavy metals in biochar discussed above, hence the absorption of flourishing pore structure, high BET surface area of biochar on heavy metals, and the formation of organometallic complex could be used to explain the low bio-available heavy metals contents in biochar [4, 13, 16].
Conclusion
Biochar was prepared with sewage sludge by pyrolysis at the temperature range of 300 and 700 °C. A great percentage of heavy metals remained in biochar, the concentrations of heavy metals in biochar (except Cd in B7) were higher than that in sewage sludge, and the enrichment of the heavy metals in biochar enhanced with the pyrolysis temperature. The chemical speciation distribution of heavy metals indicated biochar had less eco-toxicity and bioavailability than sewage sludge, although it was largely determined by their chemical speciation distribution in sewage sludge. Although leaching toxicity of heavy metals in biochar was lower than that in sewage sludge, the effect of pyrolysis temperature was limited, the leaching toxicity of heavy metals in various biochar was similar to each other except in B3. Pyrolysis process also repressed the release of heavy metals in DTPA extractant, which indicated biochar had lower bio-available heavy metals contents than sewage sludge. Thus, biochar has low potential risk on soil and groundwater contamination when it is used as soil amendment, however, there are still some discrepancies between the experimental situation and the actual soil environment, the further farm experiment is necessary to verify the result.
References
Tian Y, Zhang J, Zuo W, Chen L, Cui Y, Tan T (2013) Nitrogen conversion in relation to NH3 and HCN during microwave pyrolysis of sewage sludge. Environ Sci Technol 47:3498–3505
Singh RP, Agrawal M (2008) Potential benefits and risks of land application of sewage sludge. Waste Manag 28:347–358
Agrafiotia E, Bourasa G, Kalderisb D, Diamadopoulos E (2013) Biochar production by sewage sludge pyrolysis. J Anal Appl Pyrol 101:72–78
Jin H, Arazo RO, Gao J, Capared S, Chang Z (2014) Leaching of heavy metals from fast pyrolysis residues produced from different particle sizes of sewage sludge. J Anal Appl Pyrol 109:168–175
Sohi SP, Krull E, Lopez C-E, Bol R (2010) A review of biochar and its use and function in soil. Adv Agron 105:47–82
Zhang LH, Xu CB, Champagne P (2010) Overview of recent advances in thermo-chemical conversion of biomass. Energy Convers Manag 51:969–982
Spokas KA (2010) Review of the stability of biochar in soils: predictability of O:C molar ratios. Carbon Manag 1(2):289–303
Peng X, Ye LL, Wang CH, Zhou H, Sun B (2011) Temperature- and duration-dependent rice straw-derived biochar: characteristics and its effects on soil properties of an Ultisol in southern China. Soil Tillage Res 112(2):159–166
Zhai Y, Peng W, Zeng G, Fu Z, Lan Y, Chen H, Wang C, Fan X (2012) Pyrolysis characteristics and kinetics of sewage sludge for different sizes and heating rates. J Therm Anal Calorim 107:1015–1022
Chun Y, Ji D, Yoshikawa K (2013) Pyrolysis and gasification characterization of sewage sludge for high quality gas and char production. J Mech Sci Technol 27:263–272
Velghe I, Carleer R, Yperman J, Schreurs S (2013) Study of the pyrolysis of sludge and sludge/disposal filter cake mix for the production of value added products. Bioresour Technol 134:1–9
Hossain MK, Strezov V, Chan KY, Ziolkowski A, Nelson PF (2011) Influence of pyrolysis temperature on production and nutrient properties of wastewater sludge biochar. J Environ Manag 92(1):223–228
Lu H, Zhang W, Wang S, Zhuang L, Yang Y, Qiu R (2013) Characterization of sewage sludge-derived biochars from different feedstocks and pyrolysis temperatures. J Anal Appl Pyrolysis 102:137–143
Hernande AB, Ferrasse JH, Chaurand P, Saveyn H, Borschneck D, Roche N (2011) Mineralogy and leachability of gasified sewage sludge solid residues. J Hazard Mater 191:219–227
He YD, Zhai YB, Li CT, Yang F, Chen L, Fan XP, Peng WF, Fu ZM (2010) The fate of Cu, Zn, Pb and Cd during the pyrolysis of sewage sludge at different temperatures. Environ Technol 35:567–574
Hwang IH, Ouchi Y, Matsuto T (2007) Characteristics of leachate from pyrolysis residue of sewage sludge. Chemosphere 68:1913–1919
Kistler RC, Widmer F, Brunner PH (1987) Behavior of chromium, nickel, copper, zinc, cadmium, mercury, and lead during the pyrolysis of sewage sludge. Environ Sci Technol 21:704–708
Quevauviller PH, Rauret G, Lópe-Sánchez J-F, Rubio R, Ure A, Muntau H (1997) Certification of trace metal extractable contents in a sediment reference material (CRM 601) following a three-step sequential extract ion procedure. Sci Total Environ 205:223–234
Méndeza A, Terradillosb M, Gascó G (2013) Physicochemical and agronomic properties of biochar from sewage sludge pyrolysed at different temperatures. J Anal Appl Pyrol 102:124–130
Yuan X, Leng L, Huang H, Chen X, Wang H, Xiao Z, Zhai Y, Chen H, Zeng G (2015) Speciation and environmental risk assessment of heavy metal in bio-oil from liquefaction/pyrolysis of sewage sludge. Chemosphere 120:645–652
Yuan X, Huang H, Zeng G, Li H, Wang J, Zhou C, Zhu H, Pei X, Liu Z, Liu Z (2011) Total concentrations and chemical speciation of heavy metals in liquefaction residues of sewage sludge. Bioresour Technol 102:4104–4110
Walter I, Martínez F, Cala V (2006) Heavy metal speciation and phytotoxic effects of three representative sewage sludges for agricultural uses. Environ Pollut 139:507–514
Wong JWC, Li K, Fang M, Su DC (2001) Toxicity evaluation of sewage sludges in Hong Kong. Environ Int 27:373–380
Huang H, Yuan X, Zeng G, Zhu H, Li H, Liu Z, Jiang H, Leng L, Bi W (2011) Quantitative evaluation of heavy metals’ pollution hazards in liquefaction residues of sewage sludge. Bioresour Technol 102:10346–10351
Koichiro K (1996) Melting system and reuse of products by arc processing. Waste Manag 16:423–430
Chen T, Yan B (2012) Fixation and partitioning of heavy metals in slag after incineration of sewage sludge. Waste Manag 32:957–964
Acknowledgments
This work is financially supported by the National 973 Project of China (2011CB201501), National Science and Technology Program of the Twelfth Five-year Plan for rural development in China (2012BAD14B16-04), National Natural Science Foundation of China (51161140330), Pearl River Nova Program of Guangzhou (2013J2200008), and Guangdong Science and Technology Program (2012B091400011).
Author information
Authors and Affiliations
Corresponding author
Rights and permissions
About this article
Cite this article
Lu, T., Yuan, H., Wang, Y. et al. Characteristic of heavy metals in biochar derived from sewage sludge. J Mater Cycles Waste Manag 18, 725–733 (2016). https://doi.org/10.1007/s10163-015-0366-y
Received:
Accepted:
Published:
Issue Date:
DOI: https://doi.org/10.1007/s10163-015-0366-y