Abstract
Enhanced oil recovery (EOR) process is used to recover the oil left in the reservoirs after primary and secondary recovery methods. Polymer material plays an important role in oil recovery by decreasing the mobility ratio and through the mechanism of disproportionate permeability reduction. However, the application of polymer flooding for oil recovery is limited in high-temperature and high-salinity oil reservoirs due to degradation of its molecules, thereby hindering the polymer EOR process efficiency. Recently, the oil and gas industry is adopting the application of nanotechnology to solve the problem plaguing the application of polymers for EOR. This has been attributed to the nanoparticles unique properties and availability in large quantities. The addition of nanoparticles into polymer solution has been reported to improve the rheological and shear properties, and lower the adsorption of polymers, thereby consequently enhancing oil recovery. This paper reviews the current trend of incorporating nanoparticles into polymers to improve polymer flooding process by explaining the mechanism involved, opportunities offered and challenges encountered. Technical solutions to overcome the challenges identified are also discussed.
Similar content being viewed by others
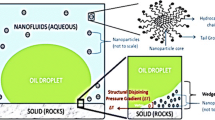
Explore related subjects
Discover the latest articles, news and stories from top researchers in related subjects.Avoid common mistakes on your manuscript.
Introduction
The recovery of oil from an oil reservoir is in stages namely primary, secondary and tertiary (or enhanced oil recovery) stage [1,2,3,4]. From studies, it has been noted that substantial amount of oil is left behind in the reservoir after the application of primary and secondary recovery techniques [5,6,7,8]. This is either because it is trapped by capillary forces (residual oil) or because it is bypassed in some other way. The bypassed oil arises due to reservoir heterogeneities or because of unfavourable viscosity ratio between the aqueous and oleic phase. The residual oil, on the other hand, is made up of discrete ganglia that are produced when a finger-like protrusion of the oleic mass forms a narrow neck by the combined effects of local pressure gradient and interfacial tension [9]. To ensure adequate recovery of the channelled, bypassed and residual oil, numerous EOR methods such as gas flooding [10,11,12], foam flooding [13, 14], surfactant flooding [15,16,17], and steam flooding [18,19,20] have been proposed.
Among all the EOR techniques, polymer flooding, a chemical EOR method has been adjudged to be the most promising because of its high efficiency, technical and economic feasibilities, and lower capital cost [21]. This EOR technique did not get much attention in the past due to inadequate understanding of its mechanism, unavailability of efficient chemicals, and low crude oil prices. Currently, polymer flooding is now attracting interest of researchers around the world due to depletion of conventional crude oil reserves, higher crude oil prices, technological advancements, and the availability of chemicals at relatively low price [21, 22].
The process of polymer flooding EOR technique involves adding high molecular weight water-soluble polymers to injection water. This results in an increase in the viscosity of the injected aqueous phase and leads to an improved recovery of bypassed and residual oil [23,24,25]. The bypassed oil is recovered through an improvement in the mobility ratio of the displaced fluid and disproportionate permeability reduction of water, while the residual oil is recovered through the viscoelastic nature of the polymers injected into the reservoir whose mechanism is as a result of stripping, pulling, oil thread or column flow, and shear thickening effects [26,27,28]. Polymer flooding has been successfully implemented in many oilfields either on a pilot scale or commercial scale for several decades. This includes the Daqing oil field in China, East Bodo Reservoir and Pelican Lake field in Canada, Marmul field in Oman, and Tambaredjo field, Suriname to mention just a few [29, 30]. In addition, polymer flooding has maintained its increasing importance to the current energy market [31]. The most notable contribution is the reported incremental oil production of up to 300,000 bbl/day from Daqing oil field in China [32].
Hydrolysed polyacrylamide (HPAM) is the most widely used polymer for EOR. HPAM is preferred in EOR field applications because it can tolerate the high mechanical forces present during flooding of a reservoir. Moreover, HPAM is resistant to bacteria attack, it has good water solubility, mobility control and it is a low-cost polymer [25, 33]. However, HPAM is very susceptible and sensitive to harsh reservoir conditions such as elevated temperature, high salinity, shear forces and hardness [23, 34, 35]. Its viscosity enhancement property is significantly reduced when it dissolves in high salinity or hardness brine, and elevated temperatures often encountered in reservoirs [35, 36]. To solve the problems plaguing the potentials of HPAM application in EOR, the oil and gas industry is adopting nanotechnology to help improve the efficiency of polymers during flooding operations and consequently the overall polymer EOR process [37].
Some reviews of the application of nanotechnology for EOR have been conducted in previous studies [13, 37,38,39]. Cheraghian and Hendraningrat [20] reviewed the application of nanotechnology in EOR by explaining the effects of nanoparticles on interfacial tension, wettability and core flooding. Bera and Belhaj [31] reviewed the application of nanotechnology in the oil and gas industry. They collected and summarised relevant works done in the past decade with particular emphasis on laboratory and simulation works. Sun et al. [32] reviewed the recent progress of the application of nanoparticles in EOR with focus on nanofluids and nanodispersions mechanisms and efficiency. Meanwhile, a comprehensive review of the application of nanoparticles for chemical EOR, especially polymer flooding process has not been conducted. Consequently, this is the first detailed review on the application of nanoparticles for polymer flooding. This review seeks to explain the mechanism and identify the challenges of nanoparticles application for polymer flooding. Trending opportunities and new developments in polymer flooding application for EOR through the formed hybrid suspension of nanoparticles and polymers were discussed and knowledge gaps highlighted. It is worthy to note that most of the works on the application of hybrid suspension of polymers and nanoparticles are still in laboratory and pilot scale.
Limitations of polymer flooding EOR
The polymers that have been tested for use in polymer flooding are classified into two major categories namely Biological polymers and synthetic polymers. Both have been found to be effective in improving mobility ratio and reducing the relative permeability in porous media [42, 43]. However, the field application of biological polymers such as xanthan gum is limited because of high bacterial degradation. Thus, synthetic polymers such as polyacrylamides are widely used for field applications of polymer flood as can be inferred from Table 1 [22, 44]. This partly explains the reason why research works of the application of nanotechnology to improve polymers used for flooding processes have focussed more on the use of acrylamide-based polymers.
When used during polymer flooding, polyacrylamides have undergone partial hydrolysis which converts some of the amide groups (CONH2) to carboxyl groups (COO−) as illustrated in Fig. 1. The molecule has a flexible chain structure known as a random coil and since it is a polyelectrolyte, it interacts with ions in solution. Typical degree of hydrolysis (DOH) for this polymer is 15–35% of the acrylamide (AM) monomers. The DOH accounts for many of the physical and rheological properties of the polymer solution such as adsorption, viscosity and water solubility [36]. Two major factors that influence the performance of HPAM solution properties are the injection and formation salinity, and temperature. Other factors that impact the polymer solution negatively are pH, shear rate and the presence of contaminants such as oxygen and iron [22, 64].
Structure of polyacrylamide (PAM) and partially hydrolysed polyacrylamide (HPAM) [65]
Salinity effect
Polymers such as HPAM are polyelectrolytes with multiple charges distributed along their chain [66]. The viscosity property of this polyelectrolyte solution is sensitive to solution salinity and hardness commonly encountered in reservoir formation brines [9]. Polymers tend to conform when in solution and the average conformation of a typical flexible HPAM is spherical. However, it can change conformation, and hence overall size, quite readily [67]. To better explain the effect of salt concentration on HPAM viscosity, it is necessary to consider the interaction between the fixed charges along the polymer chain and the mobile ions in solution.
In ionic solution, charged bodies are surrounded by a cloud of oppositely charged ions (counter ions) coulombically attracted to each other, and consequently, an electric double layer is formed. The thickness of the double layer formed is inversely proportional to the ionic strength. Thus, in solutions of lower salt concentration, repulsion between the similar charged bodies will be greater, and the polyelectrolyte will expand because of the mutual repulsion of the charges along the chain [68]. On the other hand, at high salt concentration (such as NaCl), the carboxylate group (–COO−) on the backbone of the polymer is surrounded by the Na+ cations, which shield the charge. Then, –COO− group is reduced, the thickness of the electric double layer decreases, the hydrodynamic volume of the polymer becomes smaller, the flexible polymer contract (see Fig. 2b), and finally the viscosity of the polymer is reduced (see Fig. 3) [69, 70].
Effect of salt on the hydrodynamic size of polymer molecule [22]
Effect of increased salt concentration on viscosity of polymer solution [69]
The presence of divalent ions such as Ca2+ and Mg2+ will be more significant due to their increased electric charge. They bind tightly to the –COO− on the backbone of HPAM. They reduce the viscosity of the polymer solution even further as seen in Fig. 4 and may result in precipitation of the polymer from solution [64, 65].
Effect of salt concentration on viscosity of polymer solution [71]
Temperature effect
HPAM is thermally stable at low temperature [72]. At high reservoir temperature, the pendant amide group present in the polymer backbone tend to hydrolyse [73]. A high degree of hydrolysis (DOH) is not desirable as precipitation of the polymer in the presence of divalent ions occur [74]. In cases where there is no precipitation, strong interactions of cations with the carboxylate group of the polymer lead to a reduction in the viscosity of HPAM. Elevated temperature causes a higher DOH, thereby resulting in significant changes in solution properties, rheology, and stability of the polymeric system and ultimately phase separation occurs [22, 66, 70, 75]. Davison and Mentzer [76] performed the viscosity measurements of several polymers in a sealed capillary viscometer at 90 °C to screen their application for North Sea oil fields. Though most of the polymer recorded viscosity loss, the viscosity of polyacrylamide was observed to be particularly poor at high temperature and precipitation of the polymer was observed (see Fig. 5).
Thereafter, the mechanism of the precipitation of the polyacrylamide solution was studied via Raman spectroscopic analysis of the heated solution which shows the hydrolysis of the amide group on the backbone of the polymer to carboxylate group. Seright et al. [77] corroborated the hydrolysis of amide side groups on the backbone of partially hydrolysed polymer at elevated temperatures. Besides, they asserted that the precipitation of HPAM noticed in previous researches was due to the presence of divalent cations. They claimed that in the absence of dissolved oxygen and divalent cations, their experiment shows that the polymer backbone can remain stable for longer periods.
Other factors
High shearing conditions occasionally encountered during: (1) the mixing of polymer solution in tanks, (2) conveyance of the polymer solution in pumps, chokes and valves, and (3) the injection of polymer solutions, ruptures the polymer chains leading to a loss in viscosity and consequently efficiency of the polymer flooding operations [78]. As depicted in Fig. 3, HPAM is sensitive to shear degradation [9, 79, 80]. pH is another factor that influences the behaviour of polymer solutions during their use in flooding operations. Lower pH causes carboxyl group present in the backbone of HPAM polymer solution to be protonated. Hence, the polymer associates the hydrogen ion (H+) to form an electrostatic repulsion of the intermolecular polymer [81]. Subsequently, effective screening of the polymer –COO− groups by the H+ reduces the electrostatic repulsion within the polymer chain and the intermolecular action becomes weak. Consequently, a tightly coil conformation of the HPAM molecules occurs, thus resulting in a decrease in the polymer viscosity [36, 82]. Furthermore, the presence of contaminants impacts the viscosity and rheological behaviour of polymer negatively [73, 83]. The contaminants such as dissolved oxygen and iron (specifically Fe3+) often lead to oxidative degradation of HPAM polymer [73]. Shupe [84] and Yang and Treiber [85] in their respective studies of the chemical stability of polyacrylamide solution reported that the rate and extent of polymer degradation were governed by the oxygen content of the solution, although they remarked that limited level of oxygen produced only limited degradation. The contaminants attack the polymer and reduce their viscosity and chemical stability (see Fig. 6a, b).
a Effect of Oxygen on HPAM stability at (1) low level of oxygen, (2) air, and (3) high oxygen level b effect of Fe3+ on HPAM viscosity [70]
Hybrid of nanoparticle and polymer solution application for improving polymer flooding defects
Though it is never required that the polymer is stable indefinitely, however, it must simply last long enough to be effective on the timescale of the oil recovery mechanism through which it is operating [9]. Several methods have been proposed in the past to improve the behaviour of acrylamide polymer to improve their efficiency in high temperature and high salinity reservoirs and prevent the shear degradation of their molecules. This includes development of new salt tolerant polymers [86,87,88,89,90]. Kamal et al. [22] provided a thorough review of newly developed polymers for EOR application. While some were unsuccessful for EOR applications, others were found to be uneconomical [22]. Advancement in research and new trends in polymer EOR involves the addition of nanoparticles to polymers used during flooding operations to improve polymer EOR process efficiency [26]. Nanoparticles, also referred to as “engineered nanomaterial,” are the collections of atom bonded together with sizes ranging from 1 to 100 nm [32, 91]. Generally, the application of nanoparticles for EOR has been found to possess the ability to improve the overall oil recovery factor due to their unique properties [92]. The unique properties resulted from their small sizes and greater surface area per unit volume as shown in Fig. 7 [32]. These properties include thermal properties like heat transfer, and properties of mechanical strength like ultra-high strength of material [40].
Schematic of increase in surface area with decreasing particle size [41]
Initially, most researches of the effect of nanoparticles on oil recovery have been focussed on the application of nanofluids (i.e. nanoparticles in brine) to enhance oil recovery with varying degree of success [93,94,95,96,97]. By expansion of nanotechnology, scientists have discovered that appropriate addition of nanoparticles into polymers can lead to high-performance polymer characteristics, such as enhanced viscosity and rheological behaviour and improved thermal and chemical resistance beyond what traditional polymeric materials exhibit [98,99,100]. The interaction of nanoparticles with polymer has opened routes for the tuning of the rich phase behaviour of these systems as well as formation of new hybrid functional materials, which are being employed in a wide range of applications [101, 102]. The formed hybrid suspension (nanopolymer) made from the mixture of nanoparticles with polymers have been found to exhibit and display enhanced properties such as improved mechanical, optical, thermal, and other properties [103,104,105]. Thus, the oil and gas industry is tapping into the potential of nanoparticles to improve polymer flooding defects in harsh conditions [106].
The combination of nanoparticles and polymer system individually interact through several forces depending on the solution conditions. Their interactions govern the properties of the hybrid solution formed and at the same time dictate the phase behaviour of their complex system. The control over interaction parameters basically enables the integration of the two components, tuning of their properties, and implementation of complex structures for desired functions [107]. Using small-angle neutron scattering (SANS) and dynamic light scattering (DLS), Kumar et al. [94, 95] investigated the behaviour of nanoparticles polymer system and concluded that the resultant phase behaviour is governed by an interplay of attractive and repulsive forces. The commonly present interactions between nanoparticles and polymers in solution are [108,109,110]: (1) electrostatic and van der Waals interaction, (2) steric repulsion, (3) hydrogen bonding and (4) hydrophobic interaction.
Mechanism of improved oil recovery by nanoparticle-induced polymer
The mechanism of improved polymer performance and consequently oil recovery of nanoparticle-induced polymer flooding process has been attributed to the ability of nanoparticles to prevent degradation and improve the viscosity of the polymer molecules in the presence of salt and temperature. In the absence of nanoparticles, the cations of the salt (e.g. Na+, Ca2+, Mg2+) present in injection or formation water attacks the oxygen atom on the surface of the anionic polyelectrolyte by electrostatic attraction due to the opposite charges on their surfaces (see Fig. 8). Also, an interaction occurs between the amino group (NH2) and cations, but this reaction is less important compared to the interaction between the cations and oxygen. As compared to the monovalent cation Na+, the divalent cations Ca2+ and Mg2+ have a higher charge density; thus, they have a stronger ion–dipole interaction with the amide group. Strong ion–dipole interaction between the divalent cations and the amide group weakens the bond strengths of the N–H and the C=O bonds. This results in shrinking of the polymer molecules, formation of precipitates and a reduction in solution viscosity of the polymers [111, 112].
Ion–dipole interactions between cations of salt and oxygen of polymer solution [112]
The reaction is not the same for polyacrylamide solution containing nanoparticles. According to Maghzi et al. [112], when nanoparticles are added to polyacrylamide solution in the presence of saline water containing either monovalent or divalent ion or both, an ion–dipole interaction is generated between the cation of the salts and the oxygen atom on the surface of the nanoparticles (e.g. SiO2) as shown in Fig. 9. Therefore, the attack of cations on polymer molecules is decreased by the shielding effect of the nanoparticles, and the decrease in viscosity of polymer solution is not observed in the presence of the SiO2 nanoparticles used.
Ion–dipole interactions between cations of salt and oxygen of polymer solution in the presence of nanoparticles [112]
Similarly, Hu et al. [113] in their research work on the rheological properties of partially hydrolysed polyacrylamide seeded by nanoparticles observed improved rheological properties of HPAM in the presence of SiO2 nanoparticles. Using Fourier transform infrared (FTIR) spectra data, the improved rheological properties of the polymers in the presence of nanoparticles are attributed to the formation of a hydrogen bond between the carbonyl groups in HPAM and the silanol functionalities on the surface of SiO2 nanoparticles (see Fig. 10). The experimental works of Zhu et al. [114, 115] and Maurya and Mandal [116] corroborated this opinion.
FTIR spectra of SiO2 nanoparticle and HPAM polymer solution [113]
Furthermore, Barati et al. [117] suggested that the higher incremental oil recovery for a polymer/nanoparticle system may be explained by two dominant reasons. Firstly, they opined that since nanoparticles are tiny enough to readily move through the small pores, their presence during polymer flooding will cause the nanoparticle-augmented polymer solution to have less retention in porous media compared to ordinary polymeric solution. This practically means that nanoparticles compensate the inaccessible pore volume phenomenon which reduces polymer solution injection efficiency. Secondly, they alluded the incremental oil recovery to improved rheological behaviour of polymer solutions in the presence of nanoparticles.
Summarily, the hybrid suspension of polymer and nanoparticles indubitably improves the polymeric fluid property. The mechanism of improved oil recovery by nanoparticle-induced polymer involves a combination of ion-exchange reaction, shielding effects of the nanoparticles on the polymer, formation of a stronger bond between the hydroxyl group of the nanoparticle and amide group of the polymer, and finally, the scalable and quantum effects of the nanoparticles which grant them easy access to flow through inaccessible pore volumes of porous media.
Review of the effects of nanoparticles on polymers
Hitherto, several experimental investigations for the application of hybrid of nanoparticles and polymers for EOR have focussed on silica (SiO2) nanoparticles because SiO2 are highly stable, possess high specific surface area, largely available, and can work effectively in the presence of other molecules. To this extent, SiO2 nanoparticles are being widely regarded as the benchmark nanoparticle for application in the oil and gas industry. Recently, other various nanomaterials have been considered and are studied as additives to polymer for EOR applications. This includes non-metallic oxide nanoparticles such as nanoclay, carbon nanomaterials (e.g. carbon nanotubes and graphene), and metallic oxide nanoparticles such as alumina (Al2O3), iron oxide (Fe2O3), and titanium oxide (TiO2). According to the various studies carried out on hybrid suspension of nanoparticles and polymers for EOR, the addition of nanoparticles brings about an improvement in wettability alteration, shear stability, rheological and viscosity behaviour, and lower adsorption, thereby resulting in higher oil recovery of the polymer flooding process [26, 118,119,120].
Adsorption
Cheraghian et al. [121] conducted an adsorption experiment of polymer on reservoir rock and investigated the role of clay and SiO2 nanoparticles in the adsorption of water-soluble polymers onto the solid surfaces of carbonate and sandstone rocks. The results obtained from static adsorption experiments shows that nanoparticles play a major role in the case of polymer molecules adsorption on rock surface. Polymer solutions containing nanoparticles were found to have less adsorption based on weight per cent than similar samples of ordinary polymer solution. This ultimately led to a higher oil recovery as the polymer rheological and viscosity behaviour were not impacted negatively.
Similarly, Khalilinezhad et al. [122] and AlamiNia and Khalilinezhad [123] evaluated static adsorption of nano-SiO2-polymer suspension on sandstone rock. The adsorption values from their experiments were matched using polymer adsorption model in University of Texas Chemical Compositional Simulation (UTCHEM) software, and the adsorption isotherm was generated. They reported ultra-low values of adsorption of nanopolymer suspensions compared to conventional HPAM solution. Bagaria et al. [124] studied the stability and transportation behaviour of iron oxide nanoparticles in the presence of poly(2-acrylamido-2-methylpropane sulfonate-co-acrylic acid) (AMPS–co–AA). Due to the steric stabilisation between the nanoparticle and polymer, the hybrid dispersion formed displayed colloidal stability in standard American Petroleum Institute (API) brine (8 wt% NaCl + 2 wt% CaCl2) at 90 °C for 1 month and resist undesirable adsorption on silica surfaces.
Wisniewska et al. [125] examined the adsorption interaction of Al2O3 and anionic polyacrylamide at different temperature (15–35 °C), pH (in the range 3–9), and carboxyl groups’ content in the PAM chains (in the range 5–30%) via turbidimetry method. The polymer adsorption process causes changes in the thermal stability of the examined systems. Besides, the adsorption of anionic polyacrylamide decreases with rising pH. At lower pH 3, adsorption proceeds due to hydrogen bond formation and slight electrostatic attraction for all examined temperatures. Meanwhile, stronger electrostatic interaction between the positively charged Al2O3 surface and negatively charged polymer surface accounts for the adsorption at pH 6. The smallest polymer adsorption is recorded at pH 9 as a result of repulsion between the polyacrylamide chains and Al2O3 nanoparticles. Finally, they observed that large amounts of polyelectrolyte are adsorbed when the content of anionic carboxyl group and temperature are high [125, 126].
Thus far, these are the available studies of the influence of nanoparticle on decreasing the adsorption of polymer molecules in porous media. Though the result of the researches was positive and insightful, nonetheless, the researches have some shortcomings. The rock samples used in the experiments lack clay mineral contents. The lack of clay mineral content in the porous media means that the rock samples studied are not representative of real reservoir rock system (unconsolidated). Moreover, there has been no study to evaluate the dynamic retention of the nanopolymer suspensions in porous media. Thus, the researches available are not enough to generalise the efficiency and effectiveness of the adsorption behaviour of nanopolymer suspensions. Further studies of the static adsorption and dynamic retention behaviour of nanopolymer system in sandstone rocks, carbonate rocks, dolomite, mixture of some proportion of sandstone and carbonate rocks and other rock samples which are representative of real reservoir rocks are required to ascertain and understand the adsorption behaviour of nanopolymer suspensions in porous media.
Wettability alteration
Nanoparticle-induced polymers were also found to alter wettability of porous medium. It is well documented that oil recovery is affected by wettability of porous medium with more oil been recovered from water-wet compared to oil-wet reservoirs [127, 128]. In their experiment of the pore scale monitoring of wettability alteration by silica nanoparticles during polymer flooding of heavy oil, Maghzi et al. [129] injected polyacrylamide and DSNP solution in a five-spot glass micromodel containing heavy oil. They measured the contact angles of the glass surface at different states of wettability after coating by heavy oil, distilled water, polyacrylamide solution, and DSNP solution using sessile drop method. The results of the sessile drop experiment showed that coating with heavy oil makes an oil-wet surface, coating with distilled water and polymer solution partially alters the wettability of the surface to water-wet, and coating with DSNP solution makes a strongly water-wet surface. By altering the wettability, an improved oil recovery factor of 10% was observed by DSNP compared to ordinary polyacrylamide solution.
Zheng et al. [130] performed an experimental study of the wettability alteration of nano-SiO2 during nanopolymer flooding for oil recovery. The surface of the nano-SiO2 used in this experiment was coated with hexamethyldisilazane and hexadecyltrimethoxysilane to make them partially hydrophobic. Thereafter, the contact angle measurements of the suspension were conducted using a drop shape analyser. It was observed that the contact angle of the system decreases and the oil-wet system was rendered water-wet. Consequently, the oil recovery of the system improved compared to the conventional polymer system. Furthermore, Yousefvand and Jafari [118] and Sedaghat et al. [131] observed that hybrid suspension of nanopolymer system resulted into wettability alteration of an oil-wet micromodel into partially water-wet and water-wet using SiO2 and TiO2 as the nanoparticles in the injected nanopolymer suspension.
Finally, Nezhad et al. [132] confirmed that the use of multi-walled carbon nanotube with polyacrylamide-graft-lignin copolymer led to a wettability alteration of glass surface from oil-wet to water-wet condition. However, it was noted that all the wettability experiments of nanopolymer suspensions were carried out on glass surfaces. More experiments of wettability alteration effects of nanopolymer suspension on rock surfaces typical or representative of real reservoir rocks are required to ascertain the authenticity of the wettability alteration claim of the nanopolymer system.
Rheology and viscosity
Maghzi et al. [111] performed a series of polymer flooding experiments in a quarter five-spot glass micromodel saturated with heavy oil to investigate the role of nanoparticles on improvement of polymer solution performance in the presence of salt. To examine the effects of silica nanoparticles on the polyacrylamide performance in the presence of salts during polymer flooding of heavy oil, solutions of polyacrylamide and DSNP with different salinities are used as injectant during the flooding experiments. Viscosity measurements were performed to analyse the results of the polymer flooding tests and the oil recovery values were measured at different salinities via analysis of the continuously captured images during the displacement tests. The results of the experiment showed that viscosity of silica nanosuspension in polyacrylamide was higher than that of polyacrylamide solution at the same salinity and the increase in viscosity becomes more noticeable by increasing the silica nanoparticles concentration. In addition, oil recovery decreases by increasing the salt concentration during polyacrylamide flooding, whereas, in the case of flooding with suspension of silica nanoparticles in polyacrylamide, a higher oil recovery factor was observed [112].
Rezaei et al. [133] conducted an experimental investigation of the effect of Surface Modified Clay Nanoparticles (SMCN) on rheological behaviour of HPAM solution, its resistance against increase in temperature and salinity and its ability in improving polymer flooding. In this research, surface of certain clay nanoparticles specifically montmorillonite (MMT) nanoclay were modified by specific tetrahedral redundant located between polymer chains to prevent deformation of polymers. The MMT crystal structure used is made up of a layer of aluminium hydroxide octahedral sheet sandwiched between two layers of silicon oxide tetrahedral sheets (see Fig. 11).
Schematic illustration of MMT crystal structure [133]
Tetraethylammonium chloride was chosen by the authors to modify the surface of the nanoscale MMT plates through ion-exchange reaction. This is to ensure MMT compatibility with HPAM, and to increase dispersal of nanoplates through the solution, and consequently to improve solution stability. A concentration of 0.1 wt% was found to be the optimum concentration of SMCN which results in an excellent rheological behaviour and stable solution. The location of the clay nanoparticles between the polymer chains was found to cause an increase in the viscosity of the solution and enhance the polymer solution resistance against temperature, salinity, shear stress and mechanical degradation. Results of the core flooding test confirm that the SMCN particles could improve HPAM flooding and increase oil recovery factor by 33% more than ordinary HPAM flooding (see Fig. 12).
Oil recovery versus pore volume injected for water flooding, polymer flooding and nanoparticle-induced polymer flooding [133]
Using experimental and numerical simulations, Khalilinezhad et al. [134] investigated the effects of nanoparticles on flow behaviour of polymer solution in porous media. They performed core scale simulation of polymer flood, nanoparticle-assisted polymer (NAP) flood, and sensitivity analysis of NAP flood with respect to divalent cations concentration. The nanoparticles used in the study were clay and silica nanoparticles. The simulation model was validated against well-controlled laboratory experiment to have reliable predictions of the full-field implementations. The results showed that the amount of polymer adsorption is lower, and the rheology and viscosity of the polymer solution were improved when clay or silica nanoparticles is present in the injectant. The result of sensitivity analysis demonstrates that polymer molecules are more degradable during polymer flooding compared to NAP flooding. Based on the validated model, three-dimensional simulations of NAP field pilot were performed and the results revealed that the cumulative oil recovery, water cut and breakthrough time improved when the injectant has some dispersed nanoparticles.
Hu et al. [113] developed a novel aqueous hydrolysed polyacrylamide-based SiO2 nanocomposites for enhanced oil recovery, and their rheological properties were investigated under different salinities, temperature, and ageing times. Results from their experiment show that the inclusion of silica nanoparticles significantly increased the viscosity properties of acrylamide-based polymer especially under high temperature and high salinities (see Fig. 13). Also, it was found that the hybrid suspension showed an impressive thermal stability compared to ordinary polymer solution. Oscillation test of the experiment indicated that seeding the polymer solution with nanoparticles remarkably facilitated cross-links among polymer molecules and made the hybrid solution formed more elastically dominant. Details of these researches and others are summarised in Table 2.
Viscosity versus a temperature and b salinity concentration, for polymer and nanoparticle-induced polymer [113]
Our research group appraised the impact of Al2O3 and TiO2 nanoparticles on the rheological and core flooding properties of HPAM. The rheological properties of the Al2O3–HPAM and TiO2–HPAM hybrid dispersions in different electrolyte solutions characteristic of monovalent and divalent ions was examined at low and medium shear rates. Thereafter, core flooding experiment in glassbead pack was conducted study the oil displacement. 0.1 wt% was determined as the optimal nanoparticles concentration that yielded the highest viscosity of the hybrid dispersion on rheological characterisation. Moreover, the presence of the nanoparticles in the hybrid dispersion shielded the polymer and minimised their degradation in the presence of synthetic reservoir brines. A higher oil recovery of 10% and 5% was recorded for the hybrid dispersions of Al2O3–HPAM and TiO2–HPAM, respectively, compared to conventional HPAM molecules [135].
Cheraghian et al. [136] evaluated the role of TiO2 nanoparticles on polymer viscosity and the consequent oil displacement efficiency of the hybrid dispersion in synthetic field brine of 20,000 ppm containing varying proportions of monovalent and divalent ions. The TiO2–HPAM nanopolymer was reported to exhibit an outstanding flow behaviour at 0.001–100 s−1 shear rates considered. Additionally, a 4% incremental recovery of heavy oil compared to base polymer was recorded after one pore volume injection of fluid in medium-permeability sandstone cores. Furthermore, Kumar et al. [137] used TiO2 nanoparticles to reduce homoaggregation and rate of sedimentation of synthesised oilfield nanocomposite. Results from scanning electron microscopy (SEM), electrical conductivity and ultraviolet visible spectroscopy (UV–vis) confirmed that the presence of TiO2 nanoparticles enhanced the dispersion stability for more than 10 weeks and led to an improved rheological behaviour of the nanocomposite. Hence, TiO2 was proffered for application in EOR.
Lima et al. [138] examined the effect of carbon black (CB) nanoparticle on rheological properties of HPAM under high salinity and high-temperature conditions because of the excellent chemical and thermal stability of the nanoparticle additive. Due to its poor dispersibility in polar solvents, the CB surfaces were sequentially modified with ethylenediamine. Transmission electron microscopy (TEM) and atomic force microscopy (AFM) confirmed the formation of oligomers on the CB, ethylenediamine and acrylamide surfaces. At 0.6 mol/L ionic strength and 70 °C temperature, the nanopolymer formulated exhibited 50% higher viscosity than the conventional polymer itself. Besides, the hybrid dispersion was more stable under static conditions, thereby showing strong potentials for EOR. Similarly, Kadhum et al. [139] formulated an excellent nanopolymer using multi-walled carbon nanotube dispersions which demonstrated a high stability in standard API brines and high-temperature condition (80 °C).
To avoid the time consumption and high expense involved in the selection and synthesis of appropriate structural polymer for EOR, Nguyen et al. [140] investigated the effect of graphene oxide (GO) nanoparticles as an additive for viscosity stabilisation of HPAM polymer for high-temperature applications. Field electron scanning electron microscopy (FESEM) images of the samples showed good distribution of the GO’s in the polymer network resulting from the integration of hydrophilic polymer chains and the functional group in the GO’s surfaces. The addition of the GO additives resulted in improved stability of diluted polymer/seawater solutions aged at reservoir conditions for 31 days.
Other researchers such as Cheraghian et al. [119], Zheng et al. [130] and Zhu et al. [114, 115] also discussed the improvement in rheological behaviours of polymeric solutions through the addition of nanoparticles. Nevertheless, a critical assessment of the viscoelastic properties of the nanopolymer suspension formed has not been investigated. This is necessary to ascertain the effect (if any) of the nanoparticles on the viscous and elastic modulus of the polymer solution. This is of paramount importance because viscoelasticity of polymer solution is a major mechanism proposed for the microscopic displacement of efficiency of polymers for EOR [141,142,143].
Shear stability
To address the problem regarding poor shear resistance of commonly employed polymers for oil displacement, Lai et al. [78] performed copolymerisation of nano-SiO2 with acrylic acid (AA) and acrylamide (AM) polymer to obtain hydrolysed polyacrylamide nano-SiO2 (HPMNS) suspension. After shearing with Mixing Speed Governor and porous media shear model, the formulated HPMNS was found to have a good shear resistance and a higher oil recovery compared to HPAM.
Zhu et al. [114, 115] formed nanopolymer hybrid suspension by introducing silica nanoparticles into hydrophobically associating polyacrylamide (HAPAM) solution. They investigated the effect of the silica nanoparticles on the shear resistance and rheological behaviour at high temperature (85 °C) and high salinity conditions (33,000 ppm). They observed that the shear viscosity of the hybrid nanopolymer suspension increases with the addition of silica nanoparticles and they exhibited a better shear resistance than the corresponding HAPAM solution. Moreover, the rheological properties of the nanoparticle-induced polymer were observed to be higher and an incremental oil recovery factor of almost 50% was recorded.
Rezaei et al. (2016) studied the shear degradation of nanopolymer suspension at typical reservoir shear rates and the experimental data was validated with power law model. The results show that by adding clay nanoparticles to polymer solution, the pseudoplasticity and shear-thinning behaviour of the polymer was improved, especially a low shear rates. They concluded that in addition to preventing the shear degradation of polymers, the presence of the clay nanoparticle in the nanopolymer suspension enhances the resistance of the polymer solution to mechanical degradation.
Major challenges of application of hybrid suspension of polymer and nanoparticles
Stability of nanoparticles
Though the numerous researches of the application of nanopolymer suspension for EOR were quick to point out an increase in the viscosity of the polymer caused by the presence of NPs, most of the researches toned down on the strong tendency for the NPs to agglomerate and aggregate at HTHS typical of reservoir conditions due to electrostatic and other interaction forces, thereby limiting the optimisation of the NPs efficiency for the polymer flooding process [101, 148, 149]. Agglomeration is the sharing of a plane or side between two particles, while aggregation indicates one-point linking of particles. The strong tendency to agglomerate and aggregate is caused by the great differences in the properties of polymer and NPs, especially the incompatibility of the NPs with the organic medium, thereby leading to phase separation at HTHS conditions. The phase separation means that the so-called NP filled polymer will contain a number of loosened clusters of particles and they are likely to exhibit properties worse than the conventional polymer system [150]. In fact, Zeyghami et al. (2014) questioned the applicability of nanoparticles as an additive for solutions of high molecular weight polymer at HTHS. It is noteworthy that the interfacial interaction between the two phases (NP and polymer) present in the hybrid solution formed is the most decisive factor affecting the properties of the resulting materials. Thus, the dispersion of the nanometre-sized particles in the polymer matrix has a significant impact on the properties of the nanopolymer solution [149]. Zheng et al. [130] achieved the stability of SiO2 NP dispersion for 25 days only by using modifiers. However, offshore oil recovery operations often require longer periods. Thus, the stability of nanoparticles remains a challenge of nanopolymer application that must be tackled.
Scaling-up of nanoparticles for real field application
Laboratory results presented in several studies have shown that hybrid suspension of polymer and nanoparticles increases the recovery of oil with viscosities ranging from light to medium and heavy crude oil. Despite the large number of publications on the evaluation of nanoparticles for chemical EOR, the scale-up of nanoparticles for real field applications remains a challenge and constitute a major obstacle for field trials. Another challenge of the application of this method as an EOR technique lies in developing cost-effective and cost-efficient techniques for the large-scale production of nanoparticles for field application.
Technical solutions
-
To optimise the efficiency of nanoparticle in polymer solution especially at HTHS, there is the need to keep the nanoparticles dispersed in the polymer medium. This can be achieved by surface modification of the nanoparticles during their application for the polymer flooding process [150, 151]. Two methods exist to achieve the surface modification of nanoparticles in the literature, namely the modification by chemical interaction and physical modification process. The chemical treatment method involves modification with either modifier agents such as the silane coupling agents which possess hydrolysable and organofunctional ends, while the surface modification of NPs based on physical interaction is usually implemented by adsorption of surfactants or macromolecules onto the surface of NPs [150].
-
More technological research into efficient ways for large-scale application of nanoparticles should be devised. Moreover, future research should focus on investigating the use of nanoparticles from cheaply available resources such as nanoclay.
Conclusion and recommendations
This paper presents the new trend of nanotechnology application for improving polymer flooding defects and oil recovery. Hybrid suspension of polymer and nanoparticles indubitably holds promising features for EOR processes. The mechanism involved in the formation, opportunities offered and major challenge likely to be encountered by the hybrid suspension of the nanoparticle-induced polymer suspension were reviewed. In nearly all the experimental researches, it was observed that the addition of nanoparticles resulted in an improved rheological behaviour, wettability alteration of rock system, lower adsorption on rock surface, and an improved shear stability of the polymer. Consequently, this resulted in a higher oil recovery of nanoparticle-induced polymer flood compared to ordinary polymer flooding in all the scenarios considered. But more studies are required to better understand and optimise the potentials of the nanopolymer suspension for EOR. To actualise the eventual field implementation of nanopolymer suspension for EOR, we highlight the areas of emphasis that needs to be addressed below.
-
Firstly, the transport behaviour of nanopolymer suspension is lacking in studies and needs to be investigated. This is necessary to understand the propagation of the suspension in reservoirs of different porosity and permeability. Moreover, an understanding of their transport mechanism will help prevent physical filtration, a phenomenon where the particles are larger than some of the pores in the porous media.
-
Secondly, a comprehensive understanding of the adsorption behaviour of the nanopolymer suspensions in the presence of clay mineral content is required. Most oil reservoirs contain specific amount of clay mineral content and types ranging from montmorillonite to kaolinite and illite. This clay materials and contents are known to adsorb macromolecules which can lead to a reduction of the efficiency of injected chemicals and consequently increase the overall cost of the EOR process.
-
Thirdly, the flooding application of nanopolymer suspension in reservoir rock system other than sandstone has neither been investigated nor presented in studies. An evaluation of their suitability and applications in carbonate rocks, dolomite, and other reservoir rock system containing oil deposits will prove beneficial for EOR.
-
An accurate modelling of the hybrid suspension of nanoparticle and polymer is needed for their large-scale full-field implementation. This will help operators in the design and execution of field projects and stand as a guideline for extending polymer flooding technology.
-
Finally, the possibility of tuning the morphology of the nanoscale materials to nanofibrils for EOR applications should be considered for future research.
References
Alvarado V, Manrique E (2010) Enhanced oil recovery: an update review. Energies 3:1529–1575. https://doi.org/10.3390/en3091529
Muggeridge A, Cockin A, Webb K et al (2014) Recovery rates, enhanced oil recovery and technological limits. Philos Trans A Math Phys Eng Sci 372:20120320. https://doi.org/10.1098/rsta.2012.0320
Sheng JJ (2014) A comprehensive review of alkaline–surfactant–polymer (ASP) flooding. Asia-Pacific J Chem Eng 9:471–489. https://doi.org/10.1002/apj
Mandal A (2015) Chemical flood enhanced oil recovery: a review. Int J Oil Gas Coal Technol 9:241–264
Hirasaki G, Miller CA, Puerto M (2011) Recent advances in surfactant EOR. Soc Pet Eng J SPE-115386-PA 16:889–907. https://doi.org/10.2118/115386-pa
ShamsiJazeyi H, Miller CA, Wong MS et al (2014) Polymer-coated nanoparticles for enhanced oil recovery. J Appl Polym Sci 131:1–13. https://doi.org/10.1002/app.40576
Kumar S, Aswal VK, Kohlbrecher J (2016) Small-angle neutron scattering study of interplay of attractive and repulsive interactions in nanoparticle-polymer system. Langmuir 32:1450–1459. https://doi.org/10.1021/acs.langmuir.5b03998
Pogaku R, Mohd Fuat NH, Sakar S et al (2017) Polymer flooding and its combinations with other chemical injection methods in enhanced oil recovery. Polym Bull 74:1–22. https://doi.org/10.1007/s00289-017-2106-z
Sorbie KS (1991) Polymer-improved oil recovery. Springer, Netherlands, p 359. http://dx.doi.org/10.1007/978-94-011-3044-8
Ahmadi Y, Eshraghi SE, Bahrami P et al (2015) Comprehensive water–alternating-gas (WAG) injection study to evaluate the most effective method based on heavy oil recovery and asphaltene precipitation tests. J Pet Sci Eng 133:123–129. https://doi.org/10.1016/j.petrol.2015.05.003
Yusof MA, Agi A, Gbadamosi A et al (2018) Uncertainty analysis of hydrocarbon in place calculation using 3D seismic and well data during appraisal stage—case study of Goldie Field, offshore Sarawak. J Nat Gas Sci Eng 57:238–265. https://doi.org/10.1016/j.jngse.2018.06.038
Gbadamosi AO, Kiwalabye J, Junin R, Augustine A (2018) A review of gas enhanced oil recovery schemes used in the North Sea. J Pet Explor Prod Technol. https://doi.org/10.1007/s13202-018-0451-6
Yekeen N, Manan MA, Idris AK et al (2018) A comprehensive review of experimental studies of nanoparticles-stabilized foam for enhanced oil recovery. J Pet Sci Eng 164:43–74. https://doi.org/10.1016/j.petrol.2018.01.035
Yekeen N, Idris AK, Manan MA et al (2017) Bulk and bubble-scale experimental studies of influence of nanoparticles on foam stability. Chinese J Chem Eng 25:347–357. https://doi.org/10.1016/J.CJCHE.2016.08.012
Ahmadi MA, Shadizadeh SR (2013) Implementation of a high-performance surfactant for enhanced oil recovery from carbonate reservoirs. J Pet Sci Eng 110:66–73. https://doi.org/10.1016/J.PETROL.2013.07.007
Kamal MS, Hussein IA, Sultan AS (2017) Review on surfactant flooding: phase behavior, retention, IFT, and field applications. Energy Fuels 31:7701–7720. https://doi.org/10.1021/acs.energyfuels.7b00353
Abbas AH, Sulaiman WRW, Jaafar MZ et al (2018) Numerical study for continuous surfactant flooding considering adsorption in heterogeneous reservoir. J King Saud Univ Eng Sci. https://doi.org/10.1016/j.jksues.2018.06.001
Ogiriki SO, Agunloye MA, Gbadamosi AO, Olafuyi AO (2016) Exploitation of bitumen from Nigerian tar sand using hot-water/steam stimulation process. Pet Coal 58:407–413. https://doi.org/10.1021/ac010415i
Afolabi F, Ojo T, Udeagbara S, Gbadamosi A (2017) Bitumen extraction from tar sands using solvent techniques. Int J Sci Eng Res 8:783–790
Agi A, Junin R, Syamsul MF et al (2018) Intermittent and short duration ultrasound in a simulated porous medium. Petroleum. https://doi.org/10.1016/j.petlm.2018.03.012
Pope GA (2011) Recent developments and remaining challenges of enhanced oil recovery. J Pet Sci Eng SPE-0711-0065-JPT 63:65–68. https://doi.org/10.2118/0711-0065-jpt
Kamal MS, Sultan AS, Al-Mubaiyedh UA, Hussein IA (2015) Review on polymer flooding: rheology, adsorption, stability, and field applications of various polymer systems. Polym Rev 55:491–530. https://doi.org/10.1080/15583724.2014.982821
Maia AMS, Borsali R, Balaban RC (2009) Comparison between a polyacrylamide and a hydrophobically modified polyacrylamide flood in a sandstone core. Mater Sci Eng C 29:505–509. https://doi.org/10.1016/j.msec.2008.09.018
Wei B, Romero-Zerón L, Rodrigue D (2014) Oil displacement mechanisms of viscoelastic polymers in enhanced oil recovery (EOR): a review. J Pet Explor Prod Technol 4:113–121. https://doi.org/10.1007/s13202-013-0087-5
Wei B (2016) Advances in polymer flooding
Ponnapati R, Karazincir O, Dao E et al (2011) Polymer-functionalized nanoparticles for improving waterflood sweep efficiency: characterization and transport properties. Ind Eng Chem Res 50:13030–13036. https://doi.org/10.1021/ie2019257
Mandal A, Samanta A, Ojha K (2013) Mobility control and enhanced oil recovery using partially hydrolysed polyacrylamide (PHPA). Int J Oil Gas Coal Technol 6:245–258. https://doi.org/10.1504/IJOGCT.2013.052236
Wegner J (2015) Investigation of polymer enhanced oil recovery (EOR) in microfluidic devices that resemble porous media—an experimental and numerical approach
Sheng JJ (2013) Polymer flooding—fundamentals and field cases. In: Enhanced oil recovery field case studies. Elsevier, pp 63–82
Delamaide E, Zaitoun A, Renard G, Tabary R (2014) Pelican Lake field: first successful application of polymer flooding in a heavy-oil reservoir. Soc Pet Eng J SPE-165234-PA 17:1–22. https://doi.org/10.2118/165234-pa
Li Q, Pu W, Wei B et al (2017) Static adsorption and dynamic retention of an anti-salinity polymer in low permeability sandstone core. J Appl Polym Sci 134:44487–44488. https://doi.org/10.1002/app.44487
Cheraghian G, Hendraningrat L (2016) A review on applications of nanotechnology in the enhanced oil recovery part A: effects of nanoparticles on interfacial tension. Int Nano Lett 6:129–138. https://doi.org/10.1007/s40089-015-0173-4
Abidin AZ, Puspasari T, Nugroho WA (2012) Polymers for enhanced oil recovery technology. Procedia Chem 4:11–16. https://doi.org/10.1016/j.proche.2012.06.002
Cheraghian G (2015) Effects of nanoparticles on wettability: a review on applications of nanotechnology in the enhanced oil recovery. Int J Nano Dimens 6:443–452. https://doi.org/10.7508/ijnd.2015.05.001
Raffa P, Broekhuis AA, Picchioni F (2016) Polymeric surfactants for enhanced oil recovery: a review. J Pet Sci Eng 145:723–733. https://doi.org/10.1016/j.petrol.2016.07.007
Levitt D, Pope GA (2008) Selection and screening of polymers for enhanced-oil recovery. In: SPE-113845-MS, presented at SPE symposium on improved oil recovery, 20–23 April, Tulsa, Oklahoma, USA. Society of Petroleum Engineers, pp 1–18
Hendraningrat L, Li S, Torsæter O (2013) A coreflood investigation of nanofluid enhanced oil recovery. J Pet Sci Eng 111:128–138. https://doi.org/10.1016/j.petrol.2013.07.003
Agi A, Junin R, Gbadamosi A (2018) Mechanism governing nanoparticle flow behaviour in porous media: insight for enhanced oil recovery applications. Int Nano Lett 8:1–29. https://doi.org/10.1007/s40089-018-0237-3
Gbadamosi AO, Junin R, Manan MA et al (2018) Recent advances and prospects in polymeric nanofluids application for enhanced oil recovery. J Ind Eng Chem. https://doi.org/10.1016/j.jiec.2018.05.020
Bera A, Belhaj H (2016) Application of nanotechnology by means of nanoparticles and nanodispersions in oil recovery—a comprehensive review. J Nat Gas Sci Eng 34:1284–1309. https://doi.org/10.1016/j.jngse.2016.08.023
Sun X, Zhang Y, Chen G, Gai Z (2017) Application of nanoparticles in enhanced oil recovery: a critical review of recent progress. Energies 10:345. https://doi.org/10.3390/en10030345
Mothé CG, Correia DZ, de França FP, Riga AT (2006) Thermal and rheological study of polysaccharides for enhanced oil recovery. J Therm Anal Calorim 85:31–36. https://doi.org/10.1007/s10973-005-7339-7
Ghoumrassi-Barr S, Aliouche D (2016) A rheological study of xanthan polymer for enhanced oil recovery. J Macromol Sci Part B 55:793–809. https://doi.org/10.1080/00222348.2016.1207544
Wu Y, Mahmoudkhani A, Watson P et al (2012) Development of new polymers with better performance under conditions of high temperature and high salinity. In: SPE EOR conference at oil and gas West Asia, 16–18 April, Muscat, Oman. Society of Petroleum Engineers, pp 1–11
Vargo J, Turner J, Bob V et al (2000) Alkaline-surfactant-polymer flooding of the Cambridge Minnelusa Field. SPE-68285-PA. SPE Reserv Eval Eng 3:552–558. https://doi.org/10.2118/68285-PA
Tiwari D, Marathe RV, Patel NK et al (2008) Performance of polymer flood in Sanand Field, India—a case study. In: SPE-114878-MS, presented at SPE Asia Pacific oil and gas conference and exhibition, 20–22 October, Perth, Australia. Society of Petroleum Engineers, pp 1–9
Pitts MJ, Wyatt K, Surkalo H (2004) Alkaline-polymer flooding of the David Pool, Lloydminster Alberta. In: SPE-89386-MS, presented at SPE/DOE symposium on improved oil recovery, 17–21 April, Tulsa, Oklahoma. Society of Petroleum Engineers, pp 1–6
Pitts MJ, Dowling P, Wyatt K et al (2006) Alkaline-surfactant-polymer flood of the Tanner Field. In: SPE-100004-MS, presented at SPE/DOE symposium on improved oil recovery, 22–26 April, Tulsa, Oklahoma, USA. Society of Petroleum Engineers, pp 1–5
Mogollon JL, Lokhandwala T (2013) Rejuvenating viscous oil reservoirs by polymer injection: lessons learned in the field. In: SPE-165275-MS, presented SPE enhanced oil recovery conference, 2–4 July, Kuala Lumpur, Malaysia. Society of Petroleum Engineers, pp 1–12
Abbas S, Sanders AW, Donovan JC (2013) Applicability of hydroxyethylcellulose polymers for chemical EOR. In: SPE-165311-MS, SPE enhanced oil recovery conference, 2–4 July, Kuala Lumpur, Malaysia. Society of Petroleum Engineers, pp 1–9
Zhijian Q, Yigen Z, Xiansong Z, Jialin D (1998) A successful ASP flooding Pilot in Gudong Oil Field. In: SPE-39613-MS, presented SPE/DOE improved oil recovery symposium, 19–22 April, Tulsa, Oklahoma. Society of Petroleum Engineers, pp 107–121
Pu H, Xu Q (2009) An update and perspective on field-scale chemical floods in Daqing Oilfield, China. In: SPE-118746-MS, presented at SPE Middle East oil and gas show and conference, 15–18 March, Manama, Bahrain. Society of Petroleum Engineers, pp 1–8
Zhang J, Wang K, He F, Zhang F (1999) Ultimate evaluation of the alkali/polymer combination flooding pilot test in XingLongTai Oil Field. In: SPE-57291-MS, presented at SPE Asia Pacific improved oil recovery conference, 25–26 October, Kuala Lumpur, Malaysia. Society of Petroleum Engineers, pp 1–10
Meyers JJ, Pitts MJ, Wyatt K (1992) Alkaline-surfactant-polymer flood of the West Kiehl, Minnelusa Unit. In: SPE-24144-MS, presented at SPE/DOE enhanced oil recovery symposium, 22–24 April, Tulsa, Oklahoma. Society of Petroleum Engineers, pp 423–433
Pratap M, Gauma MS (2004) Field implementation of alkaline-surfactant-polymer (ASP) flooding : a maiden effort in India. In: SPE-88455-MS, presented at SPE Asia Pacific oil and gas conference and exhibition, 18–20 October, Perth, Australia. Society of Petroleum Engineers, pp 1–5
Clemens T, Deckers M, Kornberger M et al (2013) Polymer solution injection—near wellbore dynamics and displacement efficiency, pilot test results, Matzen Field, Austria. In: SPE-164904-MS, presented at EAGE annual conference & exhibition incorporating SPE Europec, 10–13 June, London, UK. Society of Petroleum Engineers, pp 1–13
Loppinet A, Iakovlev S, Glenat P (1997) Five years of injection of hydroxyethylcellulose : an ecological pure product for enhanced oil recovery in the field of Romashkino. In: Offshore Europe 97. Conference. pp 39–43
Al-saadi FS, Al-amri BA, Al Nofli SM et al (2012) Polymer flooding in a large field in South Oman—initial results and future plans. In: SPE-154665-MS, presented at SPE EOR conference at oil and gas West Asia, 16–18 April, Muscat, Oman. Society of Petroleum Engineers, pp 1–7
Wassmuth FR, Green K, Arnold W, Cameron N (2009) Polymer flood application to improve heavy oil recovery at East Bodo. J Can Pet Technol 48:55–61. https://doi.org/10.2118/09-02-55
Watson A, Trahan GA, Sorensen W (2014) An interim case study of an alkaline-surfactant-polymer flood in the Mooney Field, Alberta, Canada. In: SPE-169154-MS, presented at SPE improved oil recovery symposium, 12–16 April, Tulsa, Oklahoma, USA. Society of Petroleum Engineers, pp 1–16
Liu J, Adegbesan KO, Bai J (2012) Suffield area, Alberta, Canada—Caen polymer flood pilot project. In: SPE-157796-MS, SPE heavy oil conference Canada, 12–14 June, Calgary, Alberta, Canada. Society of Petroleum Engineers, pp 1–10
Delamaide E, Bazin B, Rousseau D, Degre G (2014) Chemical EOR for heavy oil: the Canadian experience. In: SPE-169715-MS, presented at SPE EOR conference at oil and gas West Asia, 31 March–2 April, Muscat, Oman. Society of Petroleum Engineers, pp 1–31
Hryc A, Hochenfellner F, Paponi H et al (2013) Design and execution of a polymer injection pilot in Argentina. In: SPE-166078-MS, presented at SPE annual technical conference and exhibition, 30 September–2 October, New Orleans, Louisiana, USA. Society of Petroleum Engineers, pp 1–15
Chauveteau G, Zaitoun A (1981) Basic rheological behavior of xanthan polysaccharide solutions in porous media: effect of pore size and polymer concentration. In: Proceedings of the first European symposium on enhanced oil recovery, Bournemouth, England, Society of Petroleum Engineers, Richardson, TX. pp 197–212
Willhite GP, Dominguez JG (1977) Mechanisms of polymer retention in porous media. In: Improved oil recovery by surfactant and polymer flooding. pp 511–554
Drumeanu AC (2017) Some considerations concerning four-ball machine testing of the polyacrylamide solutions. In: IOP conference series: materials science and engineering. pp 1–7
Zaitoun A, Makakou P, Blin N et al (2012) Shear stability of EOR polymers. SPE-141113-PA. SPE J 17:335–339. https://doi.org/10.2118/141113-PA
Afolabi RO (2015) Effect of surfactant and hydrophobe content on the rheology of poly (acrylamide-co-N-dodecylacrylamide) for potential enhanced oil recovery application. Am J Polym Sci 5:41–46. https://doi.org/10.5923/j.ajps.20150502.02
Aluhwal OKH (2008) Simulation study of improving oil recovery by polymer flooding in a malaysian reservoir
Sheng JJ (2011) Chapter 5—polymer flooding. In: Modern chemical enhanced oil recovery. pp 101–206
Sandvik EI, Maerker JM (1977) Application of Xanthan Gum for enhanced oil recovery. In: Extracellular microbial polysaccharides. pp 242–264
Ryles RG (1983) Elevated temperature testing of mobility control reagents. In: SPE-12008-MS, SPE annual technical conference and exhibition, 5–8 October, San Francisco, California. Society of Petroleum Engineers, pp 1–10
Caulfield MJ, Qiao GG, Solomon DH (2002) Some aspects of the properties and degradation of polyacrylamides. Chem Rev 102:3067–3084. https://doi.org/10.1021/cr010439p
Rashidi M, Blokhus AM, Skauge A (2011) Viscosity and retention of sulfonated polyacrylamide polymers at high temperature. J Appl Polym Sci 119:3623–3629. https://doi.org/10.1002/app.33056
Mungan N (1969) Rheology and adsorption of aqueous polymer solutions. J Can Pet Technol 8:45–50. https://doi.org/10.2118/69-02-01
Davison P, Mentzer E (1982) Polymer flooding in north sea reservoirs. Soc Pet Eng J 22:353–362. https://doi.org/10.2118/9300-PA
Seright RS, Campbell A, Mozley P, Han P (2010) Stability of partially hydrolyzed polyacrylamides at elevated temperatures in the absence of divalent cations. SPE-121460-PA. SPE J 15:341–348. https://doi.org/10.2118/121460-PA
Lai N, Guo X, Zhou N, Xu Q (2016) Shear resistance properties of modified nano-SiO2/AA/AM copolymer oil displacement agent. Energies 9:1037. https://doi.org/10.3390/en9121037
Seright RS, Adamski RP, Roffall JC, Liauh WW (1983) Rheology and mechanical degradation of EOR polymers. In: Presented at SPEI British Society of rheology conference on rheology in crude oil production, Imperial College, London, 13–15 April
Zhang L, Zhang D, Jiang B (2006) The rheological behavior of salt tolerant polyacrylamide solutions. Chem Eng Technol 29:395–400. https://doi.org/10.1002/ceat.200500306
Liu P, Mu Z, Wang C, Wang Y (2017) Experimental study of rheological properties and oil displacement efficiency in oilfields for a synthetic hydrophobically modified polymer. Sci Rep 7:8791. https://doi.org/10.1038/s41598-017-09057-9
Chul JJ, Ke Z, Hyun CB, Jin CH (2012) Rheology and polymer flooding characteristics of partially hydrolyzed polyacrylamide for enhanced heavy oil recovery. J Appl Polym Sci 127:4833–4839. https://doi.org/10.1002/app.38070
Lee KS (2011) Performance of a polymer flood with shear-thinning fluid in heterogeneous layered systems with crossflow. Energies 4:1112–1128. https://doi.org/10.3390/en4081112
Shupe RD (1981) Chemical stability of polyacrylamide polymers. SPE-9299-PA. J Pet Technol 33:1513–1529. https://doi.org/10.2118/9299-PA
Yang SH, Treiber LE (1985) Chemical stability of polyacrylamide under simulated field conditions. In: SPE-14232-MS, presented at SPE annual technical conference and exhibition, 22–26 September, Las Vegas, Nevada. Society of Petroleum Engineers, pp 1–12
Zhong C, Luo P, Ye Z, Chen H (2009) Characterization and solution properties of a novel water-soluble terpolymer for enhanced oil recovery. Polym Bull 62:79–89. https://doi.org/10.1007/s00289-008-1007-6
Chen Q, Wang Y, Lu Z, Feng Y (2013) Thermoviscosifying polymer used for enhanced oil recovery: rheological behaviors and core flooding test. Polym Bull 70:391–401. https://doi.org/10.1007/s00289-012-0798-7
Zhou C, Yang W, Yu Z et al (2011) Synthesis and solution properties of novel comb-shaped acrylamide copolymers. Polym Bull 66:407–417. https://doi.org/10.1007/s00289-010-0360-4
Li F, Luo Y, Hu P, Su G (2017) Study on the self-assembly properties of surface active hydrophobically associating polyacrylamide with nonionic surfmer units. Polym Bull 74:2873–2886. https://doi.org/10.1007/s00289-016-1871-4
Zhang P, Bai S, Chen S et al (2017) Preparation, solution characteristics and displacement performances of a novel acrylamide copolymer for enhanced oil recovery (EOR). Polym Bull 74:1–11. https://doi.org/10.1007/s00289-017-2080-5
Ragab AMS (2014) Investigating the potential of nanomaterial for enhanced oil recovery: state of art. J Sci Technol 6:25–40
Negin C, Ali S, Xie Q (2016) Application of nanotechnology for enhancing oil recovery—a review. Petroleum 2:324–333. https://doi.org/10.1016/j.petlm.2016.10.002
Bayat AE, Junin R (2015) Transportation of metal oxide nanoparticles through various porous media for enhanced oil recovery. In: SPE/IATMI Asia Pacific oil & gas conference and exhibition, 20–22 October, Nusa Dua, Bali, Indonesia https://doi.org/10.2118/176365-ms. Society of Petroleum Engineers, pp 1–15
Esfandyari Bayat A, Junin R, Samsuri A et al (2014) Impact of metal oxide nanoparticles on enhanced oil recovery from limestone media at several temperatures. Energy Fuels 28:6255–6266. https://doi.org/10.1021/ef5013616
Zhang H, Nikolov A, Wasan D (2014) Enhanced oil recovery (EOR) using nanoparticle dispersions: underlying mechanism and imbibition experiments. Energy Fuels 28:3002–3009. https://doi.org/10.1021/ef500272r
Ogolo NA, Olafuyi OA, Onyekonwu MO (2012) Enhanced oil recovery using nanoparticles. In: SPE Saudi Arabia section technical symposium and exhibition, 8–11 April, Al-Khobar, Saudi Arabia https://doi.org/10.2118/160847-ms. Society of Petroleum Engineers, pp 1–9
Onyekonwu MO, Ogolo NA (2010) Investigating the use of nanoparticles in enhancing oil recovery. In: Nigeria annual international conference and exhibition, 31 July–7 August, Tinapa—Calabar, Nigeria https://doi.org/10.2118/140744-ms. Society of Petroleum Engineers, pp 1–14
Paul DR, Robeson LM (2008) Polymer nanotechnology: nanocomposites. Polymer (Guildf) 49:3187–3204. https://doi.org/10.1016/j.polymer.2008.04.017
Pavlidou S, Papaspyrides CD (2008) A review on polymer–layered silicate nanocomposites. Prog Polym Sci 33:1119–1198. https://doi.org/10.1016/j.progpolymsci.2008.07.008
Li S, Meng Lin M, Toprak MS et al (2010) Nanocomposites of polymer and inorganic nanoparticles for optical and magnetic applications. Nano Rev. https://doi.org/10.3402/nano.v1i0.5214
Chandran S, Begam N, Padmanabhan V, Basu JK (2014) Confinement enhances dispersion in nanoparticle–polymer blend films. Nat Commun 5:3697
Kennedy JRM, Kent KE, Brown JR (2015) Rheology of dispersions of xanthan gum, locust bean gum and mixed biopolymer gel with silicon dioxide nanoparticles. Mater Sci Eng C 48:347–353. https://doi.org/10.1016/j.msec.2014.12.040
Zheng Y, Zheng Y, Ning R (2003) Effects of nanoparticles SiO2 on the performance of nanocomposites
Yang-Chuan K, Guang-Yao W, Yi W (2008) Hattemer et al 2015. Eur Polym J 44:2448–2457. https://doi.org/10.1016/j.eurpolymj.2008.06.007
Xin H, Ao D, Wang X et al (2015) Synthesis, characterization, and properties of copolymers of acrylamide with sodium 2-acrylamido-2-methylpropane sulfonate with nano silica structure. Colloid Polym Sci 293:1307–1316. https://doi.org/10.1007/s00396-015-3512-0
Hanemann T, Szabó DV (2010) Polymer-nanoparticle composites: from synthesis to modern applications
Kumar S (2015) Structural studies of nanoparticles interactions with different macromolecules
Kumar S, Ray D, Aswal VK, Kohlbrecher J (2014) Structure and interaction in the polymer-dependent reentrant phase behavior of a charged nanoparticle solution. Phys Rev E—Stat Nonlinear Soft Matter Phys 90:1–10. https://doi.org/10.1103/PhysRevE.90.042316
Otsubo Y, Umeya K (1984) Rheological properties of silica suspensions in polyacrylamide solutions. J Rheol (N Y N Y) 28:95–108. https://doi.org/10.1122/1.549742
Kawaguchi M (1994) Rheological properties of silica suspensions in polymer solutions. Adv Colloid Interface Sci 53:103–127. https://doi.org/10.1016/0001-8686(94)00214-2
Maghzi A, Mohebbi A, Kharrat R, Ghazanfari MH (2013) An experimental investigation of silica nanoparticles effect on the rheological behavior of polyacrylamide solution to enhance heavy oil recovery. Pet Sci Technol 31:500–508. https://doi.org/10.1080/10916466.2010.518191
Maghzi A, Kharrat R, Mohebbi A, Ghazanfari MH (2014) The impact of silica nanoparticles on the performance of polymer solution in presence of salts in polymer flooding for heavy oil recovery. Fuel 123:123–132. https://doi.org/10.1016/j.fuel.2014.01.017
Hu Z, Haruna M, Gao H et al (2017) Rheological properties of partially hydrolyzed polyacrylamide seeded by nanoparticles. Ind Eng Chem Res 56:3456–3463. https://doi.org/10.1021/acs.iecr.6b05036
Zhu D, Han Y, Zhang J et al (2014) Enhancing rheological properties of hydrophobically associative polyacrylamide aqueous solutions by hybriding with silica nanoparticles. J Appl Polym Sci 131:1–8. https://doi.org/10.1002/app.40876
Zhu D, Wei L, Wang B, Feng Y (2014) Aqueous hybrids of silica nanoparticles and hydrophobically associating hydrolyzed polyacrylamide used for eor in high-temperature and high-salinity reservoirs. Energies 7:3858–3871. https://doi.org/10.3390/en7063858
Maurya NK, Mandal A (2016) Studies on behavior of suspension of silica nanoparticle in aqueous polyacrylamide solution for application in enhanced oil recovery. Pet Sci Technol 34:429–436. https://doi.org/10.1080/10916466.2016.1145693
Barati N, Zargartalebi M, Kharrat R (2013) Rheological behaviour of nanosilica suspensions and the potential to enhance polymer flooding performance. Spec Top Rev Porous Media 4:327–337
Yousefvand H, Jafari A (2015) Enhanced oil recovery using polymer/nanosilica. Procedia Mater Sci 11:565–570. https://doi.org/10.1016/j.mspro.2015.11.068
Cheraghian G (2016) Application of nano-fumed silica in heavy oil recovery. Pet Sci Technol 34:12–18. https://doi.org/10.1080/10916466.2015.1114497
Tang JC, Lin GL, Yang HC et al (2007) Polyimide-silica nanocomposites exhibiting low thermal expansion coefficient and water absorption from surface-modified silica. J Appl Polym Sci 104:4096–4105. https://doi.org/10.1002/app.26041
Cheraghian G, Khalili Nezhad SS, Kamari M et al (2014) Adsorption polymer on reservoir rock and role of the nanoparticles, clay and SiO2. Int Nano Lett 4:114. https://doi.org/10.1007/s40089-014-0114-7
Khalilinezhad SS, Cheraghian G, Roayaei E et al (2017) Improving heavy oil recovery in the polymer flooding process by utilizing hydrophilic silica nanoparticles. Energy Sources Part A Recover Util Environ Eff. https://doi.org/10.1080/15567036.2017.1302521
AlamiNia H, Khalilinezhad SS (2017) Application of hydrophilic silica nanoparticles in chemical enhanced heavy oil recovery processes. Energy Sources, Part A Recover Util Environ Eff. https://doi.org/10.1080/15567036.2017.1299257
Bagaria HG, Xue Z, Neilson BM et al (2013) Iron oxide nanoparticles grafted with sulfonated copolymers are stable in concentrated brine at elevated temperatures and weakly adsorb on silica. ACS Appl Mater Interfaces 5:3329–3339. https://doi.org/10.1021/am4003974
Wiśniewska M, Chibowski S, Urban T et al (2016) Impact of anionic polyacrylamide on stability and surface properties of the Al2O3–polymer solution system at different temperatures. Colloid Polym Sci 294:1511–1517. https://doi.org/10.1007/s00396-016-3906-7
Wiśniewska M, Chibowski S, Urban T (2015) Modification of the alumina surface properties by adsorbed anionic polyacrylamide—Impact of polymer hydrolysis. J Ind Eng Chem 21:925–931. https://doi.org/10.1016/j.jiec.2014.04.034
Christensen M, Tanino Y (2017) Waterflood oil recovery from mixed-wet limestone: dependence upon the contact angle. Energy Fuels 31:1529–1535. https://doi.org/10.1021/acs.energyfuels.6b03249
Christensen M, Tanino Y (2017) Enhanced permeability due to apparent oil/brine slippage in limestone and its dependence on wettability. Geophys Res Lett 44:6116–6123. https://doi.org/10.1002/2017GL073603
Maghzi A, Mohebbi A, Kharrat R, Ghazanfari MH (2011) Pore-scale monitoring of wettability alteration by silica nanoparticles during polymer flooding to heavy oil in a five-spot glass micromodel. Transp Porous Media 87:653–664. https://doi.org/10.1007/s11242-010-9696-3
Zheng C, Cheng Y, Wei Q et al (2017) Suspension of surface-modified nano-SiO2 in partially hydrolyzed aqueous solution of polyacrylamide for enhanced oil recovery. Colloids Surfaces A Physicochem Eng Asp 524:169–177. https://doi.org/10.1016/j.colsurfa.2017.04.026
Sedaghat MH, Mohammadi H, Razmi R (2016) Application of SiO2 and TiO2 nano particles to enhance the efficiency of polymer-surfactant floods. Energy Sources Part A Recover Util Environ Eff 38:22–28. https://doi.org/10.1080/15567036.2012.740552
Nezhad JR, Jafari A, Abdollahi M (2018) Proficiency feasibility of multi-walled carbon nanotubes in the presence of polymeric surfactant on enhanced oil recovery. In: AIP conference proceedings. pp 1–7
Rezaei A, Abdi-Khangah M, Mohebbi A et al (2016) Using surface modified clay nanoparticles to improve rheological behavior of hydrolized polyacrylamid (HPAM) solution for enhanced oil recovery with polymer flooding. J Mol Liq 222:1148–1156. https://doi.org/10.1016/j.molliq.2016.08.004
Khalilinezhad SS, Cheraghian G, Karambeigi MS et al (2016) Characterizing the role of clay and silica nanoparticles in enhanced heavy oil recovery during polymer flooding. Arab J Sci Eng 41:2731–2750. https://doi.org/10.1007/s13369-016-2183-6
Abdullahi MB, Rajaei K, Junin R, Bayat AE (2018) Appraising the impact of metal-oxide nanoparticles on rheological properties of HPAM in different electrolyte solutions for enhanced oil recovery. J Pet Sci Eng. https://doi.org/10.1016/j.petrol.2018.09.013
Cheraghian G (2016) Effect of nano titanium dioxide on heavy oil recovery during polymer flooding. Pet Sci Technol 34:633–641. https://doi.org/10.1080/10916466.2016.1156125
Kumar RS, Sharma T (2018) Stability and rheological properties of nanofluids stabilized by SiO2 nanoparticles and SiO2–TiO2 nanocomposites for oilfield applications. Colloids Surfaces A Physicochem Eng Asp 539:171–183. https://doi.org/10.1016/j.colsurfa.2017.12.028
Lima MCFS, do Amparo SZ, Ribeiro H et al (2016) Aqueous suspensions of carbon black with ethylenediamine and polyacrylamide-modified surfaces: applications for chemically enhanced oil recovery. Carbon N Y 109:290–299. https://doi.org/10.1016/j.carbon.2016.08.021
Kadhum MJ, Swatske D, Weston J et al (2017) Polymer-stabilized multi-walled carbon nanotube dispersions in high-salinity brines. Energy Fuels 31:5024–5030. https://doi.org/10.1021/acs.energyfuels.7b00522
Nguyen BD, Ngo TK, Bui TH et al (2015) The impact of graphene oxide particles on viscosity stabilization for diluted polymer solutions using in enhanced oil recovery at HTHP offshore reservoirs. Adv Nat Sci Nanosci Nanotechnol 6:1–7. https://doi.org/10.1088/2043-6262/6/1/015012
Wang D, Cheng J, Yang Q et al (2000) Viscous-elastic polymer can increase microscale displacement efficiency in cores. In: SPE-63227-MS presented at SPE annual technical conference and exhibition, 1–4 October, Dallas, Texas. Society of Petroleum Engineers, pp 1–10
Xia H, Wang D, Wang G et al (2008) Mechanism of the effect of micro-forces on residual oil in chemical flooding. In: SPE-114335-MS, presented at SPE symposium on improved oil recovery, 20–23 April, Tulsa, Oklahoma, USA. Society of Petroleum Engineers, pp 1–10
Afsharpoor A, Balhoff MT, Bonnecaze R, Huh C (2012) CFD modeling of the effect of polymer elasticity on residual oil saturation at the pore-scale. J Pet Sci Eng 94:79–88. https://doi.org/10.1016/j.petrol.2012.06.027
Zeyghami M, Kharrat R, Ghazanfari MH (2014) Investigation of the applicability of nano silica particles as a thickening additive for polymer solutions applied in EOR processes. Energy Sources Part A Recover Util Environ Eff 36:1315–1324. https://doi.org/10.1080/15567036.2010.551272
Cheraghian G, Khalilinezhad SS (2015) Effect of nanoclay on heavy oil recovery during polymer flooding. Pet Sci Technol 33:999–1007. https://doi.org/10.1080/10916466.2015.1014962
Cheraghian G (2015) An experimental study of surfactant polymer for enhanced heavy oil recovery using a glass micromodel by adding nanoclay. Pet Sci Technol 33:1410–1417. https://doi.org/10.1080/10916466.2015.1062780
Sharma T, Sangwai JS (2017) Silica nanofluids in polyacrylamide with and without surfactant: viscosity, surface tension, and interfacial tension with liquid paraffin. J Pet Sci Eng 152:575–585. https://doi.org/10.1016/J.PETROL.2017.01.039
Bagwe RP, Hilliard LR, Tan W (2006) Surface modification of silica nanoparticles to reduce aggregation and nonspecific binding. Langmuir 22:4357–4362. https://doi.org/10.1021/la052797j
Kango S, Kalia S, Celli A et al (2013) Surface modification of inorganic nanoparticles for development of organic–inorganic nanocomposites—A review. Prog Polym Sci 38:1232–1261. https://doi.org/10.1016/j.progpolymsci.2013.02.003
Zou H, Wu S, Shen J (2008) Polymer/silica nanocomposites: preparation, characterization, properties, and applications. Chem Rev 108:3893–3957. https://doi.org/10.1021/cr068035q
Mittal V (2015) Surface modification of nanomaterials for application in polymer nanocomposites : an overview. In: Surface modification of nanoparticle and natural fiber fillers. pp 1–27
Acknowledgements
The authors would like to thank the Ministry of Higher Education, Malaysia, and Universiti Teknologi Malaysia, for supporting this research through Research Management Grant Vot. Nos. Q.J30000.2546.14H50 and R.J130000.7846.4F946.
Author information
Authors and Affiliations
Corresponding author
Additional information
Publisher's Note
Springer Nature remains neutral with regard to jurisdictional claims in published maps and institutional affiliations.
Rights and permissions
About this article
Cite this article
Gbadamosi, A.O., Junin, R., Manan, M.A. et al. Hybrid suspension of polymer and nanoparticles for enhanced oil recovery. Polym. Bull. 76, 6193–6230 (2019). https://doi.org/10.1007/s00289-019-02713-2
Received:
Revised:
Accepted:
Published:
Issue Date:
DOI: https://doi.org/10.1007/s00289-019-02713-2