Abstract
The environmental quality of a Ramsar wetland site located at the Cananéia-Iguape-Peruíbe Protected Area (CIP-PA), in São Paulo, Brazil, was assessed by geochemical analyses and biomarker assessments (GPx, GST, GSH, GST, MT, LPO, DNA damage) performed in swimming crab Callinectes danae Smith, 1869 organs (posterior and anterior gills and hepatopancreas) to estimate sediment contaminant bioavailability. The results indicated that two sampling stations, PT and PM, exhibited the worst environmental conditions, as sediments collected at both points contained metal contamination, while crabs exhibited significant responses for GPx, GST, and LPO (mostly during winter). Sediment contamination tended to be associated to fine sediments (both seasons) and organic matter (winter). During the summer survey, Pb concentrations in sediments of station PT exceeded the Brazilian Sediment Quality Guidelines (SQGs) and the Canadian Interim Marine Sediment Quality Guidelines. Metal concentrations in sediments sampled in winter were higher compared with summer, with Co, Ni, and Pb exceeding SQGs levels at PT, whereas Co, Ni, Hg, Zn, and Pb exceeded SQGs at PM. Biomarker induction during summer appeared to be caused by natural variables (water salinity and temperature, and molting cycle), whereas oxidative stress and tissue damage during winter appeared to be more clearly linked to metal contamination. Anterior gills presented the clearest signs of seasonal variability, being more responsive to sediment contamination. The results suggest that metals originated from the upper Ribeira de Iguape River are transported toward the estuarine system, causing effects on C. danae individuals. Additionally, seasonality is a strong factor concerning CIP-PA toxicity, since the rainfall regime significantly modifies the freshwater flow and, consequently, estuarine water salinity, suspended particle and metal inputs, as well as the location of depositional areas. Thus, efforts to mitigate CIP-PA contamination should be based on the control of upstream pollution sources.
Similar content being viewed by others
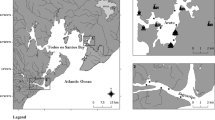
Explore related subjects
Discover the latest articles, news and stories from top researchers in related subjects.Avoid common mistakes on your manuscript.
Approximately one-third of the world population lives at or close to coastal zones, leading to intense pressure on these ecosystems (UNEP 2006). Urban growth, agriculture expansion and the corresponding increases in industrial and mining activities are necessary to deal with population growth and increasing land, food, and product demands. Mining activities, in particular, are of significant concern (Ayangbenro and Babalola 2017), because they are highly impactful (Farjana et al. 2018) and have been frequently related to environmental contamination by metals.
Marine organisms can be exposed to several contaminants, which may display accumulation potential. Thus, these organisms have been frequently applied as contamination biomonitors in both estuarine and coastal environments (Rainbow 1995). Crustaceans, in particular, are useful indicators used to monitor ecotoxicological effects caused by pollutants (Vogt et al. 2018). Crabs, in particular, play an important role in nutrient and energy transfer throughout the marine trophic chain and may transfer pollutants to other trophic levels (Lavradas et al. 2014). In addition, they are an important fishery resource, especially for artisanal fishers, with significant social and economic relevance (Bordon et al. 2012a). Callinectes danae is one of the several crab species recommended as an environmental biomonitor, providing crucial biological responses in contaminated areas (Bordon et al. 2012b, 2016, 2018).
Pollution effects can be assessed by a range of biomarkers (Jemec et al. 2009, 2017; Lavradas et al. 2014). Biomarkers consist in sub-individual level responses, ranging from molecular and cellular effects to physiological responses and behavioral changes (van der Oost et al. 2002). These responses can be detected early, after short-term exposures, before more severe disorders can take place (e.g., illness, death or population changes) (Hamza-Chaffai 2014). In this sense, the use of an ecotoxicological approach capable of detecting effects at the molecular, biochemical, and cellular levels can provide information that may be used to control pollution impacts before they spread to upper levels of biological organization (Adams et al. 1989). Biomarkers are, thus, crucial in environmental quality assessments, especially in low to moderately contaminated areas, where the effects may not be severe to the point of causing lethality or short-term disturbances in native organisms (Corsi et al. 2005; Bonacci et al. 2007).
Estuaries receive significant anthropic inputs from both point and diffuse sources located upstream or in their surrounding areas (Chapman and Wang 2001). They are, however, among the most productive marine ecosystems worldwide (Nixon et al. 1986). Therefore, estuarine sediment contamination is a critical issue, due to biological and ecological implications (Chapman and Wang 2001). Estuarine and marine ecosystem contamination have been studied worldwide (Kennish 1994; Weis et al. 2011; Burgeot and Gagné 2013), but, surprisingly, assessments concerning Estuarine and Marine Protected Areas (EMPAs) are not usual, although these areas are at significant risk for pollution (Jameson et al. 2002; Pozo et al. 2009; Araujo et al. 2013; Partelow et al. 2015; Moreira et al. 2017). In fact, in a recent review, Abessa et al. (2018) demonstrated that data on contamination or ecotoxicological disturbances are available for less than 1% of the world’s EMPAs, recommending that EMPAs pollution should be further assessed.
A wide mosaic of protected areas (PAs) is found along the coastal zone of the state of São Paulo (Southeastern Brazil), comprising terrestrial, estuarine, and marine PAs. The Cananéia-Iguape-Peruíbe Protected Area (CIP-PA) is noteworthy in this regard. This PA was established by Brazilian Federal decrees 90.347/84, and 91.892/85, aiming to protect its natural and cultural heritage and improving conditions for its inhabitants. In addition, CIP-PA was recognized as a World Natural Heritage Site by UNESCO (2000), is a part of the UNESCO’s Atlantic Rainforest Biosphere Reserve, and has been recently included in the Ramsar List of Wetlands of International Importance (https://rsis.ramsar.org/ris/2310).
The Ribeira de Iguape River (RIR) is the main aquatic contributor to the CIP-PA. Historically, mining residues have been deposited on the river banks of the upper RIR portion (Guimarães and Sígolo 2008; Kummer et al. 2011) and, currently, still constitute a source of metals to surrounding aquatic systems, via ground water and surface drainage (Cotta et al. 2006; Melo et al. 2012), as well as by leaching from slags piles and ores (Guimarães and Sígolo 2008; Piedade et al. 2014). According to Abessa et al. (2014), the metals are then carried downstream and adsorbed to suspended particles (fines and organic matter), reaching the CIP-PA. The metal input to the region increased after the construction of the Valo Grande channel, when over 60% of the RIR flow began to be discharged into the estuarine complex (Barcellos et al. 2005; Mahiques et al. 2009). Consequently, some CIP-PA areas present sediments containing moderate to high metal concentrations, which are related to upper RIR basin sources (Mahiques et al. 2013, 2009; Gusso-Choueri et al. 2018a, b).
After reaching aquatic systems, contaminants may spread and be transported throughout the environment by currents and waves. However, they tend to remain in the water column for limited periods, accumulating in low energy area sediments. Once deposited, contaminants may be released back to the water column and, consequently, to the biota. Thus, sediments have been reported as a secondary source of contaminants (Bordon et al. 2012a; Lavradas et al. 2014), constituting a potential threat to aquatic organisms.
Since the CIP-PA is under the influence of former mining activities, and considering that it plays an important role in marine/estuarine biological resource conservation, environmental quality assessments at this MPA are required to evaluate whether the presence of metals is causing negative impacts on the local biota. In this context, this study aimed to identify the adverse biological effects related to environmental contamination by metals at the CIP-PA, through the use of blue crab Callinectes danae biomarkers in different organs (posterior and anterior gills and hepatopancreas), associated to chemical sediment evaluations applying a seasonal approach (summer and winter).
Materials and Methods
Study Area
The study area comprises the Cananéia-Iguape-Peruíbe Estuarine System (CIP), which includes the lower RIR course and four islands (Cananéia, Cardoso, Comprida, and Iguape). The RIR and its tributaries form one of the largest drainage basins of the S–SE Brazilian coast, reaching 470 km in length (Abessa et al. 2014). The river outflow in its lower course ranges from 300 to more than 1200 m3/s, strongly controlled by the climatic regime (Mahiques et al. 2013). The rainy season begins in October and ends in March (120 mm/month), while the dry season ranges from April to September (70 mm/month) (Abessa et al. 2014). Anthropic occupation is more consolidated and dense in the northern portion of the system, especially near the Iguape and Comprida Island urban centers and in the lower RIR valley (Barcellos et al. 2005).
The Valo Grande canal is an artificial waterway constructed to connect the RIR and the estuary to reduce distances to the ocean and agricultural trade costs. However, after its construction, most of the RIR flow was diverted towards the estuary through the Valo Grande canal, transforming the RIR into the main freshwater, sediment, nutrient, and contaminant contributor to the estuary (Mahiques et al. 2013, 2009). Since then, CIP-PA sediments have exhibited increasing metal levels, especially Pb (Rodrigues et al. 2012).
Sediment and C. danae sampling
Sediment sampling was performed close to the Valo Grande canal, where high contamination levels are expected, and followed southward up to Cardoso Island (reference area), totaling six stations. Figure 1 presents the geographical coordinates and the map of sampling stations, respectively. The sampling surveys were performed in March (summer) and September (winter) of 2013. For sediment collection, a stainless steel Van Veen trap (0.036 m2) was used. Sediments were maintained in plastic flasks and chilled (4 °C) for grain size distribution evaluations and frozen (− 20 °C) for the geochemistry analyses.
Callinectes danae specimens and sediments were obtained from each sampling station. Organisms were collected by otter-trawling, each trawl lasting about 10–15 min at each station. When trawling was not possible, crab traps were used to obtain a satisfactory number of individuals. At least eight organisms were collected from each station and taken to the laboratory, where they were conditioned for 12 h in plastic buckets containing estuarine saltwater (with aeration) for acclimation.
The organisms were then immobilized, anesthetized with ice, euthanized, and dissected to remove soft tissues (muscle, gills, and hepatopancreas) for the biomarker analyses. These organs were selected based on their roles, as both the gills and hepatopancreas present depuration functions, while muscle acetylcholinesterase (AChE) determinations are appropriate for neurotoxicity measurements.
Sediment Properties
The sediment texture analysis was based on the protocol described by McCave and Syvitski (2007). Briefly, 30 g of dry sediment were wet sieved (63µm) to isolate fine particles (mud), such as clay and silt. Retained particles were dried and weighed, and the mud content was given by initial and final weight variations. The sandy fraction was sieved (ɸ scale) to distinguish different sand classes, which were then classified according to the Wentworth scale. Calcium carbonate (CaCO3) content was determined according to Hirota and Szyper (1975). According to this method, 10 mL of hydrochloric acid (12 mol L−1 HCl) were added to 5 g of sediment for 24 h. Subsequently, sediment samples were washed and dried (60 °C). The difference between the initial and final weights indicates the amount of CaCO3. The loss on ignition method (Luczak et al. 1997) was used to determine the organic matter (OM) content. Five grams of dry sediment from each sample were incinerated in a muffle at 500 °C, for 4 h and OM content was given by the difference between initial and final weights.
Inductively Coupled Plasma Optical Emission Spectrometry (ICP OES) and Inductively Coupled Plasma Mass Spectrometry (ICP-MS) Analyses
Sediment samples from the austral summer (March) were analyzed through weak acid extraction. This method selected metals that are solubilized by a weak acidification (simultaneously extracted metals, SEM). The sediment aliquots used for the SEM analyses were maintained frozen in glass flasks and were subsequently submitted to the purge and trap extraction technique (Allen et al. 1993). Wet samples were submitted to a cold acid distillation using a 6 mol L−1 HCl solution, and the released H2S was trapped in 0.5 mol L−1 NaOH, using N2 as a carrier gas. A Jobin–Yvon Horiba Ultima 2 model (Longjumeau, France) ICP OES was used for metal concentration determinations (Fe, Mn, Cd, Cu, Ni, Pb, and Zn). Adopted QA/QC procedures included the use of replicates, blanks and standards for these analyses. For each metal, curves were constructed using fortified solutions with known concentrations.
Sediment samples from winter (September) were analyzed through HNO3 extraction and determined by inductively coupled plasma mass spectrometry (ICP-MS) using an ELAN DRC II ICP-MS (Perkin Elmer-Sciex, Norwalk, CT) without the use of a reaction cell. A standard Meinhard nebulizer type with twisted cyclonic chamber was used for sample introduction. 103Rh (20 μg L−1) was used as internal standard. Al, Cr, Fe, Co, Ni, Cu, Zn, Cd, Hg, and Pb were determined and blanks and certified materials (NIST 2711 (Montana Soil) and PACS-2 (Marine Sediment Reference Materials for Trace Metals)) were used for accuracy, in duplicate, as recommended by the Environmental Protection Agency (US EPA 1999).
Biomarkers
Biochemical biomarkers were assessed in C. danae anterior and posterior gills, muscle and hepatopancreas tissues. After acclimation, anesthetization and dissection, the soft tissues were removed and kept frozen at − 80 °C. Immediately before the biochemical analyses, samples were thawed on ice and homogenized with at 4% w/v in proper buffer solution (in Tris–HCl buffer (Tris 50 mmol L−1; Ethylenediamine Tetraacetic Acid (EDTA) 1 mmol L−1; Dithiothreitol (DTT) 1 mmol L−1; sucrose 50 mmol L−1; KCl 150 mmol L−1; Phenylmethylsulfonyl Fluoride (PMSF) 1 mmol L−1, pH 7.6). The extracts were then centrifuged (Eppendorf 5804R) at 12.000×g at 4 °C for 20 min, obtaining a supernatant fraction (S12), which was used for the enzymatic analyses.
Glutathione S-transferase (GST) and glutathione peroxidase (GPx) activities were assessed, following the protocols described by Keen et al. (1976) and Sies et al. (1979), respectively. Intracellular reduced glutathione (GSH and other thiols) levels were also evaluated, following the protocol described by Sedlak and Lindsay (1968). GSH also takes part in cellular detoxification processes, used as a substrate for GST and GPx. AChE activity was analyzed according to Ellman et al. (1961), adapted for microplate determinations by Guilhermino et al. (1996). Due to the metal contamination background at CIP-PA, metallothionein (MTs) concentrations also were evaluated, based on the protocol described by Viarengo et al. (1997). DNA damage (strand breaks) was analyzed by the alkaline precipitation assay (Olive 1988), using fluorescence to quantify DNA traces (Gagné and Blaise 1993). Lipid peroxidation (LPO) analyses were performed through the thiobarbituric acid method, proposed by Wills (1987). The samples were not centrifuged for the DNA and LPO analyses.
Enzyme activities were normalized by total protein content, determined by the Bradford (1976) method. All of the biochemical biomarkers were analyzed using a Synergy HT® Bio Tek microplate reader band.
Condition Factor
The condition factor (CF) indicates the degree of adjustment of a given species to the environment (Wolff 2007). It can be used as a quantitative indicator of the health or “well-being” of a species in its environment (Vazzoler 1996). It is considered a biomarker, as it is related to the nutritional state of a population, varying trough time. The CF can be associated to fat accumulation, susceptibility to environmental changes or to the gonadal development period (Pinheiro and Fransozo 1993; Froese 2006; Le Cren 1951). At the laboratory, the crab specimens were measured and weighed, obtaining carapace width (CW), without taking into account the lateral spines, and wet weight (WW), respectively, by using of a 0.05-mm precision caliper and a 0.1-g precision balance. CF was described for each station (not allotted by sex) through the equation a = WW/CWb (Le Cren 1951), where WW is the wet weight, CW is the carapace width, “b” is the slope of the regression line, and “a” the condition factor.
Statistical Analyses
Biomarkers responses were first checked for homoscedasticity and normality by the Bartlett’s and Shapiro-Wilks tests, respectively. After verifying sample homoscedasticity and normality, the data were compared between the reference area (IC) and between seasons (summer–winter) by a two-way ANOVA (p < 0.05). CF was evaluated by the coefficient of determination (r2) obtained from its equation (cited above).
Before the analysis, data were transformed. Data expressed in % were first Arcsin transformed to allow comparisons with continuous data, whereas log-transformation (y = log10(x + 1)) was used for all data to reduce the magnitudes of the different variables. After data transformation, associations between the different biomarkers and geochemistry data were assessed using a principal component analysis (PCA).
Results
Sediment Properties
Sediment properties are presented in Table 1. Sediments from VG, PE, AR, and IC were predominantly sandy (> 80% sand), whereas sediment from PT was muddy, as well as samples from PM during the summer. Sediment from station AR presented the highest OM content (> 16%, summer). The winter survey showed similar results for most of the samples, except for sediment from PM, which was sandier. PT and PM sediments presented the highest OM contents (14.4% and 6.06%, respectively, winter). Sediments showed variable CaCO3 and OM contents for both seasons.
Metal concentrations in sediments collected during the summer and winter are presented in Table 2. Even considering the weak acid digestion to extract the metals for the summer survey, Pb concentrations in sediments from the PT station exceeded both the Canadian Interim Marine Sediment Quality Guidelines (ISQGs = 30.20 mg kg−1; CCME (2001) and the regional Brazilian Sediment Quality Guidelines (SQGs ≥ 21.1 mg kg−1 indicate highly polluted sediments) proposed by Choueri et al. (2009). Pb concentrations ranged from 1.52 to 32.84 mg kg−1. No other metal presented values above the threshold values for both sediment guidelines.
During the winter sampling, higher metal concentrations were detected in sediments from PT and PM, which also contained high OM and mud contents (Table 2). Comparisons to SQGs (Choueri et al. 2009) indicate that Co (> 4.1 mg kg−1), Ni (> 5.9 mg kg−1), and Pb (> 10.3 mg kg−1) were above toxic thresholds (moderately polluted) in PT sediments, while PM sediments presented Co (> 4.1 mg kg−1), Ni (> 5.9 mg kg−1), Zn (> 39.7 mg kg−1), and Hg (> 0.08 mg kg−1) above threshold values (moderately polluted). PM sediment also contained Pb (> 22.1 mg kg−1, highly polluted) levels above local SQGs toxic values and also exceeded the Interim Marine Sediment Quality Guidelines (ISQGs) (ISQGs = 30.20 mg kg−1; CCME 2001).
Biomarkers
Biochemical biomarkers in C. danae posterior gill tissues are displayed in Fig. 2. During the summer, no statistical difference was detected among stations for GPx activity. However, during winter, organisms from stations VG and PT presented statistically higher GPx activities in comparison to IC (reference station). For GST, organisms from station PM presented lower activities, statistically differing from IC, in both seasons. Organisms from the reference area presented higher GSH levels and other thiols, differing from the crabs collected at the other stations during summer. In the winter, animals from VG and PT presented lower thiol levels. In the summer campaign, MT contents in the posterior gills did not differ among stations. In the winter, however, organisms from station PE presented higher MT levels, statistically differing from IC. Regarding LPO, no statistical difference was observed in the summer among organisms from all stations, while, in winter, crabs from station PM presented higher LPO levels than the control organisms. DNA damage did not differ among stations for each season, but this was the only biomarker that indicated seasonal difference for the posterior gills, with organisms sampled during summer presenting lower DNA damage values than those collected in the winter.
CIP-PA Callinectes danae posterior gill biomarkers responses. Summer results are shown in darker boxplots and winter results in white boxplots (25th to 75th percentiles · line = median · Min to max. (down to the smallest value and up to the largest)). Asterisks (*) indicate statistical differences between stations and the controls (IC). “Stations” displayed in a black box indicates statistical differences between seasons (summer vs. winter), p < 0.05
The biomarker responses for anterior gills are presented in Fig. 3. No statistical differences during summer were observed for any biomarker among stations (i.e., each station in comparison to IC). On the other hand, significant differences were observed for winter. Animals from stations PT, PE, and AR exhibited higher GPx and GST activities in comparison to IC. Regarding GSH and other thiols, no significant differences were observed between organisms from the different sampling sites for both campaigns. Summer levels, however, were much higher than those observed in the winter, for all sites. MT proteins during winter presented higher values than summer. In the winter, animals from the station PE presented higher MT levels than crabs from IC. Regarding LPO, crabs from PE and IC presented the highest values in winter, and no differences were detected in summer. No difference regarding seasonality was evidenced. DNA damage levels showed no divergence among stations in both winter and summer. DNA damage in animals collected in the winter, however, was significantly higher than during the summer. The results also indicate a clear seasonal pattern in anterior gills for GST, GSH, MTs, and DNA damage.
CIP-PA Callinectes danae anterior gill biomarker responses. Summer results are shown in darker boxplots and winter results in white boxplots (25th to 75th percentiles · line = median · Min to max. (down to the smallest value and up to the largest)). Asterisks (*) indicate statistical differences between stations and the controls (IC). “Stations” displayed in a black box indicates statistical differences between seasons (summer vs. winter), p < 0.05
Hepatopancreas responses are displayed in Fig. 4. Crustaceans from the reference site (IC) presented the highest GPx activities in comparison to other stations during summer, statistically different from the activities observed in organisms from station AR. In the winter, lower GPx activities were observed in animals from station VG (in comparison to IC). In the summer, GST activities were similar for all sampling sites. In the winter, some differences were observed, where animals from VG presented lower GST activities and specimens from PT presented higher GST activities in comparison with IC. In the summer, crabs from station PM exhibited significantly higher GSH levels, while in winter GSH levels were similar for all stations. Significantly higher MT levels were observed in crabs from station PE during summer and PT during winter. Lipid peroxidation levels were significantly higher in organisms from stations PM and AR during summer and PT during winter. DNA damage followed the same pattern as observed in posterior and anterior gills, with no statistical differences among stations and statistically higher winter DNA damage compared with summer.
CIP-PA Callinectes danae hepatopancreas biomarker responses. Summer results are shown in darker boxplots and winter results in white boxplots (25th to 75th percentiles · line = median · Min to max. (down to the smallest value and up to the largest)). Asterisks (*) indicate statistical differences between stations and the controls (IC). “Stations” displayed in a black box indicates statistical differences between seasons (summer vs. winter), p < 0.05
No spatial differences for the analyzed neurotoxicity biomarker (AChE) in muscle tissues were detected (Fig. 5). However, this biomarker presented significant seasonality, with statistically lower AChE activity during the summer.
CIP-PA Callinectes danae muscle acetylcholinesterase (AChE) levels. Summer results are shown in darker boxplots and winter results in white boxplots (25th to 75th percentiles · line = median · Min to max. (down to the smallest value and up to the largest)). Asterisks (*) indicate statistical differences between stations and the controls (IC). “Stations” displayed in a black box indicates statistical differences between seasons (summer vs. winter), p < 0.05
Condition Factor
The condition factors presented small variations, when considering sampling sites and periods; values ranged from 0.11 to 0.15). During the summer, the lowest CF values were observed in organisms from stations VG and PT. In the winter, the lowest values were observed in animals from stations VG, PT and IC. The CF did not differ statistically among stations in comparison to the reference station (IC) in any season (Table 3).
Principal Component Analysis
The three first components (PCs) of the summer principal component analysis (PCA) explained 83.84% of variances (Table 4). PC1 grouped sediment characteristics, such as metal content, and mud and CaCO3. Station PT (high metal, CaCO3, and mud content) indicated a high correlation with PC1 (1.85). This PC separated stations PT and PM (positive correlations) from the other stations (VG, PE, AR, and IC) (negative correlations). This PC showed an inverse association to sandy sediments, DNA damage in anterior gills, LPO in posterior gills, and MT in hepatopancreas were linked to a high negative higher association for station PE. PC2 correlated positively CaCO3 and GPx in anterior gills, LPO in posterior gills and GST, LPO, and DNA damage in hepatopancreas. This PC inversely associated neurotoxicity (AChE) and one of the antioxidant enzymes (GPx) in posterior gills and MT in hepatopancreas, associated to station VG (− 1.62). The third axis (PC3) indicates an association of OM content to one of the antioxidant enzymes (GST) in both gills. This result was mostly associated to the station IC (1.10). An inverse association was shown for MT in both gills, LPO in anterior gills and DNA in hepatopancreas, associated to stations VG (− 0.96) and PM (− 1.46).
In the winter campaign, the three first PCA factors explained > 86% of the variances (Table 4). PC1 grouped all metals, mud, OM, and CaCO3, which were inversely associated with sandy sediments. PC1 also associated both antioxidant enzymes (GPx and GST) in hepatopancreas and LPO in both gills. This axis clearly separated stations presenting lower contamination levels (AR, IC, PE, VG; negatively correlated) from those presenting higher contamination levels (PM and PT, positively correlated). PC2 correlated mud, OM, and CaCO3 with the CF and both antioxidant enzymes (GST and GPx) in anterior gills and GST, MT, and LPO in hepatopancreas. A negative correlation was shown between sand, GST (posterior gills), LPO from both gills, and DNA damage in hepatopancreas. A high positive correlation was shown for station PT, while all other stations showed a negative correlation to this axis. The third axis (PC3) indicated gill biomarker associations (GPx and DNA damage in posterior gills and MT in anterior gills) with the CF, correlating stations AR and IC (high CF, 0.19). An inverse correlation was detected for DNA damage in anterior gills, GPx in hepatopancreas, and AChE activity, with a high correlation to station VG.
Discussion
Summer
During the summer sampling, the only metal whose concentration exceeded the sediment quality guidelines was Pb; ISQGs (30.20 mg kg−1) and regional SQGs (21.1 mg kg−1) were exceeded at PT (CCME 2001; Choueri et al. 2009; respectively). However, animals from this station presented only reduced GSH levels in posterior gills in comparison to animals from the reference station IC, as well as all other stations. These results suggest that CIP-PA sediments present a low to moderate level of contamination (due to surpassed limits), which does not lead to severe effects in crabs, at least during the summer. The results also suggest that Pb may not be fully bioavailable or is not absorbed by C. danae.
The results of the multivariate analysis corroborate this hypothesis, positively correlating all metals with specific sediment characteristics (mud and CaCO3 content) to station PT, inversely associating sandy sediments. This station presented the highest metal content (compared to the other stations for all metals, except Fe) during the summer survey. PT and PM were separated from other stations by the multivariate approach. Both stations presented higher mud, CaCO3, and metal contents. Fine sediments (PT and PM) present a higher surface area in comparison to sandy sediments, thus being able to adsorb increased amounts of contaminants (John and Leventhal 1994). PT (> Pb) is closer to the Valo Grande channel, receiving RIR drainage, whereas PM is closer to the city of Cananéia. Cruz et al. (2014) indicated chronic toxicity to the copepod Nitocra sp. for sediments in the vicinities of PT and PM. This corroborates previous studies indicating that the sediments from some portions of the assessed estuarine complex are contaminated by metals (Azevedo et al. 2012; Gusso-Choueri et al. 2018a, b; Mahiques et al. 2009; Tramonte et al. 2018).
Regarding biomarkers, individuals from all stations (posterior gills) presented GSH synthesis inhibition compared with those from the reference station (IC). Moreover, animals from PM presented statistically lower GST activities in posterior gills compared to IC and higher GSH activities in hepatopancreas, with this station exhibiting higher biomarker variability compared to IC. The biomarker results for summer suggest that an oxidative effect occurred, regarding the responses stated above and the lower GSH activities observed at all stations compared with IC (posterior gills), alongside increased MT synthesis (hepatopancreas) at stations PE and AR and lower GPx activity (hepatopancreas) at station AR compared with IC. However, since the animals from the most contaminated station (PT) did not exhibit critical effects regarding biomarker responses during summer, the enzymatic variations detected herein were probably due to natural factors, such as decreased salinity (rainy season), enhanced temperature and endogenous cycles, such as molting and reproduction, or even non-determined chemicals (e.g., polycyclic aromatic hydrocarbons (PAH) and aliphatic hydrocarbons (AHs) from boating activities and sewage inputs from nearby cities, such as Cananéia). PAHs can complex with metals and may also inhibit the detoxification of metals via metallothionein (Gauthier et al. 2014), which could have affected the MT synthesis shown in station PE. Co-exposure of PAHs and metals can generate unpredicted effects, because it may increase metal bioavailability. However, further studies are necessary to enlighten such conclusions (Gauthier et al. 2014). Natural factors (e.g., salinity and temperature) affecting enzymatic biomarkers in other crustaceans (Eurytemora affinis) have been previously reported (Cailleaud et al. 2007), as well as in C. danae (Freire et al. 2011). Interactions between chemical and natural stressors, which may increase toxicant effects, also are reported in the literature (Capparelli et al. 2016; Walters et al. 2017).
Regarding enzymatic activity among tissues, posterior gills tended to present higher antioxidant enzyme activities (GST and GSH) compared with anterior gills (no statistical difference compared with IC). A similar result was reported for the estuarine crab C. granulata (Oliveira et al. 2005). Crabs may present a clear functional difference between anterior and posterior gills, with the former responsible for respiration (gas exchange), and the second, for salt transport (osmoregulation) (Péqueux 1995). Thus, enhanced activities of these enzymes are expected in posterior gills, given the probable increased metabolism for hyper-osmoregulation (Van Horn et al. 2010), especially during summer, when salinities are lower in most of the sampling sites due to the rainy season. Increased GPx and GST activities were detected previously for C. ornatus and C. danae exposed to a hypersaline media (Freire et al. 2011), corroborating the results presented herein (lower GPx and GST activity compared with IC), because salinity increases toward the reference station (IC). Moreover, C. danae performs ecdysis throughout the entire year, with higher activity in spring and summer, periods also influenced by temperature and salinity (Shinozaki-Mendes et al. 2013). Matching the molting process, blue crabs have present an extensive reproductive cycle, coinciding with warmer periods (Branco 1991; Branco and Masunari 2000). Such physiological processes are critical regarding biomarker responses, since oxidative stress levels are increased throughout the molting cycle and the risk of harmful reactive oxygen species (ROS) effects is higher in the post-molt, creating metabolic stress (Vieira and Gomes 2010), which may have influenced biomarker responses. Several authors have previously described enzymatic activity variations according to the molting stage. For example, Mohanty (2017) indicated increased GSH synthesis at the post-molt stage. LPO levels increase at the pre-molt stage and antioxidant enzymes decrease activities during the intermolt stage, reaching a minimum at the early post-molt and increasing again during the late post-molt (Salaenoi et al. 2015).
Winter
During the winter sampling, specific metals (Co, Ni, and Pb) at PT and PM surpassed regional SQGs (Choueri et al. 2009). Zn, Hg, and Pb exceeded regional SQGs and Pb also surpassed Canadian ISQGs (CCME 2001; Choueri et al. 2009) at PM. Both stations (PT and PM) presented sediments with higher OM and mud contents, indicating enhanced capacity to adsorb contaminants, as stated previously.
The multivariate analysis (PCA) indicated an association between specific sediment characteristics (mud, OM, and CaCO3), metals, LPO in both anterior and posterior gills, and oxidative stress (GPx and GST) in hepatopancreas. The PCA also clearly separated PT and PM (the most contaminated) from others, with a positive correlation observed between PT and PM and the other stations negatively correlated to PC1. This suggests that metal in CIP-PA sediments are negatively affecting C. danae tissues, leading to LPO and oxidative stress, corroborating the study performed by Lavradas et al. (2014), where metal-related oxidative damage in Callinectes was also reported.
Linking C. danae biomarker responses with CIP-PA metal concentrations in sediments, crabs from station PT presented increased oxidative stress (GPx activates in both gills, GSH in posterior gills and GST in anterior gills and hepatopancreas) compared with the reference station (IC). The induction of antioxidant enzymes is an important protective mechanism aiming at minimizing cell oxidative damage in polluted environments (Poljšak and Fink 2014). Such enzymes are crucial regarding the detoxification system against chemicals (e.g., metals, which can lead to ROS production), aiming at cellular protection (Lavradas et al. 2014). The gills are the main respiratory organs in crabs and are in direct contact with the surrounding environment, being directly exposed to contaminants from the water column (Henry 1994; Henry et al. 2012), which could explain the responses observed in this tissue.
Animals from PM exhibited activation of conjugation system (GST, compared to IC), which may be related to ROS production and, consequently, LPO in posterior gills. These results suggest that the first line of defense (such as antioxidant system enzymes) is not being enough to repair the stress caused by environmental contamination, leading to gill damage. Gills frequently represent the first barrier against xenobiotics in aquatic organisms, (Kostić et al. 2017). C. danae maintains high levels of antioxidant enzymes (hepatopancreas and gills) to avoid oxidative damage of tissues and lipids (Togni 2007), and studies have demonstrated increased ROS formation in response to metal exposure in organisms, which can provoke widespread damage to cells, such as LPO and genotoxicity (Stohs and Bagchi 1995; Pan and Zhang 2006; Bae et al. 2016). Increased GST activity suggests activation of detoxification mechanisms (Singaram et al. 2013), which probably was not enough in this study, leading to cell damage (LPO).
Conclusions
This study indicated that blue crabs are capable to respond to metal contamination through different metabolic pathways. Results indicate that biomarkers in crabs may be related to natural factors, such as salinity, temperature, and the molting process, during summer, which makes it difficult to visualize contaminant effects. In winter, sediment metal contamination is linked to oxidative stress and tissue damage, suggesting that seasonality strongly influences biomarker responses in crabs and the environmental quality of the CIP-PA, a wetland Ramsar area, despite of the different sediment metal extraction for each season. Anterior gills presented the clearest seasonal variability pattern, more responsive to sediment contamination. During winter, animals from PT and PM presented the worst conditions, corroborated by metal levels in sediments, as both sampling sites were the most contaminated. Crabs from some CIP-PA areas presented altered biochemical responses (GPx, GST, and LPO), which may indicate effects due to metals and/or natural factors. Therefore, biomarkers analyses were capable to detect environmental degradation signs in a moderately contaminated estuarine protected area and indicate that metal levels in some areas of this estuary are enough to produce negative effects on the native biota.
References
Abessa DMS, Gonçalves L, Perina F et al (2014) Sediment geochemistry and climatic influences in a river influenced by former mining activities: the case of Ribeira de Iguape River, SP-PR, Brazil. Open J Water Pollut Treat 1:43–54
Abessa DMS, Albuquerque HC, Morais LG et al (2018) Pollution status of marine protected areas worldwide and the consequent toxic effects are unknown. Environ Pollut. https://doi.org/10.1016/j.envpol.2018.09.129
Adams SM, Shepard KL, Greeley MS et al (1989) The use of bioindicators for assessing the effects of pollutant stress on fish. Mar Environ Res 28:459–464
Allen HE, Fu G, Deng B (1993) Analysis of acid volatile sulfide (AVS) and simultaneously extracted metals (SEM) for the estimation of potential toxicity in aquatic sediments. Environ Toxicol Chem 12:1441–1453
Araujo GSS, Moreira LBB, Morais RDD et al (2013) Ecotoxicological assessment of sediments from an urban marine protected area (Xixová-Japuí State Park, SP, Brazil). Mar Pollut Bull 75:62–68. https://doi.org/10.1016/j.marpolbul.2013.08.005
Ayangbenro AS, Babalola OO (2017) A new strategy for heavy metal polluted environments: a review of microbial biosorbents. Int J Environ Res Public Health. https://doi.org/10.3390/ijerph14010094
Azevedo JS, Braga ES, Roche H et al (2012) Evidence of contamination by oil and oil products in the Santos-São Vicente Estuary, São Paulo, Brazil. Braz J Oceanogr 60:117–126
Bae E, Samanta P, Yoo J, Jung J (2016) Effects of multigenerational exposure to elevated temperature on reproduction, oxidative stress, and Cu toxicity in Daphnia magna. Ecotoxicol Environ Saf 132:366–371. https://doi.org/10.1016/j.ecoenv.2016.06.034
Barcellos RL, Berbel GBB, Braga E, Furtado VV (2005) Distribuição e Características Do Fósforo Sedimentar No Sistema Estuarino Lagunar De Cananéia-Iguape. Geochim Bras 19:22–36
Bonacci S, Iacocca A, Fossi S et al (2007) Biomonitoring aquatic environmental quality in a marine protected area: a biomarker approach. JSTOR 36:308–315
Bordon ICAC, Sarkis JES, Tomás ARG et al (2012a) Assessment of metal concentrations in muscles of the blue crab, Callinectes danae S., from the Santos Estuarine System. Bull Environ Contam Toxicol 89:484–488. https://doi.org/10.1007/s00128-012-0721-9
Bordon ICAC, Sarkis JES, Tomás ARG et al (2012b) A preliminary assessment of metal bioaccumulation in the blue crab, Callinectes danae S., from the Sao Vicente Channel, Sao Paulo State, Brazil. Bull Environ Contam Toxicol 88:577–581. https://doi.org/10.1007/s00128-012-0539-5
Bordon ICAC, Sarkis JES, Andrade NP et al (2016) An environmental forensic approach for tropical estuaries based on metal bioaccumulation in tissues of Callinectes danae. Ecotoxicology 25:91–104. https://doi.org/10.1007/s10646-015-1570-1
Bordon IC, Emerenciano AK, Melo JRC et al (2018) Implications on the Pb bioaccumulation and metallothionein levels due to dietary and waterborne exposures: the Callinectes danae case. Ecotoxicol Environ Saf 162:415–422. https://doi.org/10.1016/j.ecoenv.2018.07.014
Bradford MM (1976) A rapid and sensitive method for the quantitation of microgram quantities of protein utilizing the principle of protein-dye binding. Anal Biochem 72:248–254
Branco JO (1991) Estudo Populacional de Callinectes danae Smith, 1869 (Decapoda, Portunidae) da Lagoa da Conceição. Florianópolis, SC, p 1869
Branco JO, Masunari S (2000) Reproductive ecology of the blue crab, Callinectes danae Smith, 1869 in the Conceição Lagoon system, Santa Catarina Isle, Brazil. Rev Bras Biol 60:17–27
Burgeot T, Gagné F (2013) Contaminant exposure and ecotoxicological impacts in estuaries. Environ Sci Pollut Res 20:599–600. https://doi.org/10.1007/s11356-012-1324-z
Cailleaud K, Maillet G, Budzinski H et al (2007) Effects of salinity and temperature on the expression of enzymatic biomarkers in Eurytemora affinis (Calanoida, Copepoda). Comp Biochem Physiol A Mol Integr Physiol 147:841–849. https://doi.org/10.1016/j.cbpa.2006.09.012
Capparelli MV, Abessa DM, McNamara JC (2016) Effects of metal contamination in situ on osmoregulation and oxygen consumption in the mudflat fiddler crab Uca rapax (Ocypodidae, Brachyura). Comp Biochem Physiol Part C Toxicol Pharmacol 185–186:102–111. https://doi.org/10.1016/j.cbpc.2016.03.004
CCME CC of M of the E (2001) Canadian sediment quality guidelines for the protection of aquatic life. Can Counc Minist Environ 5
Chapman PM, Wang F (2001) Assessing sediment contamination in estuaries. Environ Toxicol Chem 20:3–22
Choueri RB, Cesar A, Abessa DMS et al (2009) Development of site-specific sediment quality guidelines for North and South Atlantic littoral zones: comparison against national and international sediment quality benchmarks. J Hazard Mater 170:320–331. https://doi.org/10.1016/j.jhazmat.2009.04.093
Corsi I, Mariottini M, Badesso A et al (2005) Contamination and sub-lethal toxicological effects of persistent organic pollutants in the European eel (Anguilla anguilla) in the Orbetello lagoon (Tuscany, Italy). Hydrobiologia 550:237–249. https://doi.org/10.1007/s10750-005-4392-y
Cotta JAO, Rezende MOO, Piovani MR (2006) Avaliação do Teor De Metais em Sedimento Do Rio Betari No Parque Estadual Turístico Do Alto Ribeira—Petar, São Paulo, Brasil. Quim Nov 29:40–45
Cruz ACF, Davanso MB, Araujo GS et al (2014) Cumulative influences of a small city and former mining activities on the sediment quality of a subtropical estuarine protected area. Environ Monit Assess. https://doi.org/10.1007/s10661-014-3908-1
Ellman GL, Courtney KD, Andres V Jr, Featherstone RM (1961) A new and rapid colorimetric determination of Acetylcholinesterase activity. Biochem Pharmacol 7:88–95
Environmental Protection Agency UE (1999) Determination of metals in ambient particulate matter using inductively coupled plasma/mass spectrometry (ICP/MS). EPA/625/R-96/010a
Farjana SH, Huda N, Mahmud MAP (2018) Environmental impact assessment of european non-ferro mining industries through life-cycle assessment. Earth Environ Sci. https://doi.org/10.1088/1755-1315/154/1/012019
Freire CA, Togni VG, Hermes-Lima M (2011) Responses of free radical metabolism to air exposure or salinity stress, in crabs (Callinectes danae and C. ornatus) with different estuarine distributions. Comp Biochem Physiol A Mol Integr Physiol 160:291–300. https://doi.org/10.1016/j.cbpa.2011.06.024
Froese R (2006) Cube law, condition factor and weight-length relationships: history, meta-analysis and recommendations. J Appl Ichthyol 22:241–253. https://doi.org/10.1111/j.1439-0426.2006.00805.x
Gagné F, Blaise C (1993) Hepatic Metallothionein Level and mixed function oxidase activity in fingerling rainbow trout (Oncorhynchus mykiss) after acute exposure to pulp and paper mill effluents. Water Res 27:1669–1682
Gauthier PT, Norwood WP, Prepas EE, Pyle GG (2014) Metal-PAH mixtures in the aquatic environment: a review of co-toxic mechanisms leading to more-than-additive outcomes. Aquat Toxicol. https://doi.org/10.1016/j.aquatox.2014.05.026
Guilhermino L, Lopes MC, Carvalho AP, Soares AMVM (1996) Acetylcholinesterase activity in Juveniles of Daphnia magna Straus. Bull Environ Contam Toxicol 57:979–985. https://doi.org/10.1007/s001289900286
Guimarães V, Sígolo JB (2008) Associação de Resíduos da Metalurgia com Sedimentos em Suspensão—Rio Ribeira de Iguape. Rev do Inst Geociências—USP 1–10
Gusso-Choueri PK, Araújo GSD, Cruz ACF et al (2018a) Metals and arsenic in fish from a Ramsar site under past and present human pressures: consumption risk factors to the local population. Sci Total Environ. https://doi.org/10.1016/j.scitotenv.2018.02.005
Gusso-Choueri PK, de Araújo GS, Cruz ACF et al (2018b) Metals and arsenic in fish from a Ramsar site under past and present human pressures: consumption risk factors to the local population. Sci Total Environ 628–629:621–630. https://doi.org/10.1016/j.scitotenv.2018.02.005
Hamza-Chaffai A (2014) Usefulness of bioindicators and biomarkers in pollution biomonitoring. Int J Biotechnol Wellness Ind 3:19–26. https://doi.org/10.6000/1927-3037.2014.03.01.4
Henry RP (1994) Morphological, behavioral, and physiological characterization of bimodal breathing crustaceans. Integr Comp Biol 34:205–215. https://doi.org/10.1093/icb/34.2.205
Henry RP, Lucu Č, Onken H, Weihrauch D (2012) Multiple functions of the crustacean gill: osmotic/ionic regulation, acid-base balance, ammonia excretion, and bioaccumulation of toxic metals. Front Physiol. https://doi.org/10.3389/fphys.2012.00431
Hirota J, Szyper JP (1975) Separation of total particulate carbon into inorganic and organic components and organic components: carbon into inorganic of total particulate separation. Limnol Oceanogr 20:896–900
Jameson SC, Tupper MH, Ridley JM (2002) The three screen doors: can marine “protected” areas be effective? Mar Pollut Bull 44:1177–1183
Jemec A, Drobne D, Tišler T, Sepčić K (2009) Biochemical biomarkers in environmental studies-lessons learnt from enzymes catalase, glutathione S-transferase and cholinesterase in two crustacean species. Environ Sci Pollut Res 17:571–581. https://doi.org/10.1007/s11356-009-0112-x
Jemec A, Škufca D, Prevorčnik S et al (2017) Comparative study of acetylcholinesterase and glutathione S-transferase activities of closely related cave and surface Asellus aquaticus (Isopoda: Crustacea). PLoS ONE. https://doi.org/10.1371/journal.pone.0176746
John DA, Leventhal JS (1994) Bioavailability of metals. In: Du Bray EA (ed) Preliminary compilation of descriptive geoenvironmental mineral deposit models. U.S. Denver, Colorado, pp 10–18
Keen H, Habig W, Jakoby B (1976) Mechanism for the several activities of the Glutathione S-Transferases. J Biol Chem 251: 6183–6188
Kennish MJ (1994) Pollution coastal in estuaries marine. J Coast Res, Special Issue No 12. Coastal Hazards: Perception Susceptibility and mitigation, pp 27–49
Kostić J, Kolarević S, Kračun-Kolarević M et al (2017) The impact of multiple stressors on the biomarkers response in gills and liver of freshwater breams during different seasons. Sci Total Environ 601–602:1670–1681. https://doi.org/10.1016/j.scitotenv.2017.05.273
Kummer L, Melo VF, Barros YJ, Azevedo JCR (2011) Sequential extraction of lead and zinc from soils of heavy metal mining and processing area. Rev Bras Ciência do Solo 35:2005–2018. https://doi.org/10.1590/S0100-06832011000600017
Lavradas RT, Hauser-Davis RA, Lavandier RC et al (2014) Metal, metallothionein and glutathione levels in blue crab (Callinectes sp.) specimens from southeastern Brazil. Ecotoxicol Environ Saf 107C:55–60. https://doi.org/10.1016/j.ecoenv.2014.04.013
Le Cren ED (1951) The length-weight relationship and seasonal cycle in gonad weight and condition in the Perch (Perca Fluviatilis). J Anim Ecol 20:201–219
Luczak C, Janquin MA, Kupka A (1997) Simple standard procedure for the routine determination of organic matter in marine sediment. Hydrobiologia 345:87–94. https://doi.org/10.1023/A:1002902626798
Mahiques M, Burone L, Figueira R et al (2009) Anthropogenic influences in a lagoonal environment: a multiproxy approach at the valo grande mouth, cananéia-iguape system (SE Brazil). Braz J Oceanogr 57:325–337
Mahiques MM, Figueira RCL, Salaroli AB et al (2013) 150 years of anthropogenic metal input in a Biosphere Reserve: the case study of the Cananéia-Iguape coastal system, Southeastern Brazil. Environ Earth Sci 68:1073–1087. https://doi.org/10.1007/s12665-012-1809-6
McCave IN, Syvitski JPM (2007) Principles, methods, and application of particle size analysis. Cambridge University Press, Cambridge. https://doi.org/10.1017/CBO9780511626142
Melo VF, Andrade M, Batista AH et al (2012) Chumbo e zinco em águas e sedimentos de área de mineração e metalurgia de metais. Quim Nov 35:22–29
Mohanty A (2017) Effects of water deprivation stress on GSH level in the mud crab Scylla serrata. Orissa University of Agriculture and Technology
Moreira LB, Vicente TM, Taniguchi S et al (2017) Assessing legacy contaminants in sediments from marine protected areas of the central coast of São Paulo (Brazil). Braz J Oceanogr 65:146–154
Nixon SW, Oviatt CA, Frithsen J, Sullivan B (1986) Nutrients and the productivity of estuarine and coastal marine ecosystems. J Limnol Soc S Afr 12:43–71. https://doi.org/10.1080/03779688.1986.9639398
Olive PL (1988) DNA precipitation assay: a rapid and simple method for detecting DNA damage in mammalian cells. Environ Mol Mutagen 11:487–495
Oliveira UO, Rosa Araújo AS, Belló-Klein A et al (2005) Effects of environmental anoxia and different periods of reoxygenation on oxidative balance in gills of the estuarine crab Chasmagnathus granulata. Comp Biochem Physiol Part B 140:51–57. https://doi.org/10.1016/j.cbpc.2004.09.026
Pan L, Zhang H (2006) Metallothionein, antioxidant enzymes and DNA strand breaks as biomarkers of Cd exposure in a marine crab, Charybdis japonica. Comp Biochem Physiol C Toxicol Pharmacol 144:67–75. https://doi.org/10.1016/j.cbpc.2006.06.001
Partelow S, von Wehrden H, Horn O (2015) Pollution exposure on marine protected areas: a global assessment. Mar Pollut Bull 100:352–358. https://doi.org/10.1016/j.marpolbul.2015.08.026
Péqueux A (1995) Osmotic regulation in crustaceans. Crustac Soc 15:1–60
Piedade TC, Melo VF, Souza LCP, Dieckow J (2014) Three-dimensional data interpolation for environmental purpose: lead in contaminated soils in southern Brazil. Environ Monit Assess 186:5625–5638. https://doi.org/10.1007/s10661-014-3808-4
Pinheiro MAA, Fransozo A (1993) A ~ Ãlise Da Relação Bio ~ Trica Do Peso Úmida Pela Largura Da Carapaça Para o Siri Arenaeus Cribrarius (La ~ Rck. Ibi8) (Crustacea. Brachyura. P ~ Rttm!Idae Y. Arq Biol Tecnol 36:331–341
Poljšak B, Fink R (2014) The protective role of antioxidants in the defence against ROS/RNS-mediated environmental pollution. Oxid Med Cell Longev 2014:22. https://doi.org/10.1155/2014/671539
Pozo K, Lazzerini D, Perra G et al (2009) Levels and spatial distribution of polychlorinated biphenyls (PCBs) in superficial sediment from 15 Italian Marine Protected Areas (MPA). Mar Pollut Bull 58:773–776. https://doi.org/10.1016/j.marpolbul.2009.03.003
Rainbow PS (1995) Biomonitoring of heavy metal availability in the marine environment. Mar Pollut Bull 31:183–192. https://doi.org/10.1016/0025-326X(95)00116-5
Rodrigues VGS, Fujikawa A, Abessa DMS et al (2012) Uso do bivalve límnico anodontites tenebricosus (LEA, 1834) No biomonitoramento de metais do rio Ribeira de Iguape. Quim Nov 35:454–459
Salaenoi J, Setthamongkol P, Srimeetian P (2015) Lipid peroxidation and antioxidant activity in molting stages of mud crab (Scylla serrata). Kasetsart J (Nat Sci) 49:422–432
Sedlak J, Lindsay H (1968) Estimation of total, protein-bound, and nonprotein sulfhydryl groups in tissue with Ellman’s reagent. Anal Biochem 25:192–205
Shinozaki-Mendes R, Manghi RF, Lessa R (2013) Comparative study of the molting cycle of wild and reared swimming crabs Callinectes danae (Crustacea: Portunidae). J Appl Ichthyol. https://doi.org/10.1111/jai.12236
Sies H, Koch OR, Martino E, Boveris A (1979) Increased biliary gluta-thione disulfide release in chronically ethanol-treated rats. FEBS Lett 103:287–290
Singaram G, Harikrishnan T, Chen F et al (2013) Modulation of immune-associated parameters and antioxidant responses in the crab (Scylla serrata) exposed to mercury. Chemosphere 90:917–928
Stohs SJ, Bagchi D (1995) Oxidative mechanisms in the toxicity of metal ions. Free Radic Biol Med 18:321–336. https://doi.org/10.1016/0891-5849(94)00159-H
Togni VG (2007) Efeito da salinidade sobre a resposta do sistema antioxidante e expressão de hsp70 em siris (Gênero Callinectes). Universidade Federal do Paraná
Tramonte KM, Figueira RCL, Majer AP et al (2018) Geochemical behavior, environmental availability, and reconstruction of historical trends of Cu, Pb, and Zn in sediment cores of the Cananéia-Iguape coastal system, Southeastern Brazil. Mar Pollut Bull 127:1–9. https://doi.org/10.1016/j.marpolbul.2017.11.016
UNEP (2006) Marine and coastal ecosystems and human well-being: a synthesis report based on the findings of the Millennium Ecosystem Assessment. J Bertrand Russell Arch 80
van der Oost R, Visa CP, van den Brink NW (2002) Biomarkers in environmental assessment. In: Biological testing in marine & freshwaters: trends, relevance and linkages to ecosystem health, chap 4. pp 87–152
Van Horn J, Malhoe V, Delvina M et al (2010) Molecular cloning and expression of a 2-Cys peroxiredoxin gene in the crustacean Eurypanopeus depressus induced by acute hypo-osmotic stress. Comp Biochem Physiol Part B 155:309–315. https://doi.org/10.1016/j.cbpb.2009.11.015
Vazzoler AEAM (1996) Biologia da Reprodução de Peixes Teleosteos: Teoria e Prática
Viarengo A, Ponzano E, Donderob F, Fabbrih R (1997) A simple spectrophotometric method for metallothionein evaluation in marine organisms: an application to Mediterranean and antarctic molluscs. Mar Environ Res 44:69–84
Vieira JLF, Gomes A (2010) Oxidative stress at different stages of the molting cycle of captive Coturnix coturnix. Res J Biol Sci 5:610–614
Vogt ÉL, Model JFA, Vinagre AS (2018) Effects of organotins on crustaceans: update and perspectives. Front Endocrinol (Lausanne) 9:1–8. https://doi.org/10.3389/fendo.2018.00065
Walters C, Cheng P, Poo E, Somerset V (2017) Combined silver nanoparticles and temperature effects in the Cape River crab potamanautes perlatus—interactions between chemical and climatic stressors. J Nanomater Mol Nanotechnol. https://doi.org/10.4172/2324-8777.1000216
Weis JS, Bergey L, Reichmuth J, Candelmo A (2011) Living in a contaminated estuary: behavioral changes and ecological consequences for five species. Bioscience 61:375–385. https://doi.org/10.1525/bio.2011.61.5.6
Wills ED (1987) Evaluation of lipid peroxidation in lipids and biological membranes. In: Snell K, Mullack B (eds) Biochemical toxicology: a practical approach. IRL Press, Oxford
Wolff LL (2007) Estrutura Populacional, Reprodução e Dinâmica Alimentar do lambari Astyanax sp. b (Characidae: Tetragonopterinae) em dois trechos do Rio das Pedras, Guarapuava—Paraná
Acknowledgments
The authors thank the São Paulo Research Foundation (FAPESP, grant #09/52762-6) for financial support and the National Council for Scientific and Technological Development (CNPq) for Giuliana S. Araujo’s master’s fellowship (grant #479899/2013-4). Paloma K. Gusso-Choueri thanks the Coordination for the Qualification of Higher Level Staff in Brazil (CAPES). Tatiana D. Saint' Pierre thanks CNPq for grant # 311820/2017-4. The authors are also grateful to the USP Oceanographic Institute for providing support to the field work in Cananéia. DMSA would like to thank CNPq for fellowships and financial support (grants #303620/2008-0, #308649/2011- and #311609/2014-7).
Author information
Authors and Affiliations
Corresponding author
Ethics declarations
Conflict of interest
The authors declare no conflict of interest.
Rights and permissions
About this article
Cite this article
Araujo, G.S., Gusso-Choueri, P.K., Favaro, D.I.T. et al. Metal-Associated Biomarker Responses in Crabs from a Marine Protected Area in Southeastern Brazil. Arch Environ Contam Toxicol 78, 463–477 (2020). https://doi.org/10.1007/s00244-020-00710-5
Received:
Accepted:
Published:
Issue Date:
DOI: https://doi.org/10.1007/s00244-020-00710-5