Abstract
Long duration of anesthesia may induce toxicity in the developing brain. However, little is known about the effects of the combination of surgery and anesthesia on the developing brain. The mechanisms for the effects are not clear. To determine these effects, postnatal day 7 male and female Sprague-Dawley rats were exposed to 3% sevoflurane for 2 h with or without right common carotid exposure. Pyrrolidine dithiocarbamate (PDTC), an anti-inflammatory agent, was given 30 min before and 6 h after the carotid exposure. Anti-glial cell-derived neurotrophic factor (GDNF) antibody or GDNF was given at the end of sevoflurane exposure. We found that anesthesia-surgery induced learning and memory impairment assessed by Barnes maze and fear conditioning. Anesthesia-surgery also induced neuroinflammation and reduced the level of glial cell-derived neurotrophic factor (GDNF, 10.6 ± 0.6 pg/mg protein of control rats vs. 7.7 ± 0.4 pg/mg protein of anesthesia-surgery rats, n = 17, p = 0.007) and neurogenesis in the hippocampus. PDTC inhibited these surgical effects (GDNF level 9.7 ± 0.6 pg/mg protein of anesthesia-surgery plus PDTC rats, n = 17, p = 0.763 vs. control rats). Intracerebroventricular injection of an anti-GDNF antibody but not its heat-inactivated form induced learning and memory impairment in control rats. Intracerebroventricular injection of GDNF attenuated learning and memory impairment after anesthesia-surgery. We conclude that anesthesia-surgery in neonatal rats induces neuroinflammation, which then leads to a decreased level of GDNF and neurogenesis in the hippocampus and cognitive impairment. GDNF decrease plays an important role in anesthesia-surgery-induced cognitive impairment.
Key message
-
Anesthesia-surgery in neonatal rats induces neuroinflammation.
-
Neuroinflammation leads to decreased levels of GDNF.
-
Neuroinflammation reduces hippocampal neurogenesis and induces cognitive impairment.
-
GDNF decrease is important for anesthesia-surgery-induced cognitive impairment.
Similar content being viewed by others
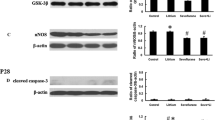
Avoid common mistakes on your manuscript.
Introduction
Long duration of anesthesia in neonatal rodents has been shown to induce brain cell apoptosis, neuroinflammation, and impairment of learning and memory [1, 2]. Obviously, it is important to understand the underlying pathophysiology for the impairment of learning and memory. The role of brain cell apoptosis in the learning and memory impairment has been questioned by recent studies [3, 4]. Although neuroinflammation is shown to contribute to the impairment of learning and memory [5, 6], anesthetic- and surgery-induced neuroinflammation shall be short-lived [6, 7]. Thus, it is not clear yet how an acute event, such as anesthetic exposure, can induce cognitive impairment weeks or months later.
Anesthetics may reduce neurogenesis [8, 9], a process that is involved in learning and memory [10–12]. It has been shown that neuroinflammation can reduce neurogenesis [13, 14]. Thus, it is possible that anesthetics can induce neuroinflammation that then reduces neurogenesis to lead to the delayed cognitive impairment.
Interestingly, previous basic science research has been focused on anesthetic effects on developing brain [1, 2]. However, patients are not usually given anesthesia without an accompanying surgical procedure. Surgery is a significant insult that can induce systemic inflammation [15]. We and others have shown that anesthetics including volatile anesthetics may provide protection against various insults [1, 16, 17]. Interestingly, little is known about the effects of the combination of anesthesia and surgery on learning and memory in neonatal animals. Sevoflurane is the most commonly used general anesthetic in pediatric patients. Thus, we designed this study to determine whether surgery under sevoflurane anesthesia induced learning and memory impairment in developing brain. If impairment was induced, whether the cascade of neuroinflammation-growth factor decrease-neurogenesis inhibition played a role in the impairment of learning and memory.
Materials and methods
The animal protocol was approved by the Institutional Animal Care and Use Committee of the University of Virginia (Charlottesville, VA). All animal experiments were carried out in accordance with the National Institutes of Health Guide for the care and use of laboratory animals (NIH publications number 80-23) revised in 2011.
Animal groups
Postnatal day 7 (PND7) male and female Sprague-Dawley rats were littermate-matched and randomly assigned to three groups in the first experiment: (1) control (not being exposed to anesthesia, surgery, or any drugs); (2) anesthesia with 3% sevoflurane for 2 h; and (3) anesthesia-surgery group (right carotid artery exposure under anesthesia with 3% sevoflurane for 2 h). In the second experiment, the rats were assigned to (1) control group, (2) pyrrolidine dithiocarbamate (PDTC) group, (3) anesthesia-surgery group, and (4) anesthesia-surgery plus PDTC group. PDTC, an anti-inflammatory agent, was given intraperitoneally 30 min before and 6 h after the surgery. In the third experiment, the neonatal rats were assigned to (1) control group, (2) heat-inactivated anti-glial cell-derived neurotrophic factor (GDNF) antibody group, and (3) anti-GDNF antibody group. In the last experiment, neonatal rats were assigned to (1) control group, (2) anesthesia-surgery group, and (3) anesthesia-surgery plus GDNF group. The heat-inactivated anti-GDNF antibody, anti-GDNF antibody, and GDNF peptide were injected intracerebroventricularly. Rats were started to be tested in Barnes maze and then fear conditioning from postnatal day 30.
Anesthesia and surgery
The surgery was a right carotid artery exposure [18]. Briefly, PND7 rats were anesthetized by 3% sevoflurane. During the procedure, the rat was breathing spontaneously with a facemask supplied with 100% oxygen. Rectal temperature was monitored and maintained at 37 °C with the aid of a heating blanket (TCAT-2LV, Physitemp Instruments Inc., Clifton, NJ). A 1.5-cm midline neck incision was made after the rat was exposed to sevoflurane at least for 30 min. Soft tissues over the trachea were retracted gently. One-centimeter-long right common carotid artery was carefully dissected free from adjacent tissues without any damage on the vagus nerve. Care was taken not to interrupt the blood flow in the artery for any significant amount of time (>30 s). The wound was then irrigated and closed by using surgical suture. The surgical procedure was performed under sterile conditions and lasted around 15 min. After the surgery, all animals received a subcutaneous injection of 6 mg/kg bupivacaine. Since this procedure was minimally invasive, additional medication for postoperative pain control was not needed based on the observation of animal activity and presentation. The total duration of general anesthesia was 2 h. No response to toe pinching was observed during the anesthesia. Rats in the sevoflurane anesthesia group also received bupivacaine injection to the neck.
Barnes maze
Twenty three days after being exposed to various experimental conditions, animals were subjected to Barnes maze to test their spatial learning and memory as previously described [18]. Barnes maze is a circular platform with 20 equally spaced holes (SD Instruments, San Diego, CA, USA). One of the holes was connected to a dark chamber called target box. The test was started by placing animals in the middle of the Barnes maze. Aversive noise (85 db) and bright light from a 200-W bulb shed on platform were used to provoke mice to find and enter the target box. Animals were first trained for 4 days with 3 min per trial, two trials per day, and 15-min interval between each trial. The memory test was carried out on day 5 (short-term retention) and day 12 (long-term retention). No test was performed during the period from days 5 to 12. The latency to enter the target box during each trial was recorded by an ANY-Maze video tracking system (SD Instruments).
Fear conditioning
Fear conditioning test was performed 24 h after the Barnes maze test was completed as we previously described [18]. Rats were placed in a test chamber wiped with 70% alcohol and subjected to three tone-foot shock pairings (tone 2000 Hz, 85 db, 30 s; foot shock 1.0 mA, 2 s) with 1-min inter-trial interval in a relatively dark room. Rats were removed from the test chamber 30 s after training and returned to their regular cages. Rats were placed back 24 h later to the same chamber for 8 min without tone and shock. The freezing behavior was recorded in an 8-s interval. Two hours later, mice were placed in a new test chamber that had different context and smell from the first test chamber. This chamber was wiped with 1% acetic acid and was in a relatively light room. After no stimulation for 3 min, the tone stimulus was then turned on for three cycles with each cycle for 30 s followed by 1-min inter-cycle interval (total 4.5 min). The freezing behavior in this 4.5 min was recorded too. All freezing behavior was recorded by a camera, and the video was scored by an observer who was blinded to the group assignment of animals.
BrdU administration and immunofluorescent staining
Rats received two doses of 50 mg/kg 5′-bromo-2′-deoxyuridine (BrdU; Sigma-Aldrich) given intraperitoneally 16 and 40 h after the surgery [9] and sacrificed 64 h after surgery to harvest the hippocampus for staining BrdU or co-staining BrdU and SRY (sex-determining region Y)-box 2 (Sox-2), a marker for multi-potential neural stem cells [19], or sacrificed 23 days after surgery to harvest the hippocampus for staining BrdU or co-staining BrdU and neuronal nuclei (NeuN), a marker for mature neurons [20]. Rats were sacrificed 64 h after the surgery to harvest the hippocampus for staining ionized calcium-binding adaptor molecule 1 (Iba-1), a marker for microglial activation [7, 18].
To harvest the hippocampus, rats were deeply anesthetized by sevoflurane and then perfused with normal saline. The hippocampus was fixed in 4% paraformaldehyde in 0.1 M phosphate-buffered saline at 4 °C for 24 h and embedded in paraffin. Four-micron-thick coronal brain sections were cut sequentially from bregma −1 to −4 mm. Antigen retrieval was performed by incubating the sections with sodium citrate buffer containing 10 mM sodium citrate and 0.05% Tween 20 (pH 6.0) at 95–100 °C for 20 min. DNA denaturation was done by incubating with 1 N HCl (catalogue number: H1758, Sigma-Aldrich) on ice for 3 min and 2 N HCl at room temperature for 3 min and at 37 °C for 6 min. Sections were blocked with 5% normal donkey serum and 1% bovine serum albumin in Tris-buffered saline containing 0.5% Triton-X 100 for 2 h at room temperature. The sections were then incubated overnight at 4 °C with the following primary antibodies: rat monoclonal anti-BrdU antibody (1:100 dilution, catalogue number: ab6326 Abcam), goat polyclonal anti-Sox-2 antibody (1:100 dilution, catalogue number: sc-17320; Santa Cruz Biotechnology), mouse monoclonal anti-NeuN antibody (1:200 dilution, catalogue number: MAb377; Millipore), or rabbit polyclonal anti-Iba-1 antibody (1:500 dilution, catalogue number: 019-19741; Wako Chemicals USA, Richmond, VA). The sections were incubated with donkey anti-rat IgG antibody conjugated with Alexa Fluor 594 (1:200 dilution, catalogue number: A-21209; Invitrogen), donkey anti-goat IgG antibody conjugated with Alexa Fluor 488 (1:200 dilution, catalogue number: A-11055; Invitrogen), donkey anti-mouse IgG antibody conjugated with Alexa Fluor 488 (1:200 dilution, catalogue number: A-21202; Invitrogen), or donkey anti-rabbit IgG antibody conjugated with Alexa Fluor 488 (1:200 dilution, catalogue number: A-21206; Invitrogen) for 1 h at room temperature in a dark room. After washed in phosphate-buffered saline, sections were counterstained with Hoechst 33342 (Thermo Scientific), rinsed, and mounted with Vectashield mounting medium (H-1000; Vector Labs, Burlingame, CA).
For each rat brain, six sequential hippocampal sections were used for cell counting. The number of all cells positively stained for an interesting marker or the combination of two markers in the dentate gyrus of each section was counted. The Iba-1 immunoreactivity in the sections was measured as we described before [18]. Briefly, images of two non-overlapping fields in the dentate gyrus area per section were randomly acquired. Four sections per rat were imaged. The areas whose immunostaining intensity was above a predetermined threshold level were considered as positively stained areas and quantified using the ImageJ 1.47n software (National Institutes of Health, Bethesda, MD). The immunoreactivity to Iba-1 was quantified by the percentage area with positive Iba-1 staining to the total area of the imaged field. All quantitative analyses were performed in a blinded manner.
Preparation of nuclear and cytosolic extracts of the hippocampus and Western blotting
The hippocampus was harvested 24 h after the surgery. The cytoplasmic and nuclear fractionations were performed using the nuclear and cytoplasmic extraction reagents according to the manufacturer’s instructions (Pierce Biotechnology, IL). A protease inhibitor cocktail (catalogue number: P2714, Sigma) was added to the extraction reagents. Fractionation efficiency was confirmed by examining cytoplasm (β-actin)- and nucleus (histone 3)-specific markers with immunoblotting. Nuclear protein extracts were quantitated by using a BCA protein assay kit (23225 Pierce Biotechnology, IL).
Twenty micrograms of proteins per lane was separated on a polyacrylamide gel and then blotted onto a polyvinylidene difluoride membrane. The membranes were blocked with Protein-Free T20 blocking buffer (catalogue number: 37573, Thermo Scientific, Logan, UT) and incubated with the following primary antibodies overnight at 4 °C: rabbit monoclonal anti-nuclear factor (NF)-κB p65 antibody (1:1000 dilution, catalogue number: 8242; Cell Signaling Technology, Danvers, MA) or rabbit polyclonal anti-histone H3 antibody (1:1000 dilution, catalogue number: 9715; Cell Signaling Technology). Goat anti-rabbit IgG-HRP secondary antibody (1:5000 dilution, catalogue number: sc-2004; Santa Cruz) was used. Protein bands were visualized by Genesnap version 7.08 and quantified by Genetools version 4.01. The relative protein expression of nuclear p65 was normalized to that of histone H3. The results from animals under various experimental conditions then were normalized by the mean values of the corresponding control animals.
ELISA assay of neurotrophic factors in the hippocampus
The protein levels of brain-derived neurotrophic factor (BDNF) and glial cell-derived neurotrophic factor (GDNF) at 48 h after surgery were determined by using ELISA kits (catalogue number: BEK-2000 and BEK-2230, Biosense Laboratories AS, Bergen, Norway) according to the manufacturer’s instruction and as described previously [9]. Briefly, the hippocampus was homogenized on ice in the RIPA buffer containing 25 mM Tris-HCl (pH 7.6), 150 mM NaCl, 1% NP-40, 1% sodium deoxycholate, and 0.1% sodium dodecyl sulfate (catalogue number: 89901; Thermo Scientific, Rockford, IL) as well as protease inhibitor cocktail (10 mg/ml aproteinin, 5 mg/ml peptastin, 5 mg/ml leupetin, and 1 mM phenylmethanesulfonylfluoride) (Sigma-Aldrich). Homogenates were centrifuged at 13,000×g for 20 min at 4 °C. The supernatant was collected and used for ELISA detection. The amount of BDNF and GDNF in each sample was then normalized by its protein content.
Intracerebroventricular injection of an anti-GDNF antibody or GDNF
In the third and fourth experiments, some rats received intracerebroventricular injection of 3 μl (500 μg/ml) rabbit polyclonal anti-GDNF antibody (catalogue number: ab18956; Abcam, Cambridge, MA) based on a previous study [18]. Others received the injection of 3 μl heat-denatured (5 min at 100 °C) anti-GDNF antibody. Some rats received intracerebroventricular injection of 0.3 μg recombinant rat GDNF (catalogue number: 512-GF; R&D Systems, Minneapolis, MN) in 3 μl phosphate-buffered saline (PBS) as described in previous studies [18, 21]. Each rat received only one injection to the right lateral ventricle immediately at the end of the surgery. We injected only once because proteins injected into cerebroventricles could be in the brain tissues for a long time. For example, GDNF was still detected in the brain tissues at least 7 days after its intracerebroventricular injection [22].
The intracerebroventricular injection was performed as we described before [18] with the aid of a stereotactic apparatus (SAS-5100, ASI Instruments, Warren, MI) using the following coordinates: 1.0 mm posterior to bregma, 1.5 mm lateral from midline, and 4.0 mm ventral from the surface of the skull. After the injection, the needle was kept in place for 1 min to prevent backflow of the injected solution. Rats were anesthetized with 3% sevoflurane during the injection. They were turned from supine position during surgery to prone position in a stereotactic apparatus during the injection.
Statistical analysis
Results were presented as means ± SEM (n ≥ 6) when the data were in normal distribution or in box plot if the data were not in normal distribution. The data from the training sessions of Barnes maze test within the same group were tested by one-way repeated measures analysis of variance followed by Tukey’s test. Data from the training sessions of Barnes maze between groups were analyzed by two-way repeated measures analysis of variance followed by Tukey’s test. The other data were analyzed by one-way analysis of variance followed by Tukey’s test if the data were normally distributed or by one-way analysis of variance on ranks followed by the Tukey test if the data were not normally distributed. Differences were considered significant at a p < 0.05 based on two-tailed hypothesis testing. All statistical analyses were performed with SigmaStat (Systat Software, Inc., Point Richmond, CA, USA).
Results
All animal survived anesthesia and surgery and the following observation period. Data from all animals are included for analysis. Rats in the control, sevoflurane, and anesthesia-surgery groups took less time to identify the target hole on day 4 than on day 1 in the training sessions of Barnes maze. Anesthesia-surgery but not sevoflurane anesthesia alone was a significant factor to influence the time to identify the target hole in the training sessions [F(1,29) = 6.035, p = 0.020 for anesthesia-surgery and F(1,29) = 3.722, p = 0.064 for sevoflurane anesthesia]. Rats in the anesthesia-surgery group also took a longer time than control rats to identify the target hole 1 and 8 days after the training sessions. Similarly, surgical rats also had less freezing behavior than control rats in context-related fear conditioning. Rats exposed to sevoflurane did not take significantly more time to identify the target hole during the memory phase of Barnes maze test and did not have less freezing behavior in the context-related fear conditioning than control rats. Neither sevoflurane nor anesthesia-surgery affected the tone-related fear conditioning (Fig. 1). In the second experiment, the results of rats subjected to anesthesia-surgery were very similar to those of rats with anesthesia-surgery in the first experiment described above. Although PDTC alone did not affect the learning and memory of rats assessed by Barnes maze and fear conditioning, PDTC attenuated anesthesia-surgery-induced prolongation in time to identify the target hole and decreased freezing behavior in context-related fear conditioning (Fig. 2).
Surgery impaired learning and memory assessed by Barnes maze and fear conditioning. a Performance during the training sessions of Barnes maze test. b Performance during the memory phase of Barnes maze test. c Performance during the fear conditioning test. Results are mean ± SEM (panels a and c) or in box plot (panel b) (n = 15–16). *p < 0.05 compared with the results on day 1 of the same group of animals. ^ p < 0.05 compared with the control group. Sevo sevoflurane, Sur surgery
PDTC attenuated surgery-induced learning and memory impairment. a Performance during the training sessions of Barnes maze test. b Performance during the memory phase of Barnes maze test. c Performance during the fear conditioning test. Results are mean ± SEM (panel a ) or in box plot (panels b and c ) (n = 15–18). *p < 0.05 compared with the results on day 1 of the same group of animals. ^ p < 0.05 compared with the control group. # p < 0.05 compared with the sevoflurane-surgery group. Sevo sevoflurane, Sur surgery
Anesthesia-surgery but not sevoflurane alone increased the Iba-1 expression in the hippocampus. This increase was inhibited by PDTC. Similarly, anesthesia-surgery increased the nuclear p65, an NFκB subunit, and this increase was attenuated by PDTC (Fig. 3). Anesthesia-surgery and sevoflurane did not affect the expression of BDNF in the hippocampus. However, anesthesia-surgery reduced the expression of GDNF at 48 h after surgery and PDTC attenuated this reduction (Fig. 4). Consistent with the decrease of GDNF, anesthesia-surgery also reduced brain cell genesis. The newly formed neural progenitor cells as positively marked by Sox2 and mature neurons as marked by NeuN in the hippocampus were decreased by anesthesia-surgery. These decreases were attenuated by PDTC (Fig. 5).
PDTC attenuated surgery-induced neuroinflammation. a Representative images of Iba-1 (green) and Hoechst 33342 (blue) staining, scale bar in the panels = 200 μm. b Quantification of Iba-1 immunoreactivity in the dental gyrus (DG). c Representative images of Western blots of nuclear proteins. d Quantitative results of Western blots. Results are mean ± SEM (n = 6–8 for panel b, = 8 for the left panel of panel d, and = 14 for the right panel of panel d). *p < 0.05 compared with the control group. ^ p < 0.05 compared with the sevoflurane-surgery group. Sevo sevoflurane, Sur surgery
PDTC attenuated surgery-induced decrease of GDNF at 48 h after surgery. a BDNF concentrations in the hippocampus. b GDNF concentrations in the hippocampus. Results are mean ± SEM (n = 9 for panel a, = 9 for the left panel of panel b, and = 14–17 for the right panel of panel b). *p < 0.05 compared with the control group. ^ p < 0.05 compared with the sevoflurane-surgery group. Sevo sevoflurane, Sur surgery
PDTC attenuated surgery-induced neurogenesis. a Representative images of Hoechst 33342 (blue), BrdU (red), and Sox2 (green) staining. b Quantification of BrdU-positive cells or cells positive for both BrdU and Sox2 in the dental gyrus. c Representative images of Hoechst 33342 (blue), BrdU (red), and NeuN (green) staining. d Quantification of BrdU-positive cells or cells positive for both BrdU and NeuN in the dental gyrus. e Representative images of Hoechst 33342 (blue), BrdU (red), and Sox2 (green) staining. f Quantification of BrdU-positive cells or cells positive for both BrdU and Sox2 in the dental gyrus. g Representative images of Hoechst 33342 (blue), BrdU (red), and NeuN (green) staining. h Quantification of BrdU-positive cells or cells positive for both BrdU and NeuN in the dental gyrus. Scale bar in panels a, c, e, and g = 200 μm. Results are mean ± SEM (n = 6–8 for panels b, d, f, and h). *p < 0.05 compared with the control group. ^ p < 0.05 compared with the sevoflurane-surgery group. Sevo sevoflurane, Sur surgery
To determine whether decreased GDNF plays a role in the anesthesia-surgery-induced learning and memory impairment, two sets of experiments were performed. First, intracerebroventricular injection of an anti-GDNF antibody increased the time needed for control rats to identify the target hole 1 or 8 days after training sessions in Barnes maze. Also, anti-GDNF antibody but not its heat-inactivated form is a significant factor to affect the time for rats to identify the target hole in the training sessions of Barnes maze [F(1,43) = 9.937, p = 0.003 for the antibody and F(1,42) = 0.0058, p = 0.94 for its heat-inactivated form]. The anti-GDNF antibody also reduced the freezing behavior in the context-related but not tone-related fear conditioning. However, the heat-inactivated anti-GDNF antibody did not have any effects on learning and memory assessed by Barnes maze and fear conditioning (Fig. 6). In the second experiment, anesthesia-surgery increased the time for rats to identify the target hole 1 and 8 days after the training sessions in the Barnes maze and reduced the context-related freezing behavior in fear conditioning as described above. These surgical effects were attenuated by intracerebroventricular injection of GDNF (Fig. 7).
Anti-GDNF antibody induced learning and memory impairment. a Performance during the training sessions of Barnes maze test. b Performance during the memory phase of Barnes maze test. c Performance during the fear conditioning test. Results are mean ± SEM (panels a and c) or in box plot (panel b) (n = 22–23). *p < 0.05 compared with the results on day 1 of the same group of animals. ^ p < 0.05 compared with the control group
GDNF attenuated surgery-induced learning and memory impairment. a Performance during the training sessions of Barnes maze test. b Performance during the memory phase of Barnes maze test. c Performance during the fear conditioning test. Results are mean ± SEM (panels a and c) or in box plot (panel b) (n = 21–22). *p < 0.05 compared with the results on day 1 of the same group of animals. ^ p < 0.05 compared with the control group. # p < 0.05 compared with the sevoflurane-surgery group
Discussion
Sevoflurane is the most commonly used general anesthetic in children in the USA. It has been shown that sevoflurane exposure can induce brain cell apoptosis, neuroinflammation, and cognitive impairment in neonatal rodents [1, 2]. Of note, this exposure needs to be relatively long time (>3 h) or be repeated (2 h daily for 3 days) [1–3]. However, sevoflurane anesthesia at surgical plane for 5 h in 6-day-old cynomolgus monkeys did not cause learning and memory deficits and behavioral abnormality [23]. Consistent with these studies, sevoflurane anesthesia for 2 h did not induce neuroinflammation, decrease neurogenesis, and impair learning and memory in this current study. Similar to our findings, a recent study showed that a 6-h exposure of 21-day-old mice to isoflurane induced immediate brain cell death but did not affect total neuronal number and neurogenesis in the hippocampus evaluated 2 weeks after the exposure [24]. However, anesthesia is often performed to help patients to get through surgery. Interestingly, the effects of the combination of sevoflurane and surgery on developing brain of animals are not known yet. Our results showed that this combination induced neuroinflammation, reduced neurogenesis, and impaired learning and memory. These results suggest that surgery may be a strong stimulus to induce brain injury in the developing brain.
Neuroinflammation has been shown to contribute to cognitive dysfunction after anesthesia and surgery in adult animals [5, 6]. However, neuroinflammation usually lasts for days [6, 7]. Cognitive function is often tested weeks after the anesthetic exposure in the neonatal animals [1, 2]. Events downstream of neuroinflammation to lead to the delayed cognitive impairment are not clear. Neurogenesis is important for learning and memory [10–12]. Sevoflurane exposure can reduce neurogenesis [8]. Thus, it is possible that neuroinflammation results in reduction of neurogenesis, which leads to the delayed learning and memory impairment. Consistent with this possibility, our results showed that PDTC, an NFκB inhibitor [25, 26], attenuated the neuroinflammation, decrease of neurogenesis, and impairment of learning and memory after the surgery and anesthesia. These results suggest the role of neuroinflammation in the decreased neurogenesis and impaired learning and memory of developing brain. In addition, we further showed that anesthesia-surgery reduced GDNF and that PDTC attenuated this reduction. Since neurogenesis required growth factors [9, 27], our results suggest a contribution of reduced GDNF to the decreased neurogenesis after anesthesia-surgery.
The role of GDNF in the anesthesia-surgery-induced learning and memory impairment is supported by two lines of evidence. First, an anti-GDNF antibody but not its heat-inactivated form induced learning and memory impairment in control rats. Second, GDNF attenuated anesthesia-surgery-induced learning and memory impairment. These results strongly suggest anesthesia-surgery-induced decrease of GDNF contributes to the impairment of learning and memory after anesthesia-surgery. Thus, our findings suggest the following event cascade: anesthesia-surgery induces neuroinflammation that decreases growth factors including GDNF, which then reduces neurogenesis. This effect ultimately contributes to the delayed learning and memory impairment. In addition to the PDTC, GDNF, and anti-GDNF antibody data that support this event cascade, our data indicate active neuroinflammation 1 day after anesthesia-surgery, decreased GDNF 2 days after anesthesia-surgery, and reduced neurogenesis thereafter, which is consistent with the proposed event cascade. Interestingly, our study may be the initial study to provide evidence for the inhibition of GDNF expression by neuroinflammation. However, it is known that GDNF can inhibit neuroinflammation [18, 28, 29]. Thus, reduced GDNF production and neuroinflammation may form a vicious cycle, which ultimately leads to reduced neurogenesis and the development of learning and memory impairment.
We and others have shown that GDNF is mainly produced in astrocytes [18, 28, 29]. Thus, our current study suggests a protective role of astrocytes against anesthesia-surgery-induced neurotoxicity. Astrocytes reduce isoflurane-induced neuronal injury in the mouse neuron-astrocytes co-cultures, possibly by buffering over-expressed pro-brain-derived neurotrophic factor [30]. Astrocytes express a large number of glutamate transporters that are important to maintain extracellular glutamate homeostasis and to prevent glutamate excitotoxicity [31, 32]. On the other hand, microglia produce pro-inflammatory mediators [33] and, therefore, may play a detrimental role in the effects of anesthesia-surgery on developing brain.
Other mechanisms have been reported to contribute to sevoflurane-induced injury to developing brain in the literature. For example, inhibition of extracellular signal-regulated kinases is reported to play a role in sevoflurane-induced brain cell apoptosis [34]. Over-phosphorylation of tau has also been shown to lead to learning and memory impairment after sevoflurane exposure [35]. It is not clear yet how these mechanisms may interact with the pathway we identified here to result in cognitive impairment of the youth.
We performed right carotid arterial exposure to P7 rats. Although abdominal surgeries are commonly performed in pediatrics, these surgical procedures can affect their bowel functions, which may affect their nutrition status. Our laboratory has standardized the carotid arterial exposure procedure. It does not affect the motor functions like surgeries on extremities or bowel functions as in the case of abdominal surgeries. Thus, carotid arterial exposure is a surgical procedure that will most likely not to affect the general well-being of the animals. Also, surgeries, such as thyroglossal cyst removal, do involve neck incision in pediatric patients.
We started to measure rat learning and memory when they were 30 days old. This time is 23 days after the exposure to anesthesia-surgery. Maturation from progenitors to neurons takes 2 to 4 weeks in rats [36]. Waiting for this length of time is necessary to measure the effects of anesthesia-surgery on neurogenesis and the contribution of these effects to learning and memory impairment. However, puberty is associated with synaptic loose and improvement in a reversal task [37, 38]. The onset of puberty in female rats is about 10 days earlier than that in male rats (postnatal day 35 vs. postnatal day 45) [38]. Interestingly, space learning and memory are similar in rats just before and after the onset of puberty [37]. Space learning and memory were major functions we measured here.
Anesthetic-induced brain cell apoptosis in the developing brain was initially considered to contribute to the learning and memory impairment [1]. However, anesthetics can induce brain cell apoptosis in neonatal mice but do not cause learning and memory impairment later in these mice [4]. Also, sevoflurane may not cause brain cell apoptosis in neonatal rats but induce cognitive impairment [3]. Thus, the role of brain cell apoptosis in the learning and memory impairment is not clear yet. Therefore, we did not study brain cell apoptosis but focused on identifying mechanisms contributing to the learning and memory impairment after surgery under sevoflurane anesthesia in this study.
Although anesthetic-induced neurotoxicity has been a focus of research for the last decade, clinical evidence for this effect is very limited [1]. Some retrospective studies showed that children with more than one surgery before 4 years of age had impairment of learning and memory [39–42]. However, many retrospective studies did not show an effect [43–46]. Recently, a prospective study has shown that neonates who had inguinal hernia repair under either sevoflurane anesthesia or regional anesthesia were not different in neurodevelopmental outcome at 2 years of age [47]. In addition, children who had one inguinal hernia surgery before 3 years of age were not different from their sibling in neurocognitive outcome when they were 8 to 15 years old [48]. Although these two latest multi-center studies included children who had a relatively short surgical procedure, they did not show that surgery and anesthesia significantly impair learning and memory of children later in their life. Also, most of the studies included children who had surgery. Thus, it is not known yet whether anesthetic-induced neurotoxicity shown in rodents is clinically relevant yet. Nevertheless, surgery and anesthesia may be significant insults to the developing brain. Understanding this risk and potential mechanisms for the possible brain injury shall be important for basic science research and improving outcome of pediatric patients after surgery. Thus, future clinical investigation focusing on identifying risks and laboratory research determining mechanisms are needed to move forward the field of the effects of anesthesia and surgery on developing brain.
Our study has limitations. First, it is very difficult to fully monitor the neonatal rats during anesthesia and surgery. We only monitored their arterial blood oxygenation by a pulse oximeter and maintained their body temperature. Second, we performed carotid arterial exposure. Although this type of surgery may not be performed in children, surgeries, such as thyroglossal cyst removal, do involve neck incision in pediatric patients.
In summary, we have shown that surgery under sevoflurane anesthesia induced neuroinflammation, decrease of neurogenesis, and impairment of learning and memory. GDNF plays an important role in anesthesia-surgery-induced learning and memory impairment.
Author contributions
ZZ conceived the project. LG and ZZ designed the study, and LG and XL performed the experiments. LG did the initial data analysis and drafted the “Materials and methods” section. ZZ performed the final data analysis and wrote the manuscript.
References
Chiao S, Zuo Z (2014) A double-edged sword: volatile anesthetic effects on the neonatal brain. Brain Sci 4:273–294
Shen X, Dong Y, Xu Z, Wang H, Miao C, Soriano SG, Sun D, Baxter MG, Zhang Y, Xie Z (2013) Selective anesthesia-induced neuroinflammation in developing mouse brain and cognitive impairment. Anesthesiology 118:502–515
Lu Y, Huang Y, Jiang J, Hu R, Yang Y, Jiang H, Yan J (2016) Neuronal apoptosis may not contribute to the long-term cognitive dysfunction induced by a brief exposure to 2% sevoflurane in developing rats. Biomed Pharmacother 78:322–328
Yang B, Liang G, Khojasteh S, Wu Z, Yang W, Joseph D, Wei H (2014) Comparison of neurodegeneration and cognitive impairment in neonatal mice exposed to propofol or isoflurane. PLoS One 9:e99171
Cao L, Li L, Lin D, Zuo Z (2012) Isoflurane induces learning impairment that is mediated by interleukin 1beta in rodents. PLoS One 7:e51431
Cibelli M, Fidalgo AR, Terrando N, Ma D, Monaco C, Feldmann M, Takata M, Lever IJ, Nanchahal J, Fanselow MS et al (2010) Role of interleukin-1beta in postoperative cognitive dysfunction. Ann Neurol 68:360–368
Zhang J, Jiang W, Zuo Z (2014) Pyrrolidine dithiocarbamate attenuates surgery-induced neuroinflammation and cognitive dysfunction possibly via inhibition of nuclear factor kappaB. Neurosci 261:1–10
Fang F, Xue Z, Cang J (2012) Sevoflurane exposure in 7-day-old rats affects neurogenesis, neurodegeneration and neurocognitive function. Neurosci Bull 28:499–508
Fan D, Li J, Zheng B, Hua L, Zuo Z (2016) Enriched environment attenuates surgery-induced impairment of learning, memory, and neurogenesis possibly by preserving BDNF expression. Mol Neurbiol 53:344–354
van Praag H, Schinder AF, Christie BR, Toni N, Palmer TD, Gage FH (2002) Functional neurogenesis in the adult hippocampus. Nature 415:1030–1034
Shors TJ, Townsend DA, Zhao M, Kozorovitskiy Y, Gould E (2002) Neurogenesis may relate to some but not all types of hippocampal-dependent learning. Hippocampus 12:578–584
Sakamoto M, Imayoshi I, Ohtsuka T, Yamaguchi M, Mori K, Kageyama R (2011) Continuous neurogenesis in the adult forebrain is required for innate olfactory responses. Proc Natl Acad Sci U S A 108:8479–8484
Wang B, Jin K (2015) Current perspectives on the link between neuroinflammation and neurogenesis. Metab Brain Dis 30:355–365
Song C, Zhang Y, Dong Y (2013) Acute and subacute IL-1beta administrations differentially modulate neuroimmune and neurotrophic systems: possible implications for neuroprotection and neurodegeneration. J Neuroinflammation 10:59
Zhang J, Tan H, Jiang W, Zuo Z (2015) The choice of general anesthetics may not affect neuroinflammation and impairment of learning and memory after surgery in elderly rats. Journal Neuroimmune Pharm 10:179–189
Lee JJ, Li L, Jung H-H, Zuo Z (2008) Postconditioning with isoflurane reduced ischemia-induced brain injury in rats. Anesthesiology 108:1055–1062
Zhao P, Peng L, Li L, Xu X, Zuo Z (2007) Isoflurane preconditioning improves long-term neurologic outcome after hypoxic-ischemic brain injury in neonatal rats. Anesthesiology 107:963–970
Zhang J, Tan H, Jiang W, Zuo Z (2014) Amantadine alleviates postoperative cognitive dysfunction possibly by increasing glial cell line-derived neurotrophic factor in rats. Anesthesiology 121:773–785
Ellis P, Fagan BM, Magness ST, Hutton S, Taranova O, Hayashi S, McMahon A, Rao M, Pevny L (2004) SOX2, a persistent marker for multipotential neural stem cells derived from embryonic stem cells, the embryo or the adult. Dev Neurosci 26:148–165
Wolf HK, Buslei R, Schmidt-Kastner R, Schmidt-Kastner PK, Pietsch T, Wiestler OD, Blumcke I (1996) NeuN: a useful neuronal marker for diagnostic histopathology. J Histochem Cytochem 44:1167–1171
Zhang Z, Miyoshi Y, Lapchak PA, Collins F, Hilt D, Lebel C, Kryscio R, Gash DM (1997) Dose response to intraventricular glial cell line-derived neurotrophic factor administration in parkinsonian monkeys. J Pharmacol Exp Ther 282:1396–1401
Lapchak PA, Jiao S, Collins F, Miller PJ (1997) Glial cell line-derived neurotrophic factor: distribution and pharmacology in the rat following a bolus intraventricular injection. Brain Res 747:92–102
Zhou L, Wang Z, Zhou H, Liu T, Lu F, Wang S, Li J, Peng S, Zuo Z (2015) Neonatal exposure to sevoflurane may not cause learning and memory deficits and behavioral abnormality in the childhood of cynomolgus monkeys. Sci Rep 5:11145
Jiang Y, Tong D, Hofacer RD, Loepke AW, Lian Q, Danzer SC (2016) Long-term fate mapping to assess the impact of postnatal isoflurane exposure on hippocampal progenitor cell productivity. Anesthesiology 125:1159–1170
Wang Z, Zhao H, Peng S, Zuo Z (2013) Intranasal pyrrolidine dithiocarbamate decreases brain inflammatory mediators and provides neuroprotection after brain hypoxia-ischemia in neonatal rats. Exp Neurol 249:74–82
Li H, Yin J, Li L, Deng J, Feng C, Zuo Z (2013) Isoflurane postconditioning reduces ischemia-induced nuclear factor-kappaB activation and interleukin 1beta production to provide neuroprotection in rats and mice. Neurobiol Dis 54:216–224
Kobayashi T, Ahlenius H, Thored P, Kobayashi R, Kokaia Z, Lindvall O (2006) Intracerebral infusion of glial cell line-derived neurotrophic factor promotes striatal neurogenesis after stroke in adult rats. Stroke 37:2361–2367
Rocha SM, Cristovao AC, Campos FL, Fonseca CP, Baltazar G (2012) Astrocyte-derived GDNF is a potent inhibitor of microglial activation. Neurobiol Dis 47:407–415
Chen SH, Oyarzabal EA, Sung YF, Chu CH, Wang Q, Chen SL, Lu RB, Hong JS (2015) Microglial regulation of immunological and neuroprotective functions of astroglia. Glia 63:118–131
Stary CM, Sun X, Giffard RG (2015) Astrocytes protect against isoflurane neurotoxicity by buffering pro-brain-derived neurotrophic factor. Anesthesiology 123:810–819
Zuo Z (2001) Isoflurane enhances glutamate uptake via glutamate transporters in rat glial cells. Neuroreport 12:1077–1080
Robinson MB (1999) The family of sodium-dependent glutamate transporters: a focus on the GLT-1/EAAT2 subtypes. Neurochem Int 33:479–491
Yin J, Li H, Feng C, Zuo Z (2014) Inhibition of brain ischemia-caused notch activation in microglia may contribute to isoflurane postconditioning-induced neuroprotection. CNS NEUROL DISORD-DR 13:718–732
Yufune S, Satoh Y, Akai R, Yoshinaga Y, Kobayashi Y, Endo S, Kazama T (2016) Suppression of ERK phosphorylation through oxidative stress is involved in the mechanism underlying sevoflurane-induced toxicity in the developing brain. Sci Rep 6:21859
Tao G, Zhang J, Zhang L, Dong Y, Yu B, Crosby G, Culley DJ, Zhang Y, Xie Z (2014) Sevoflurane induces tau phosphorylation and glycogen synthase kinase 3beta activation in young mice. Anesthesiology 121:510–527
Snyder JS, Choe JS, Clifford MA, Jeurling SI, Hurley P, Brown A, Kamhi JF, Cameron HA (2009) Adult-born hippocampal neurons are more numerous, faster maturing, and more involved in behavior in rats than in mice. J Neurosci 29:14484–14495
Willing J, Drzewiecki CM, Cuenod BA, Cortes LR, Juraska JM (2016) A role for puberty in water maze performance in male and female rats. Behav Neurosci 130:422–427
Drzewiecki CM, Willing J, Juraska JM (2016) Synaptic number changes in the medial prefrontal cortex across adolescence in male and female rats: a role for pubertal onset. Synapse 70:361–368
Wilder RT, Flick RP, Sprung J, Katusic SK, Barbaresi WJ, Mickelson C, Gleich SJ, Schroeder DR, Weaver AL, Warner DO (2009) Early exposure to anesthesia and learning disabilities in a population-based birth cohort. Anesthesiology 110:796–804
DiMaggio C, Sun LS, Kakavouli A, Byrne MW, Li G (2009) A retrospective cohort study of the association of anesthesia and hernia repair surgery with behavioral and developmental disorders in young children. J Neurosurg Anesthesiol 21:286–291
Dimaggio C, Sun LS, Li G (2011) Early childhood exposure to anesthesia and risk of developmental and behavioral disorders in a sibling birth cohort. Anesth Analg 113:1143–1151
Flick RP, Katusic SK, Colligan RC, Wilder RT, Voigt RG, Olson MD, Sprung J, Weaver AL, Schroeder DR, Warner DO (2011) Cognitive and behavioral outcomes after early exposure to anesthesia and surgery. Pediatrics 128:e1053–e1061
Kalkman CJ, Peelen L, Moons KG, Veenhuizen M, Bruens M, Sinnema G, de Jong TP (2009) Behavior and development in children and age at the time of first anesthetic exposure. Anesthesiology 110:805–812
Bartels M, Althoff RR, Boomsma DI (2009) Anesthesia and cognitive performance in children: no evidence for a causal relationship. Twin Res Hum Genet 12:246–253
Hansen TG, Pedersen JK, Henneberg SW, Morton NS, Christensen K (2013) Educational outcome in adolescence following pyloric stenosis repair before 3 months of age: a nationwide cohort study. Paediatr Anaesth 23:883–890
Hansen TG, Pedersen JK, Henneberg SW, Pedersen DA, Murray JC, Morton NS, Christensen K (2011) Academic performance in adolescence after inguinal hernia repair in infancy: a nationwide cohort study. Anesthesiology 114:1076–1085
Davidson AJ, Disma N, de Graaff JC, Withington DE, Dorris L, Bell G, Stargatt R, Bellinger DC, Schuster T, Arnup SJ et al (2016) Neurodevelopmental outcome at 2 years of age after general anaesthesia and awake-regional anaesthesia in infancy (GAS): an international multicentre, randomised controlled trial. Lancet 387:239–250
Sun LS, Li G, Miller TL, Salorio C, Byrne MW, Bellinger DC, Ing C, Park R, Radcliffe J, Hays SR et al (2016) Association between a single general anesthesia exposure before age 36 months and neurocognitive outcomes in later childhood. JAMA 315:2312–2320
Author information
Authors and Affiliations
Corresponding author
Ethics declarations
Grant support
This study was supported by grants (GM098308 and NS094030 to Z Zuo) from the National Institutes of Health, Bethesda, MD, the Robert M. Epstein Professorship endowment, University of Virginia, Charlottesville, VA, and a grant (81641160) from National Natural Science Foundation of China, Beijing, China.
Conflict of interest
The authors declare that they have no competing interests.
Rights and permissions
About this article
Cite this article
Gui, L., Lei, X. & Zuo, Z. Decrease of glial cell-derived neurotrophic factor contributes to anesthesia- and surgery-induced learning and memory dysfunction in neonatal rats. J Mol Med 95, 369–379 (2017). https://doi.org/10.1007/s00109-017-1521-9
Received:
Revised:
Accepted:
Published:
Issue Date:
DOI: https://doi.org/10.1007/s00109-017-1521-9