Abstract
The implant-mediated drug delivery system (IMDDS) is a novel, innovative device that allows drug delivery through bone marrow. The purpose of this study was to investigate the effect of an active plunger component made of super absorbent polymer (SAP) on the plasma concentration of dexamethasone released from the IMDDS. The IMDDSs were installed in a total of 18 rabbits. After complete healing, dexamethasone was loaded with the SAP active plunger and with water to cause expansion in the test group (n = 9), while only the drug was loaded in the control group, as per the original protocol (n = 9). The release patterns of each group were monitored for 2 weeks by measuring the plasma concentration of the drug. Both groups showed sustained release of drug. However, the test groups showed more rapid increase in plasma concentration and higher area under the curve (AUC) throughout the observation period. The incorporation of a SAP active plunger component in the IMDDS resulted in an increase in initial release of drug and higher bioavailability within the observation period of 2 weeks after dexamethasone administration.
Similar content being viewed by others
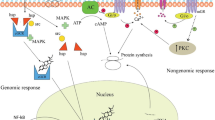
Explore related subjects
Discover the latest articles, news and stories from top researchers in related subjects.Avoid common mistakes on your manuscript.
Introduction
The implant-mediated drug delivery system (IMDDS), first introduced in 2014, is a novel application for dental implants [1]. It was uniquely devised for the systemic delivery of drugs for chronic diseases, rather than for local drug delivery [2, 3]. Its design was based on hollow cylinder titanium implants in which a drug cartridge containing the intended drug can be accommodated. The fixture part of the IMDDS has multiple holes that allow for drug diffusion into the bone marrow space, through which the released drug molecules then enter into the systemic circulation. The major advantages of this system include a sustained release profile and needle-free painless parenteral delivery. In addition, the system acts as a permanent gateway, connecting the internal body with the outside environment, for repetitive and on-demand use. In this regard, the route of administration itself has been termed “Osseogate” [4].
Previous studies have shown promising results, and evaluations of the drug delivery efficacy using several candidate chronic medications are being performed using the IMDDS in animal models [2, 5]. In addition, the sustained release from IMDDS installed in a canine mandible was confirmed [4]. However, a substantial amount of essential information regarding this new system will need to be accumulated in order for it to be used clinically. One of the important issues pointed out in previous studies is that the prototype IMDDS has no active controlling components for drug release; rather, the delivering mechanism depends mainly on diffusion at the interface between drug cartridges and intrabony tissue. Therefore, the release pattern of a specific drug is generally dependent upon its physicochemical characteristics [5, 6].
In order to deliver the drug molecule as intended when using the IMDDS, several approaches can be proposed. For example, modification of drug cartridges is worth investigating so that the amount of drug released can be adjusted to the physiology of the bone marrow circulation. This can be achieved by either modifying the drug itself or through the use of an appropriate carrier. Furthermore, the incorporation of an active component into the IMDDS is a possibility [2]. If there were a plunger incorporated into this system, as with other injection types of administration, it would be possible to actively discharge the pharmaceutical substance into the bone marrow space.
For this purpose, there is need for a mechanism that operates spontaneously in response to a specific stimulus, without the involvement of manual handling. From various plausible options, the super absorbent polymer (SAP), which is common and easy to acquire, was chosen in the present study for the role of active plunger [7, 8]. The SAP, originally developed for the agricultural purpose of water conservation in soil, has undergone technical improvements and is now used frequently in everyday life in products such as feminine sanitary napkins, adult incontinence products, and, most prevalently, in baby diapers [9]. Prior to the advent of SAP, water-absorbing materials were usually cellulosic or fiber-based and retained water at levels at most about 20 times their weight. In contrast, these polyelectrolyte gels swell and absorb water up to several hundred times their dried weights [10]. They are lightly cross-linked hydrophilic polymers, and their superior swelling capacities originate from the electrostatic repulsion between the charges on the polymers. Since they respond to a specific environmental stimulus by changing size, they have been called “smart materials” and have been a focus of interest not only from fundamental, but applied perspectives [9].
The SAP has a very simple mechanism of action in that it swells in response to moisture, resulting in extrusion of the drug powder. The aim of the present study was to evaluate the effect of an active plunger made of SAP on the release pattern of dexamethasone when administered via the IMDDS in a rabbit tibia model.
Materials and methods
Animals
A total of 18, 10-week-old male New Zealand white rabbits, each weighing 2.5 to 3.0 kg, were used in this study. Animal selection, management, and surgical protocols conformed to the guidelines of the Institutional Animal Care and Use Committee of Seoul National University, Seoul, Korea. Before the experiments, an acclimation period of 7 days was allowed. The rabbits were housed in individual cages, and standard rabbit diet and water were provided ad libitum.
Implant-mediated drug delivery system
The custom devices were made of grade IV titanium using a milling machine (Fig. 1). The description of the device is provided in detail in a previous report [1]. A dexamethasone drug cartridge was inserted into the bottom of a hollow cylinder. The cover screw used with the test group was different from that used with the control group and was designed with a hole for moisture passage. However, prior to drug loading, a cover screw without a hole was used for both groups to ensure tight sealing.
Implant-mediated drug delivery system (IMDDS) (a) and schematic diagram of the implant-mediated drug delivery system with and without the active plunger, which releases drug through a novel route of administration, known as Osseogate. Green portion is plunger made of super absorbent polymer (SAP) and the violet portion is drug powder (b, c, d). The control IMDDS without an active plunger (b). The IMDDS with SAP plunger at the time of drug loading (c). The IMDDS with SAP plunger when the polymer expanded after contact with moisture (d). The drugs are pushed out through the hole of intrabony part of IMDDS
Preliminary experiments on SAP efficacy
The SAP balls were made of sodium polyacrylate and had a diameter of 2 mm. The swelling capacities (Q) of SAP in distilled water and in normal saline were measured as described previously [11]. The Q was calculated according to the equation, Q (wt/wt) = (Wwet − Wdry)/Wdry,, where Wwet and Wdry are the weights of the wet and dried gels, respectively.
The STL file for the IMDDS was imported into prototype software (Clinet software) and submitted to an RP machine (Project HD 3000, 3D Systems, Rock Hill, SC, USA) for fabrication of the actual sized model. The accuracy of an RP machine ranges between ± 0.001 and 0.002 in. (0.025; 0.05 mm). The mock IMDDS made of transparent acrylic photopolymer (VisiJett EX200 Plastic, 3D Systems) was constructed by overlaying a 32-μm layer of resin polymerized with ultraviolet light curing in high-definition mode.
The preliminary experiments were performed using two conditions. In the first instance, the mock IMDDS was filled with mock drug material, one SAP ball, and two drops of distilled water. As the mock drug material, poly vinyl siloxane impression material with high viscosity was used (Vonflex S Putty, Vericom Co., Ltd., Chuncheon, Korea). In the second condition, only one SAP ball was inserted in the IMDDS. A cover screw with a hole was tightened. The IMDDSs were then inverted onto a petri dish filled with saline to a level below the first hole. They were visually observed following a set time schedule (0, 1, 2, 4, 8, 12, 24, 48, 72, 120, and 168 h).
Surgical procedures
Prior to bringing the rabbits to the surgical room, their hind legs were shaved. The animals were then anesthetized with an intramuscular injection of 10 mg/kg zolazepam and 0.15 ml/kg xylazine hydrochloride, and local anesthesia comprised of 2% lidocaine with 1:100,000 epinephrine (0.5 to 1 ml/site) was applied around the surgical site. The site was accessed with 3-cm-long periosteal incision through the skin. The flat lateral surface of the proximal tibia metaphysis was selected for IMDDS installation. The IMDDS was placed after sequential site preparation using a series of increasing diameter twist drills and a screw tap. Each rabbit received one IMDDS randomly in the left or right tibia. The cover screw without a hole was tightened to seal the IMMDS, as described. The surgical site was closed in layers with appropriate absorbable sutures. The surgery procedure was performed using an aseptic technique. After surgery, each rabbit received a non-narcotic, non-steroidal analgesic agent for pain control and a broad-spectrum antibiotic for infection control.
Loading of dexamethasone
Dexamethasone powder (D1756-1G, Sigma-Aldrich, St Louis, MO, USA) was prepared as 10 mg cartridges. A single cartridge of the drug was then inserted into the implant. For the test group of rabbits (n = 9), one SAP ball was inserted as an active plunger component upon the drug cartridge. In addition, one water drop of approximately 2 cm3 was applied to initiate expansion, and the cover screw with a hole was tightened (Fig. 1). For the control group of rabbits (n = 9), only the cover screw without a hole was tightened.
Measurement of dexamethasone plasma concentrations
Blood samples of 3 ml were collected from the marginal vein of the ear at predetermined time intervals ranging from immediately after drug administration to the follow-up time of 2 weeks. The time schedules for blood sampling were as follows: 0, 1, 2, 4, 6, 8, 12, and 24 h and then once daily from 2 to 14 days. The samples were collected in heparinized tubes and divided into two 1.5 mL tubes for centrifugation. The gathered plasma was centrifuged (3000 rpm, 10 min at 4 °C). The plasma concentration of the drug was determined using liquid chromatography tandem mass spectroscopy (LC-MS/MS System, AB SCIEX, Framingham, MA) at each time point.
Statistical analysis
Repetitive observations over time within individuals were correlated for each rabbit. To control for this correlated data structure, a mixed-effects analysis was applied. Statistical analyses were performed using the R statistical language, and p values < 0.05 were considered statistically significant.
Results
In the preliminary observation of SAP properties, the swelling capacity was 125.3-fold compared to baseline in distilled water and 41.5-fold in 0.9% normal saline. The extrusion of mock materials was confirmed after 40 min in the specimens treated with two drops of water prior to cover screw tightening. As time progressed, the materials showed the appearance of pressed tooth paste, which was caused by expansion of SAP (Fig. 2).
There were no abnormalities, mobility issues, or inflammation of the implant sites during the observation period after placement. The cumulative release profiles of the drug were obtained for a total of 18 rabbits. There were considerable individual variations in the plasma concentrations in each experimental rabbit. In both groups, the release profiles showed no lag time immediately after drug administration and a sustained pattern. However, there was a difference between the two groups in that the plasma concentration was significantly higher in the test group than in the control group during the entire observation period.
Animals in both groups demonstrated maximum plasma concentration at 8 h after drug administration. The plasma concentrations of the test group measured from day 1 to day 5 were at least five times higher than those of control groups. Interestingly, a decreasing tendency of drug concentration in the control group was reversed to increase slightly from the measurement at 2 days. This tendency was not found in the test group. The concentration in the test group then decreased for the remainder of the observation period. Despite this decrease, dexamethasone was detected in the plasma throughout the observation period in both groups. The calculated areas under the curve (AUCs) for 2 weeks of observation for the test and control groups were 5.98 and 1.87 mg/ml h, respectively (Fig. 3).
Discussion
The present study took advantage of the peculiar swelling property of SAP and introduced it as an active plunger component in the IMDDS. This active plunger expanded with contact of an initial water drop, and then the moisture from the surrounding tissue penetrated the drug powder. The expanded polymer expelled the drug powder into the bone marrow space around the IMDDS. The amount of release in the test group during this expelling movement was hypothesized to be much greater than that of the control group. The results of the study validated this hypothesis. In addition, the results are in line with the other applications in the aspect of sustained release of dexamethasone [12, 13].
In deionized and distilled water, normal SAP is known to absorb up to 500 times its weight, equaling 30 to 60 times its own volume. This absorbency decreases to around one-tenth in normal saline solution (0.9%) [14]. The swelling capacity of the polyacrylamide SAP used in this study was about 41.5 times its original weight in the preliminary in vitro experiment. The expelling efficiency of this SAP is thought to be dependent on many factors, such as the material strength of the swollen gel, the physicochemical properties of the drug powder, and the characteristics of the tissue surrounding the IMDDS. The mock drug material loaded in the transparent plastic IMDDS model was successfully pushed out after 40 min when imbedding in the water; however, without the provision of additional water, there could be no further expansion or powder release. In the animal experiment, a cover screw with a hole and one drop of water for initiation of expansion were applied because there was nearly no moisture contact possible at drug loading, and the hole was expected to act as a continuous source of moisture. Interestingly, a sustained release profile was evident not only in the test group, but also in the control group. Possibly, the moisture from blood flow contacted the SAP plunger with some elimination of drug powder.
A drug delivery system (DDS) is a pharmaceutical formulation or device for introducing drug molecules into the body in order to improve the efficacy to safety ratio by modulating the pharmacokinetic and pharmacodynamic properties of a specific drug [15, 16]. The IMDDS can be categorized as the latter, a device, but it is composed of many components such as a fixture, a cover, a diffusion hole, a drug cartridge, and the “active plunger.” Therefore, it has been named a “system.” Traditional plungers are one component of an injection syringe or pump, and they can be moved along a barrel to take in or expel liquid drug. The design of a plunger is known to affect the pump performance and infusion compliance [17]. There are many types of plunger-incorporated drug delivery devices in which the plungers are modified according to the system’s intended purposes [18,19,20,21]. The SAP incorporated in the IMDDS in this study was not the traditional elastomeric type and had the role of expelling drug powder through expansion triggered by water contact. The most notable advantage of this system is no need for needle injection compared with other types of drug delivery devices incorporating plungers.
The physicochemical properties of a specific drug, such as water solubility, are known to affect the release profiles of a prototype IMDDS [6]. In the previous study, the release profile from two drugs of different solubility, i.e., metformin and dexamethasone, was compared. The results demonstrated that the metformin diffused into plasma more rapidly compared with the dexamethasone. Thus, highly soluble drugs like metformin or pilocarpine require slow release to allow for prolonged action. Another previous experiment showed that gelatin hydrogel vehicle can slow the release speed of the highly soluble drug, the pilocarpine [5]. In contrast, some drugs, such as dexamethasone, require active propulsion into bone marrow in order to achieve a fast effect or to maintain the plasma concentration within the therapeutic window.
Exact control of drug release is one of the most important hurdles for the IMDDS to be used clinically. There are many factors to affect the release pattern in addition to the physicochemical properties of drug. In one previous study of our group, the release features of the same drug, the dexamethasone is somewhat different from the present study, probably due to the differences in the anatomic site and experimental animal species [4]. The intrabony structure and circulation of canine mandible model are quite different from the rabbit tibia model used in the present study. In this regard, the understanding of diverse basic features regarding the delivery though the bone marrow of a specific installed site is a prerequisite for precise control. In addition, numerous subsequent studies should be performed to reveal the science of IMDDS delivery, which include changing the amount and kinds of drug as well as the polymer plunger. However, since the capacity of prototype IMDDS is fixed to 20 cm3 as for now, regardless of any other factors such as drug, animal, and anatomic site, the pharmacokinetics and pharmacodynamics of experiments are subject to the maximum limit of loading volume. In other words, the amount of drug and the size of plunger are in an inverse relationship, without changing the design of prototype IMDDS. According to the result from several pilot study, it seemed that the bigger the size of the SAP plunger, the more vigorous release of the dexamethasone occurred. However, with the increase of the SAP plunger size, the loaded amount of dexamethasone had to be decreased. Therefore, sustaining periods would be shortened subsequent to the depletion of the drug. With this in mind, our team is currently performing further studies using spacers to make the comparison under the same condition, while designing other IMDDS of different loading capacity.
The SAP of the present system showed some promise in active infusion but, as a prototype application, do not have capability for on-demand control like an insulin pump [3]. In fact, many kinds of active infusion modules could be combined in this IMDDS. In this respect, incorporation of a micro-electro-mechanical system (MEMS) is highly recommended for delicate control according to specific needs [18, 22, 23]. In other words, the constant plasma concentration of a drug can be achieved in the presence of an on-demand mechanism of temporal control [24, 25]. Further studies should take into consideration differences between species and anatomical sites.
References
Park YS, Cho JY, Lee SJ, Hwang CI. Modified titanium implant as a gateway to the human body: the implant mediated drug delivery system. Biomed Res Int. 2014;2014:801358.
Park YS, Lee SJ, Hwang CI. New application of endosseous implants—implant mediated drug delivery system (IMDDS). J Korean Dent Assoc. 2014;52:550–7.
Park YS. Novel route of insulin delivery using an implant-mediated drug delivery system. Drug Deliv Transl Res. 2017;7:286–91.
Park JH, Cha HS, Kim HK, Park YS. Dexamethasone delivery by an implant-mediated drug delivery system in the canine mandible. J Periodontol. 2016:1–9.
Cha S, Kim HK, Kho HS, Park YS. The sustained effects on tear volume of pilocarpine hydrochloride in gelatin by hydrogel administered by an implant-mediated drug delivery system. Curr Drug Deliv. 2017;14:581–6.
Kim HK, Park YS. Plasma concentration of metformin and dexamethasone after administration through Osseogate. Drug Deliv. 2017;24:437–42.
Kabiri K, Omidian H, Hashemi SA, Zohuriaan-Mehr MJ. Synthesis of fast-swelling superabsorbent hydrogels: effect of crosslinker type and concentration on porosity and absorption rate. Eur Polym J. 2003;39:1341–8.
Ono T, Sugimoto T, Shinkai S, Sada K. Lipophilic polyelectrolyte gels as super-absorbent polymers for nonpolar organic solvents. Nat Mater. 2007;6:429–33.
Omidian H, Rocca JG, Park K. Advances in superporous hydrogels. J Control Release. 2005;102:3–12.
Ullah F, Othman MB, Javed F, Ahmad Z, Md Akil H. Classification, processing and application of hydrogels: a review. Mater Sci Eng C Mater Biol Appl. 2015;57:414–33.
Elyashevich GK, Bel’nikevich NG, Vesnebolotskaya SA. Swelling-contraction of sodium polyacrylate hydrogels in media with various pH values. Polym Sci Ser A. 2009;51:550–3.
El Kechai N, Mamelle E, Nguyen Y, Huang N, Nicolas V, Chaminade P, et al. Hyaluronic acid liposomal gel sustains delivery of a corticoid to the inner ear. J Control Release. 2016;226:248–57.
Kalam MA. The potential application of hyaluronic acid coated chitosan nanoparticles in ocular delivery of dexamethasone. Int J Biol Macromol. 2016;89:559–68.
Horne WJ, Andrews MA, Terrill KL, Hayward SS, Marshall J, Belmore KA, et al. Poly(ionic liquid) superabsorbent for polar organic solvents. ACS Appl Mater Interfaces. 2015;7:8979–83.
Gupta S, Jain A, Chakraborty M, Sahni JK, Ali J, Dang S. Oral delivery of therapeutic proteins and peptides: a review on recent developments. Drug Deliv. 2013;20:237–46.
Jain KK. Drug delivery system. Second edition. ed: Humana Press; 2008.
Weiss M, Fischer J, Neff T, Baenziger O. The effects of syringe plunger design on drug delivery during vertical displacement of syringe pumps. Anaesthesia. 2000;55:1094–8.
Borot S, Franc S, Cristante J, Penfornis A, Benhamou PY, Guerci B, et al. Accuracy of a new patch pump based on a microelectromechanical system (MEMS) compared to other commercially available insulin pumps: results of the first in vitro and in vivo studies. J Diabet Sci Technol. 2014;8:1133–41.
King BR, Goss PW, Paterson MA, Crock PA, Anderson DG. Changes in altitude cause unintended insulin delivery from insulin pumps: mechanisms and implications. Diabetes Care. 2011;34:1932–3.
Waldner C, Friedl HE, Dunnhaupt S, Kollner S, Leonaviciute G, Bernkop-Schnurch A. Development of a dosage form for accelerated release. Int J Pharm. 2014;471:189–96.
Xie X, Yang Y, Yang Y, Li Z, Zhang H, Chi Q, et al. The development and evaluation of a subcutaneous infusion delivery system based on osmotic pump control and gas drive. Drug Deliv. 2015:1–12.
Elman NM. The next generation of drug delivery system and diagnostics based on micro-electro-mechanical-system (MEMS) and nanotechnology. Curr Pharm Biotechnol. 2010;11:318–9.
Wang J, Hong B, Kai J, Han J, Zou Z, Ahn CH, et al. Mini sensing chip for point-of-care acute myocardial infarction diagnosis utilizing micro-electro-mechanical system and nano-technology. Adv Exp Med Biol. 2009;645:101–7.
Gandhi BR, Mundada AS, Gandhi PP. Chronopharmaceutics: as a clinically relevant drug delivery system. Drug Deliv. 2011;18, 18(1)
Yoo JW, Doshi N, Mitragotri S. Adaptive micro and nanoparticles: temporal control over carrier properties to facilitate drug delivery. Adv Drug Deliv Rev. 2011;63:1247–56.
Funding
This research was supported by a grant from the Korea Health Technology R&D Project through the Korea Health Industry Development Institute (KHIDI), funded by the Ministry of Healthand Welfare, Republic of Korea (Grant no. H I13C1474).
Author information
Authors and Affiliations
Corresponding author
Ethics declarations
Conflict of interest
The authors declare that they have no conflict of interest.
Rights and permissions
About this article
Cite this article
Yang, ST., Park, YS. Release pattern of dexamethasone after administration through an implant-mediated drug delivery device with an active plunger of super absorbent polymer. Drug Deliv. and Transl. Res. 8, 702–707 (2018). https://doi.org/10.1007/s13346-018-0516-6
Published:
Issue Date:
DOI: https://doi.org/10.1007/s13346-018-0516-6