Abstract
Background/objectives
Oral sensorial perception is a key aspect in food choices and knowing the mechanisms modulating such perception is of major importance in the context of child obesity, which is reaching high rates in Mediterranean countries. Salivary proteome has been linked to taste sensitivity in adults. The aim of this study was to search for differences in salivary proteomes of children with different bitter or sweet taste sensitivities and to assess if these potential differences are associated with their body mass index percentile (BMI percentile).
Subjects/methods
387 children aged 8–9 years old were assessed for BMI percentile and classified according to their sensitivity to bitter and sweet tastes, according to their caffeine and sucrose detection thresholds, respectively. Saliva protein composition was compared among taste sensitivity groups, taking into account BMI percentile and gender, using gel-based proteomics approaches, coupled to mass spectrometry for protein identification.
Results
Among the salivary proteins related to bitter taste sensitivity, higher levels of cystatins were observed in bitter-sensitive children, in the case of those of normal weight, and in bitter low-sensitive, in the case of overweight children. For sweetness, the relationship between saliva and taste perception was also dependent on BMI percentile, with several proteins (including salivary cystatins) differing between taste sensitivity groups, with disparities arising between normal-weight and overweight children. Cystatin isoforms A, B and SA were observed to be considerably increased in saliva from obese children.
Conclusions
Salivary proteome is related with sensitivities to bitter and sweet tastes in children, but the association is dependent on BMI percentile and gender.
Similar content being viewed by others
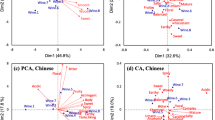
Introduction
The promotion of healthy dietary habits is one of the keys to combat children overweight and obesity, which reaches percentages as high as 28% in some southern European countries [1]. Although improvements have been observed in the last decade, in Portugal 30.7% of children are currently overweight, with 11.7% being obese [2]. Food preferences and choices are a result of the interaction of different factors, including sensory perception, emotional context and social aspects [3]. The relationship between sensorial perception of basic tastes and food preferences during childhood has been the focus of a number of studies. Some of these attest to the influence of bitter taste perception on food preferences, with bitterness being one of the reported reasons for avoiding fruit and vegetables [4]: for example, responsiveness to the bitterness compounds PTC (phenylthiocarbamide) and PROP (6-n-propylthiouracil) has been related to higher levels of preference for vegetables by children who are less sensitive to these bitter compounds [5]; nevertheless, this relationship is not observed in all studies [reviewed in ref. [6]]. For sourness, it was reported that children have a tendency for higher preferences for beverages perceived as less sour [7]. In terms of sweet taste, the liking for it represents an innate response, aimed at supporting the search for energy-rich foods. However, differences in inter-individual sweet taste sensitivity may influence food preferences and choices. Recently, we observed that sweet taste sensitivity is positively correlated with the preference for vegetables, although this correlation is influenced by gender (Rodrigues et al., data not published). This reinforced that sweet taste sensitivity may be an important determinant for food choices in children. Despite some authors failing to observe a relationship between sweet taste sensitivity and BMI [8] during childhood, this does not mean the absence of consequences in later life.
Variations in taste sensitivity among individuals is known to be influenced by genetic factors, namely polymorphisms in genes codifying for bitter and sweet taste receptors and/or for proteins involved in taste cell maintenance, such as gustin [9]. Moreover, aspects such as age, dietary habits or medical complications may also influence taste perception. Over recent years, evidence has emerged that saliva composition may also contribute to taste sensitivity: in adults, differences in salivary proteome have been observed to correlate with inter-individual differences in the perception of bitter [10,11,12], sweet [13] or salty [14] tastes; for children, the influence of saliva in taste sensitivity has been less investigated, but one study by Morzel and colleagues [15] reported that 3–6 month-old babies with higher salivary amounts of carbonic anhydrase VI or zinc-α-2-glycoprotein and lower salivary amounts of S-type cystatins have a lower acceptance of bitterness. However, in the latter study, no assessment of the sensitivity to this basic taste was performed. We recently showed that normal-weight female children with higher levels of salivary leptin present lower sweet taste sensitivity, reinforcing the association between saliva and taste sensitivity and the influence of factors such as gender in such association [16].
Recently, it was reported that taste perception is changed in obesity, suggesting that BMI may be a factor influencing taste sensitivity phenotypes [17]. Moreover, differences in obese salivary proteome have already been reported by our team [18]. As such, when looking for the existence of a relationship between saliva and taste sensitivity it is necessary to take an individual’s BMI into account.
This study has two main aims: (1) to look for differences in salivary proteome for children with high or low sensitivities to bitter and sweet tastes; (2) to investigate how BMI percentile (BMI percentile) and gender might be associated with such differences in proteome. Sweet and bitter tastes were the ones selected for this study, since they elicit opposite innate affective responses.
Material and methods
Participants
Three hundred eighty-seven Caucasian children (199 boys and 188 girls), aged 8–9 years old, from the 3rd year of basic schooling at schools in Évora (Alentejo region, south of Portugal), all in apparent good health, participated in this study. Only children presenting no signs of poor respiratory or oral health were allowed to the study. This design was chosen to have a representative sample. Tests were performed in the morning and children were advised not to eat nor drink anything other than water 1.5 h before testing. Anthropometric parameters (height and weight) were collected at the beginning of the sessions, as described elsewhere [16]. Children were classified according to World Health Organization (WHO) standards, which defines overweight (pre-obesity + obesity) as a BMI equal or greater than +1 standard deviation of reference median equivalent to the 85th percentile (and coinciding with BMI of 25 kg/m2 of adult age), and obesity when BMI is equal or greater than +1 standard deviation of reference median equivalent to 95th percentile (and coinciding with BMI of 30 kg/m2 of adult age).
For taste tests and saliva collection, parents or tutors were informed of the procedures and objectives of the study and gave their written informed consent. All procedures were in accordance with the Declaration of Helsinki for Medical Research involving Human Subjects and were approved by the institutional ethical committees (University of Évora and Alentejo Health Regional Administration).
Taste sensitivity tests
Sweet and bitter taste sensitivities, through sucrose and caffeine thresholds respectively, were assessed as described by Rodrigues and colleagues [16]. In brief, solutions prepared with distilled water were presented in ascendant order of concentration (3.0, 4.0, 8.0, 12.0, and 16.0 g/l of sucrose and 0.15, 0.20, 0.25, 0.30, and 0.40 mg/mL of caffeine). Children also received a cup with the same distilled water used to prepare the solutions. Tests were carried out as a game, in which children were detectives and searched for the solutions that differed in taste from water. For each taste, the lower stimuli concentration detected as differing from water was considered the threshold of the respective taste. Sweet and bitter taste sets of solutions were presented in the same session, in a randomised order. Children were classified in sensitivity groups for bitter and sweet tastes according to their caffeine and sucrose detection thresholds respectively. If their threshold detection was below the median threshold concentration of the full sample, it was considered as sensitive; otherwise, it was low-sensitive.
Saliva collection, flow rate, total protein and glucose determination
Before taste tests, unstimulated whole saliva was collected using the drooling technique. Each child rinsed his/her mouth with water, after which they received instructions to drain all the saliva produced during 4 min into a tube maintained on ice. Saliva obtained was frozen at −20 °C. After that, samples were thawed on ice and centrifuged at 13,000g for 30 min, at 4 °C, to remove mucinous and insoluble material.
The saliva flow rate was calculated by weighing the tubes with the saliva (considering that saliva has a density of 1 g/mL) and dividing the weight by the 4 min of collection. Total protein concentration was determined using the Bradford method. Salivary glucose levels were determined for a subsample of 98 children (51 boys and 47 girls), encompassing normal-weight and obese children. Determinations were performed using the colorimetric Kit Glucose (GO) Assay (Sigma-Aldrich, Inc.), following manufacturer recommendations, as described above [13].
SDS-PAGE electrophoresis
Saliva samples from 285 children (151 boys and 134 girls) were subjected to SDS-PAGE. The remaining 102 samples were not used for SDS-PAGE, as they contained a low concentration of protein or very low volumes. Of these 285 children, whose salivary profiles were separated by SDS-PAGE, 33 could not be classified according to their sensitivity for bitterness and 11 could not be classified according to their sensitivity for sweet taste, due to a lack of meaningful answers in taste sensitivity tests (e.g., reporting low concentrations as different and high concentrations as equal to water). As such, SDS-PAGE salivary protein profiles corresponded to: 67 and 66 boys high- and low-sensitive for bitterness, respectively; 49 and 70 girls high- and low-sensitive for bitterness, respectively; 49 and 96 boys high- and low-sensitive for sweetness, respectively; and 47 and 82 girls high- and low-sensitive for sweetness, respectively. Each sample was run in triplicate. A total of 7.5 µg protein from each saliva sample was run on each lane, in a 14% polyacrylamide mini-gel (Protean xi, Bio-Rad, California, USA), using a Laemmli buffer system [19]. An electrophoretic run was performed at a constant voltage of 140 V until front dye reached the end of the gel. Gels were fixed for 1 h in 40% methanol/10% acetic acid, followed by staining for 2 h with Coomassie Brilliant Blue (CBB) G-250. Gel images were acquired using a scanning Molecular Dynamics densitometer with internal calibration and LabScan software (GE, Healthcare), and images were analysed using Gel Analyzer software (GelAnalyzer 2010a by Istvan Lazar, www.gelanalyzer.com) for the percentage of volume of each protein band. Molecular masses were determined in accordance with molecular mass standards (Bio-Rad Precision Plus Protein Dual Colour 161–0394) run with protein samples.
Two-dimensional electrophoresis
Taking into account the diversity of factors to be compared (gender, BMI percentile group, sweet taste sensitivity group and bitter sensitivity group), together with technical and analytical requirements for two-dimensional electrophoresis (2-DE), 16 saliva pools were constituted. Each pool was obtained by mixing an equal total protein amount of 10 individual samples from the same gender and with similar BMI and taste sensitivity characteristics. The aim was to have pools representative of each condition to be compared (Table 1).
Only normal weight and obese (and not pre-obese) children were considered, in order of having groups of ‘extreme’ bodily condition scores.
For 2-DE, a volume of each saliva pool, corresponding to 175 µg total protein, were desalted and concentrated using 3 kDa cut-off ultra-filtration microfuge tubes (Nanosep 3 K omega, PALL Corporation, Madrid, Spain). After that, proteins were subjected to isoelectric focusing in 13 cm pH 3–10NL IPG strips, for 30 kVh, followed by vertical separation according to molecular masses in 14% acrylamide gels, following the protocol described elsewhere [20]. Each saliva pool was run in triplicate.
Digital images of the 2-DE gels were acquired using the same procedure previously described for SDS-PAGE image acquisition. Gel analysis was performed using Image Master Platinum v.7 software (Amersham Biosciences, Europe GmbH, Freiburg, Germany), with automatic spot detection, followed by manual editing for spot splitting and noise removal.
Protein identification by mass spectrometry
Identification of differently expressed proteins by MALDI TOF-TOF
After analysis of SDS-PAGE and two-dimensional electrophoretic profiles, the protein bands/spots differently expressed among groups were excised and tryptic digested with porcine trypsin (Sequencing Grade Modified Trypsin, Promega) following the protocol previously described elsewhere [12].
Protein identification was performed in a MALDI TOF-TOF (4800 plus AB Sciex) mass spectrometer using the analysis software 4000 Series Explorer, v 3.5.3.3 (Applied Biosystems). Samples were desalted and concentrated using reverse-phase Poros R2 (Applied Biosystems, California, EUA) and eluted directly to the MALDI target with matrix solution (α-cyano-4-hydroxycinnamic acid; CHCA; Fluka), which was prepared at a concentration of 10 µg/µL in 50% ACN with 0.1% TFA. External calibration was executed using CalMix5 (Protea). The 30 highly intense precursor ions of MS spectra were selected to analysis by MS/MS.
The monoisotopic masses of the peptides were used to search for protein identification through the use of Protein Pilot v 4.5 (ABSciex) software with the Mascot search engine (MOWSE algorithm). The Swiss Prot database, restricted to Homo sapiens, was used for all the searches. A minimum mass accuracy of 50 ppm and a mass tolerance of 0.3 Da, two missed cleavages in peptide masses, carbamidomethylation of Cys and oxidation of Met, as fixed and variable amino acid modifications, respectively, were considered. Criteria used to accept the identification were homology scores higher than 56 and at least one fragmented peptide with an individually significant score (P < 0.05), in Mascot.
Identification of SDS-PAGE cystatin-containing band by nano LC-MS/MS
Since the protein band, according to SDS-PAGE profiles, with approximately 13 kDa, differed between groups, its composition in proteins was investigated and compared among groups using nano LC-MS/MS. Due to the sample amount and technical limitations on running the same 16 pools, described above for 2-DE gels, the potential effect of gender was not considered and pools were constituted with samples of 20 individuals, with each containing saliva from 10 boys and 10 girls. As described above, each individual sample was added in a volume correspondent to the same amount of total protein. The 8 pools analysed are shown in Table 1.
Sample pools were run in SDS-PAGE gels, as described before, for individual samples. Each pool was run in duplicate. The 13 kDa protein bands were excised and proteins in-gel tryptic digested, following the protocol described above. Tryptic digests were analysed in a QExactive Orbitrap (Thermo Fisher Scientific, Bremen), coupled to a HPLC Ultimate 3000 (Dionex, Sunnyvale, CA). The pre-columns (5 mm × 300 µm I.D.) and analytical columns (150 mm × 75 µm I.D.) used were C18 Pepmap 100 columns (Dionex, LC Packings), with particle dimension of 3 µm. The peptides were concentrated to 30 µL/min in 95% solvent A (0.1% FA/5% ACN v/v) and eluted with solvent B (0.1%FA/100%ACN v/v) at 300 nL/min. The 50-minute run included: 0–3 min, 95% solvent A; 3–35 min, 5–45% solvent B; 35–38 min, 45–80% solvent B; 38–39 min, 80% solvent B; 39–40 min, 20–95% solvent A; 40–50 min, 95% solvent A. An uncoated fused silica emitter (New Objective, Cambridge, MA; o.d. 360 µm; i.d. 50 µm; tip i.d. 15 µm) was used for nanospray at 1.8 kV and mass spectra acquisition was made in data-dependent mode. A second mass spectrometry method was used with Fourier transform scanning (FT survey scan) of 375 to 1600 m/z (resolution 35,000; AGC target 3E6). The 10 highly abundant peaks were subjected to high collision dissociation [resolution 17,500; automatic gain control (AGC) of 5E4, normalised collision energy (NCE) of 25%, maximum injection time of 120 ms, exclusion dynamics of 35 s].
The spectra were processed and analysed using Proteome Discoverer (v 2.0, Thermo) software, with MS Amanda search engine (v 2.1.4.3751, University of Applied Scinces Upper Austria, Research Institute of Molecular Pathology). Uniprot (TrEMBL) and Swis-Prot (May 2016), restricted to Homo Sapiens, were used in searches. Mass accuracy of 10ppm, mass tolerance of 0.05 Da, two missed cleavages, carbamidomethylation of Cys and oxidation of Met, as fixed and variable amino acid modifications, respectively, were considered. In order to get less than 1% false positives, the Percolator algorithm (v 2.0, Thermo) was implemented as the peptides were filtered with high confidence and a minimum length of 6 amino acids. Only the proteins with a minimum of two peptides of degree 1 were considered.
Western blot
Western blotting was used for validation of MS results and for comparison of type-S and B cystatins among groups (33 boys: 17 sensitive and 16 low sensitive to bitter; 25 girls: 16 sensitive and 9 low sensitive to bitter). Samples were run in duplicate. For each sample, a volume corresponding to 3 µg total protein (for S-type cystatins) or to 10 µg total protein (for cystatin B) was run on each lane of 14% polyacrilamide SDS-PAGE gels. Proteins were transferred to a PVDF membrane and proteins blocked using the procedure described above [20]. After that, membranes were incubated with primary antibody anti-cystatins S-SA-SN (sc-73884, Santa Cruz Biotechnology; dilution 1:200) or anti-cystatin B (sc-166561, Santa Cruz Biotechnology; dilution 1:100), overnight at 4 °C and bands were detected with a secondary antibody linked to alkaline phosphatase (anti-mouse; S372B, Promega; dilution 1:10000), using a chemifluorescent substrate (ECF Plus Western Blotting Detection Reagents, GE Healthcare). Membranes were revealed in a transilluminator (Bio-Rad Gel-doc system) and a semi-quantitative analysis of band expression was carried out using the software Bio-Rad Image Lab 5.2.1.
Statistical analysis
All data were analysed using descriptive statistics and normality, and homoscedasticity were evaluated using the Kolmogorov-Smirnoff and Levene tests respectively. For data not presenting one or both of these presuppositions, non-parametric tests were used. For comparison of salivary parameters (flow rate, total protein concentration, glucose concentration, individual SDS-PAGE protein bands and western blot bands of cystatins) between groups with different taste sensitivities, Student’s t-test or its corresponding non-parametric (Mann–Whitney) were used. Since saliva pools were used for 2-DE and LC-MS/MS analysis, differences between groups were obtained by comparing the various pools from each group (BMI percentile group or taste sensitivity groups) by T-test or Mann–Whitney. Comparisons of salivary proteins identified by LC-MS/MS were made using the values of exponentially modified protein abundance index (emPAI). Statistical significance was considered for p < 0.05. All statistical analysis procedures were achieved using the SPSS 21.0 software package (SPSS, Inc., Chicago, IL).
Results
Flow rate, total protein concentration and glucose
The salivary flow rate and total protein concentration did not differ between children with different taste sensitivities (Table 2). Moreover, no differences were observed among taste sensitivity groups for salivary glucose levels (Table 2).
SDS-PAGE profiles
Twenty-five protein bands were visualised in the SDS-PAGE profiles (Fig. 1a). The protein bands G (identified as containing a mixture of carbonic anhydrase VI and zinc-α2 glycoprotein) and J (identified as containing prolactin-inducible protein) presented higher levels in normal-weight compared to overweight children [band G: 4.02 ± 0.07 vs. 3.72 ± 0.09 (P = 0.003) and band J: 4.11 ± 0.08 vs. 3.86 ± 0.11 (P = 0.031), normal weight and overweight, respectively]. By contrast, band E (identified as containing salivary α-amylase) presented higher mean values in overweight children [8.51 ± 0.13 vs. 9.23 ± 0.24 (P = 0.009), normal weight and overweight, respectively]. Details of mass spectrometry identifications of protein bands are presented in Supplementary Table 1.
SDS-PAGE saliva profiles: a—representative SDS-PAGE gel (NW—normal weight; S—sensitive to either bitter or sweet taste; LS—low-sensitive to bitter or sweet taste); b—Differences in band K % volume between bitter-sensitive and low-sensitive children; differences were in opposite directions for normal-weight or overweight boys; * P < 0.05
SDS-PAGE saliva profiles differed between children sensitive and low-sensitive to bitter taste at the level of band K (identified as containing a mixture of cystatins SN and S). However, these differences were observed only in boys (and not in girls) and were dependent on BMI percentile: in normal-weight boys, band K was present at higher levels in bitter-sensitive children compared to low-sensitive ones [11.46 ± 0.52 vs. 9.97 ± 0.49 (P = 0.022), sensitive and low-sensitive, respectively]; in overweight boys, the higher levels of band K were observed in individuals who are low-sensitive to bitter taste [9.58 ± 0.65 vs. 12.01 ± 0.61 (0.019)] (Fig. 1b).
Concerning sweet taste, no differences in SDS-PAGE profiles between sensitive and low-sensitive groups were observed, if considering only normal-weight children. However, if considering pre-obese (but not obese) children, band F (identified as containing salivary amylase) was present in higher levels in low-sensitive individuals [9.43 ± 0.73 vs. 11.46 ± 0.56 (P = 0.045), sensitive and low-sensitive, respectively].
2-DE profiles
Normal-weight and obese children were compared for their salivary 2-DE profiles (Fig. 2). Eight protein spots were present at different levels between these two BMI percentile groups: zinc-α2-glycoprotein (spot 50) and immunoglobulin λ chain (spot 64) presented higher and lower levels, respectively, in overweight children, both in girls and boys; 1 cystatin-S (spot 0) and 1 salivary α-amylase (spot 16) spots were highly expressed in overweight boys; 5 spots (two not identified, one of prolactin-inducible protein, one chain of immunoglobulin and one lipocalin 1) were observed in lower amounts in overweight children) (details about spot level and MS identifications are present in Supplementary Table 2).
By comparing children with different bitter taste sensitivity, 19 protein spots were differently expressed between sensitive and low-sensitive individuals. From these, 7 differed only in boys and 12 only in girls (details about spot amounts and MS identification are presented in Supplementary Table 3). The spots that differ between sensitivity groups correspond to diverse proteins, namely α-amylase, carbonic-anhydrase VI (CA-VI), albumin, prolactin-inducible protein (PIP), immunoglobulin k-chain C region, immunoglobulin polymeric receptor, and leucocyte elastase inhibitor (Fig. 2, left panel).
Boys low-sensitive to bitter taste presented higher levels of PIP, CA-VI, leucocyte elastase inhibitor and immunoglobulin polymeric receptor spots. Besides these, there are some spots whose differences between bitter taste sensitivity groups depend on BMI percentile: albumin spot, for which levels are higher in bitter-sensitive normal-weight boys and actin cytoplasmic 2 protein, which is lower in sensitive obese boys.
CA-VI and immunoglobulin polymeric receptor spots were increased in girls sensitive to bitter taste, whereas α-amylase, Ig K chain C region and PIP are decreased in this group, compared to bitter low-sensitive girls. The effect of BMI in the relationship between saliva composition and bitter taste sensitivity, in girls, was only observed for the protein albumin, for which some spots presented higher levels in sensitive normal-weight girls and lower levels in sensitive obese girls.
Concerning sweet taste sensitivity, 2-DE salivary profiles also presented differences among groups. Sixteen protein spots were observed to differ between sweet-sensitive and low-sensitive children, with 4 differing only in boys and 7 only in girls (Fig. 2, right panel). These spots correspond to the protein cystatins CA-VI, zinc-α2-glycoprotein, immunoglobulin k chain C region, cytoplasmic actin 1, albumin, PIP, α-amylase, lipocalin 1 and immunoglobulin polymeric receptor (detailed information about spots levels and MS identifications are presented in Supplementary Table 4).
In boys, PIP spots were highly abundant in sweet low-sensitive children and CA-VI in sensitive ones. Besides these, α-amylase, PIP, cystatin SN, albumin and lipocalin 1 were highly abundant in sweet low-sensitive boys, but only in normal-weight ones.
In girls, α-amylase spots were also observed as more abundant in those low-sensitive to sweet taste. Cystatin S spot was also highly expressed in this group, but only for the normal-weight girls. Spots of immunoglobulin k chain C region, immunoglobulin polymeric receptor, cytoplasmic actin 1 and PIP spots were highly abundant in sweet-sensitive subjects compared to the low-sensitive ones, but only in normal-weight girls.
Analysis of band K by LC-MS/MS
After in-gel digestion and analysis by LC-MS/MS, several different proteins, including different isoforms of cystatins, were identified in band K (band with approximately 13 kDa in SDS-PAGE profiles) (Supplementary Table 5). By comparing the pools analysed, it was possible to observe differences between normal-weight and obese children in six of the cystatin isoforms: cystatin A, cystatin B, cystatin C, cystatin S, cystatin SA (Fig. 3). With the exception of cystatin SN, which was present at lower levels, all these cystatins were increased in obese children compared to normal-weight ones.
Through the comparison of individual SDS-PAGE profiles, it was possible to observe band K present in different levels in sensitive and low-sensitive children, with such difference dependent on BMI percentile. Through LC-MS/MS it was possible to observe that the cystatins S and SN were the main contributors to those differences. According to emPAI values, cystatins S and SN were present in higher amounts in sensitive children when only the normal-weight ones are considered. However, in obese children, cystatin SN was 4 times increased in bitter low sensitive individuals. Concerning cystatin S, the higher levels in children who are sensitive to bitter taste were observed also in obese (ratio between sensitive and low sensitive children of 3.8) (Supplementary Table 6).
The cystatin isoforms identified in band K as related to bitter taste sensitivity, were also related to sweet taste sensitivity. The association of cystatins SN and S with sweet taste were also dependent on BMI percentile. The isoform SN was observed to be present in higher levels in the saliva pool from normal weight and in lower levels in the pool from obese children low-sensitive to sweet taste. Cystatin S was increased in sweet taste low sensitive children, but only in obese. The association of cystatins SN and S with bitter and sweet tastes was observed also considering the levels of these proteins per unit of saliva volume (i.e., normalised for total protein concentration) (Supplementary Table 6).
Western blot
The results obtained for S-type cystatins and cystatin B were confirmed by Western blot. The cystatins S, SN and SA were identified in a band with approximately 13.6 kDa, corresponding to the band K in SDS-PAGE profiles. Normal-weight and obese children did not differ in the intensity of this band, neither when considering sexes separately. Concerning taste sensitivity groups, when obese children were considered, differences in the amounts of S-type cystatins were observed between bitter-sensitive and bitter low-sensitive children. In this case, it was the obese children with low sensitivity to the bitter taste that presented this protein band with higher intensity (Fig. 4).
The intensity of cystatins S-type band did not differ among children with different sweet taste sensitivities. In the membranes incubated with anti-cystatin B antibody, the band with approximately 13.6 kDa (correspondent to band K) was stained. This protein presented a tendency for being highly expressed in obese children (Supplementary Figure 1), with no differences among bitter or sweet taste sensitivity groups, in accordance with the results obtained by LC-MS/MS.
Discussion
In recent years, some studies have presented evidence that salivary proteome can present some differences in individuals with different basic taste sensitivities. However, most of the information present to date refers to adults and does not take the individual’s BMI into account. In this study, the relationship between saliva proteome and the basic bitter and sweet tastes was assessed in children, assessing the influence that the BMI percentile may have in this relationship.
As it was previously observed in adults [18], normal-weight and overweight children differ in the levels of some salivary proteins. One of the proteins increased in overweight children was α-amylase, which has been observed to be related to obesity in previous studies [18, 21]. Some immunoglobulin chains were decreased in overweight children, which is in accordance with studies where IgA levels were decreased in the saliva of obese children (6–13 years old) [22], suggesting potential alterations in the immune system with obesity.
One of the main findings in this study is the increased levels of some isoforms of salivary cystatins in the saliva of obese, comparatively to normal weight children. In 2-DE profiles, one spot of cystatin S was more abundant in obese children, in accordance with our previous observation of higher levels of cystatin S-type protein spots in obese women, compared to normal-weight pairs [18]. Moreover, the isoforms A, B, C, S and SA were also present in higher levels in obese children. Cystatins A and B belong to family I, which corresponds to a family of intracellular proteins, whereas cystatin C, S and SA belong to family II of extracellular cystatins. Concerning cystatins A, B and C, to our knowledge, this is the first study where the levels of these proteins in saliva have been associated with BMI. The main biological function of cystatins consists in the inhibition of cysteine proteases, with different isoforms differing in inhibition properties [23]. Previous studies did show the involvement of some cysteine proteases, such as cathepsins, in obesity development, namely cathepsins K, L and S, which have a role in adipocyte differentiation, contributing to their development (reviewed in [24]). In addition, a study of rodents demonstrated that reduced levels of cathepsins resulted in diminution of weight gain [25]. As cathepsins were the target of cystatins, higher levels of blood cystatins were associated with obesity, as these were justified as a potential regulatory mechanism for obesity [26, 27]. From the observations made in this study, it is possible to hypothesise that cystatins A, B and C may also have a role in the control of cathepsins associated with obesity and that their levels in saliva may be proportional to the levels in blood. In fact, although a major proportion of cystatins in saliva are of glandular origin, mainly from the submandibular gland [28], the presence of some coming from blood should not be excluded. Concerning cystatins S and SA, they are mainly from submandibular gland origin [29]. The reason why they are increased in obese children needs to be further explored. Interestingly, the isoform SN, which is also from glandular origin [29] related with obesity in opposite direction and this may be the reason why western blotting, using an antibody able to recognize the different isoforms of S-type cystatins, resulted in no differences between normal weight and obese children. Although this needs further clarification, these salivary proteins appear to have potential for the study of childhood obesity, namely for the understanding of biological and/or pathological processes associated with this condition.
There is a limited number of studies where saliva composition was studied in the context of taste perception. One of the studies in which children salivary proteome was related to taste was performed on babies and evaluated taste acceptance, rather than taste sensitivity [15]. One of the groups of proteins observed to relate to higher bitter taste acceptance was the group of salivary cystatins. In the present work, cystatins were also related to bitter taste sensitivity, but it was observed that this relationship was dependent on the BMI percentile. Cystatin SN presented higher levels in children with low sensitivity to bitterness, but only in those who were overweight, with the opposite relationship in normal weight ones. However, cystatin S was observed in higher amounts in saliva from bitter-sensitive children, independently of BMI. This is particularly interesting, since this was the only cystatin isoform found to be associated with bitter taste sensitivity, regardless of body weight condition, and moreover having an inverse relationship to that usually described in the literature. Different studies have determined higher levels of cystatins in the saliva of individuals with a lower response to bitter taste [10, 15, 30]. However, these studies did not take into account possible differences in BMI. Curiously, Cabras and colleagues [11] did not observe differences in the levels of S-type cystatins in individuals with different PROP bitter taste responsiveness, when studying a sample composed mainly of regular-weight adults (BMI between 18.6 and 25.3 kg/m2), but a study performed in individuals with BMI between 19 and 30 kg/m2 reported higher levels of salivary cystatin SN in individuals low sensitive to caffeine bitterness [10]. As it was reported above, salivary cystatin levels differ between normal weight and overweight children, what may, on one hand, to explain this different relationship saliva-bitter taste sensitivity; on the other hand, taste function may be altered in overweight [17] and that may, in turn, affect the relationship that saliva may have with taste perception.
Salivary CA-VI, which is the salivary protein primarily described as involved in bitter taste perception in this study [31], also presented different levels in children identified as sensitive relative to those found to be low-sensitive to this basic taste. However, whereas in boys there were protein spots present in higher amounts in low-sensitive individuals, in girls the CA-VI spots that presented differences were more intense in sensitive subjects. The involvement of CA-VI in bitter taste perception is not completely understood. This protein is a trophic factor for taste bud development [32], and deficiencies in it have been associated with decreased taste sensitivity [31]. Nevertheless, some controversy exists and some studies did not find an association between CA-VI and taste perception [9, 33]. The results we obtained for boys in this study were different from those obtained previously for adult males [20]. Why this happens needs to be further elucidated. Anyway, differences between children and adults in saliva composition [34, 35] and in taste perception [36] exist and can contribute to variations in the relationship between these two parameters.
Two other proteins observed to vary between children with different bitter taste sensitivity were PIP and albumin. Whereas the first presented higher levels in low-sensitive children, albumin spots were increased in sensitive ones. Salivary PIP had been observed in higher levels in babies with higher acceptance to bitterness [15] and in adults low-sensitive to this basic taste [20]. Increases in the levels of this protein after simulation by aversive stimuli has been reported [37], suggesting that this protein in saliva may respond to aversive oral sensations. For albumin, higher expression levels in adult males highly responsive to PROP bitterness, compared to low-responsive ones, had already been mentioned [10], but the meaning for that difference needs to be further clarified.
The salivary proteome of children with different sweet taste sensitivity also presented some differences, some of which depended on children body weight, similar to what was described above for bitter taste the salivary cystatin SN was increased in normal weight children who were low-sensitive to sweet taste and decreased in obese children with this type of sensitivity; cystatin S was increased in obese children low sensitive to sweet taste. To our knowledge, this is the first report in which these proteins have been associated with sweet taste in children, with previous studies with babies reporting the absence of differences in cystatin levels according to sweet acceptance [15]. The evaluation of different parameters, such as sensitivity and acceptance, the different ages of participants (8–9 years old in ours vs. 3–6 months in the aforementioned study), and the differences in the sweet stimuli that were tested (sucrose in ours vs. lactose in the aforementioned study) may contribute to these different results. It is curious that the relationship between salivary cystatins SN and S is in the opposite direction to bitter and sweet tastes. One might hypothesize that children with high bitter taste sensitivity would have lower sweet taste sensitivity and vice-versa. However, no relationship between these two tastes sensitivities were observed (data not shown).
In this study, α-amylase spots were observed to be increased in children low-sensitive to sweet taste. α-Amylase is abundant in saliva, being responsible for the digestion of starch in the mouth. This starch digestion may have an effect on taste perception, since it results in the rise of sweet molecules, such as maltose and subsequently glucose [38]. We previously observed higher levels of this protein in adults low-sensitive to sweet taste [13], which we hypothesised to be due to some ‘desensitisation’ of taste receptors, by the frequent presence of higher levels of sweet molecules in individuals with high amylase activity.
Besides the proteins mentioned above, different immunoglobulin chains were identified for spots differently expressed among children with different taste sensitivities. Some of the differences observed here are in accordance with previous observations in adults, whereas others are opposite [13, 20]. The levels of immunoglobulins in saliva are affected by many factors, including immunologic state [39] and oral health [40]. Moreover, we did observe different levels of immunoglobulin chains between normal-weight and obese children. Despite the participants of this study being healthy, it is not possible to guarantee the total absence of any infection/inflammation that may affect immunoglobulin levels.
In conclusion, salivary proteome relates to bitter and sweet taste sensitivities, but the way in which each salivary protein is related differs between normal-weight and overweight children, with gender also having an effect. The salivary proteins carbonic-anhydrase VI, prolactin-inducible protein, cystatins and α-amylase are the main proteins involved in different taste perception. Although also related with sweetness perception, salivary cystatins are mainly associated with bitterness, with cystatin S being particularly interesting, by its increased levels in bitter-sensitive children, independent of BMI. One of the major findings of this work was the increased levels of different isoforms of cystatins in saliva from obese children, being these cystatins predicted to be not only from blood but also from glandular origin. While additional work is needed to further investigate the role of each of these salivary proteins in taste perception, these findings support the notion that deeper knowledge about saliva composition may be useful in understanding inter-individual differences in food acceptance, which may be further used to help children to adopt healthier diets.
References
Wijnhoven TM, Van Raaij JM, Spinelli A, Starc G, Hassapidou M, Spiroski I et al. WHO European Childhood Obesity Surveillance Initiative: body mass index and level of overweight among 6-9-year-old children from school year 2007/2008 to school year 2009/2010. BMC Public Health. 2014. https://doi.org/10.1186/1471-2458-14-806.
WHO. Downward trend in overweight and obesity among Portuguese school children. 2018. http://www.euro.who.int/en/health-topics/disease-prevention/nutrition/news/news/2018/2/downward-trend-in-overweight-and-obesity-among-portuguese-school-children (accessed 12 Jun2018).
Lamy E, Pinheiro C, Rodrigues L, Capela e Silva F, Lopes OS, Tavares S et al. Determinants of tannin-rich food and beverage consumption: Oral perception vs. psychosocial aspects. 2016.
Drewnowski A, Gomez-Carneros C Bitter taste, phytonutrients, and the consumer: A review. Am. J. Clin. Nutr. 2000. https://doi.org/10.1111/j.1750-3841.2011.02471.x.
Bell KI, Tepper BJ. Short-term vegetable intake by young children classified by 6-n-propylthoiuracil bitter-taste phenotype. Am. J. Clin. Nutr. 2006;245–51.
Keller KL, Adise S. Variation in the ability to taste bitter thiourea compounds: implications for food acceptance, dietary intake, and obesity risk in children. Annu Rev Nutr 2016. https://doi.org/10.1146/annurev-nutr-071715-050916.
Kildegaard H, Tønning E, Thybo AK. Preference, liking and wanting for beverages in children aged 9-14years: role of sourness perception, chemical composition and background variables. Food Qual Prefer 2011. https://doi.org/10.1016/j.foodqual.2011.03.005.
Joseph PV, Reed DR, Mennella JA. Individual differences among children in sucrose detection thresholds: relationship with age, gender, and bitter taste genotype. Nurs Res 2016. https://doi.org/10.1097/NNR.0000000000000138.
Barbarossa IT, Melis M, Mattes MZ, Calò C, Muroni P, Crnjar R, et al. The gustin (CA6) gene polymorphism, rs2274333 (A/G), is associated with fungiform papilla density, whereas PROP bitterness is mostly due to TAS2R38 in an ethnically-mixed population. Physiol Behav. 2015;138:6–12.
Dsamou M, Palicki O, Septier C, Chabanet C, Lucchi G, Ducoroy P, et al. Salivary protein profiles and sensitivity to the bitter taste of caffeine. Chem Senses. 2012;37:87–95.
Cabras T, Melis M, Castagnola M, Padiglia A, Tepper BJ, Messana I, et al. Responsiveness to 6-n-propylthiouracil (PROP) is associated with salivary levels of two specific basic proline-rich proteins in humans. PLoS ONE. 2012;7:e30962.
Rodrigues L, da Costa G, Cordeiro C, Pinheiro CC, Amado F, Lamy E. Relationship between saliva protein composition and 6-n-Propylthiouracil bitter taste responsiveness in young adults. J Sens Stud. 2017; 32. https://doi.org/10.1111/joss.12275.
Rodrigues L, Costa G, Cordeiro C, Pinheiro C, Amado F, Lamy E. Salivary proteome and glucose levels are related with sweet taste sensitivity in young adults. Food Nutr Res. 2017;61:1389208.
Stolle T, Grondinger F, Dunkel A, Meng C, Médard G, Kuster B et al. Salivary Proteome Patterns Affecting Human Salt Taste Sensitivity. J Agric Food Chem. 2017. https://doi.org/10.1021/acs.jafc.7b03862.
Morzel M, Chabanet C, Schwartz C, Lucchi G, Ducoroy P, Nicklaus S. Salivary protein profiles are linked to bitter taste acceptance in infants. Eur J Pediatr. 2014;173:575–82.
Rodrigues L, Espanca R, Costa AR, Antunes CM, Pomar C, Capela-Silva F et al. Association between salivary leptin levels and taste perception in children. J Nutr Metab 2017; 2017. https://doi.org/10.1155/2017/7260169.
Tepper BJ, Melis M, Koelliker Y, Gasparini P, Ahijevych KL, Barbarossa IT. Factors influencing the phenotypic characterization of the oral marker, PROP. Nutrients. 2017. https://doi.org/10.3390/nu9121275.
Lamy E, Simões C, Rodrigues L, Costa AR, Vitorino R, Amado F, et al. Changes in the salivary protein profile of morbidly obese women either previously subjected to bariatric surgery or not. J Physiol Biochem. 2015;71:691–702.
LAEMMLI UK. Cleavage of structural proteins during the assembly of the head of bacteriophage T4. Nature. 1970;227:680–5.
Rodrigues L, da Costa G, Cordeiro C, Pinheiro CC, Amado F, Lamy E. Relationship between saliva protein composition and 6- n -Propylthiouracil bitter taste responsiveness in young adults. J Sens Stud 2017: e12275.
Rodrigues L, Mouta R, Costa AR, Pereira A, Capela e Silva F, Amado F, et al. Effects of high fat diet on salivary α-amylase, serum parameters and food consumption in rats. Arch Oral Biol. 2015;60:854–62.
Pallaro A, Barbeito S, Taberner P, Marino P, Franchello A, Strasnoy I, et al. Total salivary IgA, serum C3c and IgA in obese school children. J Nutr Biochem. 2002;13:539–42.
Baron AC, DeCarlo AA, Featherstone JDB. Functional aspects of the human salivary cystatins in the oral environment. Oral Dis 1999. https://doi.org/10.1111/j.1601-0825.1999.tb00307.x.
Lafarge JC, Naour N, Clément K, Guerre-Millo M. Cathepsins and cystatin C in atherosclerosis and obesity. Biochimie. 2010. https://doi.org/10.1016/j.biochi.2010.04.011.
Yang M, Sun J, Zhang T, Liu J, Zhang J, Shi MA et al. Deficiency and inhibition of cathepsin K reduce body weight gain and increase glucose metabolism in mice. Arterioscler Thromb Vasc Biol 2008. https://doi.org/10.1161/ATVBAHA.108.172320.
Wasen E, Isoaho R, Mattila K, Vahlberg T, Kivela S-L, Irjala K. Serum cystatin C in the aged: relationships with health status. Am J Kidney Dis. 2003.
Luc G, Bard JM, Lesueur C, Arveiler D, Evans A, Amouyel P et al. Plasma cystatin-C and development of coronary heart disease: The PRIME Study. Atherosclerosis. 2006;375–80. https://doi.org/10.1016/j.atherosclerosis.2005.06.017.
Veerman EC, van den Keybus PA, Vissink A, Nieuw Amerongen AV. Human glandular salivas: their separate collection and analysis. Eur J Oral Sci. 1996;104:346–52.
Dickinson DP. Salivary (SD-type) cystatins: over one billion years in the making—but to what purpose? Crit. Rev. Oral Biol. Med. 2002. https://doi.org/10.1177/154411130201300606.
Morzel M, Neyraud E, Brignot H, Ducoroy P, Jeannin A, Lucchi G et al. Multi-omics profiling reveals that eating difficulties developed consecutively to artificial nutrition in the neonatal period are associated to specific saliva composition. J Proteomics. 2015. https://doi.org/10.1016/j.jprot.2015.07.028.
Shatzman AR, Henkin RI. Gustin concentration changes relative to salivary zinc and taste in humans. Proc Natl Acad Sci USA. 1981;78:3867–71.
Henkin RI, Martin BM, Agarwal RP. Efficacy of exogenous oral zinc in treatment of patients with carbonic anhydrase VI deficiency. Am J Med Sci. 1999. https://doi.org/10.1097/00000441-199912000-00006.
Feeney EL, Hayes JE. Exploring associations between taste perception, oral anatomy and polymorphisms in the carbonic anhydrase (gustin) gene CA6. Physiol Behav. 2014;128:148–54.
Sivakumar T, Hand AR, Mednieks M. Secretory proteins in the saliva of children. J Oral Sci. 2009. https://doi.org/10.2334/josnusd.51.573.
Yang ZM, Chen LH, Zhang M, Lin J, Zhang J, Chen WW et al. Age differences of salivary alpha-amylase levels of basal and acute responses to citric acid stimulation between chinese children and adults. Front Physiol. 2015. https://doi.org/10.3389/fphys.2015.00340.
James CE, Laing DG, Oram N. A comparison of the ability of 8-9-Year-old children and adults to detect taste stimuli. Physiol Behav. 1997. https://doi.org/10.1016/S0031-9384(97)00030-9.
Lorenz K, Bader M, Klaus A, Weiss W, Görg A, Hofmann T. Orosensory stimulation effects on human saliva proteome. J Agric Food Chem. 2011. https://doi.org/10.1021/jf2024352.
Santos JL, Saus E, Smalley SV, Cataldo LR, Alberti G, Parada J, et al. Copy number polymorphism of the salivary amylase gene: implications in human nutrition research. J Nutr Nutr. 2012;5:117–31.
Lima DP, Diniz DG, Moimaz SAS, Sumida DH, Okamoto AC. Saliva: reflection of the body. Int J Infect Dis. 2010;14:e184–e188.
Butchibabu K, Kiran K, Koppolu P, Kumar S, Muralikrishna T, Swaminathan M. Estimation of salivary immunoglobulin A levels in gingivitis and chronic periodontitis patients before and after phase I periodontal therapy. J Dr NTR Univ Heal Sci. 2014;3:23.
Acknowledgements
This paper was supported by national funding from the Portuguese Science Foundation (FCT) under the project UID/AGR/00115/2013 (ICAAM – University of Évora). The authors also acknowledge financial support from FCT in the form of Elsa Lamy’s FCT investigator contract IF/01778/2013. FCT played no role in the development of this work or in its submission for publication.
Author information
Authors and Affiliations
Corresponding authors
Ethics declarations
Conflict of interest
The authors declare that they have no conflict of interest.
Additional information
Publisher’s note: Springer Nature remains neutral with regard to jurisdictional claims in published maps and institutional affiliations.
Rights and permissions
About this article
Cite this article
Rodrigues, L., Espanca, R., Costa, A.R. et al. Comparison of salivary proteome of children with different sensitivities for bitter and sweet tastes: association with body mass index. Int J Obes 43, 701–712 (2019). https://doi.org/10.1038/s41366-018-0289-5
Received:
Revised:
Accepted:
Published:
Issue Date:
DOI: https://doi.org/10.1038/s41366-018-0289-5
- Springer Nature Limited