Abstract
Based on recent studies in adult subjects, saliva composition is increasingly considered as a physiological factor contributing to taste sensitivity or acceptance. In order to evaluate a possible link between salivary protein composition and taste acceptance in infants, 73 infants participated longitudinally in taste acceptance tests and donated saliva at the age at 3 and 6 months. Intake ratios, reflecting acceptance of a taste solution relative to water were calculated for the five basic tastes. Salivary proteins were separated by one-dimensional electrophoresis and bands were semi-quantified by image analysis. Partial least square (PLS) regression analyses were performed for each taste at both ages to explain intake ratios by band intensities. Bitterness acceptance in the younger infants was unique in the sense that salivary protein profiles could partly predict bitter taste acceptance. At that age, infants were on average indifferent to the 0.18-M urea solution, but great variability in acceptance was observed. The six bands considered as the best predictors for bitterness acceptance were identified by MALDI-TOF mass spectrometry. Higher abundance of bands containing secretory component, zinc-α-2-glycoprotein and carbonic anhydrase 6 was associated to a lower bitterness acceptance, while higher abundance of bands containing lactoperoxidase, prolactin-inducible protein and S-type cystatins was associated to a higher bitterness acceptance. In a second stage, S-type cystatin abundance was measured by Western blotting in order to tentatively confirm this particular finding in an independent group of 22 infants. Although not reaching statistical significance, probably due to a relatively small sample size, it was again observed that cystatin abundance was higher in infants accepting more readily the bitter solution over water. Conclusion: saliva protein composition may contribute to bitter taste acceptance in the younger infants.
Similar content being viewed by others
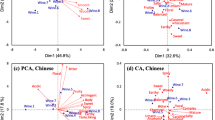
Avoid common mistakes on your manuscript.
Introduction
Overweight and obesity are increasing at an alarming rate worldwide with tremendous negative consequences on quality of life. The increase is of special concern for children, because overweight children are likely to remain so in later life stages, among other reasons [6]. In this context, promoting healthy dietary habits in childhood is recommended by many health national schemes. Food preferences and choices result from many interacting factors: sensory perception during eating, emotional context of food intake, cultural habits, etc. The relative weight of these factors most probably depends on the subject and on the food ingested. Nevertheless, many studies have focused on taste perception and preferences in children because of their direct impact on diets. To quote only one example, preference for sourness was positively correlated to fruit consumption in 8–11-year-old boys [19]. In this context, it is of particular interest to understand the formation of taste acceptance in children, reported to be partly established during infancy [1]. Studies on taste acceptance are closely linked to those on taste perception and sensitivity. In order to explain the biological sources underlying variability in taste sensitivity in humans, research has so far focused mainly on taste receptors. For example, variation in the sensitivity to the bitter compound 6-n-propylthiouracil (PROP) is explained in a large proportion by polymorphism affecting the bitter taste receptor hTAS2R38 [16]. However, recent studies have evidenced that PROP tasting phenotype is a complex genetic trait involving also genes encoding for salivary proteins, namely carbonic anhydrase 6 or CA6 [26] and possibly of specific basic proline-rich proteins [2]. More generally, the link between saliva composition and taste sensitivity or acceptance is increasingly reported. Thus, in addition to early works, linking taste dysfunction to reduced levels in CA6 [12, 34], saliva composition was shown to be associated to fat perception and liking [25] or to sensitivity to the bitter taste of caffeine or PROP [9, 20].
Infancy is a time of rapid developmental changes, and this applies both to saliva composition and taste acceptance. Thus, substantial developmental changes occur in the protein [4, 24, 29] or peptide [23] saliva composition in newborns or infants. In addition, taste acceptance also evolves between 3 and 6 months of age, as illustrated in particular by the increasing contrast between acceptance of the different tastes [33]. At 6 months, variability in the acceptance of sour, sweet and umami tastes predicts the variability in acceptance of some food groups that taste sour, sweet or umami [31]. In this context, as a contribution to understand early formation of taste acceptance, the objective of the present study was to test the hypothesis whereby taste acceptance may be associated to saliva protein composition in 3- and 6-month-old infants.
Materials and methods
Participants and overall design
Participants were recruited in the frame of OPALINE (observatory of food preferences in infants and children), a prospective longitudinal study on the development of food preferences in the first 2 years of life. We here report on results concerning 95 healthy infants who participated in taste acceptance tests and donated saliva at the ages of 3 and 6 months. Four infants were born prematurely, at 32, 32, 35 and 36 weeks of gestation, respectively. At the age of 3 months, 32 infants were breast fed, 45 were formula fed, 18 received breast milk and formula. Nine infants had started receiving solid foods. At the age of 6 months, 12 infants were breast fed, 72 were formula fed, and 9 received breast milk and formula (two missing values). Seventy-seven infants had started receiving solid foods.
A first group of 73 infants (“discovery group”) was used to explore without a priori hypotheses of a possible association between saliva protein composition and taste acceptance. A second group of 22 infants (“validation group”) was used to tentatively confirm one hypothesis of particular interest by a targeted immunological technique. Saliva sampling was performed at the end of the test sessions. The present study was conducted according to the guidelines laid down in the Declaration of Helsinki. The general OPALINE procedure, the taste acceptance and saliva sampling procedures were approved by the local ethical committee (Comité de Protection des Personnes Est I Bourgogne). Written and informed consent was obtained from the infants' parents.
Taste acceptance test, intake ratio definition and analysis
The taste acceptance four-bottle tests were performed as described in full details elsewhere [33]. Briefly, this procedure involves individual participation of infants in two sessions at both ages in a room designed for infant testing. For each taste, four bottles (water–tastant–tastant–water) were offered to the infant by an experimenter. Bottles were presented for 45 s with a pause of 15 s between them. A pause of at least 1 min was allowed between each group of four bottles. The five basic tastes (sweet, salty, sour, bitter, umami) were tested, with a solution of lactose at 0.2 M; NaCl, 0.085 M; citric acid, 0.006 M; urea, 0.18 M; and monosodium glutamate, 0.009 M, respectively. Such concentrations are considered as supra-threshold. Each bottle contained 30 ml of the taste solution except for urea where only 15 ml were presented. At each age, the five groups of bottles corresponding to the five basic tastes were presented in a double-blind balanced order over the two sessions. Ingestion was determined by weighing bottles before and after consumption to the nearest 0.1 g. A variable reflecting proportional acceptance of a taste solution relative to water (intake ratio) was calculated based on data collected. For each tastant, the intake ratio (IR) was defined as the ingested volume of this tastant relatively to the sum of ingested volume of this tastant and of water (i.e. IR for salty taste = total ingested volume of salt solution/(total ingested volume of salt solution + total ingested volume of water)). For each taste and each age, results are expressed as mean ± SD, and Student's t tests were used to assess whether the IR was different from 0.5 (i.e. whether there was a significant rejection or preference of the tastant over water).
Saliva sampling
Whole saliva collection using Salivette devices (Sarstedt, Nümbrecht, Germany). Immediately after collection, the Salivettes were placed on ice for transport, placed at −30 °C within less than 10 min and stored for a maximum of 5 days before preparation of protein concentrates. Concentration of saliva proteins were performed as previously described [24]. Concentrates were frozen at −80 °C until analysed by sodium dodecyl sulfate poly–acrylamide gel electrophoresis (SDS–PAGE).
SDS–PAGE and image analysis
SDS–PAGE was performed in a PROTEAN II Multi Cell (Bio-Rad, Richmond, USA) according to the method of Laemmli [17] by using 12 % polyacrylamide gels, 1 mm thick with 20 lanes. Protein concentration was measured in the concentrates following Bradford's method. Protein samples were denatured in a Laemmli buffer containing 100 mM DTT, and the protein load was adjusted to 20 μg per lane. The quantity of saliva collected allowed one electrophoretic separation per sample. Electrophoresis was carried out at 15 mA per gel, at 40 V during 30 min and 300 V for 8 h. Gels were subsequently stained using the “blue silver” protocol [3]. Densitometric scans of SDS–PAGE gels were performed using a flatbed scanner (GS-800, Bio-Rad). Molecular weights were determined according to the molecular weight standards run with protein samples. Intensities of the protein bands were analysed by the software Quantity One (Bio-Rad). Peak area values of bands were normalised by calculating the ratio of each band's quantity to the total quantity of all matched bands in a lane. Data were expressed in percentage and finally log-transformed. A total of 21 bands spanning the 10–66-kDa molecular weight range and present in at least 50 % of infants were retained for further statistical analysis. The molecular weight region ~20–29 kDa was highly variable between subjects, and no band was therefore retained in this region.
Identification of bands of interest by MALDI-TOF/TOF mass spectrometry
Protein bands were manually excised from gels and cut into 1 mm3 gel pieces. Gel pieces were prepared and tryptic digestion was performed as previously described [24]. The resulting peptides were concentrated by evaporation and 0.5 μL of the concentrate was loaded onto a Ground Steel target, mixed with 1 μL of matrix solution (3.5 mg/ml CHCA in acetonitrile 50 %, TFA 0.25 %). The target was introduced in a mass spectrometer matrix-assisted laser desorption ionization tandem time-of-flight (MALDI-TOF/TOF) (UltrafleXtreme, Bruker Daltonics, Bremen, Germany). Ionisation was performed in mass spectrometry (MS) and tandem mass spectrometry (MS/MS) by irradiation of a nitrogen laser (337 nm) operating at 1,000 Hz. Data were obtained using a maximum accelerating potential of 25 kV in the positive and reflectron modes. Mass calibration was done using the peptide calibration standards from Bruker Daltonics.
Identification of proteins was performed within SwissProt, restricting the taxonomy to Homo sapiens. Methionine oxidation and carbamidomethyl modification of cysteine were accepted as variable and global modifications, respectively. The mass deviation tolerance was 30 ppm in MS and 0.4 Da in MS/MS.
Semi-quantification of S-type cystatins by Western blotting
Western blotting was used in order to verify one hypothesis of special interest evidenced previously by one-dimensional electrophoresis, namely over-expression of S-type cystatins in infants accepting most readily the bitter solution. SDS–PAGE was performed on 14 % acrylamide gels using the Mini-Protean-2 device (Bio-Rad) according to the method of Laemmli [17]. The protein load was adjusted to 10 μg of protein per lane, and electrophoresis was carried out at 15 mA per gel for 90 min. Proteins were subsequently transferred onto 0.45 μm of nitrocellulose membranes at 10 V for 20 min using a Transblot SD cell (Bio-Rad). Membranes were saturated in 8 % skimmed milk powder in 9 ‰ NaCl and incubated for 1 h with a 1:200 dilution of the primary antibody (anti-cystatin S-SA-SN: sc-73884, Santa Cruz Biotechnology, Inc.) in 9 ‰ NaCl/5 % bovine serum albumin (BSA). After washing in PBS–Tween 0.05 %, membranes were blocked in 8 % skimmed milk powder in 9 ‰ NaCl and incubated for 1 h with a 1:2,000 dilution of secondary Alexa 532 goat anti-mouse antibody in 9 ‰ NaCl/5 % BSA. Membranes were finally washed in PBS–Tween. Images of blots were acquired on a Pharos FX imaging system (Bio-Rad). Semi-quantification of immunodetected bands was performed using the Quantity One software (Bio-Rad). Results were expressed in arbitrary unit.
Statistics
To reveal a possible relationship between band intensities and intake ratios in the discovery group, partial least square (PLS) regression analyses were performed to explain intake ratios (Y matrix) by band intensities (X matrix). PLS analyses were performed independently for each taste and at both ages, after standardisation of each X and Y variable so as to obtain standard deviations of 1. This first series of analyses indicated that bitterness acceptance at 3 months of age may be linked to electrophoretic band intensity (data not shown). Therefore, in order to increase the contrast in intake ratios and with the objective to unveil their correlation with salivary protein profiles, an additional PLS analysis was performed on a subsample of 40 infants selected on the basis of their intake ratios, i.e. selecting 20 infants with the highest (higher acceptance of bitterness) and the lowest (lower acceptance of bitterness) intake ratios, respectively. Model selection, i.e. choice of the number of components, relied on leave-one-out cross-validation, and the relative importance of each band were evaluated through the absolute value of related regression coefficient.
In a second stage, to test in the validation group the hypothesis that S-type cystatins were more abundant in infants with higher intake ratios, Western Blot semi-quantitative data were submitted to one-tailed Student's t test.
Results
Intake ratios at the age of 3 and 6 months
At the age of 3 months (Table 1), infants of the discovery group preferred significantly the sweet taste over water on average, while they were indifferent to salty, sour, bitter and umami tastes compared to water. At the age of 6 months (Table 1), infants significantly preferred sweet and salty tastes over water on average. They were indifferent to sour, bitter and umami tastes compared to water.
Relationship between band intensity and intake ratios
Figure 1 shows two typical electrophoretic profiles of infant saliva with the position of the 21 bands semi-quantified and retained for the statistical analysis. This illustrates that although band intensities were variable, the profile related to these 21 bands was qualitatively stable between subjects. The cross-validated PLS analyses revealed that the SDS–PAGE profiles were not predictive of intake ratios in 6-month-old infants for any of the five tastes, nor were they in 3-month-old infants for sweet, sour, salty and umami tastes. However, the salivary protein profiles were statistically related to the intake ratio of the bitter solution. The PLS regression showed that the first two components explained 53 % of the variance (Fig. 2), and the cross-validation confirmed that 20 % of the variance was explained. Figure 2a shows the projection of predictors, i.e. band intensities, on the first two PLS components. Six bands were found to be the most important predictors in the model, with regression coefficients above 0.15 in absolute value. These bands (1, 7, 2, 15, 9 and 13 in decreasing order of importance) are indicated in red in Fig. 2a. Figure 2b shows the projection of infants (score plot). For illustrative purposes, infants with intake ratios above 0.6 (i.e. those preferring the bitter solution over water, n = 5) were highlighted in black, while infants with intake ratios below 0.4 (i.e. those rejecting the bitter solution over water, n = 11) were highlighted in red. This evidences that infants most readily accepting bitterness were rather clustered, while those rejecting most bitterness constituted a more heterogeneous group. Comparing panels a and b of Fig. 2, it is proposed that acceptance of the bitter solution was related to higher abundance of bands 2, 15 and 13 together with lower abundance of bands 1, 7 and 9. Oppositely, infants most clearly rejecting the bitter solution compared to water had lower abundance of bands 2, 13 and 15 together with either high abundance of bands 1 and 9, or high abundance of band 7.
PLS analysis aiming at explaining acceptance of a bitter solution (intake ratio) by salivary proteins (intensities of electrophoretic bands) in 3-month-old infants (n = 40). a Projection of the 21 predictors (band intensities) on the first two PLS components explaining, respectively, 43 and 10 % of variance. The six predictors with the highest regression coefficients in the two-dimensional model are indicated in red. b Projection of individuals (scores plot) on the first two PLS components, where infants with intake ratios above 0.6 are indicated in black and infants with intake ratios below 0.4 are indicated in red
Identification of the six best predictors
Mass spectrometry analyses (Table 2) provided identifications for the six bands considered as the best predictors. One to three proteins were identified in each band. Apparent MW were in reasonable agreement with the theoretical MW of the identified protein, at the exception of lactoperoxidase in band 2 and α-amylase 1 in band 7 for which the apparent MW were 13 (19 %) and 18 kDa (32 %) lower than that of the entire protein. However, these bands contained probably intact proteins since peptides detected on the corresponding MALDI-TOF spectra were spread throughout the entire sequence (data not shown). Discrepancies in molecular weights may therefore be due to co-migration with other proteins.
Abundance of S-type cystatins in an independent group of 3-month-old infants
S-type cystatins were detected as a single band of approximately 14.5 kDa, in accordance with the theoretical molecular weights of cystatins S, SA and SN (Fig. 3). The over-expression of S-type cystatins in infants with intake ratios for the bitter solution ≥0.5 (n = 9) was not significant (p = 0.17), but median values tended to be higher in IR+ subjects than in IR− subjects (0.66 vs 0.60).
Discussion
The present study aimed at investigating whether taste acceptance was linked to salivary protein profiles in infants. Because of the relatively high number of samples in the discovery group, the method of choice for profiling was one-dimensional SDS–PAGE. This method allows separation of a few protein bands (typically 20 to 40) according to their molecular weight. SDS–PAGE has usefully been applied to saliva samples in order to describe specific profiles of burning mouth syndrome patients [5], to identify potential markers of caries development [28] or to describe developmental changes in infants [24, 29]. In the present study, it evidenced that acceptance of bitterness was linked to the relative abundance of specific bands in 3-month-old infants.
The bitter urea solution was on average consumed as much as water, at 3 and 6 months of age. We previously reported on this similar neutral ingestion behaviour while consuming 0.18 M urea vs water, although it was accompanied by negative facial expressions which led the experimenter who gave the bottles to judge that the infants disliked the urea solutions [33]. In terms of consumption, newborns are seemingly indifferent to the bitter taste of urea since they do not modify their ingestion behaviour when tasting a solution of urea (0.24 and 0.48 M) in comparison to water [7]. Older infants aged between 2 weeks and 6 months tend to consume less of a urea solution (0.12, 0.18 and 0.24 M) than of the diluent, with intake ratios of approximately 0.45 [15]. Focusing on the age of 3 months, which is when the link between salivary profiles and bitterness acceptance was statistically validated, infants here were, on average, indifferent to 0.18 M urea, and a great variability in acceptance was observed.
At this age, the majority of infants (64 out of 73) were still on a milk-only diet. Little is known on the impact of milk-borne sensory stimuli on taste acceptance. In 6-month-old infants, a positive correlation was established between acceptance of umami taste and duration of exclusive breastfeeding, but the type of milk did not impact on bitterness acceptance [32]. Infants consuming hydrolysed casein formulas displayed fewer negative expressions of distaste while eating bitter cereals compared to infants breastfed or consuming milk formulas [22]. One should note, however, that no infant in the present study consumed hydrolysed casein formula. Therefore, it is proposed that in the discovery group of 3-month-old infants considered in the present study, the relative weight of food-related experiential factors in bitter taste appreciation is probably moderate. As a consequence, it is likely that variability in taste acceptance depends, in a large part, on variability in taste sensitivity, i.e. infants tasting the bitter taste less acutely would accept it more readily. In other words, our central hypothesis is that salivary protein profiles contribute to bitterness acceptance in 3-month-old infants through modulation of their taste sensitivity. The fact that the statistical link between salivary protein profile and bitterness acceptance is not observed at the age of 6 months suggests that saliva-mediated mechanisms become proportionally less important, presumably in relation with the increased weight of food-related experiential factors in taste acceptance. Experiential factors have been, for example, suggested by Mennella et al. [21] who reported that a formula of hydrolysed proteins, considered bitter by adult tasters, was less accepted at 7 than at 2 months of age.
Focusing on the protein profiles, the present study suggests that higher abundance of bands containing secretory component, zinc-α-2-glycoprotein and CA6 is associated to a lower bitterness acceptance, while higher abundance of bands containing lactoperoxidase, S-type cystatins and prolactin-inducible protein is associated to a higher bitterness acceptance. Results are inconclusive for α-amylase, since bands with two variants were negatively and positively correlated to intake ratios, respectively. In addition, the two most abundant bands containing α-amylase, bands 4 and 5, as described in Morzel et al. [24], were not listed among the best predictors of taste acceptance.
Because most salivary proteins are multifunctional and, in turn, many proteins share generic roles such as mucosal defence or protection against microorganisms, it is quite difficult to functionally explain the protein patterns associated to bitterness acceptance. For example, both secretory component [27] and prolactin-inducible protein [30] play a role in mucosal innate immunity although the bands containing these proteins show respectively a negative and positive correlation with bitterness acceptance. Lactoperoxidase contributes to defence against infections and catabolises H2O2 [10], while zinc-α-2-glycoprotein is a protein with many functions [11], not all of them necessarily relevant in saliva, including, for example, acting as a carrier protein, playing a role in immunomodulation, regulating melanin production or initiating lipid mobilisation. The two remaining proteins, in contrast, are of special interest and reinforce the hypothesis that acceptance is modulated by taste sensitivity.
First, abundance of band 9 containing CA6 is negatively correlated with bitter taste acceptance. CA6 is a well-known key salivary protein when it comes to taste perception. Early works from the 1980s had already suggested that a salivary zinc-binding protein was involved in taste function, since subjects deficient in this protein have a reduced taste acuity [34]. This protein initially termed gustin was later identified as CA6 [35]. It is described as a trophic factor promoting growth and development of taste buds [13] and protecting taste buds against apoptosis [18]. More recently, CA6 was also associated to perception of the bitter compound PROP, but it was not the level of protein that was different between groups of different PROP statuses, but rather the functionality of the protein dependent on genetic polymorphism [26]. Here, infants with lower levels of band 9 (containing CA6) would therefore be less sensitive to the bitter taste of urea, which facilitates its acceptance. In theory, if CA6 is involved in taste bud development and maintenance of function, reduced levels should not affect specifically one taste modality. However, our results are in accordance with Henkin et al. [12] who reported that, within a group of 18 patients with reduced levels of CA6 and who self-declared loss of taste or smell, sensitivity to the bitter taste of urea was more affected (ten patients with detection thresholds outside of normal range) than sensitivity to other tastes (two to five patients with abnormal detection thresholds for salty, sweet or sour taste).
The second result supporting that bitterness acceptance depends on sensitivity is the positive correlation between band 15 containing S-like cystatins and intake ratios. S-like cystatins is the generic name given to three salivary cystatins, cystatin S, SA and SN. S-like cystatins are capable of inhibiting cysteine proteases such as the endogenous cathepsins H and L or exogenous papain and papain-like proteases [8]. As such, S-like cystatins participate in the control of proteolysis within the oral cavity. It was recently observed by an untargeted proteomic approach that adults hypersensitive to the bitter taste of caffeine had lower level of cystatin SN, a result that was further confirmed by Western blotting [9]. Although enlarged to S-like cystatins, the present observation in infants suggests the same trend since those rejecting urea (i.e. most likely most sensitive to its bitter taste) had lower level of band 15. The specific measurement of S-type cystatins in saliva of 3-month-old infants in the validation group also indicated that infants with lower acceptance of the bitter solution had lower median values for these proteins, although the difference in abundance between IR+ and IR− infants did not reach statistical significance possibly due to a small sample size. It would therefore be interesting to test this finding in a larger population. In Dsamou et al. [9], the hypothesis is that lower level of cystatin and the consequent enhanced proteolysis would affect the mucosal pellicle lining the oral cavity, thereby increasing accessibility of tastants to taste receptors. Again, this hypothesis seems rather generic and should not impact only on bitterness acceptance. Further investigation is needed to determine whether S-like cystatins have other more specific activities towards bitter taste receptors.
Quite interestingly, in a small-scale study comparing salivary proteome of patients suffering from taste disorders vs healthy subjects [14], five proteins were found to be under-expressed in patients: CA6, prolactin-inducible protein, zinc-α-2-glycoprotein and two S-like cystatins (S and SN). In the present study, in non-pathological conditions, these proteins also come out as influent in the process of taste perception, although it is rather the balance between them that seem to be determinant.
To conclude, the present study was conducted without any a priori hypothesis on the link between saliva protein composition and taste acceptance. The fact that salivary protein profiles were not independent from bitterness acceptance in 3-month-old infants is the major finding of this study. This raises several interesting research perspectives from a physiological point of view first, such as studying on the factors regulating saliva protein composition in infants, or from a behavioural point of view second, such as investigating early determining factors of bitter taste acceptance.
References
Beauchamp GK, Moran M (1982) Dietary experience and sweet taste preference in human infants. Appetite 3:139–152
Cabras T, Melis M, Castagnola M, Padiglia A, Tepper BJ, Messana I, Tomassini Barbarossa I (2012) Responsiveness to 6-n-propylthiouracil (PROP) is associated with salivary levels of two specific basic proline-rich proteins in humans. PLoS ONE 7:e30962
Candiano G, Bruschi M, Musante L, Santucci L, Ghiggeri GM, Carnemolla B, Orecchia P, Zardi L, Righetti PG (2004) Blue silver: a very sensitive colloidal Coomassie G-250 staining for proteome analysis. Electrophoresis 25:1327–1333
Castagnola M, Inzitari R, Fanali C, Iavarone F, Vitali A, Desiderio C, Vento G, Tirone C, Romagnoli C, Cabras T, Manconi B, Sanna MT, Boi R, Pisano E, Olianas A, Pellegrini M, Nemolato S, Heizmann CW, Faa G, Messana I (2011) The surprising composition of the salivary proteome of preterm human newborn. Mol Cell Proteomics 10
de Moura SAB, de Sousa JMA, Lima DF, Negreiros AND, Silva FD, da Costa LJ (2007) Burning mouth syndrome (BMS): sialometric and sialochemical analysis and salivary protein profile. Gerodontology 24:173–176
Deckelbaum RJ, Williams CL (2001) Childhood obesity: the health issue. Obes Res 9:239S–243S
Desor JA, Maller O, Andrews K (1975) Ingestive responses of human newborns to salty, sour, and bitter stimuli. Journal of Comparative and Physiological Psychology 89:966–970
Dickinson DP (2002) Salivary (SD-type) cystatins: over one billion years in the making-but to what purpose? Critical Reviews in Oral Biology & Medicine 13:485–508
Dsamou M, Palicki O, Septier C, Chabanet C, Lucchi G, Ducoroy P, Chagnon MC, Morzel M (2012) Salivary protein profiles and sensitivity to the bitter taste of caffeine. Chem Senses 37:87–95
Gothefors L, Marklund S (1975) Lactoperoxidase activity in human milk and in saliva of newborn infants. Infect Immun 11:1210–1215
Hassan MI, Waheed A, Yadav S, Singh TP, Ahmad F (2008) Zinc alpha 2-glycoprotein: a multidisciplinary protein. Mol Cancer Res 6:892–906
Henkin RI, Martin BM, Agarwal RP (1999) Decreased parotid saliva gustin/carbonic anhydrase VI secretion: an enzyme disorder manifested by gustatory and olfactory dysfunction. Am J Med Sci 318:380–391
Henkin RI, Martin BM, Agarwal RP (1999) Efficacy of exogenous oral zinc in treatment of patients with carbonic anhydrase VI deficiency. Am J Med Sci 318:392–405
Igarashi A, Ito K, Funayama S, Hitomi Y, Ikui A, Ikeda M, Nomura S (2008) The salivary protein profiles in the patients with taste disorders: the comparison of salivary protein profiles by two-dimensional gel electrophoresis between the patients with taste disorders and healthy subjects. Clin Chim Acta 388:204–206
Kajiura H, Cowart BJ, Beauchamp GK (1992) Early developmental change in bitter taste responses in human infants. Dev Psychobiol 25:375–386
Kim UK, Jorgenson E, Coon H, Leppert M, Risch N, Drayna D (2003) Positional cloning of the human quantitative trait locus underlying taste sensitivity to phenylthiocarbamide. Science 299:1221–1225
Laemmli UK (1970) Cleavage of structural proteins during the assembly of the head of bacteriophage T4. Nature 227:680–685
Leinonen J, Parkkila S, Kaunisto K, Koivunen P, Rajaniemi H (2001) Secretion of carbonic anhydrase isoenzyme VI (CA VI) from human and rat lingual serous von Ebner's glands. Journal of Histochemistry & Cytochemistry 49:657–662
Liem DG, Bogers RP, Dagnelie PC, de Graaf C (2006) Fruit consumption of boys (8–11 years) is related to preferences for sour taste. Appetite 46:93–96
Melis M, Aragoni MC, Arca M, Cabras T, Caltagirone C, Castagnola M, Crnjar R, Messana I, Tepper BJ, Barbarossa IT (2013) Marked increase in PROP taste responsiveness following oral supplementation with selected salivary proteins or their related free amino acids. PLoS ONE 8
Mennella JA, Beauchamp GK (1996) Developmental changes in the acceptance of protein hydrolysate formula. J Dev Behav Pediatr 17:386–391
Mennella JA, Forestell CA, Morgan LK, Beauchamp GK (2009) Early milk feeding influences taste acceptance and liking during infancy. Am J Clin Nutr 90:780S–788S
Morzel M, Jeannin A, Lucchi G, Truntzer C, Pecqueur D, Nicklaus S, Chambon C, Ducoroy P (2012) Human infant saliva peptidome is modified with age and diet transition. J Proteome 75:3665–3673
Morzel M, Palicki O, Chabanet C, Lucchi G, Ducoroy P, Chambon C, Nicklaus S (2011) Saliva electrophoretic protein profiles in infants: changes with age and impact of teeth eruption and diet transition. Arch Oral Biol 56:634–642
Neyraud E, Palicki O, Schwartz C, Nicklaus S, Feron G (2012) Variability of human saliva composition: possible relationships with fat perception and liking. Arch Oral Biol 57:556–566
Padiglia A, Zonza A, Atzori E, Chillotti C, Calo C, Tepper BJ, Barbarossa IT (2010) Sensitivity to 6-n-propylthiouracil is associated with gustin (carbonic anhydrase VI) gene polymorphism, salivary zinc, and body mass index in humans. Am J Clin Nutr 92:539–545
Phalipon A, Corthesy B (2003) Novel functions of the polymeric Ig receptor: well beyond transport of immunoglobulins. Trends Immunol 24:55–58
Rudney JD, Staikov RK, Johnson JD (2009) Potential biomarkers of human salivary function: a modified proteomic approach. Arch Oral Biol 54:91–100
Ruhl S, Rayment SA, Schmalz G, Hiller KA, Troxler RF (2005) Proteins in whole saliva during the first year of infancy. J Dent Res 84:29–34
Schenkels L, WalgreenWeterings E, Oomen L, Bolscher JGM, Veerman ECI, Amerongen AVN (1997) In vivo binding of the salivary glycoprotein EP-GP (identical to GCDFP-15) to oral and non-oral bacteria detection and identification of EP-GP binding species. Biol Chem 378:83–88
Schwartz C, Chabanet C, Lange C, Issanchou S, Nicklaus S (2011) The role of taste in food acceptance at the beginning of complementary feeding. Physiology & Behavior 104:646–652
Schwartz C, Chabanet C, Laval C, Issanchou S, Nicklaus S (2013) Breast-feeding duration: influence on taste acceptance over the first year of life. British Journal of Nutrition 109(06):1154–1161
Schwartz C, Issanchou S, Nicklaus S (2009) Developmental changes in the acceptance of the five basic tastes in the first year of life. Br J Nutr 102:1375–1385
Shatzman AR, Henkin RI (1981) Gustin concentration changes relative to salivary zinc and taste in humans. Proc Nat Acad Sci USA 78:3867–3871
Thatcher BJ, Doherty AE, Orvisky E, Martin BM, Henkin RI (1998) Gustin from human parotid saliva is carbonic anhydrase VI. Biochem Biophys Res Commun 250:635–641
Acknowledgments
The authors wish to thank the OPALINE team for participant recruitment, saliva sampling and cohort management. This study was financially supported by the French National Research Agency (grant ANR-06-PNRA-028 OPALINE) and by the Regional Council of Burgundy (grants OPALINE and GUSTOLF).
Conflict of interest
The authors declare no conflict of interest.
Author information
Authors and Affiliations
Corresponding author
Rights and permissions
About this article
Cite this article
Morzel, M., Chabanet, C., Schwartz, C. et al. Salivary protein profiles are linked to bitter taste acceptance in infants. Eur J Pediatr 173, 575–582 (2014). https://doi.org/10.1007/s00431-013-2216-z
Received:
Revised:
Accepted:
Published:
Issue Date:
DOI: https://doi.org/10.1007/s00431-013-2216-z