Abstract
Some marine animals have a dynamic mutualistic association involving heterotrophic coral animals (hosts) and autotrophic dinoflagellate endosymbionts in the genus Symbiodinium, known as zooxanthellae, which are directly involved in their physiology. Currently, there is limited knowledge of the Symbiodinium diversity associated with zoanthids from the Brazilian coast. Hence, this study assessed the diversity of zooxanthellae associated with Palythoa caribaeorum, Zoanthus sociatus and Protopalythoa variabilis commonly found on the northeast coast. Zoanthids samples were collected from sandstone reefs, and the total DNA from the samples was extracted. Diversity was assessed by denaturing gradient gel electrophoresis (DGGE) and ITS region cloning library. Data from ITS region sequences showed that zoanthids hosted two phylogenetic subclades or subgenus (C1 and A3) closely related to Symbiodinium from previous studies. Subclade C1 was found in Pa. caribaeorum, Pr. variabilis and Z. sociatus, while subclade A3 was found exclusively in Z. sociatus. This suggests that Pa. caribaeorum and Pr. variabilis are extremely selective, whereas Z. sociatus shows greater flexibility in the selection of its symbionts, and these differences may be involved with living in different reef environments. Knowledge of zooxanthellae diversity associated with zoanthids can explain their large distribution in Brazilian coast and contribute to a better understanding of zoanthid’s sensitivity to thermal stress.
Similar content being viewed by others
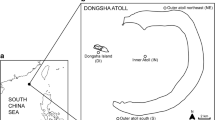
Avoid common mistakes on your manuscript.
1 Introduction
Dinoflagellates in the genus Symbiodinium known as zooxanthellae are the most common endosymbionts in invertebrates (Trench 1993, 1997; Yellowlees et al. 2008). The symbiosis between animals and zooxanthellae combines heterotrophic organisms (host animal) and autotrophs (dinoflagellate). A diverse array of marine invertebrate species are hosts to dinoflagellates of the genus Symbiodinium, including cnidarians (Baker 2003; Yellowlees et al. 2008), nudibranch molluscs (Burghardt et al. 2008; Moore and Gosliner 2011), bivalves (Carlos et al. 1999; Kirkendale 2009), foraminiferans (Pochon et al. 2001; Fay et al. 2009) and sponges (Annenkova et al. 2011; Hill et al. 2011).
The symbiotic association is often obligatory and mutualistic, and involves a controlled exchange of nutrients and energy between the parties (Muscatine and Porter 1977). The most important shared benefits of this association are nutritional since, when they are in symbiosis, dinoflagellates maintain their photosynthetic capacity and transfer many of the compounds produced to the host, contributing substantially to the supply of carbon (Muscatine 1990; Allemand et al. 1998; Furla et al. 2005) and nitrogen (Muscatine et al. 1989; Furla et al. 2005; Yellowlees et al. 2008). Zooxanthellae provide a high proportion of the daily energy requirement needed for the survival of many scleractinian corals (Muscatine and Porter 1977; Falkowski et al. 1984; Edmunds and Davies 1986) and zoanthids (Trench 1974). In addition, nutrients translocated from the symbiont contribute to the formation of the carbonate and calcium skeleton (Reef et al. 2009), and contribute to screening against ultraviolet radiation (Al-Utaibi et al. 2009; Reef et al. 2009). In return, the host contributes to the metabolism of the endosymbiont, transferring essential nutrients such as nitrogen and phosphorus (Cook and D’Elia 1987).
Molecular biology studies have revealed that the genus Symbiodinium is highly diverse, with different subgenera or subgroups, known as clades (Coffroth and Santos 2005). Many of these clades contain numerous species that posses different ecological and physiological attributes (Sampayo et al. 2008; LaJeunesse et al. 2012, 2014; Thornhill et al. 2014). Many Symbiodinium sp. are specific to certain geographical regions and hosts, but some of them can also be more generalist (Rowan and Powers 1991a, b; Visram and Douglas 2006; Baker 2003).
Cnidarians of the order Zoanthidea contain dense populations of zooxanthellae (Reimer 1971; Costa et al. 2013), and receive part of their energy requirements from photosynthesis (Trench 1974; Sebens 1977). Zoanthids are common to coral reefs and along intertidal rocky shores, forming dense colonies on the substrate (Suchanek and Green 1981; Sebens 1982; Bastidas and Bone 1996; Ryland and Lancaster 2003). Along the Brazilian coast, zoanthids are widely distributed and form dense aggregations in intertidal environments and shallow waters (Villaça and Pitombo 1997; Oigman-Pszczol et al. 2004; Pérez et al. 2005; Rabelo et al. 2013).
Although they are widely distributed, there have been few studies on the symbiotic relationship between zoanthids and Symbiodinium in South America. In Brazil, only Costa et al. (2013) and Thornhill et al. (2014) have previously examined the diversity of Symbiodinium present in zoanthids, while other studies involving zooxanthellae of Brazilian cnidarians refer to scleractinian corals (Costa et al. 2004, 2008; Thornhill et al. 2014). The present study used molecular methods to examine the diversity of zooxanthellae from three species of zoanthids that are common on the Brazilian coast: Palythoa caribaeorum (Duchassaing, Michelotti 1860), Protopalythoa variabilis (Duerden, 1898) and Zoanthus sociatus (Ellis, 1768). Considering the fundamental importance of zooxanthellae to the survival of zoanthids and the imminent threats to marine environments, an understanding of the composition, diversity and complexity of the interactions between zooxanthellae and their hosts will provide important information about the biology of these interactions, generating knowledge for the conservation of these organisms.
2 Materials and methods
2.1 Sample collection and laboratory maintenance
Samples of healthy colonies of the zoanthids Palythoa caribaeorum, Protopalythoa variabilis and Zoanthus sociatus were collected in March 2010. They were collected from sandstone reefs on Paracuru Beach, on the coast of the state of Ceará (03°23’53.0”S, 39°00’38.8”W) in northeastern Brazil (Fig. 1), during spring low tide. The sampling area consisted of a flat surface in the supralittoral and mesolittoral, with irregular relief in the infralittoral, where the zonation of zoanthids is very evident with Pa. caribaeorum and Pr. variabilis colonizing tide pools and flat areas, protected and constantly wet, whereas Z. sociatus occurring in exposure areas on the top or high rocks (Rabelo et al. 2014 in press). Since the associated microbiota can be present in the mucus surface layer as well as in the tissue, samples were scraped to the base of the column.
2.2 DNA extraction, PCR-DGGE and sequencing
The ITS (Internal Transcribed Spacer) region of DNA was used to study the diversity of zooxanthellae present in the zoanthids. This region contains 500 to 600 base pairs and is composed of ITS1, 5.8S and ITS2 regions.
Total DNA from the samples was extracted according to the protocol of Roger and Bendich (1985), using cetyltrimethylammonium bromide (CTAB), eluted using 50 μL of elution buffer and stored at −20 °C.
Total DNA extracted from the zoanthids was subjected to Denaturing Gradient Gel Electrophoresis (DGGE) to determine differences in the structure of the Symbiodinium community present in the zoanthids. The amplification reaction of the gene used the specific primers for the ITS region of DNA from Symbiodinium: ITSintfor2 (5′-GAA TTG CAG AAC TCC GTG-3′) and ITS2CLAMP (5′-CGC CCG CCG CGC CCC GCG CCC GTC CCG CCG CCC CCG CCC GGG ATC CAT ATG CTT AAG TTC AGC GGGT-3′), and was carried out using the “Touchdown” protocol as described by LaJeunesse (2002).
Due to use of a different electrophoresis system, the DGGE protocol by LaJeunesse (2002) was modified. DGGE was performed using a BioRad DCode™ system and acrylamide gels were prepared with denaturing gradients of 45 to 80 %, using a 100 % denaturing solution (7 M urea and 40 % formamide) and a 0 % solution (without urea and formamide). Electrophoresis was carried out at constant 60 °C and 150 V for 9.5 h, using the buffer solution TAE 50× (2 M Tris base, 1 M glacial acetic acid and 50 mM EDTA, pH 8). After electrophoresis the gel was immersed in a solution of SYBR Green (Invitrogen) at the concentration of 1:10,000 (v/v) for 1 h. The gel was photographed under ultraviolet light in a photodocumentor. Profiles generated were normalized and the band patterns were compared using the program Bionumerics 4.0 (Applied Maths, Sint-Martens-Latem, Belgium). The program generated matrices of absence (0) and presence (1) which were used to determine the exclusive and shared bands, and for calculating the Jaccard similarity coefficient, with a 1 % tolerance for band position.
To construct the clone library, the ITS region was amplified by PCR using specific primers: ITSfor (5’-GGGATCGTTTCCGTAGGTGAACCTGC-3’) and ITS2rev (5’-GGGATCCATATGCTTAAGTTCAGCGGGT-3’). The PCR was performed according to Smith et al. (2009). Reaction products were checked on an 1 % agarose gel. Bands were excised from the agarose gel, in order to obtain only the band of interest and exclude possible unspecific amplications. Excised bands and purified using a QIAquick® PCR Purification Kit (Qiagen, USA). Fragments were cloned in pGEM®-T Easy Vectors (Promega, USA) according to the manufacturer’s instructions, and were used to transform cells of Escherichia coli TOP 10 F‘by electroporation. The transformed bacteria were cultured, and plasmids from the clones used to sequence the ITS region. Ninety-six Symbiodinium clones were analyzed from each zoanthid host, totaling 288 clones, which were sequenced by Macrogen, Inc. (www.macrogen.com), using an ABI 3730 sequencer. For the sequencing reaction, an ABI PRISM® BigDye™ kit was used following the manufacturer’s instructions.
Sequences obtained were selected according to their quality and ‘suspect’ sequences were removed (Thornhill et al. 2007). Selected sequences were classified into operational taxonomic units (OTUs), using the program MOTHUR (Schloss et al. 2009) and numbers of each clade of Symbiodinium were used to calculate diversity indices. Program S-Libshuff 1.22 (Singleton et al. 2001) was used to statistically evaluate the differences between libraries. Sequences were aligned and compared against existing sequence datasets deposited in GenBank, the databank maintained by the National Center for Biotechnology Information (NCBI). Each sequence was compared with the databank and only similarities above 90 % were considered. Phylogenetic analysis for the Symbiodinium’s ITS region were conducted using program MEGA version 4.0, by implementing maximum likelihood (ML) analyses with Kimura’s 2-parameter model of substitution. A total of 500 bootstrap replicates were performed to assess statistical significance of internal branching. ITS sequences are available in GenBank, under the accession numbers KP134350 - KP134495.
3 Results
DGGE results indicated similarities among the three species of zoanthids in their communities of zooxanthellae, although the migration pattern of some bands identified for Z. sociatus revealed the presence of exclusive OTUs. Analysis of the OTUs shared among the zoanthids indicated that Z. sociatus harbored the most distinct symbiont community, and the zooxanthellae community present in Pa. caribaeorum most resembled the one present in Pr. variabilis (Fig. 2).
Sequencing of cloned zooxanthellae’s ITS region revealed that zoanthids only host two clades of Symbiodinium. Comparison of the sequences with those deposited in GenBank showed that 100 % of the clones identified in Pa. caribaeorum and Pr. variabilis were highly similar to sequences of Symbiodinium from clade C, subclade C1. Clones of Symbiodinium associated with Z. sociatus were classified as belonging to subclades A3 (41.8 %) and C1 (58.2 %).
With regard to the Shannon and Simpson diversity indexes, Z. sociatus showed the highest diversity of zooxanthellae, and was statistically different from Pa. caribaeorum and Pr. variabilis (Table 1). Data generated by Libshuff showed that the library obtained from Z. sociatus differed significantly from the others (p < 0.05).
Phylogenetic reconstruction based on sequence from ITS region indicates two lineages belonging to clades A and C (Fig. 3). Sequences of Symbiodinium identified in the zoanthids showed a close relationship with Symbiodinium present in bivalves (GenBank accession no. AB294609), hermatypic corals (JN711495) and cloned and cultured Symbiodinium (EU074880, EU074857, AF427465).
Neighbor-Joining (NJ) tree for internal transcribed spacer of ribosomal DNA (IST-rDNA) of Symbiodinium clades A and C from zoanthids colonies at Paracuru Beach, northeastern Brazil. Values at branches represent NJ Boostrap probability. Corresponding GenBank accession numbers are provides below each symbiont type. * Represent Sequences acquired in previous studies
4 Discussion
In this study, the diversity of Symbiodinium in three species of zoanthids was assessed using the ITS region as a marker. Although other markers are also commonly used (LaJeunesse et al. 2012; Jeong et al. 2014), ITS region has been used as a good molecular marker in previous analysis of Symbiodinium diversity and ecology (LaJeunesse 2001; van Oppen et al. 2001, 2005; Reimer et al. 2006a, b; Apprill and Gates 2007; Thornhill and Lord 2010; Pochon et al. 2012).
DGGE results showed some shared OTUs among the three species of zoanthids, suggesting a similarity among the associated Symbiodinium communities. Nevertheless, the presence of exclusive OTUs in Z. sociatus suggests specificity between some symbionts and their host. According to the DGGE analysis, Z. sociatus appeared to differ the most in its symbionts, as confirmed by the sequencing data for the cloned ITS region. The presence of multiple bands on the DGGE profile can be related to intragenomic variation within the same clade.According LaJeunesse et al. (2003), bands that co-occurred repeatedly with the same relative intensity can be codominant intragenomic variants and diagnostic of a single Symbiodinium type. Probably, the multiples bands found here represent intragenomic variants of clade C since, according to Thornhill et al. (2007) Symbiodinium Clade C have a greater number of intragenomic rDNA variations than other Symbiodinium types.
Analysis of the cloned ITS region showed that zoanthids contain two clades of Symbiodinium, with all three species of zoanthids hosting zooxanthellae of clade C, subclade C1 while sequences belonging to clade A, subclade A3 were found exclusively in Z. sociatus. This suggests that Pa. caribaeorum and Pr. variabilis are extremely selective for clade C, whereas Z. sociatus shows greater flexibility in the selection of its symbionts. Costa et al. (2013) showed a similar pattern in their study of zoanthids from another region of northeastern Brazil, with Z. sociatus harboring predominantly clades A3 and Pa. caribaeorum and Pr. variabilis harboring clade C1.
Other researchers have studied Palythoa caribaeorum and found clade C in colonies collected in the Caribbean (Lajeunesse 2002; Finney et al. 2010). Clade C was also found in colonies of the genus Palythoa collected in Cape Verde (Reimer et al. 2010), Thailand and Tanzania (Lajeunesse et al. 2010), Australia and Mexico (Lajeunesse et al. 2004a), Hawaii (Rowan and Powers 1991a; Lajeunesse et al. 2004b), Indonesia, Thailand and Maldives (Burnett 2002), Singapore (Reimer and Todd 2009), Galapagos (Reimer and Hickman 2009), Taiwan (Reimer et al. 2013) and Japan (Reimer et al. 2006b, 2011). Previous studies revealed that clade C is also geographically widely distributed in zoanthids of the genus Protopalythoa, and has been found in symbiosis with colonies collected in Mexico (Lajeunesse et al. 2008) and Hawaii (Rowan and Powers 1991a; Lajeunesse et al. 2004b). In zoanthids of the genus Zoanthus, clade C was identified in colonies collected in Japan (Reimer et al. 2007, 2011), Mexico (Lajeunesse 2002), Australia (LaJeunesse et al. 2004a), Singapore (Reimer and Todd 2009), Galapagos (Reimer and Hickman 2009) and Hawaii (Lajeunesse et al. 2004b).
The association of clade A with scleractinian corals has been widely reported (Garren et al. 2006; Loh et al. 2006; Visram and Douglas 2006; Baums et al. 2010; Hennige et al. 2011). In zoanthids, clade A is also widely distributed around the world, and has been found in Zoanthus collected in Japan (Reimer et al. 2006c, 2007) and in the Atlantic Ocean, in countries such a Mexico (LaJeunesse 2002; LaJeunesse et al. 2008), Jamaica (LaJeunesse 2001), the Bahamas (LaJeunesse 2002), the Caribbean (Rowan and Powers 1991a) and Cape Verde (Reimer et al. 2010). This study’s results are in accordance with current literature which indicates that among species of the family Zoanthidae, clade A (type A3 in this study) occurs only in the genus Zoanthus.
Although in this study only subclades A3 and C1 were found in Z. sociatus, LaJeunesse (2002) observed that colonies of this species collected in Yucatan Peninsula (Mexico) showed clades A4, A3, B1 and C1. The finding of different clades and subclades in works by LaJeunesse (2002) that were absent from the samples examined in the present study, may be a consequence of specificity to the environment, since some clades (and subclades) of Symbiodinium are closely related to the local environmental characteristics. Some authors recently described variations in Symbiodinium types collected from different depths. For Z. sansibaricus, a sibling species of Z. sociatus (Reimer et al. 2012), subclades A1 and C1 were found in shallow waters (Reimer et al. 2006c, 2007; Kamezaki et al. 2013) whereas different types of subclade C1 were found at greater depths (Kamezaki et al. 2013).
The presence of subclade C1 in hosts from intertidal zones, shallow and greater depths waters, whereas subclade A3 was typically found in more stressed areas (such as shallow waters and stressed areas of intertidal zones), similarly to what was observed by Reimer et al. (2006a,b, 2007, 2012), Costa et al. (2013) and Kamezaki et al. (2013), confirm that type C1 is more generalist regarding different environment conditions than clade A. Our results suggest that type C1 has a great ability to survive in protected and exposed areas and support the hypothesis that type A3, although less generalist, is more resistant than type C1.
Magalon et al. (2007) noted that although some corals show high specificity for their symbionts, certain corals may contain different clades of Symbiodinium depending on the region and on the tolerance characteristics of each clade. Thus, although highly species-specific, corals of the same species may vary in their symbionts depending on environmental characteristics. Many authors have associated Symbiodinium of clade C with several species of hosts capable of surviving in many geographical regions and under different environmental conditions (Chen et al. 2005; Costa et al. 2008; Garren et al. 2006; Pochon et al. 2010; van Oppen et al. 2001, 2005; Yamashita et al. 2010).
However, this is not exclusive to coral species. Similar pattern have been observed in the zoanthid Palythoa caesia where zooxanthellae clade C was found throughout the Indian Ocean, whereas type D was present in all populations east of the Maldives but absent from all populations to the west (Burnett 2002). Reimer et al. (2006c, 2007) demonstrated that Z. sansibaricus from different islands of Japan had different clades of Symbiodinium. Kamezaki et al. (2013) found clade A in Z. sansibaricus collected in intertidal zones whereas clade C was present in Z. sansibaricus from lower depths. In Brazil, Thornhill et al. (2014) found only clade C in Z. sociatus collected in the southern region, whereas in the present study we found subclades A3 and C1 in the same species in the northeastern region. This data suggests that zoanthids’ flexibility in their symbionts is dependent of local characteristics and could explain why Z. sociatus does not contain clade B (identified by LaJeunesse 2002) for the same species. According to Baker (2001), variation in the diversity of zooxanthellae according to environmental characteristics is advantageous; it provides a mechanism for the host to adapt to changes in environmental conditions, since different clades of zooxanthellae differ in their thermotolerance (Kinzie et al. 2001) and may contribute in some way to the thermotolerance of the holobiont (Magalon et al. 2007).
Identification of type A3 only in Z. sociatus agreed with the hypothesis suggested by Rabelo (2014 in press) that this zoanthid is the most resistant to desiccation and exposure to sunlight, occurring in locations that are fully exposed during low tide and where no other cnidarians are present. At this study’s collection site, zoanthids occur in the entire intertidal zone, predominantly in the middle and outer zones. In fact, the presence of dense colonies of Z. sociatus on high rocks at the study site, where they are exposed to solar radiation, requires these animals to have greater resistance to adverse factors and this resistance may be associated with the presence of clade A in their tissues. Symbiodinium clade A appears to be adapted to stressful environmental conditions, as previously observed by Kinzie et al. (2001) and Magalon et al. (2007). Reimer et al. (2006b) noted that Symbiodinium clade A is more adapted to high light intensity and high temperatures, and observed that exposure to sunlight might influence the composition of Symbiodinium in the host. It is known that only zooxanthellae clade A are capable of producing considerable amounts of mycosporine-like amino acids (MAAs) (Banaszak et al. 2000), compounds that help to protect against damage from UV radiation (Neale et al. 1998). Protection against UV rays through synthesis of MAAs may confer an adaptive advantage under conditions of high irradiance, influencing the zonation of cnidarians. In addition, the combination of different clades (such as A3 and C1 in Z. sociatus) may provide the host with greater flexibility in acclimating to different environments and greater resistance in different environmental situations (Fabricius et al. 2004; Berkelmans and van Oppen 2006).
Karako-Lampert et al. (2004) noted that zooxanthellae of clade C also show tolerance to changes in temperature and low susceptibility to bleaching (Glynn 1993). After a bleaching event, Visram and Douglas (2006) observed that clade C was present in all the species of coral studied, demonstrating the resistance of this clade to environmental changes. Considering this capability and fact that clade C is generalist to environment, the presence of clade C in zoanthids may also be advantageous for the survival and wide distribution of these animals in the intertidal zone in Brazilian coast, an area characterized by thermal and salt stress during the tidal cycle.
At study site, Pa. caribaeorum and Pr. variabilis occur in flat areas, protected and constantly wet due to the formation of small tidal pools. Apparently, these two species are less resistant to desiccation, and do not occur in the coverage area of Z. sociatus, even if substrate is available for colonization. The reason that Pa. caribaeorum and Pr. variabilis do not form associations with Symbiodinium subclade A3 is unknown, but the lower resistance of the host to adverse factors suggested that it could be associated with the absence of clade A, forcing these zoanthids to settle in areas that are less exposure to stress.
The presence of type C1 in the studied zoanthids implies that these animals developed a high specificity for this clade during the course of their evolution, and that this adaptation may have facilitated the survival of zoanthids in many different environments, allowing them to disperse widely throughout the oceans. This data also suggests that, although found in geographically distant locations, species of zoanthids contain similar symbiont composition which indicates that zoanthids (host) have developed a relation with specific symbionts. In addition, the close similarity between Symbiodinium types A3 and C1 sequences from this study and those from the GenBank database indicates that these zooxanthellae are generalists, occurring in diverse groups of animal hosts making it not only generalist with respect to the geographical regions, but also for different host.
The presence of subclade C1 in both Pa. caribaeorum and Pr. variabilis, this similarity can be explained by the close phylogenetic relationship between these two species. In recent years, there has been a certain confusion respecting the genera Palythoa and Protopalythoa, both of which were formerly united in the genus Palythoa. Current taxonomic understanding, using morphological characteristics, is that Palythoa and Protopalythoa constitute separate taxa (Ryland and Lancaster 2003). Nevertheless, Reimer et al. (2006a) used molecular techniques to show that Palythoa and Protopalythoa are not separate genera, and argued that these species comprise congeneric species and/or subspecies within the genus Palythoa. Baker (2003) agreed with the hypothesis that closely related species can have the same specificity for their symbionts, which would explain the similarity in the preference of these zoanthids only for type C1.
Even though Z. sociatus shelters two clades that confer more resistance to bleaching, this phenomenon can still occur. Under normal conditions, this zoanthid species is apparently more resistant to exposure to sunlight and increased temperature (Rabelo et al. 2014 in press). Nevertheless, temperature anomalies occurring in 2009 led to the complete bleaching and death of many colonies of this species (Soares and Rabelo 2014), which indicates that even those zoanthids that are more resistant to bleaching can be exposed to stress levels that exceed their tolerance limit, leading to the population decline of these animals. Therefore, it is necessary to know the composition of zooxanthellae in zoanthid species susceptible to bleaching, to aid in interventions such as the repopulation of zooxanthellae in hosts damaged by bleaching, which has been demonstrated to work experimentally (Kinzie and Chee 1979; Schoenberg and Trench 1980; Toller et al. 2001).
The phylogenetic trees formed two lineages, belonging to clade A and C and suggest that the genetic diversity of Symbiodinium can be large, as reported by Thornhill et al. (2014), Finney et al. (2010) and LaJeunesse and Thornhill (2011).
This study provided important information about the symbiosis between dinoflagellates and zoanthids, and contributed to a better understanding of the ecology of symbiosis in Brazilian species. Knowledge of the ability of these animals to shelter zooxanthellae of diverse types can help to comprehend the capacity of these cnidarians to survive thermal stress events, and is a starting point for a better understanding of the health of reef environments, which are extremely sensitive and currently under strong pressure from anthropogenic activities and climate change.
References
Allemand D, Furla P, Bénazet-Tambutte S (1998) Mechanisms of carbon acquisition for endosymbiont photosynthesis in Anthozoa. C J Bot 76:925–941
Al-Utaibi AA, Niaz GR, Al-Lihaibi SS (2009) Mycosporine-like amino acids in six scleractinian coral species. Oceanologia 51:93–104
Annenkova NV, Lavrov DV, Belikova SI (2011) Dinoflagellates associated with freshwater sponges from the ancient Lake Baikal. Protist 162:222–236
Apprill AM, Gates RD (2007) Recognizing diversity in coral symbiotic dinoflagellate communities. Mol Ecol 16:1127–1134
Baker AC (2001) Reef corals bleach to survive change. Nature 411:765–766
Baker AC (2003) Flexibility and specificity in coral algal symbiosis: diversity, ecology, and biogeography of Symbiodinium. Annu Rev Ecol Evol Syst 34:661–689
Banaszak AT, Lajeunesse TC, Trench RK (2000) The synthesis of mycosporine-like amino acids (MAAs) by cultured symbiotic dinoflagellates. J Exp Mar Biol Ecol 249:219–233
Bastidas C, Bone D (1996) Competitive strategies between Palythoa caribaeorum and Zoanthus sociatus (Cnidaria:Anthozoa) at a reef flat environmental in Venezuela. Bull Mar Sci 59:543–555
Baums IB, Johnson ME, Devlin-Durante MK, Miller MW (2010) Host population genetic structure and zooxanthellae diversity of two reef-building coral species along the Florida Reef Tract and wider Caribbean. Coral Reefs 29:835–842
Berkelmans R, van Oppen MJ (2006) The role of zooxanthellae in the thermal tolerance of corals: a “nugget of hope” for coral reefs in an era of climate change. Proc Biol Sci 273:2305–2312
Burghardt I, Stemmera K, Wägele H (2008) Symbiosis between Symbiodinium (Dinophyceae) and various taxa of Nudibranchia (Mollusca: Gastropoda), with analyses of long-term retention. Org Divers Evol 8:66–76
Burnett JW (2002) Longitudinal variation in algal symbionts (zooxanthellae) from the Indian Ocean zoanthid Palythoa caesia. Mar Ecol Prog Ser 234:105–109
Carlos AA, Baillie BK, Kawachi M, Maruyama T (1999) Phylogenetic position of Symbiodinium (Dinophyceae) isolates from Tridacnids (Bvalvia), Cardiids (Bivalvia), a sponge (Porifera), a soft coral (Anthozoa), and a free-living strain. J Phycol 35:1054–1062
Chen CA, Yang Y-W, Wei NV, Tsai W-S, Fang L-S (2005) Symbiont diversity in scleractinian corals from tropical reefs and subtropical non-reef communities in Taiwan. Coral Reefs 24:11–22
Coffroth MA, Santos SR (2005) Genetic diversity of symbiotic dinoflagellates in the genus Symbiodinium. Protist 156:19–34
Cook CB, D’elia CF (1987) Are natural populations of zooxanthellae ever nutrient-limited? Symbiosis 4:199–212
Costa CF, Sassi R, Amaral FD (2004) Population density and photosynthetic pigment content in symbiotic dinoflagellates in the Brazilian scleractinian coral Montastrea cavernosa (linnaeus, 1767). Braz J Oceanogr 52(2):93–99
Costa CF, Sassi R, Gorlach-Lira K (2008) Zooxanthellae genotypes in the coral Siderastrea stellata from coastal reefs in northeastern Brazil. J Exp Mar Biol Ecol 367:149–152
Costa CF, Sassi R, Gorlach-Lira K, Lajeunesse TC, Fitt WK (2013) Seasonal changes in zooxanthellae harbored by zoanthids (Cnidaria, Zoanthidea) from coastal reefs in northeastern Brazil. Pan Am J Aquat Sci 8:253–264
Edmunds PJ, Davies SP (1986) An energy budget for Porites porites (Scleractinia). Mar Biol 92:339–347
Fabricius KE, Mieog JC, Colin PL, Idip D, van Oppen MJH (2004) Identity and diversity of coral endosymbionts (zooxanthellae) from three Palauan reefs with contrasting bleaching, temperature and shading histories. Mol Ecol 13:2445–2458
Falkowski PG, Dubinsky Z, Muscatine L, Porter JW (1984) Light and bioenergetics of a symbiotic coral. Bioscience 34:705–709
Fay SA, Weber MX, Lipps JH (2009) The distribution of Symbiodinium diversity within individual host foraminifera. Coral Reefs 28:717–726
Finney JC, Pettay DT, Sampayo EM, Warner ME, Oxenford HA, Lajeunesse TC (2010) The relative significance of host–habitat, depth, and geography on the ecology, endemism, and speciation of coral endosymbionts in the genus Symbiodinium. Microb Ecol 1:250–263
Furla P, Allemand D, Shick JM, Ferrier-Pagès C, Richier S, Plantivaux A, Merle PL, Tambutté S (2005) The symbiotic anthozoan: a physiological chimera between alga and animal. Integr Comp Biol 45:595–604
Garren M, Walsh SM, Caccone A, Knowlton N (2006) Patterns of association between Symbiodinium and members of the Montastraea annularis species complex on spatial scales ranging from within colonies to between geographic regions. Coral Reefs 25:503–512
Glynn PW (1993) Coral reef bleaching: ecological perspectives. Coral Reefs 12:1–17
Hennige SJ, Mcginley MP, Grottoli AG, Warner ME (2011) Photoinhibition of Symbiodinium spp. within the reef corals Montastraea faveolata and Porites astreoides: implications for coral bleaching. Mar Biol 158:2515–2526
Hill M, Allenby A, Ramsby B, Schönberg C, Hill A (2011) Symbiodinium diversity among host clionid sponges from Caribbean and Pacific reefs: evidence of heteroplasmy and putative host-specific symbiont lineages. Mol Phylogenet Evol 59:81–88
Jeong HJ, Lee SY, Kang NS, Yoo YD, Lim AS, Lee MJ, Kim HS, Yih W, Yamashita H, LaJeunesse TC (2014) Genetics and morphology characterize the dinoflagellate Symbiodinium voratum, n. sp., (Dinophyceae) as the sole representative of Symbiodinium Clade E. J Euk Microbiol 61:75–94
Kamezaki M, Higa M, Hirose M, Suda S, Reimer JD (2013) Different zooxanthellae types in populations of the zoanthid Zoanthus sansibaricus along depth gradients in Okinawa, Japan. Mar Biodivers 43:61–70
Karako-Lampert S, Katcoff DJ, Achituv Y, Dubinsky D, Stambler N (2004) Do clades of symbiotic dinoflagellates in scleractinian corals of the Gulf of Eilat (Red Sea) differ from those of other coral reefs? J Exp Mar Biol Ecol 311:301–314
Kinzie RA, Chee GS (1979) The effect of different zooxanthellae on the growth of experimentally reinfected hosts. Biol Bull 156:315–327
Kinzie RA, Takayama M, Santos SR, Coffroth MA (2001) The adaptive bleaching hypothesis: experimental tests of critical assumptions. Biol Bull 200:51–58
Kirkendale L (2009) Their day in the sun: molecular phylogenetics and origin of photosymbiosis in the “other” group of photosymbiotic marine bivalves (Cardiidae:Fraginae). Biol J Linn Soc 97:448–465
LaJeunesse TC (2001) Investigating the biodiversity, ecology, and phylogeny of endosymbiotic dinoflagellates in the genus Symbiodinium using the ITS region: in search of a “species” level marker. J Phycol 880:866–880
LaJeunesse TC (2002) Diversity and community structure of symbiotic dinoflagellates from Caribbean coral reefs. Mar Biol 141:387–400
LaJeunesse TC, Thornhill DJ (2011) Improved resolution of reef-coral endosymbiont (Symbiodinium) species diversity, ecology, and evolution through psbA non-coding region genotyping. PloS One e29013
LaJeunesse TC, Loh WKW, van Woesik R, Hoegh-Guldberg O, Schmidt GW, Fitt WK (2003) Low symbiont diversity in southern Great Barrier Reef corals relative to those of the Caribbean. Limnol Oceanogr 48:2046–2054
LaJeunesse TC, Bhagooli R, Hidaka M, Done T, Devantier L, Schmidt GW, Fitt WK, Hoegh-Guldberg O (2004a) Closely-related Symbiodinium spp. differ in relative dominance within coral reef host communities across environmental, latitudinal, and biogeographic gradients. Mar Ecol Progr Ser 284:147–161
Lajeunesse TC, Thornhill DJ, Cox E, Stanton F, Fitt WK, Schmidt GW (2004b) High diversity and host specificity observed among symbiotic dinoflagellates in reef coral communities from Hawaii. Coral Reefs 23:596–603
Lajeunesse TC, Reyes-Bonilla H, Warner ME, Wills M, Schmidt GW, Fitt WK (2008) Specificity and stability in high latitude eastern Pacific coral-algal symbioses. Limnol Oceanogr 53:719–727
Lajeunesse TC, Pettay T, Phongsuwan N, Brown B, Obura D, Hoegh-Guldberg O, Fitt WK (2010) Long-standing environmental conditions, geographic isolation and host-symbiont specificity influence the relative ecological dominance and genetic diversification of coral endosymbionts in the genus Symbiodinium. J Biogeogr 37:785–800
LaJeunesse TC, Parkinson JE, Reimer JD (2012) A genetics-based description of Symbiodinium minutum sp. nov. and S. psygmophilum sp. nov. (Dinophyceae), two dinoflagellates symbiotic with Cnidaria. J Phycol 48:1380–1391
LaJeunesse TC, Wham DC, Pettay DT, Parkinson JE, Keshavmurthy S, Chen CA (2014) Ecologically differentiated stress tolerant endosymbionts in the dinoflagellate genus Symbiodinium Clade D are different species. Phycologia 53:305–319
Loh WKW, Loi T, Carter D, Hoegh-Guldberg O (2006) Genetic variability of the symbiotic dinoflagellates from the wide ranging coral species Seriatopora hystrix and Acropora longicyathus in the Indo-West Pacific. Mar Ecol Prog Ser 222:97–107
Magalon H, Flot JF, Baudry E (2007) Molecular identification of symbiotic dinoflagellates in Pacific corals in the genus Pocillopora. Coral Reefs 26:551–558
Moore EJ, Gosliner TM (2011) Molecular phylogeny and evolution of symbiosis in a clade of Indopacific nudibranchs. Mol Phylogenet Evol 58:116–123
Muscatine L (1990) The role of symbiotic algae in carbon and energy flux in reef corals. In: Dubinsky Z (ed) Coral reefs, 1st edn. Elsevier, Amsterdam, pp 75–87
Muscatine L, Porter JW (1977) Reef corals: mutualistic symbioses adapted to nutrient poor environments. Bioscience 27:454–460
Muscatine L, Falkowski PG, Dubinsky Z, Cook PA, Mccloskey LR (1989) The effect of external nutrient resources on the population dynamics of zooxanthellae in a reef coral. P Roy Soc Lond B Bio 236:311–324
Neale PJ, Banaszak AT, Jarriel CR (1998) Ultraviolet sunscreens in Gymnodinium sanguineum (Dinophyceae): mycosporine-like amino acids protect against inhibition of photosynthesis. J Phycol 34:928–938
Oigman-Pszczol SS, Figueiredo MAO, Creed JC (2004) Distribution of benthic communities on the tropical rocky subtidal of Armacão dos Búzios, Southeastern Brazil. Mar Ecol 25:173–190
Pérez CD, Vila-Nova DA, Santos AM (2005) Associated community with the zoanthid Palythoa caribaeorum (Duchassaing & Michelotti, 1860) (Cnidaria, Anthozoa) from littoral of Pernambuco, Brazil. Hydrobiologia 548:207–215
Pochon X, Pawlowski J, Zaninetti L, Rowan R (2001) High genetic diversity and relative specificity among Symbiodinium-like endosymbiotic dinoflagellates in soritid foraminiferans. Mar Biol 6:1069–1078
Pochon X, Stat M, Takabayashi M, Chasqui L, Chauka LJ, Logan DDK, Gates RD (2010) Comparison of endosymbiotic and free-living Symbiodinium(Dinophyceae) diversity in a Hawaiian reef environment. J Phycol 4:53–65
Pochon X, Putnam HM, Burki F, Gates RD (2012) Identifying and characterizing alternative molecular markers for the symbiotic and free-living dinoflagellate genus Symbiodinium. PLoS ONE 7:e29816
Rabelo EF, Soares MO, Matthews-Cascon H (2013) Competitive interactions among zoanthids (Cnidaria: Zoanthidae) in an intertidal zone of Northeastern Brazil. Braz J Oceanogr 61:35–42
Rabelo EF, Soares MO, Bezerra LEA, Matthews-Cascon H (2014) Distribution pattern of Zoanthids (Cnidaria: Zoantharia) on a tropical reef. Mar Biol Res (in press)
Reef R, Kaniewska P, Hoegh-Guldberg O (2009) Coral skeletons defend against ultraviolet radiation. PLoS ONE 4:e7995
Reimer AA (1971) Observations on the relationships between several species of tropical zoanthids (Zoanthidea, Coelenterate) and their zooxanthellae. J Exp Mar Boil Ecol 7:207–214
Reimer JD, Hickman CP Jr (2009) Preliminary survey of zooxanthellate Zoanthids (Cnidaria: Hexacorallia) of the Galapagos, and associated symbiotic dinoflagellates (Symbiodinium spp.). Galápagos Res 66:14–19
Reimer JD, Todd PA (2009) Preliminary molecular examination of zooxanthellate zoanthids (Hexacorallia: Zoantharia) and associated zooxanthellae (Symbiodinium spp.) diversity in Singapore. Raffles Bull Zool 22:103–120
Reimer JD, Ono S, Takishita K, Tsukahara J, Maruyama J (2006a) Molecular evidence suggesting species in the Zoanthid genera Palythoa and Protopalythoa (Anthozoa: Hexacorallia) are congeneric. Zool Sci 23:87–94
Reimer JD, Takishita K, Maruyama T (2006b) Molecular identification of symbiotic dinoflagellates (Symbiodinium spp.) from Palythoa spp. (Anthozoa: Hexacorallia) in Japan. Coral Reefs 25:521–527
Reimer JD, Takishita K, Ono S, Maruyama T, Tsukahara J (2006c) Latitudinal and intracolony ITS-rDNA sequence variation in the symbiotic dinoflagellate genus Symbiodinium (Dinophyceae) in Zoanthus sansibaricus (Anthozoa:Hexacorallia). Phycol Res 54:122–132
Reimer JD, Ono S, Tsukahara J, Takishita K, Maruyama T (2007) Non-seasonal clade-specificity and subclade microvariation in symbiotic dinoflagellates (Symbiodinium spp.) in Zoanthus sansibaricus (Anthozoa: Hexacorallia) at Kagoshima Bay, Japan. Phycol Res 55:58–65
Reimer JD, Hirose M, Wirtz P (2010) Zoanthids of the Cape Verde islands and their symbionts: previously unexamined diversity in the Northeastern Atlantic. Contrib Zool 79:147–163
Reimer JD, Hirose M, Yanagi K, Sinniger F (2011) Marine invertebrate diversity in the oceanic Ogasawara Islands: a molecular examination of zoanthids (Anthozoa: Hexacorallia) and their Symbiodinium (Dinophyceae). Syst and Biodivers 9:133–143
Reimer JD, Foord C, Irei Y (2012) Species diversity of shallow water zoanthids (Cnidaria: Anthozoa: Hexacorallia) in Florida. J Mar Biol Article ID 856079, 14 pages
Reimer JD, Irei Y, Fujii T, Yang S (2013) Molecular analyses of shallow-water zooxanthellate zoanthids (Cnidaria: Hexacorallia) from Taiwan and their Symbiodinium spp. Zool Stud 52:1–16
Roger SO, Bendich AJ (1985) Extraction of DNA from milligram amounts of fresh, herbarium and mummified plant-tissues. Plant Mol Biol 5:69–76
Rowan R, Powers DA (1991a) A molecular genetic classification of zooxanthellae and the evolution of animal-algal symbioses. Science 251:1348–1351
Rowan R, Powers DA (1991b) Molecular genetic identification of symbiotic dinoflagellates (zooxanthellae). Mar Ecol Prog Ser 71:65–73
Ryland SJ, Lancaster JE (2003) Revision of method for separating species of Protopalythoa (Hexacorallia: Zoanthidea) in the tropical West Pacific. Invertrebr Syst 17:407–428
Sampayo EM, Ridgeway T, Bongaerts P, Hoegh-Guldberg O (2008) Bleaching susceptibility and mortality of corals are determined by fine-scale differences in symbiont type. Proc Natl Acad Sci U S A 105:10444–10449
Schloss PD, Westcott SL, Ryabin T, Hall JR, Hartmann M, Hollister EB, Lesniewski RA, Oakley BB, Parks DH, Robinson CJ, Sahl JW, Stres B, Thallinger GG, Van Horn DJ, Weber CF (2009) Introducing mothur: open-source, platform-independent, community-supported software for describing and comparing microbial communities. Appl Environ Microbiol 75:7537–7541
Schoenberg DA, Trench RK (1980) Genetic variation in Symbiodinium (Gymnodinium) microadriaticum Freudenthal, and specificity in its symbiosis with marine invertebrates. III. Specificity and infectivity of S. microadriaticum. Proc R Soc Lond B 207:445–460
Sebens KP (1977) Autotrophic and heterotrophic nutrition of coral reef zoanthids. Proc 3rd Intern Coral Reef Symp 1:398–404
Sebens KP (1982) Intertidal distribuition of zoanthids on the Caribbean coast of Panama: effects of predaction and desiccation. Bull Mar Sci 32:316–335
Singleton DR, Michelle AF, Stephen LRA, William BW (2001) Quantitative comparisons of 16S rRNA gene sequence libraries from environmental samples. Appl Environ Microbiol 67:4374–4376
Smith RT, Pinzón JH, Lajeunesse TC (2009) Symbiodinium (Dinophyta) diversity and stability in aquarium corals. J Phycol 45:1030–1036
Soares MO, Rabelo EF (2014) Primeiro registro de branqueamento de corais no litoral do Ceará (Ne, Brasil): indicador das mudanças climáticas? Geociênc 33:1–10
Suchanek TH, Green DJ (1981) Interespecific competition between Palythoa caribaeorum and other sessile invertebrates on St. Croix Reefs, U.S. Virgin Islands. Proc 4rd Intern Coral Reef Symp 2:679–684
Thornhill DJ, Lord JB (2010) Secondary structure models for the internal transcribed spacer (ITS) region 1 from symbiotic dinoflagellates. Protist 161:434–451
Thornhill DJ, Lajeunesse TC, Santos SR (2007) Measuring rDNA diversity in eukaryotic microbial systems: how intragenomic variation, pseudogenes, and PCR artifacts confound biodiversity estimates. Mol Ecol 16:5326–5340
Thornhill DJ, Lewis AM, Wham DC, LaJeunesse TC (2014) Host-specialist lineages dominate the adaptive radiation of reef coral endosymbionts. Evolution 68:352–367
Toller WW, Rowan R, Knowlton N (2001) Repopulation of zooxanthellae in the Caribbean corals Montastraea annularis and M. faveolata following experimental and disease-associated bleaching. Biol Bull 201:360–373
Trench RK (1974) Nutritional potentials in Zoanthus sociatus (Coelenterata, Anthozoa). Helgol Mar Res 974:174–216
Trench RK (1993) Microalgal-invertebrate symbioses: a review. Endocytobiosis Cell Res 9:135–175
Trench RK (1997) Diversity of symbiotic dinoflagellates and the evolution of microalgal-invertebrate symbioses. Proc Eighth Int Coral Reef Symp 2:1275–1286
van Oppen MJH, Palstra FP, Piquet AM, Miller DJ (2001) Patterns of coral-dinoflagellate associations in Acropora: significance of local availability and physiology of Symbiodinium strains and host-symbiont selectivity. Proc Biol Sci 268:1759–1767
van Oppen MJH, Mieog JC, Sánchez CA, Fabricius KE (2005) Diversity of algal endosymbionts (zooxanthellae) in octocorals: the roles of geography and host relationships. Mol Ecol 14:2403–2417
Villaça R, Pitombo FB (1997) Benthic communities of shallow-water reefs of Abrolhos, Brazil. Rev Bras Oceanogr 45:35–43
Visram S, Douglas AE (2006) Molecular diversity of symbiotic algae (zooxanthellae) in scleractinian corals of Kenya. Coral Reefs 25:172–176
Yamashita H, Suzuki G, Hayashibara T, Koike K (2010) Do corals select zooxanthellae by alternative discharge? Mar Biol 158:87–100
Yellowlees D, Tav R, Leggat W (2008) Metabolic interactions between algal symbionts and invertebrate hosts. Plant Cell Environ 31:679–694
Acknowledgments
This study was conducted in partnership with researchers from the Laboratory of Microbial Ecology and Biotechnology (Lembiotech), whom we sincerely thank. The authors gratefully acknowledge the collaboration of Dr. Janet Reid for English review and valuable suggestions and MSc. Taffarel Torres for phylogenetic tree suggestions. The study received support from CAPES, through the Project Marine Sciences, process no. 0532/2010.
Author information
Authors and Affiliations
Corresponding author
Rights and permissions
About this article
Cite this article
Rabelo, E.F., Rocha, L.L., Colares, G.B. et al. Symbiodinium diversity associated with zoanthids (Cnidaria: Hexacorallia) in Northeastern Brazil. Symbiosis 64, 105–113 (2014). https://doi.org/10.1007/s13199-014-0308-9
Received:
Accepted:
Published:
Issue Date:
DOI: https://doi.org/10.1007/s13199-014-0308-9