Abstract
Purpose
Obstructive sleep apnea (OSA) is independently associated with increased risk for stroke and other cardiovascular diseases. Since activated platelets play an important role in cardiovascular disease, the objective of this study was to determine whether platelet reactivity was altered in OSA subjects with intermittent nocturnal hypoxemia.
Methods
Thirty-one subjects, without hypertension or cardiovascular disease and not taking medication, participated in the study. Subjects were stratified based on OSA-related oxygen desaturation index (ODI) recorded during overnight polysomnography. Platelet reactivity to a broad panel of agonists (collagen, thrombin, protease-activated receptor1 hexapeptide, epinephrine, ADP) was measured by monitoring platelet aggregation and ATP secretion. Expression of platelet activation markers CD154 (CD40L) and CD62P (P-selectin) and platelet-monocyte aggregates (PMA) was quantified by flow cytometry.
Results
Epinephrine-induced platelet aggregation was substantially decreased in OSA subjects with significant intermittent hypoxemia (ODI ≥ 15) compared with subjects with milder hypoxemia levels (ODI < 15) (area under curve, p = 0.01). In addition, OSA subjects with ODI ≥ 15 exhibited decreased thrombin-induced platelet aggregation (p = 0.02) and CD40L platelet surface expression (p = 0.05). Platelet responses to the other agonists, CD62P platelet surface expression, and PMA levels were not significantly different between groups. Reduction in platelet responses to epinephrine and thrombin, and decreased CD40L surface marker expression in significant hypoxemic OSA individuals, is consistent with their platelets being in an activated state.
Conclusions
Increased platelet activation was present in otherwise healthy subjects with intermittent nocturnal hypoxemia due to underlying OSA. This prothrombotic milieu in the vasculature is likely a key contributing factor toward development of thrombosis and cardiovascular disease.
Trial registration
NCT00859950
Similar content being viewed by others
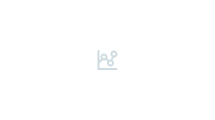
Avoid common mistakes on your manuscript.
Introduction
Obstructive sleep apnea (OSA) is a prevalent condition found to be an independent risk factor for stroke [1,2,3]. Micro- and macro-vascular complications associated with untreated OSA are often independent of other cardiovascular risk factors [1, 3, 4]. Large epidemiological studies have demonstrated the association of OSA with hypertension, atherosclerosis, and vascular thrombosis. Several pathophysiological mechanisms have been postulated, including hemodynamic, neural, circadian rhythm, vascular, endothelial, metabolic, inflammatory, and thrombotic changes [1,2,3,4,5]. Intermittent hypoxemia is often seen in OSA, consisting of recurrent drops in oxyhemoglobin saturation due to reduced airflow during sleep. It is frequently associated with increased oxidative stress and cellular damage, shown to lead to endothelial dysfunction, vascular inflammation, and increased platelet activation and aggregability [1, 2, 4,5,6].
In keeping with the overarching hypothesis that platelet dysfunction is a key mechanism leading to cardiovascular disease in sleep apnea, we evaluated platelet function in otherwise healthy subjects with intermittent hypoxemia secondary to OSA by examining platelet responses to a broad panel of prothrombotic agonists, surface markers of platelet activation, and platelet-monocyte interactions. We hypothesized that platelet activation and the aggregation response would be increased in otherwise healthy subjects with significant intermittent hypoxemia due to underlying OSA. A prothrombotic environment in the vasculature could predispose these individuals to cardiovascular disease. Importantly, this is the first study to investigate platelet responsiveness to a broad panel of agonists as well as platelet activation in OSA subjects with intermittent nocturnal hypoxemia.
Methods
Study population
A cross-sectional study design was used. Informed consent was obtained from all participants under a protocol approved by the Weill Cornell Medicine (WCM) and Rockefeller University Hospital Institutional Review Boards (IRB). The study was registered as Clinical Trial NCT00859950 (“Mechanisms of Endothelial Cell Dysfunction in Sleep Apnea”). Participants with a clinical suspicion of OSA and age-matched healthy controls (± 5 years) without underlying cardiovascular disease were offered participation. Control subjects were chosen to be of similar age to those with suspicion of OSA in order to minimize confounding by age. Healthy controls were recruited using IRB-approved flyers posted in designated research advertisement areas in the institution and through the clinical trials website. Inclusion criteria for all participants were age > 21 years old, no previous diagnosis of sleep apnea, and absence of underlying diabetes or cardiovascular disease including hypertension, coronary artery disease, stroke or peripheral vascular disease, as assessed by review of past medical history and medication list. Exclusion criteria were history of smoking, pregnancy, current or previous treatment for sleep apnea, alcohol abuse, and use of supplemental oxygen. Also excluded were subjects taking prescription or over-the-counter sedatives, respiratory suppressants, medications for cardiovascular disease, hyperlipidemia or hypertension, anticoagulants, and anti-platelet, non-steroid anti-inflammatory drugs, or aspirin during the 3 months preceding study enrollment.
Nocturnal polysomnography
A baseline full-night nocturnal polysomnography (NPSG) was performed at approximately the subjects’ regular sleep time after admission to the Clinical Translational Research Center at Rockefeller University Hospital. The NPSG recording consisted of four-channel electroencephalogram, bilateral electrooculogram, submental and anterior tibialis electromyogram, two-lead electrocardiogram, rib cage and abdominal movement by inductive plethysmography, body position, pulse oximetry, and nasal pressure respiratory flow monitoring. Scoring of all records was performed by a single experienced registered polysomnographic technician. Respiratory events were scored according to recommended rules by the American Academy of Sleep Medicine [7, 8]. The oxygen desaturation index (ODI) was used as a measure of the severity of OSA, calculated based on the hourly index of drops in oxygen saturation (SaO2) during the sleep period (where a desaturation event is defined as a decrease of ≥ 4% lasting ≥ 6 s). Intermittent hypoxemia was classified based on events per hour at a 4% ODI cutoff of 15. The conservative 4% desaturation threshold was utilized in order to be more selective of hypoxemic events and created a well-defined distinction between groups. The minimum desaturation period was included in the criteria to ensure that brief artificial drops in oxygen saturation were not included in the event index. The presence of OSA in the increased ODI group was asserted by an increased obstructive apnea-hypopnea index (OAHI) above the threshold of 5 events per hour. The percentage of sleep time at SaO2 ≤ 90% (% TST < 90%) and lowest saturation level (lowest SaO2) were also recorded and tabulated.
Blood sampling and processing for isolation of platelets
Blood samples were collected during fasting in the morning immediately after the overnight sleep study for measuring fasting glucose, triglycerides, and high-density lipoprotein (HDL) cholesterol in addition to a complete blood count (CBC). Samples were also processed for the isolation of platelets following the collection of 41 ml whole blood by free flow into a tube containing 4.6 ml 3.8% trisodium citrate. For platelet aggregometry studies, platelet-rich plasma (PRP) and platelet-poor plasma (PPP) were prepared by differential centrifugation [9] at the Clinical and Translational Science Center (WCM) within 3 h of collection, and platelet counts were determined. PRP was prepared with an initial whole blood centrifugation at 1000 RPM for 14 min (25 °C, no brake), followed by removal of the PRP into new tubes. The PPP was then prepared from the PRP by centrifugation at 5000 RPM for 5 min (25 °C) in a microcentrifuge.
Platelet aggregometry
Platelet activation and aggregation were measured by optical lumi-aggregometry in a Chrono-Log model 700 4-channel Lumi-aggregometer. Aliquots of 270 μl PRP (250,000 platelets/μl) were used for aggregometry. Following equilibration and addition of 30 μl luminescence reagent (“Chrono-Lume”, Chrono-Log), baseline was adjusted using a 300 μl PPP sample as optical reference. PRP samples were stimulated with the following agonists: collagen (0.5 and 5 μg/ml), thrombin (1 U/ml), protease-activated receptor1 (PAR1)–activating hexapeptide (SFLLRN) (50 μM), epinephrine (0.5 and 5 μM), and adenosine diphosphate (ADP) (0.5 and 5 μM). Aggregation was observed for 5 min (7 min for collagen). Platelet aggregation and adenosine triphosphate (ATP) secretion parameters were calculated using AggroLink8 software (Chrono-Log). Aggregation and secretion parameters are reported as area under the curve (AUC) (percent times minute (%*min) for aggregation and nanomoles times minute (nanomoles*min) for ATP secretion), slope (rate of aggregation (percent per minute) or rate of secretion (nanomoles per minute)), and amplitude (percent of maximum aggregation and nanomoles for ATP secretion).
Fluorescence-activated cell sorter analysis
Antibodies against CD14 and CD41 were obtained from BD Biosciences (San Jose, CA), and CD62P (P-selectin)-PE and CD154 (CD40L)-Alexa Fluor 647 were from BioLegend (San Diego, CA).
Platelet-monocyte aggregates
For each fluorescence-activated cell sorter (FACS) sample, 100 μl anticoagulated whole blood was added to a FACS tube containing the appropriate antibody (2 μl each). Following incubation for 20 min at room temperature in the dark, erythrocytes were lysed using FACS lysis buffer (BD Biosciences). Cells were then washed with phosphate-buffered saline (PBS), resuspended in 500 μl PBS, and analyzed with a FACSCanto system using FACS Diva software (BD Biosciences). Platelet-monocyte aggregates are a measure of platelet activation in vivo. Cells were single-stained with anti-CD14-FITC and anti-CD41a-APC, and also double-stained with anti-CD14/CD41a. The percentage of platelet-monocyte aggregates was determined.
Platelet activation markers
For each FACS sample, 100 μl PRP was added to a FACS tube containing 5 μl anti-CD62P-PE or 2.5 μl anti-CD154-Alexa Fluor 647. Following incubation for 20 min at room temperature in the dark, platelets were fixed using 100 μl 2% paraformaldehyde. The cells were then washed and resuspended in PBS and analyzed with a FACSCanto system using FACS Diva software (BD Biosciences). FACS analysis identified the percentage of platelets that had been activated and expressed CD62P (P-selectin) or CD154 (CD40L) on their surface.
BD FACS 7-Color Setup Beads were used during cytometer setup. These beads are used by the software to adjust detector voltages and set up fluorescence compensation by correcting for spectral overlap or spillover.
Statistical analyses
The sample size for the study was based on previous research on epinephrine-induced platelet aggregation in patients with cardiovascular disease and normal controls [10], using a power level of 80% to detect a minimally significant difference between means in amplitude (%) of epinephrine-induced platelet aggregation of 10 with a standard deviation of 4.6, at an alpha level of 0.05, leading to a minimal group size of 4.
Intermittent hypoxemia as defined by ODI criteria was the independent variable of severity used for the analyses. Baseline sleep characteristics and platelet reactivity were compared for groups stratified by ODI index at a cutoff of 15 events per hour. Data were presented using median and interquartile ranges [IQR: 25th and 75th percentiles] and compared between ODI groups using the non-parametric Wilcoxon rank-sum test (also known as the Mann-Whitney U test). Given the known association between ODI and body mass index (BMI), blood pressure (BP), glucose levels, and high-density lipoprotein (HDL) cholesterol levels, we also explored associations after adjusting for these factors in a linear model. The primary predictor was ODI ≥ 15 (vs. ODI < 15) and the outcome was the association in question, after checking that the normality assumptions were sufficiently met using a quantile-quantile plot and the Shapiro-Wilk test. Both BMI and BP were chosen a priori as potential confounders since obesity and hypertension have been previously shown to be associated with increased platelet reactivity [11, 12]. Similarly, fasting glucose and HDL cholesterol were also chosen as potential confounders based on their association with platelet reactivity [13, 14]. All analyses are two-sided, with statistical significance determined at the 0.05 level. 95% confidence intervals reported where appropriate. Data analyses were performed in R Version 3.5.0 (R Core Team, Vienna, Austria).
Results
A total of 31 subjects (25 men and 6 women), free of underlying cardiovascular or metabolic disease or hypertension, and taking no medications or supplements for the 3 months preceding their enrollment, participated in the study. Subjects were classified in groups based on ODI levels that were recorded during the overnight polysomnography. Eight (26%) subjects had an ODI ≥ 15, and 23 subjects had an ODI < 15. All subjects in the elevated ODI group had OSA based on AHI criteria of over 5 events per hour. Subject characteristics and polysomnographic data are shown in Table 1. Subjects with elevated ODI (≥ 15) had a higher BMI, more apnea-hypopnea index (AHI) events per hour, a higher obstructive AHI, a lower percentage of lowest oxyhemoglobin saturation (lowest SaO2), and a higher percentage of sleep time at oxygen saturation less than 90% (% TST <90%), as expected. Higher platelet counts, higher fasting glucose levels, and lower levels of HDL cholesterol were also seen in the subjects with higher ODI levels.
Platelet activation in subjects with intermittent hypoxemia
When platelets were treated with the prothrombotic agonist epinephrine, a substantial decrease in platelet reactivity was observed in the significant hypoxemia group (ODI ≥ 15) as compared with the milder hypoxemia group (ODI < 15), as shown in Table 2. Platelets from more severe hypoxemic subjects, upon stimulation with epinephrine (5 μM), exhibited decreased aggregation with a median AUC of 31.80%*min [IQR 25.25, 55.82%*min] as compared with those with milder hypoxemia levels with a median AUC of 117.40%*min [IQR 54.40, 211.95%*min], reaching statistical significance (p = 0.01) as shown in Fig. 1a. Moreover, the median amplitude of aggregation in response to epinephrine was 9.00% [IQR 8.00, 14.50%] in subjects with higher ODI indices as compared with 51.00% [IQR 15.00, 71.50%] for subjects with lower indices (p = 0.01), and the median rate of platelet aggregation was 20.00%/min [IQR 16.00, 29.75%/min] for the ODI ≥ 15 group vs. 51.00%/min [IQR 27.00, 65.50%/min] for the ODI < 15 group (p = 0.02), as shown in Fig. 1b, c, respectively. In addition, subjects with significant intermittent hypoxemia displayed a decrease in platelet aggregation when their platelets were stimulated with the agonist thrombin (Table 2). The median AUC for platelet aggregation in response to thrombin (1 U/ml) was 382.65%*min [IQR 277.52, 432.09%*min] for subjects with an ODI ≥ 15 as compared with 443.25%*min [IQR 433.50, 491.17%*min] for subjects with an ODI < 15 (p = 0.02), and the median amplitude of aggregation was 95.00% [IQR 70.12, 98.00%] for the ODI ≥ 15 group as compared with 101.00% [IQR 98.50, 108.75%] for the ODI < 15 group (p = 0.02), as seen in Fig. 2a, b, respectively. For all of the other platelet agonists examined, differences in platelet responses were not statistically significant between ODI groups (Table 2).
Epinephrine-induced platelet aggregation in subjects with significant intermittent hypoxemia as compared with subjects with milder hypoxemia levels. Platelet aggregation in subjects with an ODI ≥ 15 as compared with subjects with an ODI < 15 in response to the agonist epinephrine (5 μM). a The area under the curve (AUC) of agonist-induced platelet aggregation; b the amplitude of the aggregation response; and c the rate of platelet aggregation. Data are presented as median with box indicating upper and lower quartiles, whiskers indicating extrema, and with p values calculated by the non-parametric Wilcoxon rank-sum test (Mann-Whitney U test)
Thrombin-induced platelet aggregation in subjects with significant intermittent hypoxemia as compared with subjects with milder hypoxemia levels. Platelet aggregation in subjects with an ODI ≥ 15 as compared with subjects with an ODI < 15 in response to the agonist thrombin (1 U/ml). a The area under the curve (AUC) of agonist-induced platelet aggregation and b the amplitude of the aggregation response. Data are presented as median with box indicating upper and lower quartiles, whiskers indicating extrema, and with p values calculated by the non-parametric Wilcoxon rank-sum test (Mann-Whitney U test)
We also examined platelets and whole blood for the expression of markers of platelet activation (CD154 (CD40L) and CD62P (P-selectin)) and the presence of platelet-monocyte aggregates, as shown in Table 3. OSA subjects with higher ODI indices exhibited a decrease in platelet surface expression of CD40L as compared with those with milder hypoxemia levels (ODI < 15). The median for CD40L-positive platelets was 13.30% [IQR 11.85, 16.65%] in the ODI ≥ 15 group vs. 18.50% [IQR 16.05, 25.95%] in the ODI < 15 group (p = 0.05). CD62P platelet surface expression had a tendency toward decreased expression in the high ODI group with a median 22.20% [IQR 16.30, 24.70%] vs. 26.60% [IQR 21.20, 32.75%] in the low ODI group; however, it did not reach statistical significance (p = 0.174). No differences were noted in platelet-monocyte aggregate levels, with a median 55.00% [IQR 30.90, 64.30%] in the elevated ODI group vs. 48.50% [IQR 40.20, 58.80%] in the low ODI group (p = 0.931).
Adjusted analyses
The association between ODI and platelet reactivity after adjusting for BMI, BP, fasting glucose, and HDL cholesterol in a linear regression model is shown in Table 4. In response to epinephrine, the amplitude and rate of platelet aggregation remained significant after adjustment for fasting glucose and HDL cholesterol (p = 0.036) or for BMI and BP (p = 0.037), respectively, however, not significant when adjusting for all four variables. Given that the quantile-quantile plots and Shapiro-Wilk test revealed that non-normality of the residuals was not detected, coefficient, confidence intervals, and p values were reported for these analyses. This was also the case for thrombin-induced platelet aggregation after adjusting for BMI and BP. However, after adjustment for fasting glucose and HDL cholesterol or for all 4 factors, non-normality was detected, and thus, only coefficient values were reported. While almost all associations maintained the same direction after adjustment, no others maintained statistical significance.
Discussion
Our study shows an increase in platelet activation in subjects with significant intermittent hypoxemia (ODI ≥ 15) free of underlying cardiovascular or metabolic disease. The ODI is a prime index of the severity of event-related nocturnal hypoxemia, which is recognized as a key contributor to OSA pathogenesis. Although studies have examined platelet activation and aggregability in subjects with OSA who have repetitive decreases in blood oxygenation [15,16,17,18,19,20], this is the first study to investigate platelet responsiveness (platelet aggregation and ATP secretion) to a broad panel of agonists as well as quantify markers of platelet activation and platelet-monocyte interactions in an otherwise disease-free population. This is a critical factor, as subjects in this study were not exposed to any type of medication nor had any preexisting cardiovascular disease or cardiovascular risk factors that might have influenced the platelet responses. Furthermore, our study design included the gold standard for evaluation of the presence of sleep apnea, overnight polysomnography, to directly quantify respiratory events, to rule out other sleep disorders, and confirm the presence of OSA instead of other causes of hypoxemia. Importantly, ODI was used to classify patients for this specific analysis because it provides a direct measure of intermittent hypoxemia.
When platelets from our study subjects were treated with the prothrombotic agonist epinephrine, a decrease in the platelet aggregation response was observed in the group with significant intermittent hypoxemia (ODI ≥ 15) as compared with those with milder hypoxemia levels (ODI < 15). The difference in the rate and amplitude of epinephrine-induced platelet aggregation remained significant after adjusting for BMI and BP or for fasting glucose and HDL cholesterol, respectively. One possible explanation for the observed decrease is desensitization of the epinephrine receptor on platelets, specifically the α2-adrenergic receptor, in response to prior epinephrine stimulation. Motulsky et al. [21] demonstrated that preincubation of unstirred platelets (from healthy individuals on no medications) with epinephrine blunts the ensuing aggregation response when the platelets are stirred. This desensitization of epinephrine-induced aggregation was shown to not be associated with any defect in overall platelet aggregation [21]. Moreover, Kjeldsen et al. [22] examined the effect of increased circulating epinephrine within lower pathophysiological concentrations on platelet function in healthy young men, and in vitro epinephrine-induced platelet aggregation was decreased. Importantly, ADP- and collagen-induced platelet aggregation responses were not affected. The investigators concluded that platelets guard themselves against overstimulation by downregulation of receptor-dependent responses and aggregation [22]. In response to hypoxemia and hypoxia, catecholamines (e.g., epinephrine and norepinephrine) are released directly into the circulation, activating peripheral adrenergic receptors with subsequent systemic effects. Several studies have shown persistent elevated levels of catecholamines in both the plasma and urine of OSA patients with intermittent nocturnal hypoxemia, and the level of epinephrine and norepinephrine correlated with polysomnography indices (Hakim et al., Review [23]). Moreover, Eisensehr et al. [20] showed that 6AM plasma epinephrine levels (after the polysomnogram) correlated positively and independently with the apnea-hypopnea index (AHI), a measure of the frequency of respiratory events during sleep, where the morning plasma epinephrine levels increased 2-fold in subjects with severe OSA (AHI ≥ 50/h) as compared with the mild-to-moderate group (AHI 5–50/h). Importantly, this would imply that our significant hypoxemic OSA group, which had a median AHI of 75.25 events/h, could also have elevated morning plasma epinephrine levels relative to the ODI < 15 group, which had a median AHI of 6.20 events/h (Table 1). Collectively, this suggests that increased morning epinephrine levels in the plasma of subjects with significant intermittent hypoxemia may have resulted in desensitization of the platelet α2-adrenergic receptor and a decrease in the aggregation response following our stimulation with epinephrine.
Thrombin is the most potent platelet agonist, activating human platelets via the receptors PAR1 and PAR4 [24]. We found that platelets from subjects with significant intermittent nocturnal hypoxemia exhibited a decrease in in vitro aggregation when stimulated with thrombin. One possible explanation for this observed decrease is that the platelets were desensitized to thrombin stimulation, due to prior platelet activation related to nocturnal vascular oxygen reduction in OSA subjects. Interestingly, patients with acute cerebral ischemia, a condition characterized by insufficient blood flow to the brain leading to poor vascular oxygen supply and increased platelet activation, showed similar changes in platelet responses to thrombin. Jurk et al. [25] examined the role of thrombin in platelet activation in patients with acute ischemic stroke. In contrast to healthy controls, platelets from ischemic stroke patients were found to be refractory to thrombin in vitro. Importantly, this was not the case when their platelets were induced with collagen and the PAR1-activating peptide SFLLRN. This suggested that thrombin desensitization results from increased quantities of thrombin generated in acute ischemic stroke patients. Thus, platelets from these stroke patients were activated in response to prior thrombin stimulation in vivo and therefore desensitized when presented with thrombin in vitro. Notably, similar to platelets from acute ischemic stroke patients, platelets from significant hypoxemia OSA subjects displayed reduced thrombin sensitivity without changes in sensitivity to collagen and the PAR1-activating peptide. Therefore, it is possible that the intermittent hypoxemia in OSA subjects might be creating an environment in the circulation that is similar to that in ischemic stroke patients. Significantly, our study is the first study to report changes in epinephrine- and thrombin-induced platelet aggregation responses in otherwise healthy subjects with significant intermittent hypoxemia.
In contrast to our findings with epinephrine and thrombin, none of the other platelet agonists tested (collagen, ADP, and PAR1-activating hexapeptide) induced platelet responses that were statistically different between the ODI groups examined (Table 2). As aforementioned, studies have reported that increased in vivo epinephrine and thrombin levels resulted in desensitization to subsequent in vitro epinephrine- and thrombin-induced platelet aggregation, respectively, yet no changes in in vitro sensitivity of platelets to collagen-, ADP-, and PAR1 hexapeptide–induced aggregation [22, 25]. The lack of observed significant differences in platelet responses to collagen, ADP, and PAR1 hexapeptide may also be due to the sample size or the chosen ODI cutoff as these subjects could have different sensitivities and responses to intermittent hypoxemia.
To further investigate the role of intermittent hypoxemia on platelet activation, we determined the expression levels of activation markers CD40 ligand (CD40L) and P-selectin (CD62P) on the platelet surface, and the presence of circulating platelet-monocyte aggregates in the whole blood of these individuals. CD62P platelet surface expression and platelet-monocyte aggregate levels were not significantly different between the ODI groups. However, a decrease in platelet surface expression of CD40L was observed in OSA subjects with higher indices of intermittent hypoxemia (ODI ≥ 15), indicating an increase in platelet activation in these individuals. CD40L is normally present within the platelet granules when platelets are not activated, but upon platelet activation, CD40L is rapidly secreted from the platelet α-granules to the cell surface [24, 26]. Surface-expressed, platelet-bound CD40L is then cleaved within minutes to hours of platelet stimulation and released as soluble CD40L (sCD40L) [26]. Since activated platelets shed surface-expressed CD40L, our observed decrease in platelet surface expression of CD40L in OSA subjects with ODI ≥ 15 is indicative of increased platelet activation in these individuals. Importantly, our observations are consistent with platelets being in an activated state in significant hypoxemic OSA individuals and their platelets follow the normal course of events of activation marker shedding. Studies have shown that plasma soluble CD40L is significantly elevated in moderate-to-severe OSA patients as compared with subjects with mild OSA or controls [18, 19]. Plasma sCD40L levels have also been shown to be higher in individuals with cardiovascular disease [27,28,29]. Moreover, sCD40L levels have been linked to increased cardiovascular risk in healthy women [29].
Although the current study validated our main hypothesis, our study has a few limitations. The subject sample size was small and thus, our ability to detect associations was limited, especially in multivariate models. Multivariate analysis models were restricted to adjustment for BP, BMI, fasting glucose, and HDL cholesterol as potential confounders based on their association with platelet reactivity [11,12,13,14]. The fact that some of the adjusted analyses did not reveal significant associations in this small study does not equate for lack of relationships present. In addition, the direction of the relationship with the adjusted potential confounding variables and platelet reactivity might not be the same. HDL cholesterol might act as a potential protective factor while the other variables would not. Furthermore, our findings may not be fully generalizable to all subjects with intermittent hypoxemia and OSA as our subject population was free from underlying cardiovascular disease, hypertension, medication intake, or chronic diseases. For example, prevalence for hypertension in OSA patients is significant; therefore, exclusion based on hypertension may have introduced some bias into our study. However, differentiating the adverse effect of intermittent hypoxemia on platelets unrelated to hypertension or other cardiovascular disease was the unique perspective of this study, as hypertension likely is a secondary factor developing in OSA patients. In addition, the contribution of platelet dysfunction to cardiovascular disease in these patients is still unclear. Another important limitation included the use of a 4% oxygen desaturation threshold to define ODI in this study, which is higher than the 3% threshold recommended by the American Academy of Sleep Medicine. Given the fact that there is no current consensus around this choice [30], the 4% selection was utilized in order to be more selective of hypoxemic events and put in evidence the differences between groups. The use of this conservative criterion may have affected the results.
In conclusion, our study evaluated platelet reactivity in otherwise healthy subjects with intermittent hypoxemia secondary to OSA, and in controls, to identify potential determinants underlying the high incidence of occlusive vascular events in OSA. Importantly, a correlation between increased platelet activation and the severity of intermittent hypoxemia was observed, suggesting that platelet activation and response is affected by intermittent hypoxemia at night in individuals free of cardiovascular or metabolic disease. This would favor a prothrombotic milieu in the vasculature of subjects with intermittent hypoxemia during sleep, which could contribute to the development of cardiovascular disease in OSA. Thus, approaches to reduce platelet activation deserve further investigation to determine the effectiveness in attenuating undesired cardiovascular outcomes in this population.
References
Ayas NT, Taylor CM, Laher I (2016) Cardiovascular consequences of obstructive sleep apnea. Curr Opin Cardiol 31:599–605. https://doi.org/10.1097/HCO.0000000000000329
Bauters F, Rietzschel ER, Hertegonne KB, Chirinos JA (2016) The link between obstructive sleep apnea and cardiovascular disease. Curr Atheroscler Rep 18:1–11. https://doi.org/10.1007/s11883-015-0556-z
Barone DA, Krieger AC (2013) Stroke and obstructive sleep apnea: a review. Curr Atheroscler Rep 15:334–340. https://doi.org/10.1007/s11883-013-0334-8
Hoyos CM, Melehan KL, Liu PY, Grunstein RR, Phillips CL (2015) Does obstructive sleep apnea cause endothelial dysfunction? A critical review of the literature. Sleep Med Rev 20:15–26. https://doi.org/10.1016/j.smrv.2014.06.003
Gabryelska A, Lukasik ZM, Makowska JS, Bialasiewicz P (2018) Obstructive sleep apnea: from intermittent hypoxia to cardiovascular complications via blood platelets. Front Neurol 9:635–644. https://doi.org/10.3389/fneur.2018.00635
Dewan NA, Nieto FJ, Somers VK (2015) Intermittent hypoxemia and OSA: implications for comorbidities. Chest 147:266–274. https://doi.org/10.1378/chest.14-0500
Iber C, Ancoli-Israel S, Chesson AL, Quan SF (2007) The AASM manual for the scoring of sleep and associated events: rules, terminology and technical specifications, 1st edn. American Academy of Sleep Medicine, Westchester
Punjabi NM, Newman AB, Young TB, Resnick HE, Sanders MH (2008) Sleep-disordered breathing and cardiovascular disease: an outcome-based definition of hypopneas. Am J Respir Crit Care Med 177:1150–1155. https://doi.org/10.1164/rccm.200712-1884OC
Drosopoulos JHF, Broekman MJ, Islam N, Maliszewski CR, Gayle RB, Marcus AJ (2000) Site-directed mutagenesis of human endothelial cell ecto-ADPase/soluble CD39. Requirement of glutamate174 and serine218 for enzyme activity and inhibition of platelet recruitment. Biochemistry 39:6936–6943. https://doi.org/10.1021/bi992581e
Furman MI, Benoit SE, Barnard MR, Valeri CR, Borbone ML, Becker RC, Hechtman HB, Michelson AD (1998) Increased platelet reactivity and circulating monocyte-platelet aggregates in patients with stable coronary artery disease. J Am Coll Cardiol 31:352–358
Ranucci M, Aloisio T, Di Dedda U et al (2019) Platelet reactivity in overweight and obese patients undergoing cardiac surgery. Platelets 30:608–614. https://doi.org/10.1080/09537104.2018.1492108
El Haouari M, Rosado JA (2009) Platelet function in hypertension. Blood Cells Mol Dis 42:38–43. https://doi.org/10.1016/j.bcmd.2008.07.003
Sudic D, Razmara M, Forslund M, Ji Q, Hjemdahl P, Li N (2006) High glucose levels enhance platelet activation: involvement of multiple mechanisms. Br J Haematol 133:315–322. https://doi.org/10.1111/j.1365-2141.2006.06012.x
Van der Stoep M, Korporaal SJ, Van EM (2014) High-density lipoprotein as a modulator of platelet and coagulation responses. Cardiovasc Res 103:362–371. https://doi.org/10.1093/cvr/cvu137
Alkhiary W, Morsy NE, Yousef AM, El-Saddik AM, Arram EO (2017) Adenosine diphosphate-induced platelets: Aggregability in polysomnographically verified obstructive sleep apnea. Clin Appl Thromb Hemost 23:360–366. https://doi.org/10.1177/1076029615600790
Kondo Y, Kuwahira I, Shimizu M et al (2011) Significant relationship between platelet activation and apnea-hypopnea index in patients with obstructive sleep apnea syndrome. Tokai J Exp Clin Med 36:79–83
Oga T, Chin K, Tabuchi A, Kawato M, Morimoto T, Takahashi K, Handa T, Takahashi K, Taniguchi R, Kondo H, Mishima M, Kita T, Horiuchi H (2009) Effects of obstructive sleep apnea with intermittent hypoxia on platelet aggregability. J Atheroscler Thromb 16:862–869. https://doi.org/10.5551/jat.2188
Akinnusi ME, Paasch LL, Szarpa KR, Wallace PK, El Solh AA (2009) Impact of nasal continuous positive airway pressure therapy on markers of platelet activation in patients with obstructive sleep apnea. Respiration 77:25–31. https://doi.org/10.1159/000158488
Kobayashi K, Nishimura Y, Shimada T et al (2006) Effect of continuous positive airway pressure on soluble CD40 ligand in patients with obstructive sleep apnea syndrome. Chest 129:632–637. https://doi.org/10.1378/chest.129.3.632
Eisensehr I, Ehrenberg BL, Noachtar S, Korbett K, Byrne A, McAuley A, Palabrica T (1998) Platelet activation, epinephrine, and blood pressure in obstructive sleep apnea syndrome. Neurology 51:188–195
Motulsky HJ, Shattil SJ, Ferry N, Rozansky D, Insel PA (1986) Desensitization of epinephrine-initiated platelet aggregation does not alter binding to the alpha 2-adrenergic receptor or receptor coupling to adenylate cyclase. Mol Pharmacol 29:1–6
Kjeldsen SE, Weder AB, Egan B, Neubig R, Zweifler AJ, Julius S (1995) Effect of circulating epinephrine on platelet function and hematocrit. Hypertension 25:1096–1105. https://doi.org/10.1161/01.HYP.25.5.1096
Hakim F, Gozal D, Kheirandish-Gozal L (2012) Sympathetic and catecholaminergic alterations in sleep apnea with particular emphasis on children. Front Neurol 3:1–13. https://doi.org/10.3389/fneur.2012.00007
Jennings LK (2009) Mechanisms of platelet activation: need for new strategies to protect against platelet-mediated atherothrombosis. Thromb Haemost 102:248–257. https://doi.org/10.1160/TH09-03-0192
Jurk K, Jahn UR, Van Aken H et al (2004) Platelets in patients with acute ischemic stroke are exhausted and refractory to thrombin, due to cleavage of the seven-transmembrane thrombin receptor (PAR-1). Thromb Haemost 91:334–344. https://doi.org/10.1160/TH03-01-0044
Henn V, Steinbach S, Büchner K, Presek P, Kroczek RA (2001) The inflammatory action of CD40 ligand (CD154) expressed on activated human platelets is temporally limited by coexpressed CD40. Blood 98:1047–1054. https://doi.org/10.1182/blood.V98.4.1047
Antoniades C, Bakogiannis C, Tousoulis D, Antonopoulos AS, Stefanadis C (2009) The CD40/CD40 ligand system: linking inflammation with atherothrombosis. J Am Coll Cardiol 54:669–677. https://doi.org/10.1016/j.jacc.2009.03.076
Varo N, de Lemos JA, Libby P, Morrow DA, Murphy SA, Nuzzo R, Gibson CM, Cannon CP, Braunwald E, Schönbeck U (2003) Soluble CD40L: risk prediction after acute coronary syndromes. Circulation 108:1049–1052. https://doi.org/10.1161/01.CIR.0000088521.04017.13
Schonbeck U, Varo N, Libby P, Buring J, Ridker PM (2001) Soluble CD40L and cardiovascular risk in women. Circulation 104:2266–2268. https://doi.org/10.1161/hc4401.099447
Berry RB, Budhiraja R, Gottlieb DJ et al (2012) Rules for scoring respiratory events in sleep: update of the 2007 AASM manual for the scoring of sleep and associated events. Deliberations of the sleep apnea definitions task force of the American Academy of Sleep Medicine. J Clin Sleep Med 8:597–619. https://doi.org/10.5664/jcsm.2172
Acknowledgments
This work is dedicated in memory of our colleague and mentor Dr. Aaron J. Marcus, an exceptionally talented scientist and expert in thrombosis and platelet dysfunction, who made enormous contributions to the field of Hematology and was continuously funded by the National Institutes of Health for over 50 years.
Funding
This work was supported by National Institutes of Health grants HL089521 (A.J.M.) and HL047073 (A.J.M., J.H.F.D.), a Merit Review grant I01BX002122 from the United States Department of Veterans Affairs Biomedical Laboratory Research and Development Program (A.J.M., J.H.F.D.), and a National Heart Lung and Blood Institute grant K23 HL094358 (A.C.K.). We also received support from the Clinical Translational Research Center at Rockefeller University Hospital, grant UL1 TR001866 from the National Center for Advancing Translational Sciences, National Institutes of Health and Clinical and Translational Science Award programs, and grant UL1 TR000457-06 from the Clinical and Translational Science Center at Weill Cornell Medicine.
Author information
Authors and Affiliations
Author notes
Aaron J. Marcus is deceased. This paper is dedicated to his memory.
- Aaron J. Marcus
Corresponding author
Ethics declarations
Conflict of interest
The authors declare that they have no conflict of interest.
Ethical approval
All procedures performed in studies involving human participants were in accordance with the ethical standards of the institutional and/or national research committee and with the 1964 Helsinki Declaration and its later amendments or comparable ethical standards.
Informed consent
Informed consent was obtained from all individual participants included in the study.
Additional information
Publisher’s note
Springer Nature remains neutral with regard to jurisdictional claims in published maps and institutional affiliations.
Rights and permissions
About this article
Cite this article
Krieger, A.C., Anand, R., Hernandez-Rosa, E. et al. Increased platelet activation in sleep apnea subjects with intermittent hypoxemia. Sleep Breath 24, 1537–1547 (2020). https://doi.org/10.1007/s11325-020-02021-4
Received:
Revised:
Accepted:
Published:
Issue Date:
DOI: https://doi.org/10.1007/s11325-020-02021-4