Abstract
Firstly, foam trays were produced from glyoxal cross-linked wheat, potato and corn starches and their mixtures. The most suitable starch type for starch-based foam tray production was selected according to the level of water absorption, density, surface and cross-section micrographs of the foam trays. It was decided that a wheat and potato starch blend was the most suitable starch source for producing the foam trays because they have the lowest water absorption percentage (25.5 ± 0.7%), low density (0.17 ± 0.01 g/cm3) and a smooth surface. Potato–wheat starch foam trays with fibres were produced by adding wheat and wood fibres. Unlike wood fibres addition, wheat fibres significantly decreased the percentage of water absorption (16.63 ± 1.2%) and density (0.115 ± 0.013 g/cm3) of the tray. Also, the trays including wheat fibre had a lighter colour than the wheat–potato starch tray. To further reduce water absorption of the tray, the trays were made by adding two different types of lipids (beeswax or shortening and three different types of filler materials—kaolin, montmorillonite or zinc oxide nanoparticles). According to the level of water absorption of the trays, it was decided that shortening and zinc oxide nanoparticles, in addition to kaolin, were respectively the most suitable lipid and filler materials. The foam trays were produced by adding these supplementary materials. The addition of shortening slightly, zinc oxide nanoparticles moderately and kaolin greatly increased the density of the wheat potato starch tray including fibre. However, the percent of water absorption of the trays containing wheat fibre + shortening or wheat fibre + shortening + zinc oxide nanoparticles decreased 6.4 ± 0.01 and 5.9 ± 0.3%, respectively.
Similar content being viewed by others
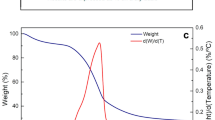
Explore related subjects
Discover the latest articles, news and stories from top researchers in related subjects.Avoid common mistakes on your manuscript.
Introduction
The polystyrene-based foam trays are used in the packaging of many foods. Nevertheless, they are not biodegradable and are difficult to recycle. Thus, much research has been done to solve this problem and has demonstrated that biopolymers are one of the possible alternative materials for making biodegradable foam [1–3], while the high production cost is still a major problem in comparison to the conventional petroleum-based foams.
Starch is considered as a potential polymer to be used in biodegradable materials because of its low cost, availability, and production from renewable resources. Corn, wheat, potato and tapioca are the main sources of starch. Starches are used mainly in the food and paper industries, with 57% of produced starch consumed in the food industries and 43% in the nonfood sector [4]. Cassava starch [5–8, 17] wheat starch [9–11] corn starch [10] and potato starch [12] were used in the production of starch-based foam trays. According to previous articles, foams made from different starch sources remain sensitive to water, and their water absorption capacities are different from each other; thus, further improvement in their water resistance is necessary for commercial applications.
Numerous research groups have tried to improve the properties of starch foam. It was suggested that cross-linking the starch is one potential way to produce water-resistant material because cross-linking considerably reduces the swelling power, solubility, water-binding capacity, and hydrophilicity of starch [13, 14]. A previous study has pointed out that cross-linking starch with glyoxal considerably reduces the density and water absorption of starch foams [15]. Many researchers showed that the use of different types of fibres such as kraft fibres [7], jute and flax fibres [16], cassava and wheat fibres [17], corn [12], cellulose [5], and corn husk fibres [18] improved the properties of the starch based foams. Also, hydrophobic additives such as latex [11], polycarpolactone [19], beeswax [18], and palm oil [8] have been used to improve the properties of starch foam. Moreover, most intensive studies are currently focused on layered silicates, such as montmorillonite [6, 20], and kaolin (a kind of mineral) [18, 20]. It was reported that the use of kaolin in starch products led to the formation of a labyrinthine structure and blocked the water molecules from being absorbed [20]. The use of nanoparticles in nanocomposite packages is a relatively new research area. Nanoparticles are used as filler material in the production of food packaging material. The potential uses of nanoparticles for the production of starch-based foam and the nanocomposite foam features produced from nanoparticles of zinc oxide (ZnO) were explained in the present paper.
In this study, firstly, the most suitable starch (glyoxal cross-linked) source was determined for a starch foam, then further development of the physical properties of the starch foam was attempted by adding fibers (wood or wheat fiber), lipids (beeswax or shortening (anhydrous hydrogenated margarine)) and filler materials (kaolin, zinc oxide nanoparticles).
Materials and Methods
Unmodified corn, potato and wheat starches were purchased from Cargill Turk (İstanbul, Turkey), Avebe Co. (İzmir Turkey) and Smart Co. (İzmir, Turkey) respectively. Glyoxal solution (40%) was gained from BASF Turk Chemical Co. İstanbul. Both wood (sized between 20 and 300 µm), and bamboo fibre (120 and 500 µm) were obtained from Smart Co. (İzmir, Turkey) and wheat fibre (60 and 550 µm) was obtained from Vitacel (Rosenberg, Germany). Nano-sized metal oxides (SiO2: 15 nm; ZnO: 50–150 nm) and nanoclay (montmorillonite) were purchased from MK Impex Co. (Missisauga, Canada) and Nanokil Co. (Antalya, Turkey) respectively. Kaolin (42.16% SiO2, 39.35% Al2O3, 1.10% Fe2O3 and other minor elements) was supplied by Kalemaden (Canakkale, Turkey). Guar gum and shortening were obtained from Incom Co. (Mersin, Turkey) and Marsan Co. (Istanbul, Turkey) respectively. Beeswax was bought from a local producer in Antalya (Turkey) and purified before use by melting and filtering it through a filter paper in a drying oven at 80 °C. Other chemicals were supplied by Sigma–Aldrich.
The Foam Tray Production Machine with Changeable Molds
The machine with changeable moulds was designed together with Kahramanlar Machine Co. (Bucak, Burdur, Turkey) and manufactured by the company. The stainless steel (code: 2316) moulds were produced by Cetin Mold (Istanbul, Turkey).
The machine had a fixed lower mould and a movable upper mould. The upper mould of the machine could move vertically on two endless screw system which are driven by two motors (1.5 HP). To heat the moulds, electrical resistances (1500 W) were placed under the lower mould and over the upper mould. Thermocouples were placed into both of the moulds to measure temperatures of the moulds. A proportional-integral-derivative controller (PID controller) system was integrated into the machine to control tray thickness, baking time and temperature.
Cross-Linkage with Glyoxal
The starches (corn, wheat, potato and their double and triple mixtures) were cross-linked with glyoxal according to Uslu and Polat [15]. For cross-linkage 0.13 g/kg of glyoxal was added into 40% starch suspensions. The suspensions were continuously stirred (300 rpm) for 24 h at 25 °C. After the cross-linkage completed, the suspensions were filtered, washed with distilled water three times and dried at 50 °C for 24 h. The dried starches were ground with a hammer mill involving a sieve with 250 μm pore size.
Production of Foam Trays
To obtain 35% starch water suspension, 42 g of cross-linked starch or starch mixture was weighed in a beaker, and 78 g of distilled water added. Then the suspension was homogenised with homogeniser (Ultraturrax T-25, IKA Labortechnik, Staufen, Germany) at 10,000 rpm for 5 min. During homogenisation, guar gum (0.5% by weight of starch) was added to prevent the starch from settling. Then the suspension was baked, and the foam trays (12 × 20 cm) were produced. The production conditions were determined by preliminary tests [15]. The amounts of cross-linked starch type in the production of foam trays along with abbreviations of the tray samples are given in Table 1.
To produce trays containing fibres, wheat and wood fibres were added separately to the water-starch suspensions in an amount of 7% of the starch. To produce trays containing fibres and lipids, beeswax or shortening (10% of the starch) was melted and added to hot water (80 °C), including guar gum. The mixture was homogenised at 10,000 rpm (Ultraturrax T-25, IKA Labortechnik, Staufen, Germany) until the temperature of the mixture decreased to 58 °C. Then, starch and if necessary, other ingredients, were added, and the mixture was homogenised before baking. Zinc oxide (ZnO) nanoparticles and kaolin were added as filler materials to the suspensions in an amount of 1% of the starch. The proportion of supplementary materials used in final tray formulations and abbreviations of tray samples are given in Table 2. Before the analysis, the trays or the samples prepared from the trays were conditioned (25 °C, 50 ± 3% R.H.) for three days.
Density and Percent Moisture Content
To determine the density of the trays, a rectangular prism shaped piece was cut, the volume of the pieces was determined by measuring the dimensions. Then the density was calculated by using the weight and volume relation.
Tray pieces weighing approximately 4 g were placed in Petri dishes and dried at 100 °C for 24 h. For calculation of the initial moisture content, the difference of original and dried weight of pieces was divided by the original weight.
Scanning Electron Microscopy
The surface and cross section of the trays were examinated with scanning electron microscope (ZEISS Leo 1430 SEM, Oberkochen, Germany). About 1x1 cm dimensions of pieces were cut from the trays, coated under vacuum pressure with a thin layer of gold/palladium alloy by using coating apparatus (Polaron SC7620, Quorem Technologies, UK). Then the pieces were monitored with scanning electron microscope.
Colour
The CIE Lab colour values of the trays were measured by a CR-400 Minolta Chromameter (Minolta, Osaka, Japan). Using the colour values, the total colour difference (ΔE) was calculated as:
where L2*, a2* and b2* are the values of the sample, L1*(67.67 ± 0.02), a1*(−0.22 ± 0.06) and b1*(7.60 ± 0.68) are the values of the control tray (PW) [15].
Percent Water Absorption
The water absorption content of the trays was determined modifying the method described by Salgado et al. [5]. Absorbed water by the trays was calculated from the percentage of weight gain after completely sinking the trays in 1000 mL of distilled water and waiting for 1 min at 25 °C.
Thermal Specifications of the Starches
Unmodified and modified starches were weighed approximately 9 mg and compressed to form pellets in aluminum containers which has 6 mm of diameter and 1 mm of height. The trays were grinded and weighed just before forming pellets. An empty container was used as reference. Then the thermal characteristic of the starches and the trays were determined using differential scanning calorimeter (DSC) (Perkin-Elmer DSC 6000 equipped with a Pyris software, Perkin-Elmer Inc., Wellesley, USA) both samples and references were heated at a rate of10 °C/min from −15 to 250 °C and cooled to −15 °C by using nitrogen flushing (20 mL/min) [18].
Mechanical Properties
Flexural test was performed according to EN ISO 178: 2003 [21] to measure the mechanical properties of the trays. The tests were conducted by using TA.XT plus (SMS, Surrey, UK) equipped with a three-point bending rig and a span setting of 3 cm. The tray pieces (25 × 100 mm) were deformed until they broke. During the test, a 50 kg load cell was used and the pre-test speed, test speed and posttest speed were 2, 0.5 and 5 mm/s also the trigger force was 1 g during the mechanical tests. The flexural strength (S f), strain to breakage (e b) and modulus of elasticity (E b) of the strips were calculated.
Glyoxal Migration
A migration test was performed for two trays (cross-linked starch + wheat fibre + hydrogenated margarine and cross-linked starch + wheat fibre + ZnO + hydrogenated margarine) that showed better properties than the other trays. For the migration test, 200 mL of distilled water was put into the trays and glyoxal migration from the trays to water was monitored by determining the free glyoxal amount in the water.
Glyoxal Determination
The consumed glyoxal amount during cross-linkage and glyoxal migration from the trays to water were determined by modifying a previous method [22]. In the method, free glyoxal reacted with orto-phenylendiamin (o-PDA) and synthethised quinoxaline. The amount of quinoxaline was determined by measuring the absorbance of the compound at 315 nm with a UV–Vis spectrophotometer.
To determine the free amount of glyoxal that remained in the suspension, 10 mL aliquot was taken from starch suspension containing glyoxal (0.13 g/kg−1) at 0, 2, 4, 6, 8 and 24 h of cross-linking process. For the migration test, 1.5 mL of aliquot was taken after 0.167, 2, 4, 8 and 24 h. For cross-linking, after filtration and diluted ten times, for a migration test without any dilution, a 1 ml sample from each solution was taken and treated with 1 mL of 1.03 × 10−3 molar o-PDA in 80% acetic acid at 50 °C for 10 min. Then, 8 mL of 80% acetic acid was added to each test tube and vortexed. The absorbance of the solution was measured at 315 nm with a UV–Vis spectrophotometer (Shimadzu UV 1800, Kyoto, Japan). The amount of unreacted glyoxal in the suspension during the cross-linking process and the amount of glyoxal migrated from the trays were calculated from a calibration curve. The calibration curve was prepared from the standard glyoxal solutions (3.44 × 10−4, 6.89 × 10−4, 1.03 × 10−3 and 1.38 × 10−3 molar).
Statistical Analysis
Tray productions were done at least in duplicate, and 10 specimens were tested in density, percent moisture content, colour measurement, percent water absorption and a mechanical test. However, two samples were used for SEM monitoring, thermal analysis and migration test. All statistical analyses were conducted using the SAS System for Windows v7.00 (SAS Institute, Cary, NC, USA). The values are presented as mean ± standard deviation. The data were subjected to analysis of variance, and significant differences were determined by Duncan’s multiple range test.
Result and Discussion
In a previous study, it was mentioned that glyoxal cross-linkage of starch develops physical properties of the starch based foams [15], but there was no information about the progress of the cross-linking reaction. The time that is sufficient for cross-linkage of starch with glyoxal was determined by monitoring unreacted glyoxal content of the starch suspension containing glyoxal (Fig. 1). The free glyoxal concentration decreased exponentially during the cross-linkage reaction; 47.3 and 59.8% of the glyoxal added at the beginning of the reaction was consumed in the first 2 and 24 h, respectively. Because of the exponential decline in the free glyoxal amount, it was found that 24 h was adequate for completion of cross-linking. At the end of the 24 h, 0.076 g/kg out of 0.13 g/kg glyoxal reacted with the starch.
Determination of Most Suitable Starch Source
In other studies, it was found that the physical properties of the starch-based foam plates/trays were affected by the starch source [23, 24]. To determine the most suitable starch source for production of a starch-based foam tray, foam trays were produced from cross-linked corn, potato, wheat starches and their mixtures. It was decided that the most suitable starch source for the production of foam trays was a wheat-potato starch mixture, by investigating the amount of water absorption and density (Table 3). In fact, the PW tray had the lowest percent water absorption (25.5%) and density (0.17 ± 0.01%), indicating that the tray was the most resistant to water and the lightest product. The main reasons restricting the commercial use of starch-based foam trays are that the trays are not sufficiently resistant to water and have higher densities compared to petroleum based foam trays. Although PW trays had a lower density and higher water resistance than other trays, the water resistance properties of the wheat–potato starch trays (PW) still need to be developed for commercial usage.
The distance between the moulds was set at 2 mm, but arising water vapour pressure during baking lifted the upper mould, so the thickness of trays was higher than 2 mm. The starch type affected the lifting amount of the upper mould and also, densities of the trays. The trays made from wheat and wheat–potato starches had the lowest density values (Table 3). The density of the starch foam depends on the source [11], concentration, amylose content of the starch [2] and concentrations of other ingredients [10]. Also, it was considered that a different gelatinisation temperature and water-binding capacities of starches during gelatinisation affected rising water vapour pressure, and also the densities of the trays. A low density is ideal for foam because of the reduced cost and lighter product.
Selection of Supplementary Materials
Many studies reported that fibre addition helped to develop the foam trays’ properties. Also, Bénézet et al. [25] reported that the source of fibre could affect the water absorption of starch-based trays. Therefore, it was decided to use wood and wheat fibres in the tray production. For the selection of the proper fibre type, the water absorption of the trays was determined. Wood fibre addition increased but wheat fibre addition significantly reduced the water absorption of the cross-linked wheat–potato starch trays. Thus, it was decided to add 7% of wheat fibre to all tray formulations to increase the water resistance of the trays.
To further improve the water resistance of the trays, shortening or beeswax was added at the level of 5 or 10% to the formulation of the trays containing 35% starch and 7% wheat fibre. It was seen that the addition of 10% shortening significantly (p < 0.01) reduced the percent water absorption of the tray (6.41 ± 0.01%), but the addition of 5% shortening did not affect the percentage of water absorption. Moreover, the addition of 5 or 10% beeswax moderately reduced the percentage of water absorption (10.39 and 9.32 %, respectively).
Also nanoclay (montmorillonite), zinc oxide (ZnO) nanoparticles, and kaolin (at the amount of 1 or 5%) were tried to decrease the percent water absorption of the tray composed of starch and fibre. The addition of nanoclay caused cracks on the surface of the tray, and so it increased the water absorption of the trays. However, ZnO nanoparticles or kaolin addition reduced the percent water absorptions of trays. In addition, the percent water absorption of the trays, including ZnO nanoparticles or kaolin were not affected significantly (p > 0.05) from the added amount of these filler material. So, the trays were produced by adding 1% ZnO nanoparticles or kaolin for other tests (Table 2).
General Properties of Trays
The baking temperature, baking time, thickness, density and percent moisture content of the trays containing supplementary materials are given in Table 4. The baking time and temperatures of cross-linked wheat and potato starch-based trays differed according to the formulation.
The fibre addition to cross-linked wheat–potato starch increased the baking temperature from 190 to 205 °C because there were insufficient pores to form the foam structure when the trays containing fibre were baked at 190 °C. Moreover, the fibre addition increased the baking time of the trays. It was thought that the increase was due to the high water absorption properties of the fibres. (In the preliminary study, it was found that the wheat fibre absorbed 588% water.) The addition of kaolin to the tray containing fibre did not cause any difference in the baking time, but the addition of shortening and ZnO nanoparticles shortened the baking time by 30 and 90 s, respectively. The decrease was thought to be a result of the hydrophobic effects of shortening and the filler effects of the nanoparticles.
It was found that the addition of supplementary materials significantly increased the thickness of the trays. In general, the thickness and density of the trays are inversely proportional, and the density of the trays decreased when the foam structure increased. The F tray had the lowest density (0.115 ± 0.013 g/cm3) and the greatest thickness (7.11 ± 0.09 mm). In other words, the addition of 7% fibre to the tray formulation decreased the density by 32.4%. But a similar work found that the addition of cassava or wheat fibre increased the density of cassava starch foams [17]. However, the addition of kaolin increased the densities of the foam trays considerably, and the addition of shortening and ZnO nanoparticles did so slightly. One of the disadvantages of the starch-based foam trays compared to the polystyrene foam trays is the higher density of the trays. This disadvantage could be partially eliminated by fibre addition. Similarly, Bénézet et al. [25] showed that the density of expanded starch products decreased from 0.236 to 0.175 when cotton linter fibres were used, and to 0.191 when cellulose fibres were used. Also, it was reported that the addition of kraft fibre and sugarcane bagasse fibre reduced the density of cassava starch-based trays [7, 26]. In another study it was found that the density of starch foams was not affected by the addition of 10% kaolin [18]. It was thought that the difference between tray densities was the result of the bulk density of the powder form ingredients. The bulk densities of wheat fibre (0.09 g/cm3) and ZnO nanoparticles (0.35 g/cm3) were found to be lower, but the bulk density of kaolin (2.62 g/cm3) was higher than that of cross-linked wheat–potato starch (0.62 g/cm3).
Morphology of Trays
The colour parameters of starch foams were significantly influenced by the additives, but in different ways (Table 5).
The degree of lightness (L*) and the degree of yellowness (+b*) of foam trays containing additives were significantly higher than that of PW trays. Except for the FNS tray, a* values of the trays containing supplementary materials were not very different from PW trays. It was reported that the colour of the trays can vary depending on the colour of the additives [7, 18].
The surface and cross-section images obtained by SEM are presented in Figs. 2 and 3, respectively. In Fig. 2, it can be seen that unlike the other trays there are some small cracks on the surface of the trays produced using cross-linked wheat–potato starch and wheat fibre (F). Also, there are some pores on the surface of the tray produced using kaolin (FK). It was thought that these cracks and pores caused the water absorption to be higher. The section images (Fig. 3) showed that the F tray with the lowest density had a thicker, tidier, and larger pore structure. The trays including shortening and/or kaolin (FS, FK, FNS, FKS) had an irregular morphology and smaller pore size. The hydrophobic nature of shortening and large particle size of kaolin (average particle size was 45 µm) may have prevented interaction of these molecules with starch, and this might be cause of the formation of smaller pore size and irregular morphology.
Water Absorption
As can be seen in Fig. 4, it was determined that all supplementary materials decreased the water absorption significantly.
Although wheat fibres absorb up water to about six times its own weight, their addition decreased 34.7% of the water absorption percentage of the PW tray, probably by entering and strengthening the cell wall of the foam structure, as seen in SEM images. The result was in agreement with the literature [8, 18, 27]. Also, the separation or combined addition of ZnO nanoparticles, kaolin, and shortening decreased the water absorption of the F tray even further. FS and FNS trays absorbed the least water compared to all the other trays, and they absorbed 74.9 and 77.1% less water than the PW tray, respectively. Also, the percent moisture content of FS and FNS trays was lower than the other trays (Table 4). The addition of ZnO nanoparticles and kaolin as filler material decreased the water absorption by filling the spaces between fibres and making the surface even smoother. Even if the trays did not seem smoother in the SEM images, they were smoother visually. Also, the further addition of shortening decreased the water absorption due to its hydrophobic effect.
The water absorption of starch foam produced from cassava starch, 20% cellulose fibre and 10% sun flower protein [5], from cassava starch, 20% sugarcane bagasse fibre and 5% Na-MMT [6], and from cross-linked corn starch, 10% kaolin, 10% corn husk fibre, and 10% beeswax [18] were found, respectively, about 27, 55 and 9.3% and the densities of these products were 0.522, 0.310 and 0.199 g/cm3, respectively. The percent water absorption of FS and FNS trays was lower than these literature results. Also, the densities of FS and FNS trays were considerably lower than these three foam products.
Mechanical Properties
The flexural test was performed to measure the mechanical properties of the trays. Table 6 lists the results of flexural strength (S f ), strain to breakage (ɛ b ), and elastic modulus (E b ), which are calculated from the results of the flexural tests. The FK tray had the highest flexural strength and the lowest strain to breakage, and thus the highest elastic modulus. In addition, the flexural strength of the PW tray and FN trays was higher than the other trays. However, there was no significant difference in strain to breakage values and elastic modulus other than FK trays. It was thought that the higher flexural strength and elastic modulus of the FK tray were the result of the tray without a good foam structure (Fig. 3). In other words, the addition of kaolin adversely affected the foam formation and made the trays rigid and brittle. The higher flexural strength of PW and FN was thought to be due to having the lowest thickness of PW and strengthened cell wall structure of FN by nanoparticles.
Thermal Properties
The thermal properties of the trays were determined using DSC. The temperature-heat flow rate curves of supplementary materials and trays are given in Figs. 5 and 6, respectively. The melting temperatures and melting energies calculated from those curves using the software are given in Table 7.
It was found that the addition of fibres increased the melting time and temperature, while other supplementary materials (kaolin, ZnO nanoparticles, and shortening) affected the melting temperature differently, but led to decreased melting energies. The melting temperature of FNS was 221.79 °C, and was much higher than that of the other trays. The melting temperatures of other trays varied between 153 and 175 °C, which showed that the FNS was the most heat-resistant tray. Thus, the FNS trays can be used for food products that are served hot.
In a similar study, it was reported that the melting temperature of trays produced using only cross-linked corn starch was 146.8 °C. The addition of kaolin and beeswax decreased the melting temperatures to 140.9 and 114.1 °C, respectively. However, corn husk fibre and other combinations of those three materials with cross-linked corn starch led to increased melting temperatures [18]. In 2008, Lee and Hanna [28] published a paper in which they reported the melting temperature of tapioca starch foam as 162.4 °C. In 2011, Tongdeesoontorn et al. [29] demonstrated the melting temperature of cassava starch-based films as 165.4 °C. The present findings seem to be consistent with previous research and contribute additional evidence of the effects of starch source and supplementary materials on melting temperature.
Migration Properties
Glyoxal migration from the two most water-resistant trays, FS and FNS, was carried out with four replicates. Distilled water was used as a food simulant for the migration test. The graphical presentation of the amount of glyoxal migration with respect to time is given in Fig. 7.
The graphics show that the amount of glyoxal migration increased linearly in the first 6 h. After that, the migration continued to increase, but more slowly. It can be seen from the gradients of the first 6 h and the amount of glyoxal at 24 h that the glyoxal migration from FS was lower than that from the FNS tray. It was thought that the addition of lipid could decrease the migration of glyoxal.
The main reason for the migration analysis was to determine the amount of compounds in the packaging materials that have an adverse effect on human health. Glyoxal used to cross-link the starch in foam trays, can be found at a level of approximately 1 ppm in fermented foods like yoghurt, beer, and wine [30]. But it was thought that a higher amount of glyoxal could be dangerous for human health. It was reported by the German Federal Risk Assessment Institute (BfR) that glyoxal, which is used to thin papers and increase their water resistance for use as a food packaging material, can only migrate at a rate of 1.5 ppm/dm2 in 1 h [31]. As a result of the migration analysis, 0.85 ppm/dm2 of glyoxal migrated from FS and 1.3 ppm/dm2 migrated from FNS into the distilled water in 1 h. These results showed that the migration from the trays was lower than the legislative limit determined by BfR.
Conclusion
The trays for food packaging were produced from different starch sources that were cross-linked with glyoxal. The tray made from cross-linked wheat–potato starch mix (1:1) had both the most water resistance and the lowest density. Water resistance of the tray was further increased by incorporating wheat fibre, especially wheat fibre + shortening or wheat fibre + shortening + zinc oxide nanoparticles to the tray formulations. Moreover, due to their very low percentage of water absorption and low densities, the biodegradable FS and FNS trays can be used as an alternative to expanded polystyrene trays in food packaging applications. Moreover, the high melting point of the FNS tray can extend their uses to hot food packaging also. The glyoxal migration level from FS and FNS trays was under the legislative upper limit (1.5 ppm/dm2.h) mentioned by the German Federal Institute for Risk Assessment (Bundesinstitut für Risikobewertung, BfR).
References
Tiefenbacher KF (1993) J Macromol Sci Pure Appl Chem A30:727
Shogren RL, Lawton JW, Doane WM, Tiefenbacher KF (1998) Polymer 39(25):6649
Shogren RL, Lawton JW, Tiefenbacher KF, Chen L (1998) J Appl Polym Sci 68:2129
BeMiller J, Whistler R (2009) Starch: chemistry and technology, 3rd edn. Elsevier, London
Salgado PR, Schmidt VC, Molina Ortiz SE, Mauri AN, Laurindo JB (2008) J Food Eng 85(3):435
Vercelheze AES et al (2012) Carbohydr Polym 87:1302
Kaisangsri N, Kerdchoechuen O, Laohakunjit N (2012) Ind Crop Prod 37:542
Kaisangsri N, Kerdchoechuen O, Laohakunjit N (2014) Carbohydr Polym 110:70
Willett JL, Shogren RL (2002) Polymer 43(22):5935
Glenn GM, Orts WJ, Nobes GAR (2001) Ind Crop Prod 14(3):201
Shey J, Imam SH, Glenn GM, Orts WJ (2006) Ind Crop Prod 24(1):34
Cinelli P, Chiellini E, Lawton JW, Imam SH (2006) Polym Degrad Stab 91(5):1147
Kaur L, Singh J, Singh N (2006) J Sci Food Agric 86:1945
Zhou J, Zhang J, Ma Y, Tong J (2008) Carbohydr Polym 74:405
Uslu MK, Polat S (2012) Carbohydr Polym 87(3):1994
Soykeabkaew N, Supaphol P, Rujiravanit R (2004) Carbohydr Polym 58:1
Carr LG, Parra DF, Ponce P, Lugao AB, Buchler PM (2006) J Polym Environ 14:179–183
Polat S, Uslu MK, Aygün A, Certel M (2013) J Food Eng 116(2):267
Gáspár M, Benko Z, Dogossy G, Reczey K, Czigany T (2005) Polym Degrad Stab 90(3):563
Kaewtatip K, Tanrattanakul V, Phetrat W (2013) Appl Clay Sci 80–81:413–416
ISO 178:2003 (2003) Plastics-determination of flexural properties. https://www.iso.org/obp/ui/#iso:std:iso:178:ed-5:v1:en
Li R, Kenyon GL (1995) Anal Biochem 230(1):37
PoungroiM K, Holló B, Szécsényi KM (2014) J Therm Anal Calorim 115(1):833
Soykeabkaew N, Thanomsilp C, Suwantong O (2015) Compos Part A 78:246–263
Bénézet JC, Stanojlovic-Davidovica A, Bergeret A, Ferry L, Crespy A (2012) Ind Crop Prod 37:435–440
Vercelheze ANS, Oliveira ALM, Rezende MI, Muller CMO, Yamashita F, Mali S (2013) J Polym Environ 21:266–274
Guan J, Hanna MA (2006) Bioresour Technol 97(14):16–26
Lee SY, Hanna MA (2008) J Appl Polym Sci 110(4):2337–2344
Tongdeesoontorn W, Mauer LJ, Wongruong S, Sriburi P, Rachtanapun P (2011) Chem Cent J 5:6
Barros A, Rodrigues JA, Almeida PJ, Oliva-Teles MT (1999) J Liq Chrom Rel Technol 22(13):2061
Anonymous (2013) Paper and board for food contact. http://bfr.zadi.de/kse/faces/resources/pdf/360-english.pdf
Acknowledgements
This research was supported by the Scientific Research Coordination Unit of Akdeniz University (Grant No. 2012.02.0121.019).
Author information
Authors and Affiliations
Corresponding author
Rights and permissions
About this article
Cite this article
Aygün, A., Uslu, M.K. & Polat, S. Effects of Starch Sources and Supplementary Materials on Starch Based Foam Trays. J Polym Environ 25, 1163–1174 (2017). https://doi.org/10.1007/s10924-016-0886-0
Published:
Issue Date:
DOI: https://doi.org/10.1007/s10924-016-0886-0