Abstract
Dimeric β-cyclodextrins (β-CD) were prepared from the reaction of native β-CD with epichlorohydrin under basic conditions, and the effects on the diacetylene (DA) and polydiacetylene (PDA) liposomes have been investigated. Vesicular DA was solubilized in the presence of dimeric β-CD with the consequent inhibition of polymerization. The result is attributed to the formation of a complex between dimeric β-CD and DA liposomes, and it is clearly differentiated from that of monomeric β-CD. Furthermore, the ordered supramolecular structure of PDA was perturbed by the dimeric β-CD, which was detected from the visible color change. Finally, the morphological characteristics and size of PDA in the absence and presence of dimeric β-CD were examined using transmission electron microscopy and dynamic light scattering The results show fused structure of size more than 200 nm along with the deformation of the vesicles, and they represent a novel phenomenon of liposome structure induced by complexation with dimeric β-CD. The evaluated physicochemical characteristics can be applied to the development of carbohydrate-based detergents.
Similar content being viewed by others
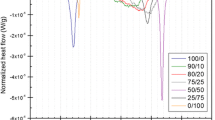
Avoid common mistakes on your manuscript.
Introduction
Diacetylenes (DA) are compounds possessing two triple bonds in conjugation that exhibit the unique property of polymerization via 1,4-addition under UV irradiation. Diacetylenyl lipids with polar head groups and long alkyl tails tend to spontaneously arrange into vesicular structures in aqueous solution. The scientific appeal of polydiacetylene (PDA) liposomes is derived from their distinctive electronic and optical properties based on the extended π-electron delocalization along their backbones [1, 2]. Variation of the effective π-conjugation lengths of the polymer backbones or conformational changes of the PDA unit induces color changes; thus, PDA undergoes a blue to red chromatic transition when thermal, mechanical, or chemical stimulus is applied [3]. Furthermore, the interesting fluorescence properties of PDA are evidenced by the lack of fluorescence of the initially polymerized blue-phase PDA, whereas the red-phase PDA fluorescence strongly at 560 and 630 nm [4]. Because of these intrinsic physicochemical properties, PDA has been exploited in the sensing of molecular recognition events, and the applications range from artificial membrane systems to biomolecular sensors [5].
Cyclodextrins (CD) are a series of cyclic oligosaccharides, and classified as α-, β- and γ-CD composed of six to eight glucose units connected through α-1,4 linkages, respectively. Based on their complex-forming ability with various substrates; these species are widely used as separation reagents, enzyme mimics, for host–guest interactions, and as drug carriers [6]. The torus shape of CD facilitates incorporation of hydrophobic compounds into the internal cavity. β-CD is the most widely studied host molecule for a variety of guest species in water because of its ready availability and appropriate size. β-CD has been extensively modified to widen and improve the catalytic and complex-forming abilities [7].
Recently, dimeric β-CD species tethered by diverse moieties have been reported to provide a number of advantages over native CD for complexation of guest species in terms of their stability, selectivity, and flexibility [8]. Based on the dimeric structure, cooperative complexations as well as simple statistical advantage are expected. For example, certain linked dimeric β-CD species have been reported to selectively bind the hydrophobic side chain in polypeptides and modulate the polypeptide aggregation process [9]. Various guest molecules, including dyes, drugs, and steroids, have been studied for complexation and solubilization by dimeric β-CD with disulfide, bipyridine, and diamine linkers [10–12]. However, there is no report on the complexation of dimeric β-CD with lipids or bio-membranes. Herein, the novel carbohydrate-lipid interaction between dimeric β-CD and diacetylenic lipids is described. This complexation induces membrane fusion, and thus further solubilizes the liposome structure.
In the present study, dimeric β-CD is synthesized using epichlorohydrin and the interactions between glyceryl-bridged dimeric β-CD and DA/PDA liposomes are investigated. In a prior study, the effects of monomeric CD on the formation and colorimetric transition of PDA were reported [13], indicating that α-CD exhibited a blue-to-red color transition as the most prominent effect of perturbation of the ordered structures of the PDA vesicles based on partial inclusion of PDA by α-CD. Thus, it is considered interesting to determine whether the effects of dimeric β-CD can be further augmented and made more selective and to explore the prospect of applications in biology and medicine.
Experimental
Chemicals
β-CD were obtained from Tokyo Chemical Industry Co., Ltd. β-CD was recrystallized from distilled water and dried in vacuo for 12 h. 10,12-Pentacosadiynoic acid (PCDA), 10,12-tricosadiynoic acid (TRCDA), and dimyristoylphosphatidylcholine (DMPC) were purchased from Sigma–Aldrich Chemicals Co. (St. Louis, Mo, USA).
Synthesis of dimeric β-CD
The dimeric β-CD host was synthesized according to a known literature procedure [14, 15]. A mixture of 2.83 g of β-CD in 10 mL of NaOH solution (5 %, w/w) was stirred for 24 h at 25 °C. Epichlorohydrin (EP) was added to the β-CD solution in a dropwise manner so that the molar ratio of β-CD to EP was 1:15. The mixture was heated to 60 °C and stirred at 600 rpm for 5 h. The reaction was terminated by the addition of 3 N HCl, and residual EP was removed by centrifugation. Finally, the dimeric fraction was separated using a Bio-Gel P4 column.
Nuclear magnetic resonance (NMR) spectroscopy
For the NMR spectroscopic analysis, a Bruker Avance 500 spectrometer was used to record the 1H-NMR, 13C-NMR, and HSQC spectra. NMR analyses were performed in D2O at room temperature.
Matrix-assisted laser desorption/ionization-time of flight mass spectrometry (MALDI-TOF MS)
The synthesized dimeric β-CD were dissolved in water and mixed with 2,5-dihydroxybenzoic acid (DHB) as the matrix. The mass spectra were recorded in DHB at a molar ratio of 10−3 with a total loading of around 1 μg of sample. For MALDI-TOF MS, we used a MALDI-TOF mass spectrometer (Voyager-DETM STR BioSpectrometry, PerSeptive Biosystems, Framingham, MA, USA) in the positive-ion mode.
Preparation of PDA liposomes
The PDA liposomes were prepared as previously described [13]. PCDA or TRCDA was dissolved in chloroform, and the organic solvent was removed by purging with N2 to generate a thin lipid film on the glass surface. To generate the artificial membrane system, a mixture of TRDCA (60 %) and DMPC (40 %) was dissolved in chloroform and dried with N2. A HEPES buffer solution (5 mM, pH 8.0) was added to yield a total lipid concentration of 1 mM. The samples were then heated at 80 °C for 15 min and probe sonicated for 12.5 min using a Sonics VC-505 instrument at 40 % of the power used. The resulting solution was filtered through a 0.8 μm filter (Satorius, Minisart), and the milky filtrate was cooled overnight at 4 °C. Polymerization was carried out at room temperature by UV irradiation at 254 nm for 15 min.
Quantitative assay
The color change of the mixed vesicles from blue to red was quantified by calculating the colorimetric response (%CR) according to the following equation:
where A blue is the absorbance at 640 nm, determined from the UV–vis spectrum of the “blue” component, and A red is the absorbance at 550 nm for the “red” component. The CR is derived from the change in the ratio of absorbances at 640 and 550 nm in the absence and presence of dimeric β-CD, β-CD, and α-CD. (A blue/(A blue+A red))0 and (A blue/(A blue+A red))1 are the red/blue ratios of a sample before and after an addition of CD, respectively.
Dynamic light scattering (DLS)
DLS measurements were carried out with a Wyatt Technology DynaPro Plate Reader at constant room temperature.
Transmission electron microscopy (TEM)
TEM images of the PDA liposomes were obtained using a JEM 1010 microscope (JEOL, Tokyo, Japan) operating at 80 kV. Vesicle samples were absorbed onto Formvar-coated copper grids (200 mesh) and air-dried for 5 min. For negative staining, 2 % uranyl acetate solution was used. The blue and purple PDA vesicles produced by interaction with dimeric β-CD were examined using transmission electron microscopy.
Results and discussion
Dimeric β-CD was synthesized using EP under basic conditions, and the dimeric fraction was separated from the reaction mixture on a Bio-gel P4 column. Although the dimerization methods vary depending on the cross-linker, EP is considered to be a powerful cross-linker because of its ease of use, flexibility, and universality [16]. However, the epoxy groups produce a network by crosslinking, thus, the reaction products are mixtures of various species from monomers to polymers [14]. In the present study, we used the prepared dimeric β-CD fraction, where they have the glyceryl bridges and the glycerol tails with different lengths. The representative chemical structures of the glyceryl bridged dimeric β-CD were shown in Fig. 1a; a diversity of substituents can be achieved because of the reactivity of the epoxy group. Although small portion of monomeric β-CD derivatives and trimeric β-CD are observed, the major fraction was dimeric β-CD (Fig. 1b). The average molecular weight (Mn) of dimeric β-CD was determined to be 2899 Da. Assignment of the dimeric β-CD protons and carbons required one-dimensional NMR (1H and 13C) experiments and two–dimensional NMR (Heteronuclear Single Quantum Coherence; HSQC) (data not shown), and the use of previous data for the 1H and 13C chemical shifts of the polymeric β-CD [14, 15]. Fig. 1c shows the –CH and –CH2 protons in the region 3.5–4.5 ppm except for H1 protons which appear down field. The location of the EP residues was evaluated from the 13C-NMR spectrum (Fig. 1d). Because of C2, C3, and C6 substitution on both sides of β-CD, resonances corresponding to C1′ (~102.6 ppm), C2′ (~80.5 ppm), C3′ (~78.5 ppm), and C6′ (~72.2 ppm) were observed. Substitution of C2 and C3 produces a downfield chemical shift (~5 ppm) of the respective carbons bearing the substituents, whereas an upfield shift (~2 ppm) is observed for β-carbon (C1′). The downfield chemical shift (~10 ppm) of the C6 resonance is attributed to substitution. Other carbon peaks affected by possible substitution are also designated, as shown in Fig. 1d. These structural analyses indicated that the glyceryl bridged dimeric β-CD was successfully synthesized.
Chemical structure (a), MALDI-TOF mass spectrum (b), 1H-NMR spectrum (c), and 13C-NMR spectrum (d) of dimeric β-cyclodextrin (β-CD). In Fig. 1b, the largest m/z of the monomeric, dimeric, and trimeric β-CD was written
To evaluate the effect of dimeric β-CD on the stability and photopolymerization of the DA liposome, dimeric β-CD (10 mM), β-CD (10 mM), and α-CD (10 mM) solutions were respectively added to PCDA liposome (1 mM). With the addition of dimeric β-CD solution, the opaque liquid became clear, as shown in Fig. 2a, b. No noticeable change was induced by addition of β-CD, and the addition of α-CD produced a white precipitate after showing a clear solution for a little while. For comparison, a clear vial containing only water was positioned on the right side (Fig. 2). After allowing the respective solutions to stand for 4 h, each was irradiated with UV light (254 nm) for 15 min. The solubilizing effect of dimeric β-CD on the DA liposomes was observed more clearly in Fig. 2e. Following UV-induced polymerization of the PCDA liposomes, the resultant alternating ene-yne structure is characterized by blue color. In the presence of the buffer and β-CD, the diacetylene liposomes exhibited the blue color because of polymerization. However, addition of dimeric β-CD generated a transparent solution, and the DA polymerization was inhibited. Although α-CD can also inhibit DA polymerization, α-CD resulted in the formation of a precipitate of the inclusion complex with PCDA [13].
Photographs of 10,12-Pentacosadiynoic acid (PCDA) liposome solutions containing CD at room temperature after 1 min (a), 30 min (b), 1 h (c), 4 h (d), and e after irradiation of sample d with UV light for 15 min. Total carbohydrate concentration is 5 mM. (1) Dimeric β-CD, (2) β-CD, (3) α-CD, (4) buffer, and (5) water
Subsequently, the samples in Fig. 2e were lyophilized and proton nuclear magnetic resonance (NMR) spectroscopic analyses were carried out in D2O to compare differences. 1H-NMR spectroscopy is a suitable method for the evaluation of non-covalent interactions at the molecular level [17]. In the case of PCDA liposomes/dimeric β-CD, blue-numbered peaks were down-shifted (0.13 ppm for ‘1’, 0.08 ppm for ‘2’, and 0.14 ppm for ‘3’) comparing with PCDA liposomes (Fig. 3a, d). Besides, new peaks appeared at 2.51 ppm. By interactions with dimeric β-CD, there may be an increase of membrane dynamics and changes of liposome conformation [18]. On the other hand, PCDA liposomes/α-CD and PCDA liposomes/monomeric β-CD have chemical shift change less than 0.01 ppm. This result indicates that dimeric β-CD disturbs the organization of the self-assembled vesicular structure by complexation of both molecules. In densely packed PCDA liposomes, the interchain distance of the PCDA supramolecules is approximately 0.5 nm; UV light initiates a radical polymerization process resulting in deeply colored PDA liposomes [19]. The reacting carbon atoms must be less than 0.5 nm away from each other or polymerization is not likely to occur. Accordingly, if dimeric β-CD perturbs the ordered DA liposome structure by solubilization because of complexation with DA, the polymerization process can be inhibited.
Partial 1H-NMR spectra of PCDA liposomes/dimeric β-CD (a), PCDA liposomes/monomeric β-CD (b), PCDA liposomes/α-CD (c), and PCDA liposomes (d) Inset in Fig. 3d shows the chemical structures of polymerized blue-phase PCDA liposomes
The effect on PDA is also shown in Fig. 4. First, 10 mM solutions of carbohydrate were added to the polymerized blue-phase PDA liposomes. After 1 h, the solution containing dimeric β-CD became purple because of decline in the absorption intensity at 640 nm and increase in the absorption at 550 nm. Although it appears that dimeric β-CD perturbs the PDA liposomes less effectively than the DA liposomes because of increased stability of the polymeric structure, the effect of dimeric β-CD is clearly different from that of monomeric β-CD (Figs. 2d, 4). The formation of red-phase PCDA liposomes by binding with α-CD was observed as previously reported [13]. Basically, the change of internal hydrogen-bonding is an important effect on the ability of carbohydrates to promote the colorimetric change of PDA [20]. Using this colorimetric assay, changes in the integrity of the membrane by dimeric β-CD can be observed indirectly with the naked eye. Previously, highly positively charged histone or lysine have been reported to induce blue-to red color changes due to electrostatic interactions of the molecules with the negatively charged PDA interface [21]. From the present result, the cooperative binding of dimeric β-CD can offer attractive alternative for molecular biosensing.
To compare the effects achieved with another lipid composition, PCDA, TRCDA and TRCDA/DMPC mixed liposomes were prepared for quantitative assay. (Fig. 5a) shows the colorimetric response (CR) of polymerized PCDA liposomes versus the concentration of CD. Both dimeric β-CD and α-CD show the same CR value of 35 % at 20 mM concentration, although the increasing patterns are different from each other. In the TRCDA liposome system, the CR values of dimeric β-CD and α-CD changed from 0 to 72 and 48 %, respectively (Fig. 5b). The higher CR values are reasonable because TRCDA liposomes are more sensitive system than PCDA liposomes. In principle, the length of.alkyl chain in TRCDA is slightly shorter than PCDA, which affects the thickness of vesicle.bilayer, chain rigidity, and overall interchain interactions. In addition, the plot of dimeric β-CD shows exponential-type growth, and the plot of α-CD reaches saturation at about 5 mM (Fig. 5b). The result indicates that the complexation pattern of dimeric β-CD/PDA is different from that of β-CD/PDA. Since the carboxylic acid tail of PCDA is capped by the cavity of α-CD, the triggered “ene-yne” backbone produces a red color until the entire liposome surface is saturated by α-CD [13]. On the other hand, it is postulated that dimeric β-CD interconnects two PDA liposomes via cooperative complexation. The PDA/DMPC liposomes are generally used as a bio-membrane mimic system, where the DMPC molecules are distributed within the conjugated PDA matrix, and most likely exist in a domain-like configuration [21]. The CR of the TRCDA/DMPC liposomes is presented in Fig. 5c. In the presence of dimeric β-CD, the exponential increase in the CR depending on the CD concentration was converted into the proportional type, and the saturation CR of α-CD increased by 30 % after adding DMPC molecules. Insertion of the DMPC domain produces an impact on the overall liposome structure and triggered conformation produced by dimeric β-CD or α-CD.
The size of the PDA liposomes was investigated using dynamic light scattering (DLS), and the size distribution is shown in Fig. 6. The diameter of the liposomes ranged from 20 to 80 nm in every case; however, dimeric β-CD produces size separation with larger vesicles, as shown in Fig. 6a. The relative intensity is 85.8 % and 14.2 % for 39 nm and 348 nm, respectively. The polydispersity index is 0.0103 and 0.0003 for 39 nm and 348 nm, respectively. The size and intensity of large nanoparticles can be varied as the interaction with dimeric β-CD proceeds. This result may occur because of membrane fusion caused by interaction with dimeric β-CD. To confirm this postulate, TEM image analyses were acquired. Fig. 7 shows the morphology of the PDA vesicles before and after the addition of dimeric β-CD. In the TEM image of the PDA (Fig. 7a), the vesicle size was less than 100 nm, and the vesicles were uniform, smooth, and spherical. The vesicles were also well separated from each other because of repulsive interactions of the carboxylic acid anion on the liposome surface. After the addition of dimeric β-CD, fused structures of size more than 200 nm were observed along with the deformation of the vesicles. The roughness of the surface is probably caused by a disruption of the hydrogen bonding between the carboxylic head-groups on the vesicle surface upon insertion of dimeric β-CD. During the fusion process, a change in the morphology from spherical to amoeboid is also apparent in the left side of Fig. 7a. In fact, polylysin induces cell fusion at a low concentration because of electrostatic binding to anionic cell surfaces [22]. Similarly, cationic polypeptide is adsorbed on the membrane of negative vesicles [23], permeation of DNA through cell membranes [20]. Although dimeric β-CD has no cationic charge, the dimeric conformation can provide the appropriate space to bind PDA vesicles. Because of the simple cavity (inner diameter: 0.78 nm; outer diameter: 1.53 nm) of β-CD, no binding with the liposome was observed. However, dimeric β-CD have two hydrophobic cavities, 1–12 units of glyceryl bridges, glycerol tails, and many hydroxyl groups. As another amphiphiles, dimeric β-CD may penetrate between membrane structures, then cause membrane fusion [24, 25]. Depending on the cross-linker, the pattern of binding and the membrane fusion ability will also vary.
In conclusion, dimeric β-CD was synthesized using EP and β-CD, and the effects of CD on the formation and color changes of PDA liposomes were evaluated. Based on NMR experiments, quantitative assay, DLS, and TEM, it is suggested that cooperative complexations between dimeric β-CD and liposomes induce fusion and solubilization of the membrane structure (Fig. 8). Thus, dimeric β-CD holds great promise for use as an effective carbohydrate-based detergent through complexation with the liposome structure. Further studies to determine the exact mechanism driving the liposome solubilization and to elucidate the mechanistic effects of the cross-linker are in progress.
References
Charych, D.H., Naqy, J.O., Spevak, W., Bednarski, M.D.: Direct colorimetric detection of a receptor-ligand interaction by a polymerized bilayer assembly. Science 261, 585–588 (1993)
Ahn, D.J., Kim, J.M.: Fluorogenic polydiacetylene supramolecules: immobilization, micropatterning, and application to label-free chemosensors. Acc. Chem. Res. 41, 805–816 (2008)
Charoenthai, N., Pattanatornchai, T., Wacharasindhu, S., Sukwattanasinitt, M., Traiphol, R.: Roles of head group architecture and side chain length on colorimetric response of polydiacetylene vesicles to temperature, ethanol and pH. J. Colloid Interface Sci. 360, 565–573 (2011)
Olmsted III, J., Strand, M.: Fluorescence of polymerized diacetylene bilayer films. J. Phys. Chem. 87, 4790–4792 (1983)
Charych, D., Cheng, Q., Reichert, A., Kuziemko, G., Stroh, M., Nagy, J.O., Spevak, W., Stevens, R.C.: A ‘litmus test’ for molecular recognition using artificial membranes. Chem. Biol. 3, 113–120 (1996)
Singh, M., Sharma, R., Banerjee, U.C.: Biotechnological applications of cyclodextrins. Biotechnol. Adv. 20, 341–359 (2002)
Easton, C.J., Lincoln, S.F.: Modified cyclodextrins: scaffolds and templates for supramolecular chemistry. Imperial College Press, London (1999)
Liu, Y., Chen, Y.: Cooperative binding and multiple recognition by bridged bis(β-cyclodextrin)s with functional linkers. Acc. Chem. Res. 10, 681–691 (2006)
Wahlström, A., Cukalevski, R., Danielsson, J., Jarvet, J., Onagi, H., Julius Rebek, J., Linse, S., Gräslund, S.: Specific binding of a β-cyclodextrin dimer to the amyloid β peptide modulates the peptide aggregation process. Biochemistry 51, 4280–4289 (2012)
Breslow, R., Chung, S.: Strong binding of ditopic substrates by a doubly linked occlusive C1 clamshell as distinguished from an aversive C2 loveseat cyclodextrin dimer. J. Am. Chem. Soc. 112, 9659–9660 (1990)
De Jong, M.R., Engbersen, J.F., Huskens, J., Reinhoudt, D.N.: Cyclodextrin dimers as receptor molecules for steroid sensors. Chemistry 6, 4034–4040 (2000)
Tabushi, I., Kuroda, Y., Shimokawa, K.: Duplex cyclodextrin. J. Am. Chem. Soc. 101, 1614–1615 (1979)
Kim, J.M., Lee, J.S., Woo, S.Y., Ahn, D.J.: Unique effects of cyclodextrins on the formation and colorimetric transition of polydiacetylene vesicles. Macromol. Chem. Phys. 206, 2299–2306 (2005)
Renard, E., Deratani, A., Volet, G., Sebille, B.: Preparation and characterization of water soluble high molecular weight β-cyclodextrin-epichlorohydrin polymers. Eur. Polym. J. 33, 49–57 (1997)
Li, J., Xiao, H., Li, J., Zhong, Y.: Drug carrier systems based on water-soluble cationic β-cyclodextrin polymers. Int. J. Pharm. 278, 329–342 (2004)
Kuniak, L., Marchessault, R.H.: Study of the crosslinking reaction between epichlorohydrin and starch. Starch Starke 24, 110–116 (1972)
Schneider, H.-J., Hacket, F., Rűdiger, V., Ikeda, H.: NMR studies of cyclodextrins and cyclodextrin complexes. Chem. Rev. 98, 1755–1786 (1998)
Timoszyk, A., Latanowicz, L.: Interactions of sialic acid with phophatidylcholine liposomes studies by 2D NMR spectroscopy. Acta Biochim. Pol. 60, 539–546 (2013)
Nie, X., Wang, G.: Synthesis and self-assembling properties of diacetylene-containing glycolipids. J. Org. Chem. 71, 4734–4741 (2006)
Lee, S., Kim, J.-M.: α-Cyclodextrin: a molecule for testing colorimetric reversibility of polydiacetylene supramolecules. Macromolecules 40, 9202–9204 (2007)
Kolusheva, S., Boyer, L., Jelinek, R.: A colorimetric assay for rapid screening of antimicrobial peptides. Nat. Biotechnol. 18, 225–227 (2000)
Yaroslavov, A.A., Sybachin, A.V., Kesselman, E.J., Talmon, Y., Rizvi, S.A.A., Menger, F.M.: Liposome fusion rates depend upon the conformation of polycation catalysts. J. Am. Chem. Soc. 133, 2881–2883 (2011)
Yaroslavov, A.A., Efimova, A.A., Lobyshev, V.I., Kabanov, V.A.: Reversibility of structural rearrangements in the negative vesicular membrane upon electrostatic adsorption/desorption of the polycation. Biochim. Biophys. Acta-Biomembr. 1560, 14–24 (2002)
Kragh-Hanse, U., Maire, M.I., Møller, J.V.: The mechanism of detergent solubilization of liposoems and protein-containing membranes. Biophys. J. 75, 2932–2946 (1998)
Kozlovsky, Y., Chernomordik, L.V., Kozlov, M.M.: Lipid intermediates in membrane fusion: formation, structure, and decay of hemifusion diaphragm. Biophys. J. 83, 2634–2651 (2002)
Acknowledgments
This work is supported by the National Research Foundation of Korea Grant funded by the Korean Government (NRF-2011-0024008) and supported by the Priority Research Centers Program through the National Research Foundation of Korea (NRF) funded by the Ministry of Education, Science and Technology (2012-0006686). SDG.
Author information
Authors and Affiliations
Corresponding author
Rights and permissions
About this article
Cite this article
Cho, E., Choi, J.M., Jeong, D. et al. Liposome solubilization induced by complexation with dimeric β-cyclodextrin. J Incl Phenom Macrocycl Chem 80, 427–435 (2014). https://doi.org/10.1007/s10847-014-0431-5
Received:
Accepted:
Published:
Issue Date:
DOI: https://doi.org/10.1007/s10847-014-0431-5