Abstract
Three different trunk heights (75 cm, 100 cm, and 125 cm), two grapevine training systems (vertical shoot positioning–shaped and Y‑shaped) were compared for their effects on yield, cluster characteristics, and must composition in Vitis vinifera L. cv. Karaerik in Erzincan, Turkey. We hypothesized that, compared to vines trained with wall-shaped support systems, vines trained with Y‑shaped support systems would have better yield, cluster characteristics, and must composition. Average yield regulations imposed by Y‑shaped support system–trained vines are usually higher than for vertical shoot positioning–shaped support system–trained vines, but similar results were obtained for total cluster number, cluster and berry weight, pruning weight, titratable acidity, maturation index, and total soluble solids among the trunk heights over the 3‑year period. Our results suggest that the trunk heights were insufficient to induce a grow-limiting response of vines, as trunk-height vines did not have lower fruit set, cluster characteristics, must composition, or bud fruitfulness. Although acceptable grape quality was obtained from three different trunk heights and two grapevine training systems, Y‑shaped support system–trained vines had greater total soluble solids at the expense of higher grape yield. We may, therefore, recommend Y‑shaped training systems because the anticipated crop yield increases of this system were logistically superior to an earlier training system. They are also recommended if the anticipated canopy structures of a training system are desired as a crop-regulation tool alternative to the Baran training system.
Similar content being viewed by others
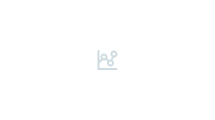
Avoid common mistakes on your manuscript.
Introduction
Every vine trellis/training system and trunk height displays a typical canopy structure, which in turn determines the microclimate quantity and quality within the canopy profile (Gladstone and Dokoozlian 2003). Indeed, the yield potential of a vine depends upon its trellis/training system and/or trunk height. Many biological processes that occur depend on the canopy characteristics of vines, such as bud differentiation, total vine photosynthesis, wood maturity, and fruit ripening, and are indeed closely related to the development of seasonal canopy (Poni et al. 1993; Kalkan and Keskın 2018; Kaya 2020a). Additionally, improper use of trellis/training systems could cause very different interactions between the surrounding environment and the leaf canopy, affecting the ratio of mature to young leaves, the spatial distribution of leaves, and canopy management (Dokoozlian and Kliewer 1995a). This situation may lead to inefficient vineyard design in low-vigor situations and in excessive fruit zone shading under vigorous conditions (Dokoozlian and Kliewer 1995b). Therefore, the way to maximize the performance of a vine is to balance the amount of fruit development with the amount of energy-producing parts (leaves) of the vine, and this could be possible by the use of a suitable training system (Kalkan et al. 2017).
Knowledge of how vines respond to different trellis/training systems and trunk heights will result in better control of vegetative growth and disease, greater yield, higher-quality clusters, optimization of the leaf area to fruit ratio, and better must composition (Reynolds and Heuvel 2009; Karadoğan et al. 2018; Kaya 2019). Due to the positioning of the bearing units, the trellis height, and the type of pruning, the amount of leaf area that may be continuously exposed to the sun and its photosynthesis is an important consideration in the selection of a trellis/training system. Research has confirmed the effects of all of these on development, vine growth, and yield. Generally, it has been found that not only quality but also growth and yield are directly proportional to the ratio of exposed leaf area to crop load. It was found that 7–14 cm2 of total leaf area was required for the ripening per gram of fruit in clusters (Howell 2001). However, this situation is significantly affected by environmental factors, and higher rates may be needed, especially in cool-climate viticulture, so that important physiological functions such as crop ripening, bud differentiation/initiation, wood and bud maturation, carbohydrate storage, and tolerance to cold can all occur with the available exposed leaf area (Howell 2001; Reynolds and Heuvel 2009; Kaya 2020b). Therefore, trellis/training systems are extremely important for maintaining the shoot, leaf, and reproductive organ balance of the vines in vineyard regions with cool climates.
Erzincan province is a cool region and is the most important grape-growing area of the northeastern Anatolian region of Turkey. The vines have been grown with the traditional Baran system, which is a prostrated system (Kaya and Köse 2017; Rende et al. 2018). For Baran-trained vines, the trunks are generally less than 0.3 m to 0.2 m high. It has been observed that the Baran vine-training method is the most commonly used of all available systems in this region. There are many negative reasons for the dominance of the Baran-training system: It is relatively very laborious to install and implement, and it is not suitable to vineyard mechanization, which is a big advantage at a time when increases in labor input and production costs per acre are ever present. Furthermore, between-row spacing, in-row vine spacing, and canopy height can all vary a great deal within the Baran-training system, and both spur-pruning and cane-pruning techniques could be difficult within its confines, resulting in a narrower range of canopy architecture. However, yields and grape quality with this system are quite variable across the region, depending upon seasonal conditions, site, and altitude. As with other cold or cool growing regions, there are concerns regarding excessive shading, insufficient photosynthesis, susceptibility to diseases, and uneven fruit ripening if canopies are not managed accordingly. Even so, many studies have found that the use or modification of different training systems to achieve balance between vine yield and vigor leads to the ability to improve fruit quality through a combination of improved fruit and canopy microclimate (Smart et al. 30,31,a, b; Reynolds and Heuvel 2009).
The objective of the work described here was to compare the cluster architecture and berry maturity, yield components, and canopy architecture response of Karaerik grape cultivar to three different trunk heights (75 cm, 100 cm, and 125 cm), two different support systems (vertical shoot positioning [VSP]-shaped and Y‑shaped), and bilateral cordon training systems throughout the entire growing season and to determine the factors that affect the performance of each system.
Materials and Methods
Experimental Design and Treatments
The experiment was conducted during the 2012–2014 growing seasons using 8‑year-old Karaerik grape cultivar (Vitis vinifera L.). The vineyard was located in Erzincan, Turkey (lat. 39° 45′06.54 N; long. 039°21′36.79 W), at an altitude of 1309 m. The lowest annual average temperatures for 2013, 2014, and 2015 were −3.6 ℃, −0.5 ℃, and −2.8 ℃, respectively, whereas the highest average temperatures were 23.4 ℃, 23.4 ℃, and 25.6 ℃, respectively. Considering the precipitation values in the working years in the vineyard area, these values were measured as 57.6 mm, 60.4 mm, and 70.3 mm, respectively, for the years 2013, 2014, and 2015. All vines were established own-rooted vines, trained to a bilateral spur-pruned cordon with the shoots vertically positioned, and support systems on vines were designed in Y‑shaped and VSP-shaped trellis configurations (Fig. 1). Row spacing and vine spacing were 3.0 m and 2.0 m, respectively, with rows oriented north–south. Three trunk heights with the same vine spacing and pruning level were used: three-bud spurs pruned bilaterally and cordon-trained at 0.75 m, 1.0 m, and 1.25 m above ground, vertically shoot-positioned, and two 12-bud canes and two three-bud spurs retained on VSP-shaped and Y‑shaped support system–trained vines. Using these three different trunk heights and two different support systems, each system and trunk height were replicated four times using six vine plots.
Yield, Cluster Characteristics, and Must Composition
Four shoots per vine (at E‑L stage 18–19) were randomly chosen and labeled (Coombe 1995). Berry maturity was measured weekly from veraison (E‑L stage 35) (Coombe 1995) until harvest for each system and trunk height. At harvest, grape clusters were collected from the labeled shoots of each vine, and the total cluster number and yield for each vine were recorded. The clusters were weighed with an electronic balance immediately after sampling to determine average cluster weight. All berries were then excised from the pedicel and were immediately weighed with an electronic balance (model C‑600-SX; Cobos, Barcelona, Spain) to determine average berry weight. The berry samples were then crushed by hand in plastic bags to extract the juice. For each measurement, 100 berries per treatment and per replicate were randomly sampled. Then the juice was centrifuged at 4000 rpm for 7 min (Hettich Zentrifugen, Universal 320, Germany) and was used for measurements of titratable acidity (TA), maturation index (MI-Brix), and total soluble solids (TSS). Total soluble solids were measured with a digital refractometer (BRX-242 Erma, Tokyo, Japan). Maturation index and TA were determined by an autotitrator, and must samples were analyzed for TA by using 0.1 M NaOH, (G20S, Mettler Toledo, Switzerland). Harvest was conducted when TSS reached approximately 17 °Bx for Karaerik, based on typical commercial harvest levels. The pruning weight of each system and trunk height replicate were calculated by weighing pruned shoots during pruning in spring by using a dynamometer. Additionally, the pruning weight of each replicate was determined by weighing pruned shoots by using a dynamometer after leaf fall.
Design and Statistical Analysis
The experiment was established in randomized blocks according to factorial design with three replicates. Data were analyzed as a wholly randomized design with four replicates. Statistical analysis was performed using two-way analysis of variance to assess whether there were significant differences between trunk heights for each support system parameter. Least significant difference (LSD) was applied at the 1% level for post hoc tests to assess differences between trunk heights for each support system parameter. All statistical analyses were performed using JMP statistical software (version 7.0, SAS Institute, Cary, NC, USA).
Results and Discussion
Although yield per vine during harvest was similar among the trunk heights, average yields for support systems varied significantly. Significant differences in average yield per vine were obtained from grapes produced in the Y system and grapes produced in the VSP system during 2013–2015 (Table 1). Canopies positioned with the VSP system had a lower average yield than canopies positioned with the Y system, with values ranging from 9.51, 9.28, and 8.06 kg.vine−1 for VSP vines to 11.09, 10.51, and 9.10 kg.vine−1 for Y vines, respectively, over all 3 years (Table 1). In the Y support system, the leaves on the shoot may have been exposed to less shade compared to the VSP support system. Indeed, the distribution pattern of the leaf region exposed to the sun may be more pronounced in Y‑positioned systems compared with VSP-positioned systems, with a localized leaf area density exposed to sunlight being generally lower in the VSP-positioned systems. It has been also reported that the orientation of the canopy to the sun could be correlated with the amount of light intercepted by the canopy, canopy architecture and the percentage of interior and exterior leaves, which is determined by training systems and row orientation (Freeman et al. 1992). It was determined in studies that arranging vines in certain positions to alter the leaf area for increased sunlight exposure of the perennial wood and shoots optimizes sunlight interception, resulting in increased yield potential and positive leaf area–to-yield relationships (Reynolds and Heuvel 2009).
There were no significant differences in total cluster number for each vine among trunk heights each year, except for the 2012 season. Trunk height of 75 cm in the Y‑support system had the highest cluster number per vine, whereas trunk height of 125 cm in Y‑support system vines had the lowest cluster number per vine during the 2012 period. In contrast, the number of clusters per vine of trunk height for 75 cm in the VSP-support system was found to be lower than for vines of trunk height of 125 cm during the 2012 season. There were significant differences in the number of average clusters per vine between the Y-support and VSP-support systems in 2012, 2013, and 2014. Differences between support systems with the highest and lowest numbers of average clusters per vine were 2.41 (total clusters/vine) in 2012, 2.22 (total clusters/vine) in 2013, and 2.32 (total clusters/vine) in 2014 (Table 2). This decrease would be expected because the VSP-support systems allowed for narrower canopies, with an associated decrease in exposed leaf area per meter of row, while the position of the shoots and cordons provided for less space at the top of the trellis. On the other hand, the Y‑support systems allowed for wider canopies, with an associated increase in exposed leaf area per meter of row, while the position of the shoots and cordons provided for more space at the top of the trellis. Thus, elimination of internal canopy shading of the Y‑support system of narrower vines through horizontal canopy expansion may lead to an increased cluster number per vine due to increased photosynthesis products throughout the season. As might be expected, it has been reported that enhancement of canopy length and volume per acre increased yields by 40% to 90% because of an increase in bud fruitfulness and in buds per vine (Shaulis et al. 1966). These results imply that the Y‑support system could improve the cluster number per vine and, therefore, improve yield by improving bud fruitfulness and subsequent flower bud initiation. The authors suggest that the manner in which a specific training system incorporates trellis to effectively accommodate vine vigor and capacity is important because of effects on microclimate and, consequently, fertility and photosynthesis (Smart et al. 1990).
In this study, trunk heights did not significantly affect average cluster or berry weight, TA, MI, or TSS in the 2013, 2014, or 2015 seasons (Tables 3, 4, 5, 6, and 7). Average cluster and berry weights were not influenced by either support system in the 2013 and 2014 seasons but were slightly decreased in the 2012 season (Tables 3 and 4). Additionally, the MI and TSS of berries were not impacted by the type of support system (Tables 5 and 6). These results are similar to those obtained by Falcao et al. (2008), where significant differences were not found in the TSS or Brix measurement of Cabernet Sauvignon grapes grown in Y‑support systems and a VSP trellis. Auvray et al. (1999) also reported no differences in final TSS values for berries grown using different systems. It was reported by Baeza et al. (2005) that in the 1990 and 1992 seasons, there were significant differences in the TSS of Tempranillo grapes grown in four training systems, but not in the 1991 season. Although there were no statistical differences between the support systems with regard to the MI of the berries, the overall maturation in the Y‑support system vines was higher than for the VSP-support system vines in the 3‑year period (Table 7). Differences between support systems with the lowest and highest sugar concentrations were 0.17 (for 75-cm trunk height) in 2013 and 5.09 (for 125-cm trunk height) in 2012 (Table 7). In particular, 125-cm trunk height vines in the Y‑support system had the highest sugar concentration in the 3 years for which significant differences were recorded, consistent with the assumption that wider surface area results in higher sugar concentrations. On the other hand, Baeza et al. (2005) reported that berries with higher surface area values had no tendency toward higher Brix measurements, suggesting that the surface area ceases to be a decisive factor after a certain value and that the solids then become dependent on other factors, such as yield. Indeed, there were no differences in yield levels between trunk heights in our 3‑year study. In this case, yield does appear to influence final Brix degree in the berries with both trunk height and support systems. As a matter of fact, previous studies have shown that increase in yield reduces sugar concentration, but different training treatments that result in lower yields also result in higher TSS, despite times with a delay in ripening (Byrne and Howell 1978; Cawthon and Morris 1977; Wolpert et al. 1983; Morris et al. 1985; Reynolds et al. 1995; Murisier 1996; Wolf et al. 2003; Karadoğan and Keskin 2017). In parallel with our results of Y‑shaped support system–trained vines, Auvray et al. (1999) did not observe differences in the final Brix values of vines with the highest yields attaining the highest TSS values, due to more abundant and better foliage growth. It has been reported that the training system did not have an important effect on cluster or berry characteristics, though an overload yielded fruits with the lowest sugar contents (Morris et al. 1985). However, it is generally known that decreasing the yield of vine per hectare is an effective method of increasing the sugar content in berries (Byrne and Howell 1978; Wolpert et al. 1983; Murisier 1996; Peterlunger et al. 2002). Our results recorded higher Brix values for the Y‑shaped support system with partly higher average yields per vine, which could be explained by the slightly higher TSS value obtained for Y‑shaped support system–trained vines because they had the larger surface area.
No significant relationship in titratable acidity was found between either the trunk heights or the two different support systems over the 3‑year period (Table 5). It is reported that berry ripening is delayed in systems with the highest pruning levels (Reynolds et al. 1995), but in our study, an equal number of buds were allowed on each vine during pruning for both trunk height and support systems, and so the acidity level in the trunk height and support systems were found to be similar. However, significant differences in TA levels have been reported, with higher levels in the juice of grapes from the bush, trellis, and lyre-trained vines as opposed to single-curtain and Geneva double-curtain vines (Auvray et al. 1999). Baeza et al. (2005) also reported that the acidity level in the vertical shoot-positioned system was similar to that for the single curtain, high bush, and short bush systems. On the other hand, no significant differences in pruning weight were observed among trunk heights, but there was a significant difference in average pruning weight between support systems over the 3‑year period. The Y‑shaped support system vines had significantly higher average pruning weights than the VSP-shaped support system vines (Table 8). Pruning weights of the vine, expressed as kilograms per meter of canopy length, are widely used to determine whether vines are well balanced, i.e., having neither too much growth nor too little (Shaulis 1982; Smart and Robinson 1991). The average pruning weight per meter in the Y‑shaped support system vines was found to be 0.35 kg in 2012, 0.39 kg in 2013, and 0.42 kg in 2014, whereas in the VSP-shaped support system vines it was 0.30 kg in 2012, 0.34 kg in 2013, and 0.36 kg in 2014 (Table 8). It has been shown that values of 0.3–0.6 kg pruning weight per meter of canopy length in a vine are usually considered to be in the optimal range for canopy density and microclimate (Shaulis 1982; Smart and Robinson 1991). If we accept that the published values of pruning weight per meter of canopy length within the range of 0.3–0.6 kg are indicative of well-balanced vines, our data show that a pruning weight per vine of 0.3 kg (VSP support system) to 0.4 kg (Y-support system) was required for fruit maturation and that differences between support systems were mainly due to variations in canopy surface area per unit weight of pruning.
Conclusions
There are several remarks to be made regarding weaknesses and advantages of the assessment of three different trunk heights (75 cm, 100 cm, and 125 cm) and two different support systems (VSP-shaped and Y‑shaped) of canopy structure after a 3‑year period. There were no significant differences in yield, total cluster number (except for the 2012 season), cluster or berry weight, pruning weight, TA, MI, or TSS for each vine among trunk heights over the 3‑year period. Even though there was a significant difference in yield and pruning weight per vine in terms of Y‑shaped and VSP-shaped support system–trained vines, other evaluations of the vines showed no significant differences in total cluster number, cluster or berry weight, TA, MI, or TSS. Those results were not unexpected, since a few researchers have indicated that Y‑trained vines have a higher percentage of their shoot position and/or leaf area at light saturation than VSP-trained vines. In general, 125-cm trunk-height trained vines had a clear advantage over the other trunk-height trained vines, as the high grape yield was guaranteed by photosynthetic efficiency and large surface area. The VSP-shaped support system–trained vines presented lower vegetative development, resulting in less leaf region exposed to the sun, making it less efficient. Based on our results, both support systems and all trunk heights achieve high exposure at lower leaf area densities or shoot number compared to Baran-trained vines (nonpositioned systems) in the region. In summary, to reduce potential shading and leaf area density within the canopy interior and to obtain better crop quality, we recommend that it would be more appropriate to use the of Y‑support system trained with 125-cm trunks and giving up the Baran system in the region.
References
Auvray A, Baeza P, Ruiz C, González-Padierna CM (1999) Influence de différentes géométries de couvert vegetal sur la composition du moût. Progr Agric Vitic 166:253–257
Baeza P, Ruiz C, Cuevas E, Sotés V, Lissarrague JR (2005) Ecophysiological and agronomic response of Tempranillo grapevines to four training systems. Am J Enol Vitic 56:129–138
Byrne ME, Howell GS (1978) Initial response to Baco noir grapevines to pruning severity, sucker removal and weed control. Am J Enol Vitic 29:192–198
Cawthon DL, Morris JR (1977) Yield and quality of Concord grapes as affected by pruning severity, nodes per bearing unit, training system, shoot positioning and sampling date in Arkansas. J Am Soc Hortic Sci 102:760–767
Coombe BG (1995) Adoption of a system for identifying grapevine growth stages. Aust J Grape Wine Res 1:104–110
Dokoozlian NK, Kliewer WM (1995a) The light environment with grapevine canopies. I. Description and seasonal changes during fruit development. Am J Enol Vitic 46:209–218
Dokoozlian NK, Kliewer WM (1995b) The light environment with grapevine canopies. II. Influence of leaf area density on fruit zone light environment and some canopy assessment parameters. Am J Enol Vitic 46:219–226
Falcao LD, Chaves ES, Burin VM, Falcão AP, Gris EF, Bonin V, Bordignon-Luiz MT (2008) Maturity of Cabernet Sauvignon berries from grapevines grown with two different training systems in a new grape growing region in Brazil. Cien Invest Agrar 35(3):321–332
Freeman BM, Tassie E, Rebbechi MD (1992) Training and trellising. Viticulture 2:42–65
Gladstone EA, Dokoozlian NK (2003) Influence of leaf area density and trellis/training system on the microclimate within grapevine canopies. Vitis 42:123–131
Howell GS (2001) Sustainable grape productivity and the growth-yield relationship: a review. Am J Enol Vitic 52:165–174
Kalkan NN, Keskın N (2018) The effects of trunk height and training systems on the some physicochemical properties of ‘Karaerik’ berries. Yuzuncu Yil Univ J Agric Sci 28(Spec):257–267
Kalkan NN, Kaya Ö, Karadoğan B, Köse C (2017) Farklı Gövde Yüksekliğine Sahip Karaerik (V. vinifera L.) Üzüm Çeşidinin Kış Gözlerinde Soğuk Zararı Ve Lipid Peroksidasyon Düzeyinin Belirlenmesi. Alinteri J Agric Sci 32(1):11–17
Karadoğan B, Keskin N (2017) Karaerik (Vitis vinifera L. cv. “Karaerik”) Klonlarının Kalite ve Fitokimyasal Özellikleri. Türk Tarım Doga Bilim Derg 4(2):205–212
Karadoğan B, Keskin N, Kunter B, Oğuz D, Kalkan NN (2018) Karaerik (Cimin) Klonlarinin Toplam Fenolik ve Antioksidan Içerikleri Bakimindan Karşilaştirilmasi. Bahce 47(Özel Sayı 1):117–120
Kaya O (2019) Effect of manual leaf removal and its timing on yield, the presence of lateral shoots and cluster characteristics with the grape variety ‘Karaerik’. Mitt Klosterneubg Rebe Wein Obstbau Früchteverwertung 69(2):83–92
Kaya O (2020a) Defoliation alleviates cold-induced oxidative damage in dormant buds of grapevine by up-regulating soluble carbohydrates and decreasing ROS. Acta Physiol Plantarum 42:1–10
Kaya O (2020b) Bud death and its relationship with lateral shoot, water content and soluble carbohydrates in four grapevine cultivars following winter cold. Erwerbs-Obstbau 62(1):43–50
Kaya Ö, Köse C (2017) Determination of resistance to low temperatures of winter buds on lateral shoot present in Karaerik (Vitis vinifera L.) grape cultivar. Acta Physiol Plant 39(9):209
Morris JR, Sims CA, Cawthon DL (1985) Yield and quality of “Niagara” grapes as affected by pruning severity, nodes per bearing unit, training system and shoot positionig. J Am Soc Hortic Sci 110:186–191
Murisier F (1996) Optimalisation du rapport feuille-fruit de la vigne pour favoriser la qualité du raisin et l’accumulation des glucides de réserve. Relation entre le rendement et la chlorose. Ph.D. thesis. L’école Polytechnique Fédérale de Zurich, Zurich
Peterlunger E, Celotti E, Dalt GDA, Stefanelli S, Gollino G, Zironi R (2002) Effect of training system on Pinot noir grape and wine composition. Am J Enol Vitic 53:14–18
Poni S, Marchol L, Intrieri C, Zerbig G (1993) Gas-exchange response of grapevine leaves under fluctuating light. Vitis 32:137–143
Rende M, Kose C, Kaya O (2018) An assessment of the relation between cold-hardiness and biochemical contents of winter buds of grapevine cv. ‘Karaerik’ in acclimation-hardening-deacclimation phases. Mitt Klosterneubg Rebe Wein Obstbau Früchteverwertung 68(2):67–81
Reynolds AG, Heuvel JEV (2009) Influence of grapevine training systems on vine growth and fruit composition: a review. Am J Enol Vitic 60(3):251–268
Reynolds AG, Wardle D, Naylor A (1995) Impact of training system and vine spacing on vine performance and berry composition of Chancellor. Am J Enolvitic 46:88–97
Shaulis NJ (1982) Responses of grapevines and grapes to spacing of and within canopies. In: Davis, Webb CAAD (eds) Grape and wine centennial symposium proceedings 18–21 June 1980 University of California, Davis, pp 353–360
Shaulis NJ, Amberg H, Crowe D (1966) Response of concord grapes to light exposure, and Geneva double curtain training. Proc Am Soc Hortic Sci 89:268–280
Smart RE, Robinson M (1991) Sunlight into wine. A handbook for winegrape canopy management. Winetitles, Adelaide, p 88
Smart RE, Robinson JB, Due GR, Brien CJ (1985a) Canopy microclimate modification for the cultivar Shiraz. I. Definition of canopy microclimate. Vitis 24:17–31
Smart RE, Robinson JB, Due GR, Brien CJ (1985b) Canopy microclimate modification for the cultivar Shiraz. II. Effects on must and wine composition. Vitis 24:119–128
Smart RE, Dick JK, Gravett IM, Fisher BM (1990) Canopy management to improve grape yield and wine quality principles and practices. S Afr J Enol Vitic 11:3–17
Wolf TK, Dry PR, Iland PG, Botting D, Dick J, Kennedy U, Ristic R (2003) Response of Shiraz grapevines to five different training systems in the Barossa Valley, Australia. Aust J Grape Wine Res 9:82–95
Wolpert JA, Howell GS, Mansfield TK (1983) Sampling Vidal blanc grapes. I. Effect of training system, pruning severity, shoot exposure, shoot origin and cluster thinning on cluster weight and fruit quality. Am J Enolvitic 34:72–76
Acknowledgements
This research work was supported by the General Directorate of Agricultural Research and Policies (Scientific Research Project-SRP, project number TAGEM/BBAD/13/AO8/PO4/02) appropriated to the Erzincan Horticultural Research Institute, Turkey.
Author information
Authors and Affiliations
Contributions
N. N. Kalkan designed the study. O. Kaya wrote the manuscript and interpreted the results. B. Karadoğan, Z. Kadioğlu, S. Albayrak, and İ. Esmek were responsible for performance of the research, collection, data analysis, and interpretation.
Corresponding author
Ethics declarations
Conflict of interest
N.N. Kalkan, B. Karadoğan, Z. Kadioğlu, İ. Esmek, S. Albayrak, and O. Kaya declare that they have no competing interests.
Rights and permissions
About this article
Cite this article
Kalkan, N.N., Karadoğan, B., Kadioğlu, Z. et al. Response of Karaerik Grape Cultivar (Vitis vinifera L.) to Two Training Systems and Three Trunk Heights. Erwerbs-Obstbau 64 (Suppl 1), 119–127 (2022). https://doi.org/10.1007/s10341-022-00672-z
Received:
Accepted:
Published:
Issue Date:
DOI: https://doi.org/10.1007/s10341-022-00672-z