Abstract
The present study examined the effect of agonist activation and antagonist co-activation on the shape of the knee extension moment–angle relationship in adults and children. Isometric knee extension maximum voluntary contractions (MVCs) were performed at every 5° of knee flexion between 55° and 90° (full extension = 0°) by ten men, ten women, ten boys and ten girls. For each trial, the knee extensors’ voluntary activation level was quantified using magnetic stimulation and the level of antagonist co-activation was quantified from their electromyographical activity. Peak MVC moment was greater for men (264 ± 63 N m) than women (177 ± 60 N m), and greater for adults than children (boys 78 ± 17 N m, girls 91 ± 28 N m) (p < 0.01). The agonistic activation level was greater for adults (~85%) than children (~70%). Similarly, antagonist co-activation was greater for adults than children, but relative to the agonist moment there were no differences between groups (all groups 7–8%). Correcting the peak moment for agonist and antagonist activation levels resulted in moments produced by fully activated agonist muscles of 334 ± 83, 229 ± 70, 114.2 ± 32 and 147 ± 46 N m, for men, women, boys and girls, respectively. Although correcting for shifts in joint angle during contraction altered the angle of peak moment by ~10° (p < 0.01), the peak moment occurred at ~60° for all groups. Changes in tendon stiffness, muscle size and architecture, and the pattern of the moment arm–angle relationship may in combination occur so that as children develop and mature into adults the shape of the moment–angle relationship is not altered.
Similar content being viewed by others
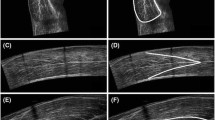
Avoid common mistakes on your manuscript.
Introduction
An increase in muscle strength during maturation has widely been reported, with increases in the joint moments that can be produced due largely to muscle hypertrophy (e.g. Kanehisa et al. 1995; Parker et al. 1990; Round et al. 1999; Wood et al. 2004). However, the measured joint moments are the sum of the moments produced voluntarily by the activated agonist muscles minus the moments of the co-activated antagonist muscles. As a result, the agonist (Bampouras et al. 2006; Newman et al. 2003) and antagonist (Kellis and Baltzopoulos 1997) muscle activation levels can play a significant role in determining the magnitude of the measured joint moment, and might contribute to some of the difference in maximal joint moment observed between adults and children.
Comparative data on voluntary activation levels in adults and children are scarce. Paasuke et al. (2000) found that the ratio between the resting twitch and MVC moment of the ankle plantarflexors decreased with age, indicating an increased level of voluntary activation. Belanger and McComas (1989) used the interpolated twitch technique (ITT) during isometric ankle plantarflexion maximum voluntary contractions (MVCs) and found that the activation level was 94% for young boys (aged 6–13 years) compared to 99.4% for older boys (aged 15–18 years). However, this did not represent a statistically significant difference, possibly due to the large variation amongst the young boys (SD of ±11.3 in the young boys compared to ±1.8 in the older boys). In both studies the use of single twitches rather than doublets or short tetani may have overestimated the true activation level (Bampouras et al. 2006).
The existing data on antagonist co-activation in children are limited to isokinetic trials, with the average EMG activity of the antagonists normalised to that of an MVC throughout the whole range of motion across a range of angular velocities. No differences between children and adults have been reported (Bassa et al. 2005; Kellis and Unnithan 1999). However, there are no reports of the magnitude of the antagonist moment and this is important since the consequences of antagonist activation will depend not only on the extent of activation but also the size and strength of the muscle.
In addition to contributing to the determination of the magnitude of a moment at a given joint angle, the level of voluntary activation and antagonist co-activation may also change with joint angle. This could alter the shape of the moment–joint angle relationship and the angle at which peak joint moment occurs. Although it has been reported that antagonist co-activation (Baratta et al. 1988; Kellis and Baltzopoulos 1997) and agonist activation (Newman et al. 2003; Bampouras et al. 2006) levels for knee extension contractions are relatively consistent across joint angles in adults, it is not known if this is the case in children. The level of agonist and antagonist activation depend on a number of factors that may differ between children and adults, such as a lack of neural coordination, joint instability (Ahmad et al. 2006) or a mechanism to protect or stabilise weaker joints at end ranges of motion. Furthermore, the level of antagonist co-activation is determined to a large extent by the level of agonist activation (Kellis and Baltzopoulos 1997). Consequently, if agonist and antagonist muscle activation levels have dissimilar relationships with joint angle in adults and children, then the resulting moment–angle relationships would also be different. This may to some extent explain the finding of a previous study that the knee extension moment–angle curve was shifted towards more extended joint angles in men compared with boys (Marginson and Eston 2001). The authors in that study suggested that the shift was caused by a change in the angle at which the optimum sarcomere length occurred as a result of the tendon becoming stiffer with maturation and growth.
In addition to the level of agonist and antagonist activation, the measured moment–knee angle relationship can also be affected by the shift in the actual “internal” knee angle during contraction from the resting dynamometer-set “external” angle (Arampatzis et al. 2004). This shift is the result of compression of the padding on the dynamometer and of the participant’s soft tissue. Since adults have a large amount of soft tissue than children and they exert greater forces to compress or displace this material, it is feasible that the discrepancy between the internal and external knee angle is greater adults, and as a result their moment–angle relationship is displaced to more extended joint angles.
If the finding of Marginson and Eston (2001), and present hypothesis of different moment–angle relationships in adults and children are correct, it would mean that muscle strength measurements in experimental and clinical applications should be made at different joint angles in adults and children to ensure assessment of the muscle in the same region of force-length relationship in both age groups.
The purpose of the current study was to examine the role of agonist voluntary activation, antagonist co-activation and the internal angle shift on the knee extension moment–joint angle relationship of adults and children of both genders. The magnitude and knee angle of peak joint moment were specifically examined as important elements of this relationship.
Methods
Participants
Forty participants, consisting of ten men, ten women, ten boys and ten girls volunteered to participate in this study (mean age, height and body mass are presented in Table 1). The physical maturity of the children, who were all aged 8–10 years, was not directly assessed; however, previous reports (Tanner 1962) suggest that children in this age range would be pre-pubertal (stage 1). The adult participants recruited were all sedentary in their daily lives and the children were not taking part in any organised sport or physical activity outside of school to negate any training effect on the measurements. The study was approved by the ethics committee of the Institute for Biomedical Research into Human Movement and Health of the Manchester Metropolitan University, and informed consent was obtained from all participants and the parents or guardians of the children prior to testing.
Experimental measurement schedule
Participants were familiarised with the experimental procedures in a separate session between 2 days and 2 weeks before the data collection session. Measurements of isometric knee extensor muscle strength and agonistic (knee extensor) and antagonistic (knee flexor) activation levels were taken on the dominant leg at knee joint angles between 55° and 90° of knee flexion (0° is full extension) at intervals of 5°. This joint angle range and interval were chosen to include joint angles surrounding the angle of peak knee extension moment in both adults and children (Marginson and Eston 2001), whilst providing sufficient precision to identify any slight changes with joint angle.
Isometric strength
The isometric moment–angle relationship of the knee extensors was determined using an isokinetic dynamometer (Cybex NORM, New York, USA). Participants were seated and securely strapped on the dynamometer chair, with the centre of rotation of the dynamometer lever arm aligned with the lateral femoral condyle of the participants’ dominant leg and the hip angle was set to 85° (0° is full extension). A warm up consisting of several sub-maximal efforts across a range of joint angles and a maximum effort at an extended (60°) and a flexed (85°) joint angle was completed before the test trials were performed.
The test trials consisted of knee extension MVCs at the joint angles of interest in a randomised order. Each MVC was developed gradually over ~2 s, with the maximum joint moment generated maintained for a further 1–2 s, this allowed the experimenter sufficient time to apply the superimposed stimulation at the point of MVC. To aid with motivation and effort, verbal encouragement was provided for all trials and participants were able to see a real-time graph plotting the joint moment they were producing (Baltzopoulos and Kellis 1998).
The internal joint angle during contraction was measured with an electronic goniometer (Biometrics Ltd., Newport, UK) placed on the lateral side across the knee joint. The proximal and distal ends of the goniometer were securely taped, equidistantly either side of the tibio-femoral cleft, on the thigh in line with the femur and on the shank in line with the tibia. Zero degrees was set with the participant at full knee extension.
Antagonist co-activation
The level of antagonistic co-activation during the knee extension MVC was estimated from the moment–EMG relationship of the knee flexor muscles. At each joint angle tested, participants performed isometric knee flexions at 10, 20, 50, 70 and 100% of MVC, during which the EMG of the biceps femoris muscle was recorded through two Ag–AgCl percutaneous electrodes 10 mm in diameter (Ambus A/s, Denmark), placed 20-mm apart at the distal third of the muscle belly and smoothed using the RMS over 50 ms. From the moment–EMG relationship constructed, the EMG of the biceps femoris during the knee extensions was reduced to a joint moment that this muscle would produce as an antagonist (Kellis and Baltzopoulos 1997; Reeves et al. 2003, 2004).
Voluntary activation level
The level of voluntary activation in the agonist muscle can be calculated using two methods, the interpolated twitch technique (ITT, Eq. 1) and the central activation ratio (CAR, Eq. 2) (Bampouras et al. 2006; Behm et al. 2001).
The ITT provides a more accurate estimate of voluntary activation level at a given joint angle, however, it is susceptible to errors resulting from slack in the series elastic elements damping the resting twitches (Bampouras et al. 2006). This effect can make the ITT an unsuitable means of quantifying activation level across a range of joint angles. The CAR method overestimates activation level at a given joint angle if the superimposed stimulation fails to activate fully the inactive portion of muscle during MVC, but it is not susceptible to errors introduced by differences in the series elastic component because it does not rely on resting twitches and therefore it has some advantages when describing differences in activation level across joint angles (Bampouras et al. 2006).
The current study is concerned with both the absolute levels of voluntary activation and its changes across a range of joint angles. Consequently neither method independently can provide a valid means for determining an accurate estimate of activation level across a range of joint angles for the reasons explained above. The approach taken was to quantify the changes in activation across joint angles through the CAR, but combining the CAR and ITT methods to obtain the actual level of activation at any joint angle using Eq. 3.
where ITTα and CARα are the ITT and CAR estimates, respectively, at the joint angle of interest, and ITT90 and CAR90 are the ITT and CAR estimates, respectively, at 90°. This equation uses the ratio between CARα and CAR90 to quantify the relative change in activation level across joint angles, and the ITTα is then calculated from ITT90, which is considered to represent the “true” level of activation, and this ratio. Ninety degrees was chosen as the reference joint angle as it was the most flexed joint angle examined, and therefore, the one that had the least muscle-tendon slack that would affect the estimation of activation using the ITT method.
The level of voluntary activation was quantified from superimposed and resting doublets evoked using magnetic stimulation applied percutaneously (pulse duration 1 ms, rise and fall durations 1 μs, inter-stimulus gap 10 ms and a magnetic field of 2.0 T, produced by a 70-mm diameter “Double coil”; MagStim, Bi-Stim2, Carmarthenshire, Wales), as previously described (O’Brien et al. 2008). The strength of the doublet evoked by magnetic stimulation depends on the placement of the coil relative to the muscle. Prior to testing the site that produced the greatest resting doublet at 90° of knee flexion was identified through a trial-and-error process, marked and used for all test trials. During the test trials the superimposed doublet was applied when the MVC reached a plateau, with the resting doublet being applied approximately 4 s later when the muscle was relaxed.
Calculation of the quadriceps muscle moment
The knee extension MVC moment (jointMVC) recorded by the dynamometer is the resultant moment of the voluntarily activated quadriceps muscle and the antagonistic knee flexors. The moment produced by the quadriceps muscle alone (quadMVC) was estimated from Eq. 4, as the sum of the jointMVC moment and the calculated antagonist moment (Kellis and Baltzopoulos 1997; Reeves et al. 2003, 2004).
To calculate the resultant joint moment that would be produced if the quadriceps muscle were fully activated (joint100%), the jointMVC moment and ITT activation level were combined in Eq. 5, assuming a linear relationship between moment and voluntary activation levels.
To estimate the moment that would be produced by the quadriceps muscle alone if they were fully active (quad100%), the QuadMVC moment calculated from Eq. 4 was corrected for voluntary activation deficit (Eq. 6)
Statistical analysis
The agonist activation level–joint angle relationship was analysed from the ITT estimates at each joint angle based on Eq. 3 using a 4 × 8-way (group × joint angle) repeated measures analysis of variance (ANOVA). The absolute and relative to quadMVC antagonist co-activation levels were analysed across joint angles and between groups using a 4 × 8 two-way (group × joint angle) repeated measures ANOVA.
The analysis of agonist activation and antagonist co-activation with joint angle was performed by grouping the data by the external joint angle rather than the internal joint angle. This was to ensure that there were a consistent number of data points, and one from each participant, within each 5° interval. Had internal joint angle been used to group the data, the number of data points within each joint angle interval would not be consistent, meaning that some intervals might have a very few data points in, whilst others might have several data points from the same person. This approach could prevent a normal distribution for each internal joint angle interval, compromising the results of the statistical testing; hence, it was not adopted.
The peak jointMVC, joint100%, quadsMVC and quads100% moments were identified for every individual in each group and analysed using a 4 × 4 (moment measure × group) mixed measures ANOVA. Similarly, the external and internal knee angles at which the jointMVC, joint100%, quadsMVC and quads100% moments peaked were identified for every individual in each group and analysed using a 2 × 4 × 4-way (angle measure × moment measure × group) repeated measures ANOVA. For each test Bonferroni corrected post hoc tests were performed where appropriate, and the level of statistical significance was set at p ≤ 0.05. All data are reported as the mean ± standard deviation.
Results
The jointMVC moment–external angle relationship for each subject group is shown in Fig. 1. The peak jointMVC moment produced by men was significantly higher (p < 0.01) than that of women, and both men and women produced significantly higher peak jointMVC moments than children (p < 0.01); although there were no statistical differences between boys and girls (Table 2).
The peak jointMVC moment occurred at similar external joint angles in all groups (~70°). During contraction, the internal joint angles were significantly lower than the corresponding external joint angles (p < 0.01) (Table 3). This led to the peak jointMVC moment occurring at an internal angle of 58.4 ± 7.5°, 62.5 ± 10°, 56.1 ± 6.6° and 60.7 ± 5.3° for men, women, boys and girls, respectively. There were no significant differences between the internal angle of peak jointMVC moment of any group. The jointMVC moment–internal angle relationship for each group is shown in Fig. 2.
The jointMVC moment–internal angle relationship for a men, b women, c boys and d girls. Open circles represent data points from all participants, filled squares represent the mean of all data points within each 5° interval. The dashed vertical line indicates the mean internal angle of peak jointMVC from all participants within each group
The moment produced by antagonist co-activation did not change across joint angles for any group (p > 0.05), with men, women, boys and girls experiencing a mean antagonist moment across all joint angles of 16.2 ± 8.3, 13.5 ± 7.9, 5.9 ± 4 and 6.8 ± 4.9 N m, respectively. The antagonist moment in men was significantly higher than in boys (p = 0.01) and girls (p = 0.05), but no other significant differences existed between the groups. The antagonist moment normalised to the quadsMVC moment was 6.6 ± 3.1, 8.3 ± 4.8, 8.4 ± 5.5 and 7.6 ± 4.3% for men, women, boys and girls, respectively, with no differences across joint angles or between groups. These results indicate that the increase from jointMVC moment to quadsMVC moment was proportional in all groups, and therefore, men were still significantly stronger than women, and both adult groups were significantly stronger than either child group. Additionally, the internal joint angle at which quadsMVC moment peaked did not change from that of jointMVC moment (~60° for all groups).
The voluntary activation level did not differ across joint angles for any group. Therefore, to quantify the actual level of voluntary activation, each participant’s ITT estimate at 90 deg was used for all joint angles, which was 85.6 ± 8.5% for men, 86.6 ± 6.6% for women, 75.1 ± 12.8% for boys and 66.9 ± 13% for girls. The ITT estimate for the girls was significantly lower (p < 0.01) than that for men or women. As voluntary activation level differed between groups, the estimated joint100% moment increased from the jointMVC moment by different amounts, proportional to the quantity of muscle that remained inactive (100-ITT %) for each group (Table 2). The use of the same ITT value for all joint angles meant that the internal angles of peak joint100% were the same as peak jointMVC moments angles.
The estimated quads100% moment was significantly higher than the measured jointMVC for all groups (p < 0.01). The quads100% moment estimated in men was significantly higher than that in women, and both adult groups were stronger than the children (Table 2). Neither antagonist co-activation nor voluntary activation level changed across joint angles, and consequently the peak quads100% moment occurred at the same angle as the jointMVC moment (~60° internal angle), which did not differ between groups. The quads100% moment–joint angle relationship is plotted in Fig. 3.
The fully activated quadriceps (quad100%) moment–internal angle relationship for a men, b women, c boys and d girls. Open circles represent data points from all participants, filled square represent the mean of all data points within each 5° interval. The dashed vertical line indicates the mean internal angle of peak quad100% from all participants within each group
Discussion
The purpose of the current study was to examine the extent of agonist voluntary activation level and antagonist co-activation during knee extension in adults and children of both genders to see whether these might explain the reported differences in the moment–joint angle relationships. As expected, it was found that men were stronger than women, and adults were stronger than children, while the strength of boys and girls was similar. However, contrary to a previous report (Marginson and Eston 2001), the peak moment measured occurred at the same joint angle for all groups. Further, it was found that the level of voluntary activation of the quadriceps and the co-activation of the antagonists did not contribute very much to the differences in strength of the different groups or cause any shift in the angle of peak moment for any of the groups.
The external angles of peak jointMVC moment were similar (~70°) in all groups. Consistent with previous findings (Arampatzis et al. 2004), the actual ‘internal’ joint angle during MVC was significantly lower than the external joint angle (Table 3). However, the resulting internal angle of peak jointMVC was ~60° for all groups, indicating that valid comparative conclusions would be drawn from measurements at a given external joint angle for both populations under the same experimental conditions.
Neither the voluntary activation level of the quadriceps muscle nor the co-activation of the antagonistic knee flexors changed with joint angle for any group. These findings are in line with previous reports on adult populations (antagonist co-activation, Baratta et al. 1988; Kellis and Baltzopoulos 1997; voluntary activation level, Newman et al. 2003; Bampouras et al. 2006), and resulted in no changes in the angle of peak moment (~60°) as a result of voluntary activation level and antagonist co-activation, either independently (joint100% and quadsMVC, respectively) or in combination (quads100%).
The current finding of similar jointMVC moment–angle relationships for adults and children contrasts the finding of Marginson and Eston (2001). These authors quantified the moment–angle relationship over a greater range of movement, while in the current study we were specifically concerned with identifying the angle of peak moment; therefore, we opted to take measurements at every 5° over a narrower range of movement around the estimated angle of peak torque, as opposed to the varying 10° or 20° steps used previously, which may have resulted in the true angle of peak moment going undetected. Nevertheless, the current finding of no differences in the angle of peak torque between children and adults was unexpected given the previous observations of Marginson and Eston. This finding raises questions over whether differences in tendon stiffness between adults and children (Kubo et al. 2001; Vogel 1983) alter the moment–angle relationship. Potentially the greater strength of adults may result in their stiffer tendon being stretched a similar amount to children’s, meaning that adults and children would experience similar absolute fascicle shortening during MVC. Alternatively differences between groups in muscle–tendon architecture (Zajac 1989) or the moment arm–knee angle relationship may contribute to produce similar moment–knee angle relationships. Future studies should consider these possibilities and determine the force-length properties of adult and child muscles.
The lack of any difference in the angle of peak moment for adults and children, and the fact that this pattern is not affected by different levels of voluntary activation or antagonist co-activation means that researchers and clinicians can test adults and children at the same joint angle and be confident that the comparative results obtained are valid. In interpreting the variation found in activation level with joint angle using the ITT method, differences in series elasticity at different muscle–tendon lengths should be taken into account, as discussed in detail recently (Bampouras et al. 2006). The approach we adopted for the quantification of changes in activation with joint angle was to use the CAR method, which is insensitive to changes in series elasticity because it does not incorporate resting twitches, but normalise the CAR value at any given joint angle to the ITT estimate at a joint angle where series slackness is minimal, thus bypassing the technical limitations of both methods when applied independently.
The typical pattern of strength differences between genders and ages has also been observed here, with men being significantly stronger than women and both adult groups being significantly stronger than children, but no difference was found between the strength of pre-pubertal boys and girls (Marginson and Eston 2001; Round et al. 1999). Correcting the jointMVC moment for antagonist co-activation resulted in significantly greater peak joint100% moment for each group. Although there were differences between the absolute antagonist moments of each group, relative to the quadsMVC moment the antagonist moments experienced by each group were similar. This indicates that differences in activation and co-activation make only a minor contribution to the differences in jointMVC between adults and children. Similarly, correcting for the level of voluntary activation level also resulted in significant increases in peak moment for each group. On the contrary, however, the level of voluntary activation did differ between adults and children, with the lower activation levels in children resulting in greater increases from jointMVC to joint100% moment compared to the adults. For instance, the jointMVC moment for boys was 29.3% of that for men, but correcting for activation level resulted in the joint100% moment for boys being 33.9% of that for men. Similarly, the jointMVC moment for the girls was 51.1% of that for women, but the joint100% moment for the girls was 64.2% of that for women (Table 2). Although the difference in the peak joint100% moment between adults and children was reduced by accounting for activation level, the typical pattern of men being stronger than women, who in turn were stronger than either of the child groups, remained. This was also the case when considering quads100% which included the relatively similar antagonist moments for each group. The remaining differences between the jointMVC of adults and children is most likely due to the increased size of adult muscle, allowing a greater number of sarcomeres in parallel, which results in a higher force-producing potential (Gans and Bock 1965).
In conclusion, the knee joint angle at which peak knee extension moment occurs is not different between adults and children of either gender. Although the ability of adults to develop higher activation levels explains some of the increase in the magnitude of peak knee extension moment with maturation, neither agonist voluntary activation level nor the antagonist co-activation level change with knee joint angle in the range 55–90° for adults or children of either gender.
References
Ahmad CS, Clark AM, Heilmann N, Schoeb JS, Gardner TR, Levine WN (2006) Effect of gender and maturity on quadriceps-to-hamstring strength ratio and anterior cruciate ligament laxity. Am J Sports Med 34:370–374. doi:10.1177/0363546505280426
Arampatzis A, Karamanidis K, De Monte G, Stafilidis S, Morey-Klapsing G, Brüggemann G (2004) Differences between measured and resultant joint moments during voluntary and artificially elicited isometric knee extension contractions. Clin Biomech (Bristol, Avon) 19:277–283. doi:10.1016/j.clinbiomech.2003.11.011
Baltzopoulos V, Kellis E (1998) Isokinetic strength during childhood and adolescence. In: Van Praagh E (ed) Pediatric anaerobic performance. Human Kinetics, Champaign, IL, pp 225–240
Bampouras TM, Reeves ND, Baltzopoulos V, Maganaris CN (2006) Muscle activation assessment: effects of method, stimulus number, and joint angle. Muscle Nerve 34:740–746. doi:10.1002/mus.20610
Baratta R, Solomonow M, Zhou BH, Letson D, Chuinard R, D’Ambrosia R (1988) Muscular coactivation. The role of the antagonist musculature in maintaining knee stability. Am J Sports Med 16:113–122. doi:10.1177/036354658801600205
Bassa E, Patikas D, Kotzamanidis C (2005) Activation of antagonist knee muscles during isokinetic efforts in prepubertal and adult males. pediatric exercise science, pp 171–181 (total no. of pages 111)
Behm D, Power K, Drinkwater E (2001) Comparison of interpolation and central activation ratios as measures of muscle inactivation. Muscle Nerve 24:925–934. doi:10.1002/mus.1090
Belanger AY, McComas AJ (1989) Contractile properties of human skeletal muscle in childhood and adolescence. Eur J Appl Physiol Occup Physiol 58:563–567. doi:10.1007/BF00418500
Gans C, Bock W (1965) The functional significance of muscle architecture: a theoretical analysis. Ergeb Anat Entwicklungsgesch 38:115–142
Kanehisa H, Ikegawa S, Tsunoda N, Fukunaga T (1995) Strength and cross-sectional areas of reciprocal muscle groups in the upper arm and thigh during adolescence. Int J Sports Med 16:54–60. doi:10.1055/s-2007-972964
Kellis E, Baltzopoulos V (1997) The effects of antagonist moment on the resultant knee joint moment during isokinetic testing of the knee extensors. Eur J Appl Physiol Occup Physiol 76:253–259. doi:10.1007/s004210050244
Kellis E, Unnithan VB (1999) Co-activation of vastus lateralis and biceps femoris muscles in pubertal children and adults. Eur J Appl Physiol Occup Physiol 79:504–511. doi:10.1007/s004210050545
Kubo K, Kanehisa H, Kawakami Y, Fukanaga T (2001) Growth changes in the elastic properties of human tendon structures. Int J Sports Med 22:138–143. doi:10.1055/s-2001-11337
Marginson V, Eston R (2001) The relationship between torque and joint angle during knee extension in boys and men. J Sports Sci 19:875–880. doi:10.1080/026404101753113822
Newman SA, Jones G, Newham DJ (2003) Quadriceps voluntary activation at different joint angles measured by two stimulation techniques. Eur J Appl Physiol 89:496–499. doi:10.1007/s00421-003-0836-0
O’Brien T, Reeves N, Baltzopoulos V, Jones D, Maganaris C (2008) Assessment of voluntary muscle activation using magnetic stimulation. Eur J Appl Physiol 104:49–55. doi:10.1007/s00421-008-0782-y
Paasuke M, Ereline J, Gapeyeva H (2000) Twitch contraction properties of plantar flexor muscles in pre- and post-pubertal boys and men. Eur J Appl Physiol 82:459–464. doi:10.1007/s004210000236
Parker DF, Round JM, Sacco P, Jones DA (1990) A cross-sectional survey of upper and lower limb strength in boys and girls during childhood and adolescence. Ann Hum Biol 17:199–211. doi:10.1080/03014469000000962
Reeves ND, Maganaris CN, Narici MV (2003) Effect of strength training on human patella tendon mechanical properties of older individuals. J Physiol 548:971–981
Reeves ND, Narici MV, Maganaris CN (2004) Effect of resistance training on skeletal muscle-specific force in elderly humans. J Appl Physiol 96:885–892. doi:10.1152/japplphysiol.00688.2003
Round JM, Jones DA, Honour JW, Nevill AM (1999) Hormonal factors in the development of differences in strength between boys and girls during adolescence: a longitudinal study. Ann Hum Biol 26:49–62. doi:10.1080/030144699282976
Tanner JM (1962) Growth at adolescence. Blackwell Scientific Publications, London
Vogel HG (1983) Age dependence of mechanical properties of rat tail tendons (hysteresis experiments). Aktuelle Gerontol 13:22–27
Wood LE, Dixon S, Grant C, Armstrong N (2004) Elbow flexion and extension strength relative to body or muscle size in children. Med Sci Sports Exerc 36:1977–1984. doi:10.1249/01.MSS.0000145453.02598.7E
Zajac FE (1989) Muscle and tendon: properties, models, scaling, and application to biomechanics and motor control. Crit Rev Biomed Eng 17:359–411
Author information
Authors and Affiliations
Corresponding author
Rights and permissions
About this article
Cite this article
O’Brien, T.D., Reeves, N.D., Baltzopoulos, V. et al. The effects of agonist and antagonist muscle activation on the knee extension moment–angle relationship in adults and children. Eur J Appl Physiol 106, 849–856 (2009). https://doi.org/10.1007/s00421-009-1088-4
Accepted:
Published:
Issue Date:
DOI: https://doi.org/10.1007/s00421-009-1088-4