Abstract
River discharge supplies nearshore communities with a terrestrial carbon source that is often reflected in invertebrate and fish consumers. Recent studies in the Beaufort Sea have documented widespread terrestrial carbon use among invertebrates, but only limited use among nearshore fish consumers. Here, we examine the carbon source and diet of rapidly growing young-of-year Arctic cisco (Coregonus autumnalis) using stable isotope values (δ13C and δ15N) from muscle and diet analysis (stomach contents) during a critical and previously unsampled life stage. Stable isotope values (δ15N and δ13C) may differentiate between terrestrial and marine sources and integrate over longer time frames (weeks). Diet analysis provides species-specific information, but only from recent foraging (days). Average δ13C for all individuals was −25.7 ‰, with the smallest individuals possessing significantly depleted δ13C values indicative of a stronger reliance of terrestrial carbon sources as compared to larger individuals. Average δ15N for all individuals was 10.4 ‰, with little variation among individuals. As fish length increased, the proportion of offshore Calanus prey and neritic Mysis prey increased. Rapid young-of-year growth in Arctic cisco appears to use terrestrial carbon sources obtained by consuming a mixture of neritic and offshore zooplankton. Shifts in the magnitude or phenology of river discharge and the delivery of terrestrial carbon may alter the ecology of nearshore fish consumers.
Similar content being viewed by others
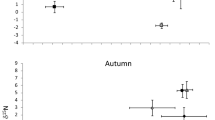
Explore related subjects
Discover the latest articles, news and stories from top researchers in related subjects.Avoid common mistakes on your manuscript.
Introduction
River discharge supplies nearshore marine communities with a carbon source that may influence ecosystem and fish production (Caddy 2000; Salen-Picard et al. 2002; Darnaude 2005; Dunton et al. 2006; Wrona et al. 2006; Vinagre et al. 2011b). The influence of terrestrial carbon on ecosystems and fisheries has been most extensively examined in relatively warm, eutrophic seas such as the Gulf of Mexico and Mediterranean Sea (Caddy 2000; Salen-Picard et al. 2002; Schlacher et al. 2008). In cool, oligotrophic Arctic seas, terrestrial carbon may also play an important role in production and understanding the current fate of terrestrial carbon in Arctic ecosystems is useful for predicting shifts as carbon dynamics are altered under climate change (Wrona et al. 2006; Dunton et al. 2012).
Arctic seas are generally oligotrophic and seasonally receive large amounts of organic carbon from rivers (Dittmar and Kattner 2003; Emmerton et al. 2008; Holmes et al. 2008). Carbon exported during the spring freshet is more biologically available than previously thought due to limited microbial use during the cold, short residence time in rivers and flood plains (Dittmar and Kattner 2003; Holmes et al. 2008). Recently, the presence of terrestrial carbon in the Beaufort Sea food web was confirmed across several taxa of benthic invertebrates using carbon isotope ratios (Dunton et al. 2006, 2012). Fewer nearshore fish species have been sampled and generally show less terrestrial carbon use (Kline et al. 1998; Dunton et al. 2006, 2012).
A lack of terrestrial carbon use was particularly surprising among anadromous and amphidromous fish associated with the band of brackish nearshore water, such as Arctic cisco (Coregonus autumnalis) (Craig 1989; Jarvela and Thorsteinson 1999). Differences in carbon sources by life stage have been noted for other species (Darnaude 2005), and the possibility that more Beaufort Sea fish species rely on terrestrial carbon at sea exists. To date, sampling has not included young-of-year (YOY) fish, a critical life stage when fish grow rapidly reaching 25–40 % of adult length (von Biela et al. 2011).
Arctic cisco are the focal species of an active and culturally important subsistence fishery in a region without commercial fishing (Fechhelm et al. 2007). Juveniles forage in the nearshore Beaufort Sea in Canadian and Alaskan waters, but spawning likely occurs throughout the Mackenzie River drainage, Northwest Territories, Canada, with some juveniles migrating to Alaska shortly after hatching (Craig 1989; Fechhelm and Griffiths 1990; Zimmerman et al. submitted). Terrestrial carbon use during the YOY life stage has been implied by significant correlations between YOY growth and river discharge (von Biela et al. 2011).
Here, we examine the food web structure of YOY Arctic cisco using stable isotope values (δ13C and δ15N) to assess carbon and nitrogen sources and stomach content analysis to identify key prey species. We hypothesized that the δ13C and δ15N values of YOY Arctic cisco would reflect a reliance on terrestrially derived carbon as opposed to a more immediate and complete reliance on marine-derived carbon (Kline et al. 1998; Darnaude 2005; Vinagre et al. 2011b). In addition, we hypothesized that stomach content analysis would identify species with life cycles of >1 year that could be responsible for the length of the lagged correlation between river discharge and YOY growth as demonstrated by von Biela et al. (2011).
Materials and methods
All YOY Arctic cisco were collected in August 2009 during annual monitoring of the YOY migration near Prudhoe Bay, Alaska, using nearshore fyke nets in water depths of ≤2 m (sites 214, 218, 220, and 230 from Fig. 1 in Fechhelm et al. 1999; Fig. 1). Arctic cisco were classified as YOY if fork length was <90 mm (Fechhelm et al. 2007). Fish were immediately frozen at capture and shipped to the USGS Alaska Science Center in Anchorage. Fish were thawed and fork length was measured to the nearest millimeter. An epaxial muscle sample was removed from each fish and frozen for stable isotope analysis. The cardiac and pyloric sections of the stomach were removed and weighed before and after stomach content removal to the nearest 0.001 g. All prey items were fixed in 10 % formalin for several weeks and later preserved in a 70 % ethanol solution. Prey items were identified to the lowest taxonomic level possible and counted under a dissection microscope. For each taxonomic group, a voucher specimen was collected for reference.
Epaxial muscle samples were freeze dried and subsampled for stable isotope analysis at the University of Alaska Anchorage, ENRI Stable Isotope Laboratory. Each sample was analyzed for δ13C (ratio of 13C/12C) and δ15N (ratio of 15N/14N) using a Costech 4010 Elemental analyzer (Costech Analytical, Valencia, CA) interfaced with a Thermo-Finnigan Delta Plus XP continuous-flow stable isotope ratio mass spectrometer (Thermo Electron Corp., Waltham, MA, USA). Stable isotope ratios were reported using standard delta notation. Lipids were not extracted prior to analysis as Arctic cisco muscle has minimal lipid content (<5 %) and terrestrial and marine nutrient sources have sufficiently different end members so that lipid correction is not critical to interpretation (Post et al. 2007; Logan et al. 2008; Tomy et al. 2009). Differences in stable isotope ratios between fish from different length classes are likely independent of body size per se and reflect shifts in diet (Vinagre et al. 2011a).
Stable isotope values were interpreted using terrestrial and marine carbon end member values for suspended particulate organic matter (SPOM) and benthic particulate organic matter (BPOM) from terrestrial (river) and marine (shelf) ecosystems in the eastern Beaufort Sea and typical literature fractionation coefficients. In this system, the δ13C of marine SPOM is enriched compared with terrestrial SPOM, but BPOM is similar between sources (Dunton et al. 2012). Nitrogen isotopes (δ15N) also differ between marine and terrestrial producers with nitrogen ratios of marine producers exceeding those of terrestrial producers (Dunton et al. 2006). Carbon fractionation was assumed to be ~1 ‰, nitrogen fractionation was assumed to be ~3.4 ‰ (Vander Zanden and Rasmussen 2001; Post 2002; Dunton et al. 2012), and a trophic level of 3 (i.e., secondary consumer) for YOY Arctic cisco was anticipated based on a diet of zooplankton (Dunton et al. 2012). Under these assumptions, predicted isotope ratios for secondary consumers were calculated and compared with isotope ratios of YOY Arctic cisco.
Key prey types were defined as any prey type that occurred in at least 10 % of samples. Prey composition was described by the relative abundance of each key prey type and the relative mass of each key prey type. The average wet weight for each species was based on literature values or calculated from a stomach that held only 1 prey type and used to calculate the relative contribution by mass (Table 1). The relative abundance and mass of each prey type was compared between length classes using a one-way analysis of variance (ANOVA) with an arcsine transformation for proportions and post hoc pairwise two-sample t-tests. Stable isotope ratios were not obtained for zooplankton prey types because they may have been influenced by preservation and gut contents (Feuchtmayr and Grey 2003). All fish were categorized into 10-mm-length bins for analysis of stable isotope ratios and stomach contents. Each stable isotope ratio was compared between length groups using an ANOVA with a significance of P < 0.05 and post hoc pairwise t-tests between groups. Stable isotope ratios were also compared between the largest YOY fish here and values reported in Dunton et al. (2012) for age 1+ Arctic cisco using a t test.
Results
A total of 84 YOY Arctic cisco were captured and ranged in fork length from 37 to 79 mm. Individuals were divided into four length classes: <50 mm (n = 5), 50–59 mm (n = 43), 60–69 mm (n = 24), and 70–79 mm (n = 12). Both carbon and nitrogen stable isotope values differed by length class (carbon F = 3.60, df = 3, P < 0.05; nitrogen F = 3.90, df = 3, P < 0.05; Fig. 2; Table 2). Fish from the smallest length class (FL < 50 mm) had significantly depleted δ13C with a mean of −27.3 ‰ compared with −25.7 ‰ for all individuals ≥50 mm (P < 0.05). Overall, the δ13C values of YOY Arctic cisco indicate that terrestrial SPOM was the most likely carbon source (Fig. 2). The δ15N values were also lower in smaller fish, with the 50–59 mm length class averaging 10.2 ‰ compared with 10.7 ‰ in the 60–69 mm length class and 10.8 ‰ in the 70–79 mm length class. The δ15N values of the <50 mm length class were not significantly different from any other class and had an average of 10.2 ‰. Compared with published values of δ13C and δ15N from older age 1+ Arctic cisco reported in Dunton et al. (2012), carbon was significantly depleted (t = 3.37, P < 0.05) and nitrogen was similar (t = 1.82, P > 0.05) in larger YOY fish (60–79 mm). Isotope values for fish from each length class were plotted with terrestrial and marine SPOM and BPOM sources from published studies and predicted values for secondary consumers reliant on each source (Fig. 2).
Carbon and nitrogen stable isotope values of YOY Arctic cisco captured in Prudhoe Bay, Alaska, by length classes plotted with published end member benthic particulate organic matter (BPOM) and suspended particulate organic matter (SPOM) values from the Beaufort Sea and values from age 1+ Arctic cisco. Solid black mixing lines begin at each organic matter source and terminate at the predicted value for a secondary consumer assuming isotope fractionations of 3.4 and 1.0 ‰ per trophic level for nitrogen and carbon, respectively. Length classes are denoted as follows: A Fish <50 mm fork length, B fish 50–59 mm fork length, C fish 60–69 mm fork length, D fish 70–79 mm fork length. Individuals from length class C and D did not differ significant in their carbon or nitrogen values and were grouped together in this plot. Symbol denotes data source (dagger) Dunton et al. 2012. Error bars denote standard error
Diet items were most often identified to genus as species level identification was not always possible. Prey groups represented in at least 10 % of samples were Pseudocalanus (76 %), Calanus (49 %), Eurytemora (76 %), Acartia (54 %), Metridia (19 %), Mysis (26 %), Harpacticoids (30 %), and Gammarids (18 %). Although nematodes were present in 64 % of fish stomachs in low numbers (average of 1.7 nematodes/stomach), they were not considered a prey item because they were likely parasitic (Stewart and Bernier 1999). More than half of the Calanus prey items were identified to species (62 %) and showed a nearly equal ratio between the frequency of C. hyperboreus (52 %) and C. glacialis (48 %). An equal ratio was assumed to calculate an average wet weight for the Calanus prey category in combination with literature values for each species. Other prey items that occurred in less than 10 % of samples were Diptera (9 %), Oncaea spp. (4 %), Centropages abdominalis (4 %), Oithona spp. (2 %), isopods (1 %), Heterorhabdus tanneri (1 %), and Tortanus discaudatus (1 %).
Diet differed by length class, with larger fish consuming a greater proportion of Calanus and Mysis prey by number and mass compared with smaller fish. The relative number of Calanus prey items was higher for fish in the 70–79 mm length class compared with fish in either the <50 mm and 50–59 mm length classes (F = 3.05, df = 3, P < 0.05, Fig. 3). By mass, a higher proportion of Calanus prey were consumed by fish in the 70–79 and 60–69 mm length classes compared with either the 50–59 mm and <50 mm length classes (F = 7.09, df = 3, P < 0.001, Fig. 4). The proportion of Mysis prey by mass was higher among fish from the 70–79 mm length class compared with all other length classes (F = 3.20, df = 3, P < 0.05, Fig. 4).
The relative numeric composition of key diet items in YOY Arctic cisco in four length classes: a Fish <50 mm fork length, b fish 50–59 mm fork length, c fish 60–69 mm fork length, d fish 70–79 mm fork length. The relative number of Calanus prey items was higher for fish in the 70–79 mm length class compared with fish in either the <50 mm and 50–59 mm length classes (F = 3.05, df = 3, P < 0.05)
The relative composition by mass of key diet items in YOY Arctic cisco in four length classes: a Fish <50 mm fork length, b fish 50–59 mm fork length, c fish 60–69 mm fork length, d fish 70–79 mm fork length. By mass, a higher proportion of Calanus prey were consumed by fish in the 70–79 and 60–69 mm length classes compared with either the 50–59 mm and <50 mm length classes (F = 7.09, df = 3, P < 0.001). The proportion of Mysis prey by mass was higher among fish from the 70–79 mm length class compared with all other length classes (F = 3.20, df = 3, P < 0.05)
Discussion
In contrast to other studies with older Arctic cisco using marine carbon sources, YOY Arctic cisco in this study used terrestrial carbon sources that are obtained through a mixture of neritic and offshore zooplankton prey (Kline et al. 1998; Dunton et al. 2006, 2012). The δ13C values from most YOY Arctic cisco were in the expected range of a secondary consumer relying on terrestrial suspended carbon sources (SPOM), but not marine sources (Fig. 2). Isotope values showed a tendency for the smaller fish to rely more heavily on terrestrial carbon sources and feed at a slightly lower trophic level, but there was evidence of terrestrial carbon use in fish of all length classes (Table 2; Fig. 2). Carbon isotope values of the smallest fish (<50 mm) were more depleted than expected for a secondary consumer using any of the potential carbon sources examined here, but still the terrestrial SPOM source was the most likely carbon source.
Terrestrial carbon has previously been recognized for its importance in the nearshore Beaufort Sea food web and other nearshore areas (Carmack and Macdonald 2002; Darnaude 2005; Dunton et al. 2006; Vinagre et al. 2011b, c; Dunton et al. 2012). When combined with an understanding of low in situ marine production in the Beaufort Sea, the presence of terrestrial carbon sources in many species suggests that nearshore production is dependent on allochthonous terrestrial inputs from river discharge and coastal erosion (Dunton et al. 2006). Arctic cisco, and other species that use terrestrial carbon, can be influenced by terrestrial processes even while at sea if river discharge controls carbon export from coastal plains, foothills, and headwater regions (Holmes et al. 2008; Vinagre et al. 2011c). Changing climates may also influence terrestrial carbon inputs and nearshore marine production by altering the timing and magnitude of river discharge and erosion rates. Interannual differences in YOY Arctic cisco growth have been strongly correlated with Mackenzie River discharge over the last several decades, and the results from this study provide evidence of the underlying mechanism leading to these observed correlations (von Biela et al. 2011).
Other fish species also show variation in carbon source with life stage or feeding location (Darnaude 2005; Dunton et al. 2006; Vinagre et al. 2011b). Near the Rhone River, France, Darnaude (2005) found shifts in carbon sources between juvenile and adult flatfish due to differences in diet and depth distribution with age. Yellow sole (Buglossidium luteum) adults primarily use terrestrial carbon and juveniles primarily use marine carbon, while the pattern is opposite in sand sole (Pegusa lascaris). Near the Tagus River, Portugal, some fish species exhibit a gradient in carbon sources with fish captured closer to the river using more terrestrial carbon than fish captured further from the river (Vinagre et al. 2011b). In the Beaufort Sea, Dunton et al. (2006) found Arctic cod (Boreogadus saida) captured in lagoons use more terrestrial carbon compared with those captured on the open coastal shelf. Future research should address the influence of river discharge and terrestrial carbon availability on life history traits of marine and anadromous fish living in river-influenced marine habitats.
Nitrogen isotope values should be interpreted with more caution than carbon isotope values in this study. Nitrogen end member values may vary more widely across seasons, the fractionation values for nitrogen are known to have wider variability, and differences due to nitrogen source can be confounded with differences in trophic level (Kling et al. 1992; Post 2002; Vizzini and Mazzola 2003; Tamelander et al. 2009; Dunton et al. 2012). The δ15N values of YOY Arctic cisco, however, were within 3.4 ‰ or one trophic level of the expected value for a secondary consumer of terrestrial SPOM and similar to those of age 1+ Arctic cisco (Kline et al. 1998; Dunton et al. 2012; Fig. 2).
Terrestrial isotope signatures of YOY Arctic cisco likely reflect recent foraging in the Beaufort Sea, rather than past freshwater residency after hatching. In general, YOY fish are known to have much faster carbon turnover compared with adults due to their rapid growth rates. In a whole-lake manipulation of δ13C, the half-life of carbon isotopes ranged from 8 to 18 days for YOY bluegill (Lepomis macrochirus), largemouth bass (Micropterus salmoides), and yellow perch (Perca flavescens) compared with more than 100 days for adults (Weidel et al. 2011). Even among adults, Perga and Gerdeaux (2005) found that the stable isotope composition of whitefish (C. lavaretus) muscle tissue reflected the food consumed during the growing season regardless of collection date. At the time of capture, YOY Arctic cisco had likely been in nearshore waters for a month or more and spent little time in freshwater after hatching. Migration time to Prudhoe Bay from the Mackenzie River delta has been estimated at 35 days (Gallaway et al. 1983; Fechhelm and Griffiths 1990; Dillinger et al. 1992; Colonell and Gallaway 1997). Hatch dates are thought to coincide with spring freshet and are quickly followed by downstream migration as in other coregonids (Naesje et al. 1986, 1995; Urpanen et al. 2005). Given the quick turnover rates for stable isotopes in YOY fish, long migration in nearshore water, and short freshwater residency, it is likely that isotope signatures only reflect nearshore foraging.
Diet composition of YOY Arctic cisco depended on fish length. The proportion of Calanus and Mysis prey items increased with length class. Calanus prey was not found in the stomachs of the smallest fish (<50 mm) and gradually increased to 26 % of prey items and 55 % of prey mass in the largest fish sampled (70–79 mm). In the largest fish, Calanus were the second most numerically dominate prey class and primary prey class by mass (Figs. 3, 4). Similarly, Mysis prey did not occur in the stomach of the smallest fish sampled (<50 mm), but accounted for 12 % of prey items and 30 % of prey mass in the largest fish sampled (70–79 mm) (Fig. 3, 4). There were no statistically significant declines in prey species with increasing length class, but the contribution of Pseduocalanus and Harpacticoid prey items tended to decrease with fish length (Fig. 3, 4).
Differences in diet composition by fish of different size can be driven by extrinsic or intrinsic factors. If fish of different length classes live in separate microhabitats within the nearshore Beaufort Sea, different encounter rates could exist for individual prey groups and the differences would be extrinsic. These fish, however, were all captured in the same nearshore fyke nets, and there was no evidence of separation by size at capture. An intrinsic limitation is the most likely mechanism leading to difference in prey composition by fish length class. Smaller YOY Arctic cisco are likely unable to consume the larger Calanus and Mysis prey due to gape limitations and might also have reduced abilities to detect or capture larger prey (Bremigan and Stein 1994; Romare et al. 1999). The Calanus and Mysis prey were by far the largest species consumed by YOY Arctic cisco in this study (Table 1).
Many prey groups identified in this study were consistent with past zooplankton distribution studies in nearshore Arctic waters. Two Mysis species, M. litoralis and M. relicta, are common epibenthic species in the nearshore brackish waters that have been suggested as important prey items for nearshore fish communities (Jarvela and Thorsteinson 1999) and are known to have longer life cycles (1, 2, or even 4 years; reviewed in Audzijonytė and Väinölä 2005). Pseudocalanus, Eurytemora, Acartia, and Harpacticoid prey items have previously been identified in nearshore zooplankton studies within the Beaufort Sea and specifically within the low-salinity waters of the Mackenzie River plume and Prudhoe Bay (Horner and Murphy 1985; Walkusz et al. 2010). Any of these nearshore prey items could be providing a conduit for terrestrial carbon to YOY Arctic cisco.
Calanus in the diet of YOY Arctic cisco were inconsistent with their typical distribution in offshore marine waters. Although Calanus species are not characteristic of nearshore brackish waters where YOY Arctic cisco are typically found, they do occur at low densities in nearshore waters (Horner and Murphy 1985; Walkusz et al. 2010). Our sampling is unable to determine whether Calanus species became available to YOY Arctic cisco in nearshore waters or whether Arctic cisco were able to move offshore to forage for Calanus. It is more likely that Calanus prey became available in the nearshore waters since nearshore Mysis prey were found in the same stomachs and all fish were captured nearshore. Of the YOY Arctic cisco consuming Mysis prey, nearly all (91 %) consumed Calanus prey as well. It is unlikely that YOY Arctic cisco moved offshore to forage on Calanus prey and later returned to the nearshore to forage on Mysis prey prior to nearshore capture. Summer storms could bring Calanus prey into nearshore waters in greater numbers, or YOY Arctic cisco may preferentially select Calanus prey for their large body size and high lipid densities that can reach 74 % of dry weight at the end of the summer (Bradstreet and Cross 1982; Hirche 1997; Scott et al. 2000; Peterson et al. 2002; Prokopowicz and Fortier 2002; Dunton et al. 2012). The large body size and high lipid densities of Calanus prey are made possibly by a longer life cycle of several years (2–3 years for C. glacialis in the Beaufort Sea and 2–4 years for C. hyperboreus) and represents an adaptation to the short Arctic growing season where individuals use resources gathered over several summers to attain larger size and ultimately higher fecundity (Hirche 1997; Wold et al. 2011).
We have previously suggested that YOY Arctic cisco may rely on longer-lived copepods, like Calanus spp. and that the life cycle of these copepods would account for the 1- and 2-year lags present in the relationships between YOY Arctic cisco growth and environmental predictors of production (von Biela et al. 2011). Here, we have shown that YOY Arctic cisco do forage on long-lived Calanus copepods and mysids. Further study is required to determine whether YOY Arctic cisco preferentially select these larger, longer-lived prey items and whether they are consistently present in YOY Arctic cisco diets across years and throughout the Beaufort Sea.
Here, we have demonstrated that Arctic cisco lie at a cross roads between terrestrial and marine ecosystems and are vulnerable to change in either system. Arctic cisco and other nearshore fish could be important indicators for the Beaufort Sea in the face of a changing climate and increased development. Future climate predictions for the Beaufort Sea were recently summarized by Walkusz et al. (2010) and include increased Mackenzie River discharge from increasing precipitation and thawing permafrost (Carmack and Macdonald 2002). Under this scenario, a resulting increase in nutrients and decrease in light penetration are anticipated (Wrona et al. 2006; Retamal et al. 2008). In the event that light does not become limiting to production, changes in the phenology and increases in the magnitude of phytoplankton production may lead to the replacement of large copepods, such as Calanus, by smaller copepods (Uye 1994). Substituting large lipid-rich species, with lower quality prey may have cascading effects for Beaufort Sea food webs. A similar process has already been identified in the Bering Sea under the Oscillating Control Hypothesis, where warm years are associated with decreased production of large zooplankton and reduced survival of young pollock (Theragra chalcogramma) (Hunt et al. 2011). Our study of Arctic cisco may also provide insight into other high profile Arctic species such as bowhead whales Balaena mysticetus L. and Arctic cod which also feed heavily on Calanus and Mysis prey items (Lowry and Burns 1979; Bradstreet and Cross 1982).
References
Audzijonytė A, Väinölä R (2005) Diversity and distributions of circumpolar fresh- and brackish-water Mysis (Crustacea: Mysida): Descriptions of M. relicta Lovén, 1862, M. salemaai n. sp., M. segerstralei n. sp. and M. diluviana n. sp., based on molecular and morphological characters. Hydrobiologia. doi:10.1007/s10750-004-8337-7
Bogorov BG (1959) On the standardisation of marine plankton investigations. Int Revue Gesamten Hydrobiol Hydrogr. doi:10.1002/iroh.19590440135
Bradstreet MSW, Cross WE (1982) Trophic relationships at high Arctic ice edges. Arctic 35:1–12
Bremigan MT, Stein RA (1994) Gape-dependent larval foraging and zooplankton size: implications for fish recruitment across systems. Can J Fish Aquatic Sci. doi:10.1139/cjfas-51-4-913
Caddy JF (2000) Marine catchment basin effects versus impacts of fisheries on semi-enclosed seas. ICES J Mar Sci. doi:10.1006/jmsc.2000.0739
Carmack EC, Macdonald RW (2002) Oceanography of the Canadian Shelf of the Beaufort Sea: a setting for marine life. Arctic 55:29–45
Colonell JM, Gallaway BJ (1997) Wind-driven transport and dispersal of age-0 Arctic cisco along the Beaufort Sea coast. Am Fish Soc Symp 19:90–103
Craig PC (1989) An introduction to anadromous fishes of the Alaskan Arctic. Biol Pap Univ Alaska 24:27–54
Darnaude AM (2005) Fish ecology and terrestrial carbon use in coastal areas: implications for marine fish production. J Anim Ecol. doi:10.1111/j.1365-2656.2005.00978.x
Dillinger EEJ, Birt TP, Green JM (1992) Arctic cisco, Coregonus autumnalis, distribution, migration and spawning in the Mackenzie River. Can Field Nat 106:175–180
Dittmar T, Kattner G (2003) The biogeochemistry of the river and shelf ecosystem of the Arctic Ocean: a review. Mar Chem. doi:10.1016/S0304-4203(03)00105-1
Dunton K, Weingartner T, Carmack E (2006) The nearshore western Beaufort Sea ecosystem: circulation and importance of terrestrial carbon in arctic coastal food webs. Prog Oceanogr. doi:10.1016/j.pocean.2006.09.011
Dunton KH, Schonberg SV, Cooper LW (2012) Food web structure of the Alaskan nearshore shelf and estuarine lagoons of the Beaufort Sea. Estuar Coast. doi:10.1007/s12237-012-9475-1
Emmerton CA, Lesack LFW, Vincent WF (2008) Mackenzie River nutrient delivery to the Arctic Ocean and effects of the Mackenzie Delta during open water conditions. Global Biogeochem Cycles. doi:10.1029/2006GB002856
Fechhelm RG, Griffiths WB (1990) Effect of wind on the recruitment of Canadian Arctic cisco (Coregonus autumnalis) into the central Alaskan Beaufort Sea. Can J Fish Aquatic Sci. doi:10.1139/f90-241
Fechhelm RG, Martin LR, Gallaway BJ, Wilson WJ, Griffiths WB (1999) Prudhoe Bay causeways and the summer coastal movements of Arctic cisco and least cisco. Arctic 52:139–151
Fechhelm RG, Streever B, Gallaway BJ (2007) The Arctic cisco (Coregonus autumnalis) subsistence and commercial fisheries, Colville River, Alaska: a conceptual model. Arctic 60:421–429
Feuchtmayr H, Grey J (2003) Effect of preparation and preservation procedures on carbon and nitrogen stable isotope determinations from zooplankton. Rapid Commun Mass Spectrom. doi:10.1002/rcm.1227
Gallaway BJ, Griffiths WB, Craig PC, Gazey WJ, Helmericks JW (1983) An assessment of the Colville River delta stock of Arctic cisco—migrants from Canada? Biol Pap Univ Alaska 21:4–23
Gronvik S, Hopkins CCE (1984) Ecological investigations of the zooplankton community of Balsfjorden, Northern Norway: generation cycle, seasonal vertical distribution, and season variations in body weight and carbon and nitrogen content of the copepod Metridia longa. J Exp Mar Biol Ecol 80:93–107
Hirche J (1997) Life cycle of the copepod Calanus hyperboreus in the Greenland Sea. Mar Biol. doi:10.1007/BF00392894
Holmes RM, McClelland JW, Raymond PA, Frazer BB, Peterson BJ, Stieglitz M (2008) Liability of DOC transported by Alaskan rivers to the Arctic Ocean. Geophys Res Lett. doi:10.1029/2007GL032837
Hopky GE, Lawrence MJ, Chiperzak DB (1994) NOGAP B2: Zooplankton data from the Canadian Beaufort Sea Shelf, 1984 and 1985. Canadian Data Report of Fisheries and Aquatic Sciences 923
Horner R, Murphy D (1985) Species composition and abundance of zooplankton in the nearshore Beaufort Sea in winter-spring. Arctic 38:201–209
Hunt GL, Coyle KO, Eisner LB, Farley EV, Heintz RA, Mueter F, Napp JM, Overland JE, Ressler PH, Salo S, Stabeno PJ (2011) Climate impacts on eastern Bering Sea foodwebs: a synthesis of new data and an assessment of the Oscillating Control Hypothesis. ICES J Mar Sci. doi:10.1093/icesjms/fsr036
Jarvela LE, Thorsteinson LK (1999) The epipelagic fish community of Beaufort Sea coastal waters, Alaska. Arctic 52:80–94
Kline J, Thomas C, Wilson WJ, Goering JJ (1998) Natural isotope indicators of fish migration at Prudhoe Bay, Alaska. Can J Fish Aquatic Sci. doi:10.1139/cjfas-55-6-1494
Kling GW, Fry B, O’Brien WJ (1992) Stable isotopes and planktonic trophic structure in Arctic lakes. Ecology 73:561–566
Logan JM, Jardine TD, Miller TJ, Bunn SE, Cunjak RA, Lutcavage ME (2008) Lipid corrections in carbon and nitrogen stable isotope analyses: Comparison of chemical extraction and modeling methods. J Anim Ecol. doi:10.1111/j.1365-2656.2008.01394.x
Lowry LF, Burns JJ (1979) Foods utilized by bowhead whales near Barter Island, Alaska, autumn 1979. Mar Fish Rev 42:88–91
Naesje TF, Jonsson B, Sandlund OT (1986) Drift of cisco and whitefish larvae in a Norwegian River. Trans Am Fish Soc 115:89–93
Naesje TF, Jonsson B, Skurdal J (1995) Spring flood: a primary cue for hatching of river spawning Coregoninae. Can J Fish Aquatic Sci. doi:10.1139/f95-811
Perga ME, Gerdeaux D (2005) “Are fish what they eat” all year round? Oecologia doi:10.1007/s00442-005-0069-5
Peterson BJ, Holmes RM, McClelland JW, Vörösmarty CJ, Lammers RB, Shiklomanov AI, Rahmstorf S (2002) Increasing river discharge to the Arctic Ocean. Science. doi:10.1126/science.1077445
Post DM (2002) Using stable isotopes to estimate trophic position: Models, methods, and assumptions. Ecology. doi:10.2307/3071875
Post DM, Layman CA, Arrington DA, Takimoto G, Quattrochi J, Montaña CG (2007) Getting to the fat of the matter: models, methods and assumptions for dealing with lipids in stable isotope analyses. Oecologia. doi:10.1007/s00442-006-0630-x
Prokopowicz A, Fortier L (2002) Population structure of three dominant Calanus species in North Water Polynya, Baffin Bay. Pol Polar Res 23:241–252
Retamal L, Bonilla S, Vincent WF (2008) Optical gradients and phytoplankton production in the Mackenzie River and the coastal Beaufort Sea. Polar Biol. doi:10.1007/s00300-007-0365-0
Romare P, Bergman E, Hansson LA (1999) The impact of larval and juvenile fish on zooplankton and algal dynamics. Limnol Oceanogr. doi:10.4319/lo.1999.44.7.1655
Salen-Picard C, Darnaude A, Arlhac D, Harmelin-Vivien M (2002) Fluctuations of macrobenthic populations: a link between climate-driven river run-off and sole fishery yields in the Gulf of Lions. Oecologia. doi:10.1007/s00442-002-1032-3
Schlacher TA, Connolly RM, Skillington AJ, Gaston TF (2008) Can export of organic matter from estuaries support zooplankton in nearshore, marine plumes? Aquat Ecol. doi:10.1007/s10452-008-9196-5
Scott CL, Kwasniewski S, Falk-Petersen S, Sargent JR (2000) Lipids and life strategies of Calanus finmarchicus, Calanus glacialis and Calanus hyperboreus in late autumn, Kongsfjorden, Svalbard. Polar Biol. doi:10.1007/s003000000114
Stewart DB, Bernier LMJ (1999) Common parasites, diseases and injuries of freshwater fishes in the Northwest Territories and Nunavut. Prepared by Arctic Biological Consultants for the Canada Department of Fisheries and Oceans, Central and Arctic Region, Winnipeg
Tamelander T, Kivimäe C, Bellerby RGJ, Renaud PE, Kristiansen S (2009) Base-line variations in stable isotope values in an Arctic marine ecosystem: effects of carbon and nitrogen uptake by phytoplankton. Hydrobiologia. doi:10.1007/s10750-009-9780-2
Tomy GT, Pleskach K, Ferguson SH, Hare J, Stern G, MacInnis G, Marvin CH, Loseto L (2009) Trophodynamics of some PFCs and BFRs in a Western Canadian Arctic marine food web. Environ Sci Technol. doi:10.1021/es900162n
Urpanen O, Huuskonen H, Marjomäki TJ (2005) Growth and size-selective mortality of vendace (Coregonus albula (L.)) and whitefish (C. lavaretus (L.)) larvae. Boreal Environ Res 10:225–238
Uye S (1994) Replacement of large copepods by small ones with eutrophication of embayments: Cause and consequence. Hydrobiologia. doi:10.1007/BF00229979
Vander Zanden MJ, Rasmussen JB (2001) Variation in δ15N and δ13C trophic fractionation: implications for aquatic food web studies. Limnol Oceanogr 46:2061–2066
Vinagre C, Maguas C, Cabral HN, Costa MJ (2011a) Effect of body size and body mass on δ13C and δ15N in coastal fishes and cephalopods. Estuar Coast Shelf Sci. doi:10.1016/j.ecss.2011.08.006
Vinagre C, Maguas C, Cabral HN, Costa MJ (2011b) Nekton migration and feed location in a coastal area—a stable isotope approach. Estuar Coast Shelf Sci. doi:10.1016/j.ecss.2010.12.011
Vinagre C, Salgado J, Cabral HN, Costa MJ (2011c) Food web structures and habitat connectivity in fish estuarine nurseries—impact of river flow. Estuar Coasts. doi:10.1007/s12237-010-9315-0
Vizzini S, Mazzola A (2003) Seasonal variations in the stable carbon and nitrogen isotope ratios (13C/12C and 15N/14N) of primary producers and consumers in a western Mediterranean coastal lagoon. Mar Biol. doi:10.1007/s00227-003-1027-6
von Biela VR, Zimmerman CE, Moulton LL (2011) Long-term increases in young-of-the-year growth of Arctic cisco (Coregonus autumnalis) and environmental influences. J Fish Biol. doi:10.1111/j.1095-8649.2010.02832.x
Walkusz W, Paulić JE, Kwaśniewski S, Williams WJ, Wong S, Papst MH (2010) Distribution, diversity and biomass of summer zooplankton from the coastal Canadian Beaufort Sea. Polar Biol. doi:10.1007/s00300-009-0708-0
Weidel BC, Carpenter SR, Kitchell JF, Vander Zanden MJ (2011) Rates and components of carbon turnover in fish muscle: insights from bioenergetics models and a whole-lake 13C addition. Can J Fish Aquatic Sci. doi:10.1139/F10-158
Wold A, Darnis G, Søreide JE, Leu E, Philippe B, Fortier L, Poulin M, Kattner G, Graeve M, Falk-Petersen S (2011) Life strategy and diet of Calanus glacialis during the winter-spring transition in Amundsen Gulf, south-eastern Beaufort Sea. Polar Biol. doi:10.1007/s00300-011-1062-6
Wrona FJ, Prowse TD, Reist JD, Hobbie JE, Levesque LMJ, Vincent WF (2006) Climate impacts on Arctic freshwater ecosystems and fisheries: background, rationale and approach of the Arctic Climate Impact Assessment (ACIA). Ambio. doi:10.1579/0044-7447(2006)35[326:CIOAFE]2.0.CO;2
Zimmerman CE, Ramey AM, Turner SM, Mueter FJ, Murphy SM, Nielsen JL (Submitted to) Genetics, recruitment, and migration patterns of Coregonus autumnalis in the Colville River, Alaska and Mackenzie River, Canada. J Fish Biol
Acknowledgments
Sample collections were made available to our laboratory by R. Fechhelm at LGL Limited with the consent of B. Streever and BP under Fish Resource Permit CF-09-109 issued by the State of Alaska, Department of Fish and Game. Diet analyses were conducted by T. C. Stark at the University of Alaska Fairbanks and S. E. Burril at the U.S. Geological Survey Alaska Science Center. K. H. Dunton provided constructive comments on this manuscript. Funding was provided by the U.S. Geological Survey and the Bureau of Ocean Energy Management with the assistance of K. Wedemeyer. Support by NSF for the Environmental and Natural Resources Institute stable isotope facility was provided by MRI grant 0953271 awarded to JMW. Any use of trade names or products is for descriptive purposes only and does not imply endorsement of the US Government.
Author information
Authors and Affiliations
Corresponding author
Rights and permissions
About this article
Cite this article
von Biela, V.R., Zimmerman, C.E., Cohn, B.R. et al. Terrestrial and marine trophic pathways support young-of-year growth in a nearshore Arctic fish. Polar Biol 36, 137–146 (2013). https://doi.org/10.1007/s00300-012-1244-x
Received:
Revised:
Accepted:
Published:
Issue Date:
DOI: https://doi.org/10.1007/s00300-012-1244-x