Abstract
Water deficit is the most limiting factor for seed yield of crop species in the arid and semi-arid regions. Due to increasing limitation of fresh water resources and importance of safflower (Carthamus tinctorius L.) as a significant oilseed crop in Iran, it is necessary to evaluate physiological responses of drought tolerance and its association with seed yield of this crop in water stress condition. In this study, 21 safflower genotypes were planted by hand in the field under water stress and non-stress conditions in a randomized complete block design with two replications during 2 years (2016–17). The results indicated that water deficit stress significantly reduced relative water content (RWC), chlorophyll a (Chl-a) and chlorophyll b (Chl-b) concentrations and seed yield, but increased ascorbate peroxidase (APX) and peroxidase (POX) activities and leaf proline concentration. Significant differences were observed among the genotypes for all studied traits except Chl-b concentration. Genotypes were discriminated according to their response to drought using stress tolerance index (STI). Drought tolerant genotypes displayed a higher capability for accumulation of proline in association with maintaining RWC, antioxidant enzymes activity and higher seed yield, compared to the drought sensitive genotypes. Presented results suggested that safflower genotypes with higher levels of antioxidant enzymes activity, RWC and proline accumulation are characterized by a higher STI. Therefore, these physiological traits can be employed as effective criteria for selecting safflower genotypes with more tolerance to water deficit stress.
Similar content being viewed by others
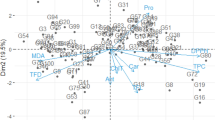
Avoid common mistakes on your manuscript.
Introduction
Drought stress severely affects plant growth and dramatically limits agricultural productivity (Fariaszewska et al. 2017; Gholami-Zali and Ehsanzadeh 2018b), especially in arid and semiarid regions of the world. Water deficit stress causes several physiological, biochemical, and molecular changes in plants resulting in modification of some metabolic pathways that may help plants to overcome injuries caused by osmotic stresses (Amini et al. 2014). Plants increase production of reactive oxygen species (ROS), and other toxic compounds under stress conditions which are highly reactive. These stress-induced metabolic products cause damage to proteins, lipids, DNA, chlorophyll and any other organic constituent of plants living cells, to the extent that these damages may bring about inactivation of the antioxidant defense system (Bouchemal et al. 2017).
To avoid ROS-induced cellular injuries, plants produce scavenging enzymes, such as superoxide dismutase, ascorbate peroxidase, catalase, and glutathione reductase and some low molecular weight non-enzyme antioxidants, such as carotenoids, proline, ascorbic acid and reduced glutathione (Sajedi et al. 2012; Sheikh-Mohammadi et al. 2017). There are some studies indicating that alleviation of oxidative damage and drought tolerance is strongly associated with a more efficient antioxidant system (Sajedi et al. 2012; Kadkhodaie et al. 2014). Carotenoids have an important role in preventing oxidative damage by quenching Chl triplets and single oxygen (Osorio et al. 2013). Proline plays a role in osmotic adjustment, acts as a free radical scavenger (Mitler 2002), and also is an alternative sink for energy to regulate the state of cellular redox (Sajedi et al. 2012). Therefore, it alleviates water deficit stress effects and increases water deficit stress tolerance in the plants. The relative water content (RWC) is considered as an important physiological index that refers to the degree of tissue and cell hydration that maintaining it is necessary for optimum physiological and biochemical activities and growth process in plants (Silva et al. 2007). It has been used as a suitable screening tool for plant water status; that reveals the stress intensity (Hassanzadeh et al. 2009). Water loss can decrease leaf water potential that cause a reduced turgor, stomatal conductance, photosynthesis, and consequently a lower seed yield (Amini et al. 2014).
High evapotranspiration and increasing limitation of water resources in arid and semi-arid regions has invoked the interests for improvement of drought-tolerant cultivars of native crop species to overcome the progressive water scarcity. Safflower (Carthamus tinctorius L.), a member of Asteraceae family, is one of the oldest cultivated crops that can relatively tolerate environmental stresses, including salinity and water stress. Furthermore, it may be grown in many areas of the world (Movahhedy-Dehnavy et al. 2009; Janmohammadi et al. 2017; Shahrokhnia and Sepaskhah 2017). It may be defined as a multipurpose crop because of its applications in producing industrial and edible oil, food coloring and flavoring, natural dyes, pharmaceuticals, poultry feeding and biofuels (Janmohammadi et al. 2017; Shahrokhnia and Sepaskhah 2017). Many studies have shown that drought stress caused a considerable reduction in seed yield of safflower (Sajedi et al. 2012; Singh et al. 2016; Shahrokhnia and Sepaskhah 2017), and this can be related to changes of the physiological traits. Therefore, the identification of physiological responses involved in drought tolerance and adopting of safflower genotypes to water stress condition can play an important role in breeding programs for developing drought tolerant cultivars of safflower. Therefore, the objective of present study was to investigate different physiological responses of safflower genotypes to water deficit stress and their relations with seed yield and drought-stress tolerance index under normal and water deficit conditions.
Materials and methods
Plant material, experimental set up and soil condition
Seeds of 21 safflower genotypes consisting of 19 Iranian breeding lines isolated from different local populations and two Canadian cultivars (Table 1) were evaluated in two separate experiments under a full irrigation (normal) and a deficient irrigation regime (water deficit stress) using a randomized complete block design with two replications in 2016 and 2017. The experiments were carried out at the Research Farm of Isfahan University of Technology, Isfahan, Iran (located in 40 km south-west of Isfahan, 32°32′ N, 51°23′ E, 1630 m a.m.s.l., with 14.5 °C mean annual temperature, and 140 mm mean annual precipitation), on a Typic Haplargid, clay loam soil. The soil contains 380 g/kg Ca-carbonate equivalent, 5.0 g/kg organic C, and 0.75 g/kg total N, with pH 7.5 and electrical conductivity 1.6 dS/m. The minimum and maximum mean air temperatures in the region across the growing season were 15.5 and 30.8 °C, respectively in 2016, and 15.8 and 30.1 °C, respectively in 2017. Total precipitation during growing seasons in years of 2016 and 2017 were 39.2 and 22.9 mm, respectively.
In each replication, seeds of the genotypes were planted by hand in 7 March 2016 and 5 March 2017. Each plot consisted of three rows, 2 m in length and 50 cm apart. The plants were spaced 10 cm apart within the rows. For the measurement of physiological traits, leaves were collected at the 50% flowering stage and then washed, frozen and stored using liquid N2 at − 80 °C for further analysis.
Irrigation regimes
From sowing to budding stage all experimental plots were properly irrigated. Irrigation treatments were applied at budding stage and continued to physiological maturity. In normal and water deficit stress irrigation conditions, plants were irrigated when 50% and 85% of the total available water was depleted from the root zone, respectively (Allen et al. 1998). For prediction of soil moisture depletion, daily evapotranspiration of plant (ETact) was calculated based on the climatic data and the United Nation,s Food and Agriculture Organizations (FAO) Penman–Monteith equation (Allen et al. 1998) as follows:
where \({\text{ET}}_{ \circ }\) is reference evapotranspiration (based on daily climatic data), KMC is the microclimate coefficient, and ETact is daily evapotranspiration of plant. When ΣETact reached to water allowable depletion depth (Dirrig), it was the irrigation time for the two irrigation levels. The water allowable depletion depth was calculated using the following formula:
where D was the soil column depth (cm), θFC was the volumetric soil water content at field capacity (%), θPWP was the volumetric soil water content at wilting point (%) and MAD was maximum allowable depletion (50 and 85% for normal and water deficit stress irrigation conditions, respectively).
The volume of water used in each irrigation treatment was equal and was measured using a volumetric counter. However, the intervals of consecutive irrigations were different for the two irrigation treatments. The irrigation water depth was calculated as follows:
where B is the soil bulk density, D is the root-zone depth, θFC is the volumetric soil water content at field capacity (%) and θirrig is the soil water content at irrigation time as follow:
Measurement of seed yield and drought-stress tolerance index (STI)
Seed yield per unit area was determined at physiological maturity (mild-July and late-July of 2016 and 2017, respectively) based on the total harvested seed from the whole area of each plot and expressed as Kg/ha.
The STI was defined by Fernandez (1992), which can be used to identify genotypes that produce high yield under both stressed and non-stressed conditions. It was calculated based on the seed yield under normal (Yp) and water deficit stress (Ys) irrigation conditions using the following formula:
where Ymp was the average seed yield of all genotypes under normal irrigation condition.
Measurement of relative water content (RWC) and proline, chlorophyll and carotenoids concentrations
At 50% flowering stage, RWC and proline, chlorophyll and carotenoids concentrations were measured in both years. RWC was assessed on leaf sections obtained from the fully developed upper leaves, according to a method described in Askari and Ehsanzadeh (2015).
Free proline concentration in the leaves was determined using the method of Bates et al. (1973) and detailed by Gholami-Zali and Ehsanzadeh (2018b).
The concentration of chlorophyll and carotenoids in fresh leaf samples was measured by the spectrophotometer using the method of Lichtenthaler and Buschmann (2005) according to the details given in Askari and Ehsanzadeh (2015). The pigment concentrations were reported as mg/g of leaf fresh weight.
Measurement of antioxidant enzymes activity
Fresh leaf samples used for enzyme extraction according to Tabatabaei and Ehsanzadeh (2016). The enzyme extract was used to assay the following antioxidant enzymes activity and protein content.
The activities of CAT (EC 1.11.1.6), APX (EC 1.11.1.11) and POX (EC 1.11.1.7) of each enzyme extraction were evaluated by methods of Chance and Maehly (1955), Nakano and Asada (1981) and Herzog and Fahimi (1973), respectively. The details of measurements of enzymes activity has been described in a previous study (Tabatabaei and Ehsanzadeh 2016). The enzymes activity was expressed as unit enzyme activity per milligram of protein.
Since measuring enzymes activity requires to evaluate available protein in the sample, the total protein content in each extract was determined using the method as described in Bradford (1976).
Statistical analysis
After performing the homogeneity test of variances, the data were subjected to a combined analysis of variance over years (2 years) by using the SAS statistical program (Ver. 9.1; SAS Institute Inc., Cary, NC, USA). In this model, year and block were considered as random and irrigation regime and genotype were considered as fixed effects. Least significant difference (LSD, p ≤ 0.05) test was employed to separate the means, where F-test was found statistically significant. The correlation coefficients among the traits were calculated using the proc CORR of SAS.
Results
Except for carotenoid concentration and CAT activity, the effect of irrigation regime was statistically significant on all examined traits. Effect of genotype was also significant for all the traits excluding Chl-b concentration. The results also indicated that the effects of year and genotype × year were significant for most of the traits (Table 2).
Water deficit stress significantly reduced seed yield by 44.5% and 47.3%, in 2016 and 2017, respectively (Table 3). Also, water deficit stress significantly reduced RWC and Chl-a, Chl-b and carotenoids concentrations, while significantly increased proline concentration and APX activity, in both years. The CAT and POX activities were significantly increased in 2017 under water deficit irrigation condition, but they were no affected by this condition in 2016 (Table 3).
Under normal irrigation condition, genotypes M420 and E2417 showed the greatest mean for seed yield and genotypes C116 and C111 produced the lowest seed yield, in 2016 and 2017, respectively. Under water deficit irrigation condition, in 2016, genotypes Kouseh produced the lowest seed yield, while E2428 showed the highest mean of this trait. In 2017, under this irrigation condition, genotypes S3110 and A2 had the lowest and highest seed yield, respectively (Table 4).
Regarding RWC, under normal irrigation condition, genotypes E2417 and S3110 had the greatest RWC, while under water deficit irrigation condition genotypes E2427 and Kouseh showed the greatest RWC, in 2016 and 2017, respectively (Table 4).
The highest concentration of photosynthetic pigments belonged to genotype M420 under normal irrigation condition in 2016. In this year, and under water deficit irrigation condition, genotypes S3110, A1 and E2428 showed the highest Chl-a, Chl-b and carotenoids concentrations, correspondingly. In 2017, under normal irrigation condition, genotypes Arak2811, K12 and A1 had the highest Chl-a, Chl-b and carotenoids concentrations, respectively while under water deficit irrigation condition genotype A2 revealed the highest concentration of all measured pigments (Table 4).
At normal irrigation condition, proline concentration ranged from 17.4 (Arak2811) to 34.2 µmol/g FW (E2417) and under water deficit irrigation condition, it was varied from 35.8 (H27) to 57.0 µmol/g FW (A2) in 2016. In 2017, proline concentration ranged from 13.9 (C116) to 32.5 µmol/g FW (K21) under normal irrigation condition; however, it was varied from 28.3 (H27) to 58.2 µmol/g FW (K21) under water deficit irrigation condition (Table 4).
In 2016, genotypes E2417, K12 and A2, under normal irrigation condition and genotypes E2417, S3110 and M420, under water deficit irrigation condition, showed the highest CAT, APX and POX activities, respectively. In 2017, under normal irrigation condition the highest CAT activity and APX and POX activities belonged to genotypes E2428 and M420, correspondingly, while under water deficit irrigation condition, genotypes A2, C111 and S149 had the highest CAT, APX and POX activities, respectively (Table 4).
Based on the drought-stress tolerance index (STI), studied genotypes were classified into drought tolerant (M420, A2, S149, E2428, E2417, K21 and K12), semi-tolerant (AC-Stirling, S122, C128, A1, C4110, Kouseh and C111) and drought sensitive (E2427, C121, H27, AC-Sunset, S3110, Arak2811 and C116) groups (Table 5).
Discussion
The water deficit stress is a serious limiting factor of plant growth and development and has adverse effects on all morphological, physiological, biochemical and anatomical attributes of plants. In this work, seed yield was significantly reduced due to water deficit stress, in both years. Movahhedy-Dehnavy et al. (2009), Abd El-lattief (2013), Janmohammadi et al. (2017) and Shahrokhnia and Sepaskhah (2017) have also reported that water deficit condition decreased seed yield in safflower. Hence, it is important and helpful to identify the physiological functions related to drought tolerance for improvement of seed yield in safflower under water deficit stress condition. The decrease of seed yield was also observed in other crops such as pot marigold (Calendula officinalis) (Ghadyeh-Zarrinabadi et al. 2019), sesame (Sesamum indicum L.) (Ghotbzadeh-Kermani et al. 2019) and common bean (Phaseolus vulgaris L.) (Karimzadeh-Soureshjani et al. 2019). Decrease of seed yield in water stress condition can be related to reduction of photosynthetic activities due to stomatal closure and limited carbon dioxide uptake (Grieve et al. 2011).
Significant effect of genotypes indicating that there was genetic variation for the traits and selection can be effective to improve these traits. However, significant interaction of genotypes by year for studied traits showed that the response of genotypes for those characteristics was different in the years, which can be due to different environmental and climatic conditions.
Seed yield under both irrigation regimes was found to have a highly significant positive correlation with drought-stress tolerance index (STI) (Table 6). Therefore, this index can be recommended as a suitable criterion for identifying drought tolerant safflower genotypes. Our results were consistent with the results of other studies on different crops in that the STI is an effective index to be used in selection programs to distinguish tolerant and sensitive genotypes (Bahrami et al. 2014; Khalili et al. 2016; Pour-Aboughadareh et al. 2017).
In this study, RWC was reduced when plants were subjected to water deficit stress, in both years. Similarly, Hosseini et al. (2018) showed that the RWC in licorice (Glycyrrhiza glabra L.) was decreased under water stress. Since RWC also had a highly positive correlation with STI and seed yield in our study, under both normal and water deficit irrigation conditions (Table 6), the drought-tolerant genotypes of this study had higher seed yield and RWC in comparison to the drought-sensitive ones. These results suggested that RWC can be used as a good indicator of drought tolerance and beneficial for screening the safflower genotypes. Anupamaa et al. (2018) was also reported that the reduction of RWC values, as an index of drought susceptibility, was less in tolerant variety as compared to the sensitive one of rice (Oryza sativa L. ssp. indica). Sobhaninan et al. (2019) stated that RWC that reflects the water statue of a leaf under drought stress, had direct association whit grain yield in SeriM82/Babax wheat population. Other previous studies have also shown that RWC was reduced under drought stress and it was correlated with stress tolerance in different plant species (Amini et al. 2014; Askari and Ehsanzadeh 2015; Sheoran et al. 2015; Pour-Aboughadareh et al. 2017).
The free proline is usually the most important amino acid that is accumulated in many plants grown in different abiotic stresses. It has been suggested that proline concentration may be used as a resistance index to an array of stresses (Merwad et al. 2018). In this study, the notable accumulation of proline in response to water deficit stress indicated the important adjusting role of this osmolyte under stressful conditions. Other researchers have also reported that leaf proline concentration has increased under water deficit stress in different plant species (Gharibi et al. 2016; Mirjahanmardi and Ehsanzadeh 2016; Amiri et al. 2018; Maswada et al. 2018). The proline maintains turgidity under stress condition (Merwad et al. 2018), reduces the level of ROS by scavenging them, and enhances the activities of antioxidative enzymes (e.g. CAT, POX) in stressed plants (Hoque et al. 2007). Significant correlation was found between RWC and leaf proline concentration (Table 6), that indicated accumulation of this osmolyte enhanced osmotic adjustment and played a key role in maintaining cell water content, particularly when the plants are subjected to water deficit. The existence of relation between water potential and osmolytes in different plant species have been reported by other researches (Askari and Ehsanzadeh 2015; Gholami-Zali and Ehsanzadeh 2018b). Since proline accumulation in studied genotypes was well correlated to their STI and RWC under both normal and water deficit irrigation conditions, it seems that osmotic adjustment enabled maintaining higher leaf water potentials in tolerant genotypes during water stress and protected them against oxidative stress, therefore increased plant seed yield and tolerance. Our results agreed with the reports of Sheikh-Mohammadi et al. (2017) and Askari and Ehsanzadeh (2015) that they found that an increase in proline concentration leads to improved seed yield and drought tolerance.
Chlorophylls and carotenoids play a major role in photosynthesis and protects photosynthetic apparatus from harmful effects of ROS (Kadkhodaie et al. 2014). Chloroplast is a major source of ROS production in plant cells during drought stress and, hence, chlorophyll (Chl) would degrade seriously due to an increased ROS level (Iturbe-Ormaetxe et al. 1998). In present study, Chl-a and Chl-b concentrations were decreased, when genotypes were subjected to water deficit stress, in both years (Table 3). The decrease of Chl in drought-stressed plants is reported by several authors in different plant species of fennel (Foeniculum vulgare Mill) (Gholami-Zali and Ehsanzadeh 2018a), Salvia sinaloensis (Caser et al. 2018), and maize (Zea mays L.) (Maswada et al. 2018). Reduction of chlorophyll content might reduce growth parameters of plants under water stress condition and consequently, reduces seed yield (Caser et al. 2018). The decrease in leaf Chl concentration could be due to an increase of chlorophyllase activity and, hence, Chl degradation and impairment in Chl biosynthetic pathways (Santos 2004). Carotenoids are the main lipid-soluble antioxidants in plant cells that quench photooxidation, and involve signaling during plant development under stressful conditions (Mirjahanmardi and Ehsanzadeh 2016). In our study, carotenoids concentration was decreased under water deficit stress. Numerous studies have reported damage to carotenoids as a result of water deficit (Hammad and Ali 2014; Mirjahanmardi and Ehsanzadeh 2016; Gholami-Zali and Ehsanzadeh 2018b). Mirjahanmardi and Ehsanzadeh (2016) stated that the decrease of carotenoids could be described by the presence of other photoprotective mechanisms in plant species.
Plants increase production of ROS under stress conditions that could cause severe oxidative damage and membrane destruction in plant cells, unless ROS level is controlled by the antioxidant protective enzymes activity (Fazeli et al. 2007). In this study, antioxidative enzymes activities (CAT, APX and POX) were enhanced under water deficit irrigation condition, in both years and these results are in agreement with those reported by Sajedi et al. (2012), Yousefzadeh-Najafabadi and Ehsanzadeh (2017), Chen et al. (2018) and Cheng et al. (2018). In addition, the results indicated that the activities of these antioxidative enzymes were significantly correlated with drought tolerance in safflower (Table 6). In other studies, a positive correlation between drought stress tolerance and the level of SOD, CAT, POX and APX activities in the tissues of different plant species was also found (Askari and Ehsanzadeh 2015; Sheikh-Mohammadi et al. 2017). Catalase is the most important H2O2 scavenging enzyme that eliminates H2O2 through conversion to water and oxygen molecules (Mitler 2002). APX quench cellular H2O2 through the ascorbate-glutation cycle (Bowler et al. 1992). Thus, in tolerant plants, increase in enzymes activities may be an adoptive mechanism that help to overcome the ROS-derived damages. In this research, CAT activity also had a high positive correlation with RWC and similar results have been reported by Mercado et al. (2004) in Nicotiana tabacum, Khanna-Chopra and Selote (2007) in wheat (Triticum aestivum L.) and Merwad et al. (2018) in cowpea (Vigna unguiculata L.), indicating that higher antioxidant enzyme activities were associated with higher water retention ability in leaves. Therefore, genotypes maintaining higher antioxidant enzymes activity in leaves under water deficit stress may also have higher water retention and subsequently stress tolerance capacity. In this study, drought tolerant group tended to indicate higher proline accumulation, enzymes activity, and RWC, compared to the susceptible genotypic group.
Conclusion
In this study, water deficit stress significantly affected on physiological traits of safflower genotypes and seed yield. Moreover, our finding revealed that RWC, proline accumulation and enzymes activity correlated with drought tolerance and seed yield and could be used as appropriate selection indicators to distinguish drought tolerant genotypes and to increase seed yield of safflower under water deficit condition. Genotypes M420, A2, S149, E2428, E2417, K21 and K12 with more drought tolerance than the others can be used in breeding programs for development of drought-tolerant cultivars. Also, hybridization between drought tolerant and susceptible genotypes would be useful to develop segregating populations for future genetic studies and breeding programs.
Abbreviations
- APX:
-
Ascorbate peroxidase
- CAT:
-
Catalase
- Chl:
-
Chlorophyll
- POX:
-
Peroxidase
- ROS:
-
Reactive oxygen species
- RWC:
-
Relative water content
- STI:
-
Stress tolerance index
References
Abd El-Lattife, E. A. (2013). Safflower yields and water use efficiency as effected by irrigation at different soil moisture depletion levels and plant population density under arid conditions. Archives of Agronmy and Soil Science,59, 1545–1557.
Allen, R.G., Pereira, L.S., Raes, D. & Smith, M. (1998). Crop evapotranspiration: Guidelines for computing crop requirements. FAO Irrigation and Drainage paper, 56. Food and Agriculture Organization of the United Nations, Rome.
Amini, H., Arzani, A., & Karami, M. (2014). Effect of water deficiency on seed quality and physiological traits of different safflower genotypes. Turkish Journal of Biology,38, 271–282.
Amiri, H., Dousty, B., & Hosseinzedeh, S. R. (2018). Water stress-induced changes of morphological, physiological and essential oil compounds in Thymus eriocalyx from Iran. Journal of Essential oil-Bearing Plants,21, 1210–1223.
Anupamaa, A., Bhugrab, S., Lallb, B., Chaudhuryp, S., & Chugh, A. (2018). Assessing the correlation of genotypic and phenotypic responses of indica rice varieties under drought stress. Plant Physiology and Biochemistry,127, 343–354.
Askari, E., & Ehsanzadeh, P. (2015). Drought stress mitigation by foliar application of salicylic acid and their interactive effects on physiological characteristics of fennel (Foeniculum vulgare Mill.) genotypes. Acta Physiologiae Plantarum,37, 4–14.
Bahrami, F., Arzani, A., & Karimi, V. (2014). Evaluation of yield-based drought tolerance indices for screening safflower genotypes. Agronomy Journal,106, 1219–1224.
Bates, L. S., Waldran, R. P., & Teare, I. D. (1973). Rapid determination of free proline for water studies. Plant and Soil,39, 205–208.
Bouchemal, K., Bouldjadj, R., Belbekri, M. N., Ykhlef, N., & Djekounn, A. (2017). Differences in antioxidant enzyme activities and oxidative markers in ten wheat (Triticum durum Desf.) genotypes in response to drought, heat and paraquat stress. Archives of Agronomy and Soil Science,63, 710–722.
Bowler, C., Montagu, M. V., & Inez, D. (1992). Superoxide dismutase and stress tolerance. Annual Review of Plant Physiology,43, 83–116.
Bradford, M. M. (1976). A rapid and sensitive method for the quantitation of microgram quantities of protein utilizing the principle of protein dye binding. Analytical Biochemistry,72, 248–254.
Caser, M., Angiolillo, F., Chitarra, W., Lovisolo, C., Ruffoni, B., Pistelli, L., et al. (2018). Ecophysiological and phytochemical responses of Salvia sinaloensis Fern. to drought stress. Plant Growth Regulation,84, 383–394.
Chance, B., & Maehly, A. C. (1955). Assay of catalase and peroxidase. Methods in Enzymology,2, 746–775.
Chen, Z., Wang, Z., Yang, Y., Li, M., & Xu, B. (2018). Abscisic acid and brassinolide combined application synergistically enhances drought tolerance and photosynthesis of tall fescue under water stress. Scientia Horticulturae,228, 1–9.
Cheng, L., Han, M., Yang, L.-M., Yang, L., Sun, Z., & Zhang, T. (2018). Changes in the physiological characteristics and baicalin biosynthesis metabolism of Scutellaria biacalensis Georgi under drought stress. Industrial Crops and Products,122, 473–482.
Fariaszewska, A., Aper, J., Huylenbroeck, V., Baert, J., De Riek, J., Staniak, M., et al. (2017). Mild drought stress-induced changes in yield, physiological processes and chemical composition in Festuca, Lolium and Festulolium. Journal of Agronomy and Crop Science,203, 103–116.
Fazeli, F., Ghorbani, M., & Niknam, V. (2007). Effect of drought on biomass, protein content, lipid peroxidation and antioxidant enzymes in two sesame cultivars. Biologia Plantarum,51, 98–103.
Fernandez, G.C.J. (1992). Effective selection criteria for assessing plant stress tolerance. In ʻProceeding International Symposium on Adaption of Food Crops to Temperature and Water Stress’. (Ed. C. C. Kuo), pp. 257–270. (Asian Vegetable Research and Development Center: Shanhua, Taiwan).
Ghadyeh-Zarrinabadi, I., Razmjoo, J., Abdali Mashhadi, A., Karim Mojeni, H., & Boroomand, A. (2019). Physiological response and productivity of pot marigold (Calendula officinalis) genotypes under water deficit. Industrial Crops and Products,139, 111488.
Gharibi, Sh, Tabatabaei, B. E. S., Saeidi, G., & Goli, S. A. H. (2016). Effect of drought stress on total phenolic, lipid peroxidation and antioxidant activity of Achillea species. Applied Biochemistry and Biotechnology,178, 796–809.
Gholami-Zali, A., & Ehsanzadeh, P. (2018a). Exogenously applied proline as a tool to enhance water use efficiency: case of fennel. Agricultural Water Management,197, 138–146.
Gholami-Zali, A., & Ehsanzadeh, P. (2018b). Exogenous proline improves osmoregulation, physiological functions, essential oil, and seed yield of fennel. Industrial Crops and Products,111, 133–140.
Ghotbzadeh-Kermani, S., Saeidi, Gh, Sabzalian, M. R., & Gianinetti, A. (2019). Drought stress influenced sesamin and sisamolin content and polyphenolic components in sesame (Sesamum indicum L.) populations with contrasting seed coat colors. Food Chemistry,289, 360–368.
Grieve, C. M., Grattan, S. R., & Maas, E. V. (2011). Plant salt tolerance. Agricultural Salinity Assessment and Management, Edition,2, 405–460.
Hammad, S. A. R., & Ali, O. A. M. (2014). Physiological and biochemical studies on drought tolerance of whet plants by application of amino acids and yeast extract. Annals of Agricultural Science,59, 133–145.
Hassanzadeh, M., Ebadi, A., Panahyan-e-Kivi, M., Eshghi, A. G., Jamaati-e-Somarin, S. H., Saeidi, M., et al. (2009). Evaluation of drought stress on relative water content and chlorophyll content of sesame (Sesamum indicum L.) genotypes at early flowering stage. Research Journal of Environmental Sciences,3, 345–350.
Herzog, V., & Fahimi, H. (1973). Determination of the activity of peroxidase. Analytical Biochemistry,55, 554–562.
Hoque, M. A., Banu, M. N., Okuma, N., Amako, K., Nakamura, Y., Shimoishi, Y., et al. (2007). Exogenous proline and glycinebetaine increase NaCl-induced ascorbate-glutatione cycle enzyme activities, and proline improves salt tolerance more than glycinebetaine in tobacco Bright Yellow-2 suspension-cultured cells. Journal of Plant Physiology,164, 1457–1468.
Hosseini, M. S., Samsampour, D., Ebrahimi, M., Abadia, J., & Khanahmadi, M. (2018). Effect of drought stress on growth parameters, osmolyte contents, antioxidant enzymes and glycyrrhizin synthesis in licorice (Glycyrrhiza glabra L.) grown in the field. Phytochemistry,156, 124–134.
Iturbe-Ormaetxe, I., Escuredo, P. R., Arrese-Igor, C., & Becana, M. (1998). Oxidative damage in pea plants exposed to water deficit or paraquat. Plant Physiology,116, 173–181.
Janmohammadi, M., Mohammadi, N., Shekari, F., Abbasi, A., & Esmailpour, M. (2017). The effects of silicon and titanium on safflower (Carthamus tinctorius L.) growth under moisture deficit condition. Acta Agriculturae Slovenica,109, 443–455.
Kadkhodaie, A., Zahedi, M., Razmjoo, J., & Pessarakli, M. (2014). Changes in some antioxidative enzymes and physiological indices among sesame genotypes (Sesamum indicum L.) in response to soil water deficits under field conditions. Acta Phisiologiae Plantarum,36, 641–650.
Karimzadeh-Soureshjani, H., Nezami, A., Kafi, M., & Tadayon, M. (2019). Responses of two common bean (Phaseolus vulgaris L.) genotypes to deficit irrigation. Agricultural Water Management,213, 270–279.
Khalili, M., Pour-Aboughadareh, A., & Naghavi, M. R. (2016). Assessment of drought tolerance in barley: integrated selection criterion and drought tolerance indices. Environmental and Experimental Biology,14, 33–41.
Khanna-Chopra, R., & Selote, D. S. (2007). Acclimation to drought stress generates oxidative stress tolerance in drought-resistant than susceptible wheat cultivar under field conditions. Environmental and Experimental Botany,60, 276–283.
Lichtenthaler, H. K., & Buschmann, C. (2005). Chlorophylls and carotenoids: Measurement and characterization by UV-VIS spectroscopy. Current Protocols in Food Analytical Chemistry,2005, F4.3.1–F4.3.8.
Maswada, H. F., Abd El-Razek, U. A., El-Shshtawy, A. N. A., & Elzaawely, A. A. (2018). Morpho-physiological and yield responses to exogenous moringa leaf extract and salicylic acid in maize (Zea mays L.) under water stress. Archives of Agronomy and Soil Science,64, 994–1010.
Mercado, J. A., Matas, A. J., Heredia, A., Valpuesta, V., & Quesada, M. A. (2004). Changes in the water binding characteristics of the cell walls from transgenic Nicotiana tabacum leaves with enhanced levels of peroxidase activity. Physiologia Plantarum,122, 504–512.
Merwad, A. M. A., Desoky, E. S. M., & Rady, M. M. (2018). Response of water deficit-stressed Vigna unguiculata performances to silicon, proline or methionine foliar application. Scientia Horticulturae,228, 132–144.
Mirjahanmardi, H., & Ehsanzadeh, P. (2016). Iron supplement ameliorates drought-induced alterations in physiological attributes of fennel (Foeniculum vulgare). Nutrient Cycling in Agroecosystems,106, 61–76.
Mitler, R. (2002). Oxidative stress, antioxidants and stress tolerance. Trends in Plant Science,7, 405–410.
Movahhedy-Dehnavy, M., Modarres-Sanavy, S. A. M., & Mokhtassi-Bidgoli, A. (2009). Foliar application of zinc and manganese improves seed yield and quality of safflower (Carthamus tinctorius L.) grown under water deficit stress. Industrial Crops and Products,30(1), 82–92.
Nakano, Y., & Asada, K. (1981). Hydrogen peroxide is scavenged by ascorbate-specific peroxidase in spinach chloroplasts. Plant and Cell Physiology,22(5), 867–880.
Osorio, M. L., Osorio, J., & Romano, A. (2013). Photosynthesis, energy partitioning, and metabolic adjustments of the endangered Cistaceae species Tuberaria major under high temperature and drought. Photosynthetica,51, 75–84.
Pour-Aboughadareh, A., Ahmadi, J., Mehrabi, A. A., Etminan, A., Moghaddam, M., & Siddique, H. M. (2017). Physiological responses to drought stress in wild relatives of wheat: implications for wheat improvement. Acta Physiologiae Plantarum,39, 106.
Sajedi, N. A., Ferasat, M., Mirzakhani, M., & Boojar, M. M. A. (2012). Impact of water deficit stress on biochemical characteristics of safflower cultivars. Physiology and Molecular Biology of Plants,18, 323–329.
Santos, C. V. (2004). Regulation of chlorophyll biosynthesis and degredation by salt stress in sunflower leaves. Scientia Horticulturae,103, 93–99.
Shahrokhnia, M. H., & Sepaskhah, A. R. (2017). Physiologic and agronomic traits in safflower under various irrigation strategies, planting methods and nitrogen fertilization. Industrial Crops and Products,95, 126–139.
Sheikh-Mohammadi, M. H., Etemadi, N., Nikhbakht, A., Arab, M., Majidi, M. M., & Pessarakli, A. (2017). Antioxidant defence system and physiological responses of Iranian crested wheatgrass (Agropyron cristatum L.) to drought and salinity stress. Acta Physiologiae Plantarum,39, 245.
Sheoran, S., Thakur, V., Narwal, S., Turan, R., Mamruta, H. M., Singh, V., et al. (2015). Differential activity and expression profile of antioxidant enzymes and physiological changes in wheat (Triticum aestivum L.) under drought. Applied Biochemistry and Biotechnology,177, 1282–1298.
Silva, M., Jifon, J., Silva, J. A. G., & Sharma, V. (2007). Use of physiological parameters as fast tools to screen for drought tolerance in sugarcane. Brazilian Journal of Plant Physiology,19, 193–201.
Singh, S., Angadi, S. V., Hilaire, R. S., Grover, K., & Vanleeuwen, D. M. (2016). Spring safflower performance under growth stage based irrigation in the southern high plains. Crop Science,56, 1878–1889.
Sobhaninan, N., Heidari, B., Tahmasebi, S., Dadkhodaei, A., & McIntyre, C. L. (2019). Response of quantitative and physiological traits to drought stress in the SeriM82/Babax wheat population. Euphytica,215, 32.
Tabatabaei, S., & Ehsanzadeh, P. (2016). Photosynthetic pigments, ionic and antioxidative behavior of hulled tetraploid wheat in response to NaCl. Photosynthetica,54, 340–350.
Yousefzadeh-Najafabadi, M., & Ehsanzadeh, P. (2017). Photosynthetic and antioxidative upregulatios in drought-stressed sesame (Sesamum indicum L.) subjected to foliar-applied salicylic acid. Photosynthetica,55, 611–622.
Acknowledgements
The authors appreciate the contributions of the Isfahan University of Technology for its scientific and financial support. They would also like to thank Dr. A.F. Mirlohi from Isfahan University of Technology, Iran for editing the final English draft of this manuscript.
Author information
Authors and Affiliations
Corresponding author
Ethics declarations
Conflict of interest
The authors declare that there is no conflict of interest.
Rights and permissions
About this article
Cite this article
Alizadeh Yeloojeh, K., Saeidi, G. & Ehsanzadeh, P. Effectiveness of physiological traits in adopting safflower (Carthamus tinctorius L.) genotypes to water deficit condition. Int. J. Plant Prod. 14, 155–164 (2020). https://doi.org/10.1007/s42106-019-00075-3
Received:
Accepted:
Published:
Issue Date:
DOI: https://doi.org/10.1007/s42106-019-00075-3