Abstract
Gestational diabetes mellitus (GDM) is a conditional diabetes which is defined as any degree of glucose intolerance or high blood glucose levels during any phase of pregnancy. It causes chronic severe damage to health of the pregnant women and their offspring. In this study, we aimed to study the protective effects of Cryptotanshinone on GDM-related impairments. We measured blood glucose levels, serum insulin levels, biochemical indexes, oxidative stress, inflammation and the activation of NF-κB signaling pathway in the blood and placenta of GDM mice. It is found that Cryptotanshinone significantly decreased blood glucose levels, oxidative stress, inflammation and NF-κB signaling with an increase of serum insulin levels in the placenta and blood of GDM mice. Taken together, Cryptotanshinone effectively ameliorated GDM, which suggested that Cryptotanshinone could be served as a promising therapeutic drug for GDM patients.
Similar content being viewed by others
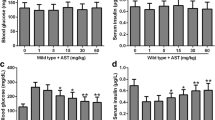
Avoid common mistakes on your manuscript.
Introduction
Gestational diabetes mellitus (GDM) is defined as any degree of glucose intolerance or high blood glucose levels with onset or first recognition during any phase of pregnancy. Unlike type I or type II diabetes mellitus, GDM is a conditional and temporary diabetes in which symptoms would disappear after delivery (Alfadhli 2015). However, it causes chronic severe damage to the health of the pregnant women and their offspring. For mothers, GDM increases the risk of pre-eclampsia, depression, and intrauterine fetal death. Moreover, GDM patients require a caesarean delivery instead of eutocia. After delivery, women with GDM are at an increased risk of diabetes. Usually type II diabetes mellitus (T2DM). The babies born from GDM patients with poorly treated increase the risk of fetal macrosomia, jaundice, neonatal hypoglycemia, polythemia, and hypocalcemia. In a long term, offspring of GDM patients have a high risk of obesity, glucose intolerance and diabetes in their late adolescence and young adulthood (Chiefari et al. 2017). Considering all these increased risks of GDM to the pregnant women and their offspring, GDM patients need to be well treated.
Though the mechanism of GDM is not clear yet, placenta is well recognized to play an important role in the development of GDM. Placenta supplies a growing fetus with nutrients and water and produces a variety of hormones to maintain the pregnancy. These placenta-derived hormones prevent the body from effectively using insulin, therefore glucose builds up in the blood instead of metabolizing by the body for energy, resulting in GDM (Chiefari et al. 2017). Moreover, some of these hormones including estrogen, cortisol, and human placental lactogen, have an inhibitory effect of insulin, as to contra-insulin effect, which usually begins about 20–24 weeks of the pregnancy. As the placenta grows, more of these hormones are produced leading to the increased risk of insulin resistance Normally, pancreas is able to produce additional insulin to overcome insulin resistance, but once insulin produced is not sufficient to diminish contra-insulin effect induced by placental hormones, pregnancy women will develop GDM. Therefore, it is necessary to investigate the mechanism of placenta in the development of GDM.
Cryptotanshinone (CTS) is a monomer of Tanshinone, which is the major lipid-soluble and active component of Traditional Chinese Medicine (TCM) Salvia miltiorrhiza bunge (Dan Shen in Chinese) extracted from dries roots and rhizomes of Labiatae Salvia. Accumulating evidence demonstrated that CTS has anti-cancer effects (Jiang et al. 2017; Wang et al. 2017; Liu et al. 2019). Furthermore, cryptotanshinone also alleviated cardiac fibrosis either in vivo by inhibiting STAT3 signaling (Chen et al. 2013) or in vitro by downregulating the expression of cyclooxygenase-2, NADPH oxidase-2 and 4 in vitro (Ma et al. 2014). Previous publication revealed that CTS remarkably improved hypoxia or reoxygenation-induced oxidative stress and apoptosis in renal tubular epithelial cells (Zhu et al. 2019). Moreover, CTS also diminished cell apoptosis mediated by reactive oxygen species in human synoviocytes (Sun et al. 2019). However, there is no study about the effect of CTS in GDM, therefore, we explored its effect in GDM. Of note, oxidative stress and inflammation are the biomarkers of several pregnant complications including GDM, pre-eclampsia and preterm birth (Badehnoosh et al. 2018; Khambule and George 2019). Moreover, considering the important role of inflammation in GDM (Pantham et al. 2015). In this study, we aimed to investigate the effect of CTS in the oxidative stress and inflammation of GDM.
Method and materials
GDM mouse model
C57BL/KsJ+/+ (wild-type) and C57BL/KsJdb+ (db/ +) purchased from Nanjing Model Animal Center (Nanjing, China) were cared under the guideline of Animal Care Committee of Cangzhou Central Hospital as previously described (Yao et al. 2015). 10–12 weeks old female mice individually mated with males of the same genotype were confirmed by the present of copulatory plug in the following morning. This is defined as gestation day (GD) 0. Pregnant female mice were selected for the following studies, and randomly divided into three groups: wild-type (normal pregnancy, C57BL/KsJ+/+), GDM (C57BL/KsJdb+, db/ + mice treated with vehicle) and GDM + CTS group (C57BL/KsJ db+, db/ + mice treated with different doses of CTS respectively). N = 18 for each group. CTS (Sigma Aldrich, St Louis, MO, USA) was dissolved in the 0.1% solution of sodium lauryl sulfate and orally administrated into mice at different doses (5,15 and 45 mg/kg body weight) daily for 18 days since pregnancy (Lo et al. 2017). At GD 0, GD 9 and GD 18, body weight of each mice was measured on the scale, and blood glucose levels were analyzed by glucometer (Roche Diagnostics, Risch-Rockreuz, Switzerland). Blood from tail venipuncture was collected. At GD 17, mice were weighed on the scale and fasting overnight prior to collecting maternal blood. After collecting blood from heart by cardiac puncture on GD 18, cesarean section was performed to obtain placentas and fetus for the following studies.
To further prove the effect of CTS, we also orally treated GDM mice with metformin (10 mg/kg, Sigma-Aldrich) daily from GD 0 for 18 days and collect blood from heart on GD 18 to compare with CTS-treated mice.
Biochemical parameters analysis
Total serum cholesterol (Tch), serum triglyceride (TG) and serum high-density lipoprotein (HDL) were measured according to the procedures of the commercially available kits (Suman et al. 2016; Zeng et al. 2017). Low-density lipoprotein (LDL) is calculated using the Friedwald’s formula.
Real-time poly chain reaction (PCR)
RNA was extracted using RNeasy Mini Kit (Qiagen, Valencia, CA, USA) after homogenizing the same amount of placental tissues from mice in Trizol (Thermo Fisher Scientific, Waltham, MA, USA). 1000 ng of RNA was reverse-transcribed into cDNA (High-Capacity cDNA Reverse Transcription kit (Thermo Fisher Scientific)). SYBR green (Molecular Probes, Waltham, MA, USA) was used to detect PCR products. All reactions were performed in a 20 μL reaction volume in triplicate. PCR amplification consisted of an initial denaturation step at 95 °C for 5 min, followed by 40 cycles of PCR at 95 °C for 20 s, 60 °C for 30 s. Relative expression of the above genes was measured by the 2−ΔΔCt method using glyceraldehyde-3-phosphate dehydrogenase (GAPDH) and then normalized to the wild-type group.
The primers for these mRNA were as below:
IL-1β: 5′-GCAACTGTTCCTGAACTCAACT-3′ (forward),
5′-ATCTTTTGGGGTCCGTCAACT-3′ (reverse);
IL-6: 5′-TAGTCCTTCCTACCCCAATTTCC-3′ (forward),
5′-TTGGTCCTTAGCCACTCCTTC-3 (reverse);
TNF-α: 5′-CCCTCACACTCAGATCATCTTCT-3′ (forward),
5′-GCTACGAGTGGGCTACAG-3′(reverse);
GAPDH: 5′-AGGTCGGTGTGAACGGATTTG-3′ (forward),
5′-TGTAGACCATGTAGTTGAGGTCA-3′ (reverse).
Western blot
Protein was extracted from lysed placenta in RIPA lysis buffer on GD 18. After centrifuged at 12,000 ×g at 4 °C for 10 min, the same amounts of protein (10 μg) from supernant were loaded into SDS-PAGE gel and transferred to PVDF membrane. After blocking in TBST with 5% milk, membrane was incubated with primary antibody overnight. Then, the membrane was incubated with peroxidase-conjugated goat IgG secondary antibodies at room temperature for one hour. Blots were visualized by chemiluminescence in an ECL kit (Thermo Fisher Scientific). All the primary and secondary antibodies were purchased from Cell Signaling Technology (Danvers, MA, USA).
Measurement of oxidative stress markers
Reduced glutathione (GSH) was measured as described before (Fu et al. 2012) and GSH activity was showed as nM/mg of placenta. The activities of catalase and glutathione peroxidase (GPx) were analyzed using kits (Jiancheng Biological, Nanjing, China). SOD activity was detected using a xanthine oxidase technique by kit (Shanghai Solarbio Bioscience & Technology Co., Shanghai, China).
ELISA
For insulin measurement, blood from mice was directly used in mouse ins1/Insulin ELISA Kit. For measuring the levels of inflammatory factors in the blood, we collected blood from heart, then centrifuged at 1000 ×g for 15 min to get serum. 10 μl aliquot of serum was used in ELISA kit respectively (IL-6, IL-1β, TNF-α). For assessment of placental inflammation, the placental tissues were dissected from the mice and homogenized. The homogenates were tested using ELISA kits respectively (IL-6, IL-1β, TNF-α). All the ELISA measurement was performed according to the manufacturer’s instruction and purchased from Sigma-Aldrich (St. Louis, MO, USA).
Statistical analysis
Data were analyzed by statistical product and service solutions (SPSS 16.0, SPSS Inc,. Chicago, IL, USA) and expressed as means ± SEM. One-way analysis of variance followed by Lease Significant Difference as its Post-Hoc test were used to compare multiple groups.
Results
CTS attenuates metabolic syndrome in GDM mice
To evaluate the safety of CTS for both mother and fetus, we measured the blood glucose levels and serum insulin levels in gestational wt mice treated with different doses of CTS (1, 5, 15, 30, 45 and 60 mg/kg body weight). After oral administration of CTS for 18 days daily, there was no significant difference in blood glucose levels and serum insulin levels between vehicle treated group and any dose of CTS treated groups (Fig. 1a, b). Therefore, we chose three doses of CTS (5, 15 and 45 mg/kg) for the following studies.
At the beginning of pregnancy (GD 0), mice in all groups displayed no difference of body weight, blood glucose levels, and serum insulin levels, and started to exhibit difference in the middle stage of pregnancy on GD 9 (Fig. 1a–c). Compared with wt mice, GDM mice had a lower body weight and serum insulin levels with higher levels of blood glucose, which are the metabolic syndromes of GDM. CTS gradually reversed all these symptoms and had a significantly protective effect at the highest dose (45 mg/kg). At the end of pregnant period (GD 18), all the GDM symptoms became more severe which were also attenuated by CTS in a dose-dependent manner. Insulin is produced in the pancreas, and lack of insulin in GDM is due to the dysfunction of β-cells. Thus, insulin levels increased by CTS treatment might indicated that CTS improved β-cells’ function. Therefore, we calculated HOMA-β to analyze the β-cell function as previously reported (Suman et al. 2016).
As expected, compared with wild-type mice, GDM mice lost β-cell function and this β-cell dysfunction could be dose-dependently improved by CTS administration (Fig. 2d).
CTS attenuates gestational diabetes mellitus symptoms in GDM mice model. Maternal body weight (a), blood glucose (b) and serum insulin (c) were measured on gestation day (GD) 0, 9 and 18 in wild type, GDM group and GDM + CTS with different concentrations. Homeostasis model assessment of β-cell function (HOMA-β) (d) were calculated on GD 18. Data are presented as means ± SEM. *p < 0.05, **p < 0.01 and ***p < 0.001 compared to wild type group, #p < 0.05, ##p < 0.01 compared to GDM mice
Moreover, we measured several biochemical indexes including total serum cholesterol (Tch), serum triglyceride (TG), serum high-density lipoprotein (HDL) and serum low-density lipoprotein (LDL) to further evaluate the effect of CTS in GDM mice. Compared with wt mice, GDM mice displayed an increased level of Tch, TG and LDL with a decreased level of HDL, which were all the syndrome of GDM and consistent with previous paper (Suman et al. 2016). CTS dese-dependently ameliorated all these syndromes induced by GDM (Fig. 3a–d). As a consequence, CTS dose-dependently attenuated metabolic syndromes of GDM in mice.
CTS ameliorated biochemical indexes in the late stage of pregnancy in GDM mice. Total serum cholesterol (TCh) (a), serum triglyceride (TG) (b), serum high-density lipoprotein (HDL) (c), serum low-density lipoprotein (LDL) (d) were tested on GD 18 among different groups. Data are presented as means ± SEM. **p < 0.01 and ***p < 0.001 compared to wild type group, #p < 0.05, ##p < 0.01 compared to GDM mice
To further prove the protective effect of CTS in GDM, we also treated GDM mice with metformin, which is been widely accepted to improve GDM as a positive control. Figure S1 and S2 showed that metformin (10 mg/kg) treatment for 18 days also attenuated the symptoms of GDM and lipid profiles in mice, which was comparable with CTS (45 mg/kg). Although metformin might have slightly more effect on decreasing blood glucose levels (Figure S1b), increasing serum insulin levels (Figure S1c) and reducing the levels of TCh and LDL (Figs. S2a, S2d) there was no significant difference in maternal body weight change, HOMA-β index and the levels of TG and HDL between CTS and metformin treated mice, therefore these results suggested that CTS could ameliorate the symptoms of GDM as metformin did.
CTS ameliorated placental oxidative stress in the late stage of pregnancy in GDM mice.
To assess the effect of CTS in placental oxidative stress, we analyzed five oxidative stress markers including malondialdehyde (MDA), superoxide dismutase (SOD), catalase (CAT) glutathione (GSH) and glutathione peroxidase (GPx). Reactive oxygen species (ROS) degrade polyunsaturated lipids to form MDA that causes toxic stress accumulation in cells. That could explain GDM significantly induced MDA expression compared with wt group, which was downregulated by CTS treatment in a dose-dependent way (Fig. 4a). Unlike MDA, the other three markers such as SOD, CAT and GPx are major antioxidant enzymes that catalyze highly reactive oxidant species leading to reduced cytotoxicity and oxidative stress. As a result, these three enzymatic activities changed in the same way, which was reduced in GDM group and dose-dependently restored by CTS treatment (Fig. 4b, c and e). Glutathione as an antioxidant exists in reduced and oxidized forms. The ratio of reduced GSH to oxidized GSSG within cells reflects cellular oxidative stress. Reduced form of GSH was also remarkably diminished in GDM mice and reversed dose-dependently in CTS treated GDM mice (Fig. 4d). Therefore, CTS effectively reduced placental oxidative stress induced by GDM in mice.
CTS ameliorated placental oxidative stress in the late stage of pregnancy in GDM mice. Malondialdehyde (MDA) (a), superoxide dismutase (SOD) (b), glutathione peroxidase (GPx) (c), glutathione (GSH) (d) and catalase (CAT) (e) in placenta were measured by ELISA on GD 18 among indicated groups. Data are presented as means ± SEM. **p < 0.01compared to wild type group, #p < 0.05, ##p < 0.01 compared to GDM mice
CTS ameliorated placental inflammation in the late stage of pregnancy in GDM mice.
Next, to evaluate the effect of CTS in inflammation induced by GDM, we measured inflammatory factors both in the serum and placenta, including IL-6, IL-1β and TNF-α. These three inflammatory factors were significantly induced by GDM both in the serum and placenta and dose-dependently reduced after CTS treatment (Fig. 5a–i). These results indicated that CTS could ameliorate the inflammation both in the serum and placenta of gestational mice, so that it suggested CTS could work effectively both for the mothers and their fetus by alleviating inflammation.
CTS ameliorated placental inflammatory in the late stage of pregnancy in GDM mice. ELISA was used to analyze the levels of IL-6 (a), IL-1β (b) and TNF-α (c) in serum from indicated mice on GD18. ELISA was used to analyze the levels of IL-6 (d), IL-1β (e) and TNF-α (f) in placenta from indicated mice on GD18. qRT-PCR was used to analyzed the mRNA levels of IL-6 (g), IL-1β (h) and TNF-α (i) in placenta from indicated mice on GD18. Data are presented as means ± SEM. **p < 0.01 and ***p < 0.001 compared to wild type group, #p < 0.05, ##p < 0.01 compared to GDM mice
NF-κB signaling pathway participated in the protective effects of CTS on GDM mice.
Accumulating evidence revealed that NF-κB signaling pathway plays an important role in the protective role of CTS (Wang et al. 2018a, 2018b), which is also involved in the progression and development of GDM (Feng et al. 2016), thus we explored the effect of CTS on NF-κB signaling pathway in GDM mice. NF-κB signaling pathway was activated in GDM mice as judge of the increased phosphorylation of p65 and decreased expression of IκBα, which were both restored gradually by the increasing doses of CTS (Fig. 6a, b). Therefore, CTS suppressed NF-κB signaling pathway stimulated by GDM.
NF-κB signaling pathways participated in the meliorative effects of cryptotanshinone on GDM mice. a Western blotting was used to detect the protein expressions of p‐NF‐κB p65, NF‐κB p65, and IκBα. β-actin was used as a loading control and relative expressions were shown in (b). Data are presented as means ± SEM. ***p < 0.001 compared to wild type group, #p < 0.05, ##p < 0.01 and ###p < 0.001 compared to GDM mice
CTS improved reproductive outcome of pregnant GDM mice
One of the adverse effects of GDM is decreased fetal survival, presented as fewer number of fetuses. Moreover, GDM usually causes higher birth weight. Therefore, we recorded the litter size of each group and weight the offspring. We found that the offspring in GDM mice group showed higher birth weight (Fig. 7a), and the GDM mice presented lower litter size (Fig. 7b). However, CTS (45 mg/kg) significantly ameliorated these effects. Thus, it is suggested that CTS improved reproductive outcome of pregnant GDM mice.
In summary, CTS could effectively ameliorate metabolic syndromes induced by GDM via reducing oxidative stress and inflammation, and inhibiting NF-κB signaling pathway, therefore CTS might serve as a potential and promising drug to treat GDM.
Discussion
Our study verified that Cryptotanshinone is effective to improve GDM symptoms by reducing oxidative stress, alleviating inflammation and inhibiting NF-κB signaling pathway, therefore all these results suggested that Cryptotanshinone might be benefit to GDM and serve as a promising and potential drug to treat GDM.
Salvia miltiorrhiza bunge (Dan Shen in Chinese) as a famous and ancient Traditional Chinese Medicine (TCM), also known as red sage, has been widely used as a multi-target drug in clinical for a long period. It has varieties of pharmacological and clinical applications to benefit human health (Liu et al. 2012). For example, it was reported to have neuro-protective effect to treat neurodegenerative disease, such as Alzheimer’s disease (Chong et al. 2019). It was also discovered to have therapeutic effects on a wide range of cardiovascular diseases, including atherosclerosis, angina pectoris, myocardial infarction, hypertension, hyperlipidemia and pulmonary heart diseases because of its anti-inflammatory, oxidative, cell-protective, anti-coagulated effects (Yu et al. 2017; Ren et al. 2019). Moreover, it was proved to have anti-osteoporotic effect by either inhibiting osteoclast differentiation or bone resorption (Guo et al. 2014). Salvia miltiorrhiza bunge as a natural herb extracted from roots of perennial plant in the genus salvia, has many bioactive chemicals constitutes which can be classified into two major groups due to their chemical property that is water-soluble phenolics and lipid-soluble Tanshinone. Water-soluble components such as pripanoid acid and salvianolic acid, are not as effective as lipid-soluble ones because they are restricted to the extracellular fluid after entering into the blood circulation leading to less distribution to the cells with their membrane structure of lipid-soluble bilayer, therefore, while the pharmacological effects of water-soluble components in Dan Shen might mostly apply on vascular activity. Lipid-soluble components can be largely distributed to the whole body that has a wide range of pharmacological activities. That is the reason we chose CTS, which is a monomer of Tanshinone and also the major lipid-soluble component in Dan Shen to explore its protective effects in GDM. Though there is no relevant publication between cryptotanshinone and gestational diabetes mellitus, CTS has been proved to have anti-diabetic and anti-obesity effects via activating AMP-activated protein kinase. Considering GDM and diabetes share some similar symptoms and mechanisms, we hypothesized whether CTS will improve GDM as well as diabetes, and our results confirmed our hypothesis.
In our current study, to better elucidate the protective effect of CTS on GDM mice, we also treated GDM mice with metformin as a positive control. Metformin is the second oral option that has been evaluated as a logical alternative to insulin in GDM, which is classified as pregnancy category B (Wensel 2009). Metformin ameliorates insulin resistance by reducing blood glucose through reduction in hepatic glucose production, decreased absorption of glucose and increased peripheral uptake and utilization of glucose (Goh et al. 2011). Although there is controversary conclusions on the safety of metformin in the clinical trials, it is well acceptable of the effect of metformin on improving insulin resistance. Therefore, we compared the protective effect of CTS with metformin in GDM mice. Although CTS might not be effective as metformin on improving some symptoms of GDM, the effect of CTS is comparable with metformin overall.
Although the mechanism of GDM remains unclear yet, it is widely accepted that GDM and T2DM share the similar mechanism, in which insulin resistance and beta-cell dysfunction are central to their pathogenesis. Previous study revealed that oxidative stress plays an important role in insulin resistance and islet beta-cell dysfunction, resulting in hyperglycemia (Wang et al. 2017). Moreover, it is believed that patients, the increase on the maternal and fetal oxidative stress response in the placenta of GDM indicated that oxidative stress is involved both in the occurrence and progression of GDM, and the development of GDM complications. Furthermore, patients with GDM displayed an increased plasma MDA levels with a decreased level of anti-oxidants and a reduced secretion and sensitivity of insulin. In our current study, we found that CTS could effectively reduce oxidative stress in the placenta and blood of GDM mice, which suggested that CTS might improve GDM by inhibiting oxidative stress in the placenta and blood. NF-κB signaling pathway participated in varieties of physiological and pathological process, which mediated a wide range of diseases as well as gestational diabetes mellitus (Coughlan et al. 2004; Wei et al. 2010; Kuzmicki et al. 2013; Feng et al. 2016). In patients with gestational diabetes mellitus, toll-like receptor 2 (TLR2) expression in peripheral blood mononuclear cells, was elevated when NF-κB signaling pathway was activated, as well as higher fasting blood glucose and insulin resistance, all of which involved in the development of GDM. Moreover, suppressing NF-κB activities could elevate glucose transporter 4 (GLUT4) expression in uterus of gestational diabetes mellitus rats, which suggested that inhibiting NF-κB activities to block the GLUT4 expression might be related with the occurrence of gestational diabetes mellitus in rats with uteru insulin resistance, and NF-κB activities also mediated placental cytokine responses in GDM. Taken together, NF-κB signaling pathway takes an important role in the occurrence and development of gestational diabetes mellitus, therefore, we evaluated the role of NF-κB signaling pathway in the development of GDM mice treated with CTS. Moreover, previous studies revealed that CTS could significantly inhibited NF-κB signaling pathway, and our results confirmed that CTS could suppress NF-κB signaling pathway leading to alleviating the symptoms of GDM.
Previous reports showed that CTS did not change the blood glucose levels in streptozotocin-induced type 1 diabetic rats, which is not consistent with our results that treatment of Cryptotanshinone could significantly decrease blood glucose levels in GDM mice (Lo et al. 2017). The difference between two different models is the frequency of CTS treatment in diabetic animals. Dr. Lo only treated diabetic rats with CTS in a single dose, and in our GDM mouse model, we administrated the mice with CTS via gavage daily for 18 consecutive days. Our long-term treatment, which is totally different from single dose treatment, could cause the accumulation of CTS in the body and have more obvious effects on decreasing blood glucose levels. That might explain that why our results are not consistent with previous paper.
In summary, our results indicate that CTS could effectively improve GDM by reducing oxidative stress, alleviating inflammation and inhibiting NF-κB signaling pathway in the placenta and blood of GDM mice. Our findings highlight the potential of CTS as a promising drug to treat GDM.
References
Alfadhli EM (2015) Gestational diabetes mellitus. Saudi Med J 36:399–406. https://doi.org/10.15537/smj.2015.4.10307
Badehnoosh B, Karamali M, Zarrati M, Jamilian M, Bahmani F, Tajabadi-Ebrahimi M, Jafari P, Rahmani E, Asemi Z (2018) The effects of probiotic supplementation on biomarkers of inflammation, oxidative stress and pregnancy outcomes in gestational diabetes. J Matern Fetal Neonatal Med 31:1128–1136. https://doi.org/10.1080/14767058.2017.1310193
Chen W, Lu Y, Chen G, Huang S (2013) Molecular evidence of cryptotanshinone for treatment and prevention of human cancer. Anticancer Agents Med Chem 13:979–987. https://doi.org/10.2174/18715206113139990115
Chiefari E, Arcidiacono B, Foti D, Brunetti A (2017) Gestational diabetes mellitus: an updated overview. J Endocrinol Invest 40:899–909. https://doi.org/10.1007/s40618-016-0607-5
Chong CM, Su H, Lu JJ, Wang Y (2019) The effects of bioactive components from the rhizome of Salvia miltiorrhiza (Danshen) on the characteristics of Alzheimer’s disease. Chin Med 14:19. https://doi.org/10.1186/s13020-019-0242-0
Coughlan MT, Permezel M, Georgiou HM, Rice GE (2004) Repression of oxidant-induced nuclear factor-kappaB activity mediates placental cytokine responses in gestational diabetes. J Clin Endocrinol Metab 89:3585–3594. https://doi.org/10.1210/jc.2003-031953
Feng H, Su R, Song Y, Wang C, Lin L, Ma J, Yang H (2016) Positive correlation between enhanced expression of TLR4/MyD88/NF-kappaB with insulin resistance in placentae of gestational diabetes mellitus. PLoS ONE 11:e0157185. https://doi.org/10.1371/journal.pone.0157185
Fu J, Fu J, Yuan J, Zhang N, Gao B, Fu G, Tu Y, Zhang Y (2012) Anti-diabetic activities of Acanthopanax senticosus polysaccharide (ASP) in combination with metformin. Int J Biol Macromol 50:619–623. https://doi.org/10.1016/j.ijbiomac.2012.01.034
Goh JE, Sadler L, Rowan J (2011) Metformin for gestational diabetes in routine clinical practice. Diabet Med 28:1082–1087. https://doi.org/10.1111/j.1464-5491.2011.03361.x
Guo Y, Li Y, Xue L, Severino RP, Gao S, Niu J, Qin LP, Zhang D, Bromme D (2014) Salvia miltiorrhiza: an ancient Chinese herbal medicine as a source for anti-osteoporotic drugs. J Ethnopharmacol 155:1401–1416. https://doi.org/10.1016/j.jep.2014.07.058
Jiang G, Liu J, Ren B, Zhang L, Owusu L, Liu L, Zhang J, Tang Y, Li W (2017) Anti-tumor and chemosensitization effects of Cryptotanshinone extracted from Salvia miltiorrhiza Bge. on ovarian cancer cells in vitro. J Ethnopharmacol 205:33–40. https://doi.org/10.1016/j.jep.2017.04.026
Khambule L, George JA (2019) The role of inflammation in the development of GDM and the use of markers of inflammation in GDM screening. Adv Exp Med Biol 1134:217–242. https://doi.org/10.1007/978-3-030-12668-1_12
Kuzmicki M, Telejko B, Wawrusiewicz-Kurylonek N, Lipinska D, Pliszka J, Wilk J, Zielinska A, Skibicka J, Szamatowicz J, Kretowski A, Gorska M (2013) The expression of genes involved in NF-kappaB activation in peripheral blood mononuclear cells of patients with gestational diabetes. Eur J Endocrinol 168:419–427. https://doi.org/10.1530/eje-12-0654
Liu S, Han Z, Trivett AL, Lin H, Hannifin S, Yang OJJ (2019) Cryptotanshinone has curative dual anti-proliferative and immunotherapeutic effects on mouse Lewis lung carcinoma. Cancer Immunol Immunother 68:1059–1071. https://doi.org/10.1007/s00262-019-02326-8
Liu Z, Luo H, Zhang L, Huang Y, Liu B, Ma K, Feng J, Xie J, Zheng J, Hu J, Zhan S, Zhu Y, Xu Q, Kong W, Wang X (2012) Hyperhomocysteinemia exaggerates adventitial inflammation and angiotensin II-induced abdominal aortic aneurysm in mice. Circ Res 111:1261–1273. https://doi.org/10.1161/circresaha.112.270520
Lo SH, Hsu CT, Niu HS, Niu CS, Cheng JT, Chen ZC (2017) Cryptotanshinone inhibits STAT3 signaling to alleviate cardiac fibrosis in Type 1-like diabetic rats. Phytother Res 31:638–646. https://doi.org/10.1002/ptr.5777
Ma Y, Li H, Yue Z, Guo J, Xu S, Xu J, Jia Y, Yu N, Zhang B, Liu S, Liu M, Shao W, Chen S, Liu P (2014) Cryptotanshinone attenuates cardiac fibrosis via downregulation of COX-2, NOX-2, and NOX-4. J Cardiovasc Pharmacol 64:28–37. https://doi.org/10.1097/fjc.0000000000000086
Pantham P, Aye IL, Powell TL (2015) Inflammation in maternal obesity and gestational diabetes mellitus. Placenta 36:709–715. https://doi.org/10.1016/j.placenta.2015.04.006
Ren J, Fu L, Nile SH, Zhang J, Kai G (2019) Salvia miltiorrhiza in treating cardiovascular diseases: a review on its pharmacological and clinical applications. Front Pharmacol 10:753. https://doi.org/10.3389/fphar.2019.00753
Suman RK, Mohanty IR, Maheshwari U, Borde MK, Deshmukh YA (2016) Natural dipeptidyl peptidase-IV inhibitor mangiferin mitigates diabetes- and metabolic syndrome-induced changes in experimental rats. Diabetes Metab Syndr Obes 9:261–272. https://doi.org/10.2147/dmso.s109599
Sun HN, Luo YH, Meng LQ, Piao XJ, Wang Y, Wang JR, Wang H, Zhang Y, Li JQ, Xu WT, Liu Y, Zhang Y, Zhang T, Han YH, Jin MH, Shen GN, Zang YQ, Cao LK, Zhang DJ, Jin CH (2019) Cryptotanshinone induces reactive oxygen speciesmediated apoptosis in human rheumatoid arthritis fibroblastlike synoviocytes. Int J Mol Med 43:1067–1075. https://doi.org/10.3892/ijmm.2018.4012
Wang W, Huang M, Hui Y, Yuan P, Guo X, Wang K (2018a) Cryptotanshinone inhibits RANKL-induced osteoclastogenesis by regulating ERK and NF-kappaB signaling pathways. J Cell Biochem. https://doi.org/10.1002/jcb.28008
Wang W, Wang X, Zhang XS, Liang CZ (2018b) Cryptotanshinone attenuates oxidative stress and inflammation through the regulation of Nrf-2 and NF-kappaB in mice with unilateral ureteral obstruction. Basic Clin Pharmacol Toxicol 123:714–720. https://doi.org/10.1111/bcpt.13091
Wang Y, Lu HL, Liu YD, Yang LY, Jiang QK, Zhu XJ, Fan HN, Qian Y (2017) Cryptotanshinone sensitizes antitumor effect of paclitaxel on tongue squamous cell carcinoma growth by inhibiting the JAK/STAT3 signaling pathway. Biomed Pharmacother 95:1388–1396. https://doi.org/10.1016/j.biopha.2017.09.062
Wei B, Ta N, Li J, Zhang JF, Chen BL (2010) Relationship between the inhibition of NF-kappaB and insulin resistance in uterrus of rats with gestational diabetes mellitus. Xi Bao Yu Fen Zi Mian Yi Xue Za Zhi 26(235–237):241
Wensel TM (2009) Role of metformin in the treatment of gestational diabetes. Ann Pharmacother 43:939–943. https://doi.org/10.1345/aph.1L562
Yao L, Wan J, Li H, Ding J, Wang Y, Wang X, Li M (2015) Resveratrol relieves gestational diabetes mellitus in mice through activating AMPK. Reprod Biol Endocrinol 13:118. https://doi.org/10.1186/s12958-015-0114-0
Yu B, Liu Z, Fu Y, Wang Y, Zhang L, Cai Z, Yu F, Wang X, Zhou J, Kong W (2017) CYLD deubiquitinates nicotinamide adenine dinucleotide phosphate oxidase 4 contributing to adventitial remodeling. Arterioscler Thromb Vasc Biol 37:1698–1709. https://doi.org/10.1161/atvbaha.117.309859
Zeng Z, Xu Y, Zhang B (2017) Antidiabetic activity of a lotus leaf selenium (Se)-polysaccharide in rats with gestational diabetes mellitus. Biol Trace Elem Res 176:321–327. https://doi.org/10.1007/s12011-016-0829-6
Zhu R, Wang W, Yang S (2019) Cryptotanshinone inhibits hypoxia/reoxygenation-induced oxidative stress and apoptosis in renal tubular epithelial cells. J Cell Biochem 120:13354–13360. https://doi.org/10.1002/jcb.28609
Acknowledgements
None.
Author information
Authors and Affiliations
Corresponding author
Ethics declarations
Conflicts of interest
No competing financial interests exist.
Additional information
Publisher's Note
Springer Nature remains neutral with regard to jurisdictional claims in published maps and institutional affiliations.
Electronic supplementary material
Below is the link to the electronic supplementary material.
Rights and permissions
About this article
Cite this article
Wang, N., Dong, X., Shi, D. et al. Cryptotanshinone ameliorates placental oxidative stress and inflammation in mice with gestational diabetes mellitus. Arch. Pharm. Res. 43, 755–764 (2020). https://doi.org/10.1007/s12272-020-01242-1
Received:
Accepted:
Published:
Issue Date:
DOI: https://doi.org/10.1007/s12272-020-01242-1