Abstract
Background
The tumor microenvironment (TME) regulates tumor progression, and cancer-associated fibroblasts (CAFs) are the primary stromal components of the TME, with the potential to drive tumor metastasis via the secretion of paracrine factors, but the specific mechanisms driving this process have not been defined.
Methods
Proteins secreted from CAFs and normal fibroblasts (NFs) were analyzed via proteomic analysis (fold change > 2, p < 0.05) to identify tumor-promoting proteins secreted by CAFs.
Results
Proteomic analysis revealed that microfibrillar-associated protein 5 (MFAP5) is preferentially expressed and secreted by CAFs relative to NFs, which was confirmed by Western blotting and RT-qPCR. Transwell and wound healing assays confirmed that MFAP5 is secreted by CAFs, and drives the invasion and migration of MCF7 breast cancer cells. We further found that in MCF7 cells MFAP5 promoted epithelial–mesenchymal transition, activating Notch1 signaling and consequently upregulating NICD1 and slug. When Notch1 was knocked down in MCF7 cells, the ability of MFAP5 to promote invasion and migration decreased.
Conclusion
CAFs promote cancer cells invasion and migration via MFAP5 secretion and activation of the Notch1/slug signaling. These data highlight this pathway as a therapeutic target to disrupt tumor progression through the interference of CAF–tumor crosstalk.
Similar content being viewed by others
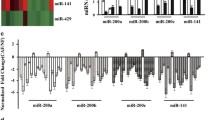
Avoid common mistakes on your manuscript.
Introduction
Breast cancer remains the most common cancer in women, with 1.7 million diagnoses and 535,000 deaths in 2016 alone [1]. Recurrence and metastasis remain the leading cause of death in breast cancer patients [2]. The tumor microenvironment (TME) is a key regulator of tumor metastasis and invasion, with cancer-associated fibroblasts (CAFs) in particular being associated with poor breast cancer prognosis, which are detectable in the peripheral blood of advanced breast cancer patients [3]. CAFs secrete growth factors and chemokines and reshape the extracellular matrix, thereby driving proximal invasion and metastasis [4]. The exact mechanisms underlying these processes remain unknown. Understanding the CAF secretome can enhance our knowledge of the TME and improve breast cancer treatment.
Epithelial–mesenchymal transition (EMT) is an important stage in tumor progression, that is characterized by reduced cell polarity and adhesion, increased motility, reduced epithelial marker expression (E-cadherin) and elevated mesenchymal marker expression (vimentin, N-cadherin) [5]. Snail, Twist, and ZEB are key transcription factors regulating EMT in tumors, with higher levels of these factors associated with poor patient prognosis [6]. CAFs are able to promote tumor EMT via paracrine secretion of the growth factors TGFβ and HGF, and the inflammatory factors IL-6 and TNFα [7]. How other secreted proteins influence this process, however, remains uncertain and requires further investigation.
MFAP5 is a key component of the elastic microfibers that constitute the extracellular matrix, which are important for the growth of bone and many other organs [8, 9]. Recently, MFAP-5 expression was shown to be elevated in an array of malignant tumors, and drives tumor invasion and metastasis [10,11,12]. In particular, MFAP5 derived from CAFs can promote tumor progression in ovarian cancer and oral squamous cell carcinoma [13, 14]. MFAP5 overexpression in breast cancer epithelial cells leads to bone metastases via the ERK/MMP pathway [15], although this represents an autocrine effect within carcinoma cells. The potential for stromal-derived MFAP5 to influence breast cancer epithelial cells via paracrine signaling has not been investigated.
In this study, to identify key proteins secreted by CAFs that promote breast cancer cell invasion and metastasis, and to provide new tumor microenvironment markers for the prognosis evaluation of breast cancer, we analyzed the supernatants of breast cancer patients’ fibroblast cells, and found that MFAP5 expression was elevated in breast CAFs relative to NFs. We also found that MFAP5 secreted by CAFs drives the invasion and migration of MCF7 breast cancer cells in a paracrine fashion. MFAP5 was further found to activate Notch1 and upregulate slug in these cells. Together, these results provide new evidence for the crosstalk between breast cancer tumor cells and local fibroblasts. This represents a potential marker from the TME to predict breast cancer recurrence and metastasis.
Materials and methods
Cells and cell culture
Fibroblast isolation
For CAF and NF isolation, tumor and normal breast tissues (> 2 cm from the tumor) were obtained from breast cancer patients undergoing primary lesion resection at the Xuanwu Hospital, Capital Medical University. No patients had received radiotherapy, chemotherapy, or endocrine therapy, and all consented to the use of resected specimens. The Institutional Review Board and the Human Ethics Committee of Xuanwu Hospital approved this study.
Paired fibroblast samples were isolated and cultured as previously described [16]. CAFs and NFs were grown in DMEM (Gibco, Waltham, MA, USA) containing 10% FBS at 37 °C in a humidified 5% CO2 incubator. Cells within six passages were used for experimental analysis.
Cell lines and reagents
The MCF-7 human breast cancer line was purchased from Cobioer Biosciences Corporation (Nanjing, China) and was grown under the conditions used for primary fibroblasts. MFAP5 human siRNA (SR305355) was purchased from Origene (USA), and Notch1 human siRNA was purchased from Genechem (Shanghai, China; sequence: CCAACAUCCAGGACAACAUTT). Cells were transfected with Lipofectamine 3000 (Invitrogen). Recombinant Human MFAP5 (4914-MG) was purchased from R&D.
Conditioned media generation
Fibroblasts were washed three times in PBS, and grown in serum-free DMEM for 24 h after reaching 70–80% confluency. Conditioned media from the cells was collected, centrifuged at 4 °C 1000 rpm for 5 min, and subjected to mass spectrometry and other experimental analysis.
Sample preparation and digestion for proteomic analysis
CAF and NF supernatants were mixed 4:1 with 100% (w/v) trichloroacetic acid, incubated for 1 h at 4 °C, and spun at 14,000 rpm for 5 min at 4 °C. Precipitates were washed in ice-cold acetone, air dried at room temperature, and dissolved in lysis buffer (8 M urea, 100 mM Tris–HCl, pH 8.0). Bradford assays (Eppendorf BioSpectrometer, Hamburg, Germany) were used to measure protein concentrations, and samples were then digested as previously described [16]. Briefly, samples were incubated in dithiothreitol (DTT) and centrifuged (Millipore) at 12,000g for 20 min. Then, the protein samples were washed in lysis buffer and digested with trypsin overnight at 37 °C.
Liquid chromatography and mass spectrometry
A nanoliter liquid phase Easy-nLC 1000 (Thermo Scientific, USA) hybrid with a mass spectrometer OrbiTrap Fusion LUMOS (Thermo Scientific, USA) was used for mass spectrometry (MS). We resolved 0.5 μg of sample in the mobile phase: A: 0.1% formic acid in water; B: 0.1% formic acid in acetonitrile. Separation was performed on an Easy-spray column (C18, 3 μm, 100 Å, 75 μm × 15 cm) with a liquid phase gradient of: 4 min, 8% B phase; 45 min, 30% phase B; 75 min, 90% B phase; 78 min, 90% phase B. The mass spectrometer was manipulated in the MS/MS data-dependent mode, with an electrospray voltage of 2.0 kV and a capillary temperature of 300 °C. Full scans were performed ranging from 300 to 1400 m/z, and the resolution was set to 30,000. The quadrupole isolation mode was selected to isolate precursor ions within a 1.6 Da window. Dynamic exclusion was determined at 20 s.
MS results were searched using Proteome Discoverer 2.2 analysis software with arginine (R) and lysine (K) enzymatic sites. Two cleavage sites were permitted, allowing for one-sided non-specific enzymatic hydrolysis, with a fixed modification set to carbamidomethylation of cysteine. The variable modifier was set to oxidation/+ 15.995 Da (M).
Western blotting
Cells were lysed in RIPA buffer (Beyotime Bio, China) containing PMSF and protease inhibitor cocktail. Proteins were quantified by BCA assays (Thermo Scientific, USA). Protein samples were resolved on 8–12% SDS-PAGE electrophoresis gels, and then transferred onto PVDF membranes. Membranes were blocked in 5% milk for 1 h at room temperature, followed by probing overnight at 4 °C with primary antibodies against β-actin (1:2000), GAPDH (1:2000), MFAP5 (1:500), E-cadherin (1:2000), N-cadherin (1:1000), Notch1(1:500) (all from Proteintech, USA), and slug (1:1000, Abcam, USA). Membranes were labeled with HRP-conjugated goat anti-rabbit or -mouse IgG (1:2000, Santa Cruz, USA) for 1 h at room temperature. An enhanced chemiluminescence system (Millipore, Germany) was used for protein detection on an AI600 imaging system (GE, USA).
RT-qPCR
Cells were lysed in TRIzol (Invitrogen, Chicago, USA) to extract RNA, and cDNA was generated using Revert Aid first strand cDNA synthesis kits (k1622, Thermo Scientific, USA). The Mx3000p RT-PCR system was used for RT-qPCR using THUNDERBIRD SYBR qPCR Mix (QPS-201, TOYOBO, Shanghai, China). Results were normalized to GAPDH expression. Primers were synthesized by Sangong Biotech (Shanghai, China) (Table 1).
Wound healing assays
MCF7 cells (8 × 104) were plated into 12-well plates overnight until the cells reached confluency. A pipette was used to scratch the monolayer, which was washed in PBS. Serum-free media (1 mL) were added and cells were imaged after 24 h on a light microscope (200 ×). Wound healing was quantified using ImageJ.
Invasion assay
Matrigel (BD, USA) was diluted 1:4 in DMEM and 25 μL was added to 8 um pore Transwell chamber filters in 24-well plates (Corning, USA). MCF7 cells (3 × 104) were added to the upper chambers in 100 μL DMEM. CAF-conditioned media containing 10% FBS (1 mL) was added to the lower chamber. After 48 h incubation, cells in the upper chamber were removed via swabbing. Remaining cells were fixed in 4% formaldehyde for 5 min, followed by staining with 5% crystal violet for 5 min. Cells on the lower inserts were counted via microscopy (200 ×).
Immunofluorescence
MCF7 cells were seeded onto coverslips in 6-well plates (2 × 104/mL). After overnight incubation, adherent cells were treated with or without recombinant MFAP5 (400 ng/mL) for 48 h. Cells were then washed in PBS and fixed in 4% paraformaldehyde for 20 min at room temperature. After three more washes, slides were blocked using methanol containing hydrogen peroxide for 10 min, and probed with anti-E-cadherin antibodies (1:200, Santa Cruz, USA) at 4 °C overnight followed by an anti-rabbit secondary antibodies for 1 h at room temperature. Cell nuclei were stained with 4′,6-diamidinophenyl-indole (DAPI) for 5 min, and then slides were imaged via fluorescence microscopy (200 ×).
Statistical analysis
SPSS v21.0 was used for all analyses. A Student’s t test was used to compare values. Data are presented as the mean ± SD of triplicate experiments. p < 0.05 indicated significant difference.
Results
Primary breast cancer CAF and NF supernatant proteomic profiling identifies MFAP5 as a CAF-associated protein
When activated, fibroblasts provide a paracrine signaling-mediated niche for tumorigenesis. To identify potential paracrine factors secreted by CAFs that promote oncogenesis, we profiled the secreted proteome of matched pairs of CAFs and NFs from four breast cancer patients. R packages were used to identify differentially expressed proteins (DEPs) between samples (fold change > 2, p < 0.05), identifying 36 DEPs, of which 20 were upregulated in CAFs, and 16 proteins were downregulated (Fig. 1a). Given our interest in tumor-promoting factors, we next assessed the upregulated proteins in CAFS via GO analysis of these 20 proteins. The cell component (CC), biological processes (BP) and molecular function (MF) analyses primarily revealed these to primarily be extracellular matrix proteins (Fig. 1b). Of these DEPs, MFAP5 expression was highest in CAFs. Previous studies identified high MFAP5 expression in tumors, which is related to invasion and metastasis, making it an interesting target for further studies. Kaplan–Meier survival analysis of the 1747 breast cancer patients in the Kaplan–Meier Plotter public database revealed that patients with increased MFAP5 expression had lower distant metastasis-free survival rates (DMFS) (HR = 1.29, p < 0.05), suggesting that MFAP5 expression is linked to poor breast cancer prognosis (Fig. 1c).
Differential analysis of the expression profiles of CAF and NF secreted proteins. a Heatmap of the differentially expressed secretory proteins from primary CAFs/NFs (one-way ANOVA, fold change > 2, p < 0.05). b GO analyses were performed on the upregulated proteins of CAFs using DAVID (https://david.ncifcrf.gov/), focusing on cell components (CC), biological processes (BP), and molecular functions (MF). c Prognosis of MFAP5 in breast cancer was identified via the Kaplan–Meier Plotter public database (https://kmplot.com/analysis/)
CAF-derived MFAP5 induces breast cancer cell invasion and metastasis
To confirm that CAFs express high levels of MFAP5, its expression was assessed via Western blotting and RT-qPCR (Fig. 2a, b). In MCF7 cells, the protein levels of MFAP5 were lower than in CAFs (Fig. 2c).To determine how CAF-derived MFAP5 influences breast cancer invasion and migration, MFAP5 was knocked down in CAFs to generate conditioned media containing reduced MFAP5 levels (Fig. 2d, e). Wound healing and Transwell assays revealed that MFAP5 knockdown in CAFs was sufficient to reduce the ability of these cells to promote tumor migration (p < 0.001; two-tailed Student’s t test) (Fig. 2f, g).
Analysis of MFAP5 expression in CAFs and its effects on breast cancer cell motility. a, b Western blot and RT-qPCR analyses demonstrated increased MFAP5 protein and RNA expression in primary CAFs relative to matched NFs. c Western blotting indicated increased MFAP5 expression in CAFs relative to MCF7 breast cancer cells. d, e Western blotting and RT-qPCR were used to verify MFAP5 knockdown in CAFs. f, g Invasion (48 h) and wound healing assays (24 h) demonstrated the effects of CAF-conditioned media on MCF7 motility. Cells were treated with DMEM (MOCK), or conditioned media from NC-siRNA (si-NC) or MFAP5-siRNA (si-MFAP5) transfected CAFs. Data are presented as mean ± SD, *p < 0.05, ***p < 0.001, ****p < 0.0001. Data were analyzed using a Student’s t test
MFAP5 promotes breast cancer cell EMT
Epithelial–mesenchymal transition is a key stage in tumor progression. To explore whether CAF-derived MFAP5 influences EMT in MCF7 cells, we added recombinant human MFAP5 to MCF7 culture media, which resulted in decreased E-cadherin expression and increased N-cadherin expression relative to untreated cells, consistent with EMT progression (Fig. 3a, b). Twist and slug are major transcription factors that control EMT, and we found that MFAP5 increased slug expression in MCF7 cells via RT-qPCR (p < 0.001), whilst Twist was unaffected (P > 0.05) (Fig. 3c).
Effects of exogenous MFAP5 on breast cancer cell EMT. a Fluorescence intensity of E-cadherin in MCF7 cells cultured with recombinant MFAP5 (400 ng/mL, 48 h). b Western blot analysis was used to compare E- and N-cadherin levels in MCF7 cells cultured with recombinant human MFAP5 (200 ng/mL and 400 ng/mL for 48 h) relative to negative controls (C0). c RT-qPCR analysis demonstrating slug and Twist mRNA expression in MCF7 cells cultured with or without recombinant human MFAP5 (400 ng/mL for 48 h). Data are mean ± SD, ***p < 0.001. Data were analyzed using a Student’s t test
MFAP5 activates Notch1 signaling in breast cancer cells
Notch1 is a key regulator of EMT and associated cancer progression. An assessment of the STRING protein interaction network revealed that MFAP5 can potentially interact with the Notch1 receptor and the Jag1 Notch ligand, suggesting MFAP5 may mediate paracrine activation of Notch1 signaling in MCF7 cells (Fig. 4). Western blotting revealed that exogenous MFAP5 induced Notch1 activation and NICD1 upregulation in a concentration-dependent manner, corresponding to increased slug expression (p < 0.05) (Fig. 5a).
Analysis of MFAP5 functional protein interaction networks. Maps were produced using the STRING database according to the highest confidence setting (0.900), with different line colors indicating different protein–protein interactions. For example, blue lines indicate evidence from curated databases, while yellow lines indicate evidence from text mining
Exogenous MFAP5 promotes breast cancer cell invasion and migration via Notch1 signaling. a Western blot analysis was used to measure NICD1 and slug levels in MCF7 cells treated with recombinant MFAP5 for 48 h. b, c Western blot and RT-qPCR analyses verified Notch1 knockdown in MCF7 cells. d, e Effects of exogenous MFAP5 on MCF7 migration and invasion following Notch1-knockdown (si-Notch1) relative to controls (si-NC). f Western blot analysis of E- and N-cadherin levels in Notch1-knockdown and control MCF7 cells. g Western blot analysis of slug expression in si-NC and si-Notch1 MCF7 cells cultured with or without 400 ng/mL recombinant MFAP5 for 48 h. Data are mean ± SD, *p < 0.05, **p < 0.01, ***p < 0.001, ****p < 0.0001. Data were analyzed using a Student’s t test
Notch1 ablates the ability of MFAP5 to promote breast cancer cell invasion and migration
To further confirm that MFAP5 promotes MCF7 invasion and migration via Notch1 signaling, we knocked down Notch1 in MCF7 cells (Fig. 5b, c). Wound healing and Transwell assays revealed that Notch1 knockdown in MCF7 cells substantially impaired the MFAP5-mediated enhancement of breast cancer cell migration and invasion (Fig. 5d, e). Western blotting also confirmed increased levels of the epithelial marker E-cadherin in MCF7 cells in which Notch1 was knocked down (Fig. 5f). The ability of MFAP5 to promote slug upregulation in these cells was also significantly reduced following Notch1 knockdown (Fig. 5g).
Discussion
The tumorigenic potential of tumor stromal fibroblasts as the primary cell type promoting the formation of the TME are well defined. We have previously shown that CAF-conditioned media can drive MCF7 cell invasion to higher levels than NF-conditioned media [16]. In this study, to identify key proteins from tumor fibroblasts that drive tumor progression, we assessed the secreted proteins in primary CAFs and NFs from four breast cancer patients. In total, we detected 2795 proteins in CAFs, of which 36 were differentially expressed (20 upregulated, 16 downregulated). Functional analysis revealed these DEPs to be extracellular matrix proteins, consistent with the fact that altered extracellular matrix component production is linked to oncogenesis [17, 18]. These results suggest that breast cancer-associated fibroblasts secrete extracellular matrix proteins which enhance tumor progression.
MFAP5 is a matrix glycoprotein composed of microfibrils that are present in elastic tissues including ligaments, arteries, and the lungs [19]. However, MFAP5 is overexpressed in several cancers, and is associated with metastasis and drug resistance. Paracrine MFAP5 produced by fibroblasts can drive invasion and metastasis of ovarian cancer or oral squamous cell carcinoma cells [13, 14]. In this study, we found that MFAP5 is significantly upregulated and secreted by CAFs relative to NFs. Upon MFAP5 silencing, CAFs lost their ability to promote MCF7 cell migration, indicating its role in enhancing breast cancer cell metastasis.
EMT is essential to tumor invasion and metastasis [20]. We found that exogenous MFAP5 led to the downregulation of E-cadherin (epithelial marker) and the upregulation of N-cadherin (mesenchymal marker) in MCF7 cells, which was associated with an MFAP5-dependent increase in expression of the slug transcription factor. Li et al. recently found that epithelium-derived MFAP5 drives EMT in cervical cancer cells [10]. Together, our data show that MFAP5 promotes EMT, suggesting this to be a key mechanism by which CAFs promote breast cancer progression. In addition, CAFs drive breast cancer cell EMT through the secretion of other growth factors including TGFβ or inflammatory factors including CXCL12 and IL32 [21,22,23]. In this study, we found that only TGFβ was differentially regulated in the CAFs and NFs, but the trends were not stable. Liu et al. showed that TGFβ or Notch inhibitor can reverse the enhancing effect of MFAP5 on triple-negative breast cancer cells metastasis [24]. Whether an interaction between MFAP5 and TGFβ secreted by CAFs exists requires further investigation.
We also found that MFAP5 promotes MCF7 migration via Notch1. Previous reports suggest that MFAP5 can bind to the αvβ3 cell surface integrin receptor via its N-terminal RGD domain [19]. Recently, Miyanoto et al. found that MFAP5 also activates the Notch1 receptor via its C-terminal domain that interacts with Notch EGF-like repeats [25, 26]. This effect differs between different tissue types. Albig et al. showed that MFAP5 drives vascular endothelial cell proliferation via disrupting Notch1 activation, whilst the opposite scenario occurs in non-endothelial cells [27]. Deford et al. suggested that the outcome of MFAP5-Notch1 binding is dependent on the expression level of integrin αvβ3 receptor on the cell surface [28]. Cells with high αvβ3 receptor levels preferentially bind the MFAP5 N-terminal domain, thus activating the integrin pathway and competitively inhibiting Notch1 activation, whereas in cells lacking integrin αvβ3 receptor, the MFAP5 C-terminal domain binds to the Notch1 receptor and triggers Notch1 signaling. We found that exogenous MFAP5 induced Notch1 activation in MCF7 cells, possibly due to the αvβ3 receptor being poorly expressed on these cells. Confirmation of this is required in future studies [29,30,31].
Slug is a transcription factor that regulates EMT. When Notch1 was silenced in MCF7 cells, MFAP5-mediated slug upregulation was inhibited, indicating that MFAP5 activates slug via the Notch1 pathway. Shao et al. found that NICD1, an intracellular protein downstream of Notch1, regulated both breast cancer cell EMT and subsequent slug activation/upregulation [32]. Based on these findings, we conclude that MFAP5 promotes EMT in MCF7 cells via activating Notch1/slug signaling.
We have produced novel evidence that MFAP5 is secreted by breast cancer stromal fibroblasts, and that it drives MCF7 breast cancer cell EMT via activating the Notch1/slug pathway, enhancing the invasion and migration of these cells. This offers new information regarding the role of the TME in promoting breast cancer metastasis, and provides a potential marker and research target from tumor stroma to predict breast cancer prognosis. Even so, these findings are preliminary in vitro studies and future in vivo and clinical studies are now required to verify these findings.
References
Fitzmaurice C, Allen C, Barber RM, Barregard L, Bhutta ZA, Brenner H, et al. Global, regional, and national cancer incidence, mortality, years of life lost, years lived with disability, and disability-adjusted life-years for 32 cancer groups, 1990 to 2015. JAMA Oncol. 2017;3(4):524. https://doi.org/10.1001/jamaoncol.2016.5688.
Ghoncheh M, Pournamdar Z, Salehiniya H. Incidence and mortality and epidemiology of breast cancer in the world. Asian Pac J Cancer Prev. 2016;17(S3):43–6.
Ao Z, Shah SH, Machlin LM, Parajuli R, Miller PC, Rawal S, et al. Identification of cancer-associated fibroblasts in circulating blood from patients with metastatic breast cancer. Cancer Res. 2015;75(22):4681–7. https://doi.org/10.1158/0008-5472.CAN-15-1633.
Chen X, Song E. Turning foes to friends: targeting cancer-associated fibroblasts. Nat Rev Drug Discov. 2018;18:99–115. https://doi.org/10.1038/s41573-018-0004-1.
Lamouille S, Xu J, Derynck R. Molecular mechanisms of epithelial–mesenchymal transition. Nat Rev Mol Cell Biol. 2014;15(3):178–96. https://doi.org/10.1038/nrm3758.
Sanchez-Tillo E, Liu Y, de Barrios O, Siles L, Fanlo L, Cuatrecasas M, et al. EMT-activating transcription factors in cancer: beyond EMT and tumor invasiveness. Cell Mol Life Sci. 2012;69(20):3429–56. https://doi.org/10.1007/s00018-012-1122-2.
Guo S, Deng CX. Effect of stromal cells in tumor microenvironment on metastasis initiation. Int J Biol Sci. 2018;14(14):2083–93. https://doi.org/10.7150/ijbs.25720.
Combs MD, Knutsen RH, Broekelmann TJ, Toennies HM, Brett TJ, Miller CA, et al. Microfibril-associated glycoprotein 2 (MAGP2) loss of function has pleiotropic effects in vivo. J Biol Chem. 2013;288(40):28869–80. https://doi.org/10.1074/jbc.M113.497727.
Barbier M, Gross MS, Aubart M, Hanna N, Kessler K, Guo DC, et al. MFAP5 loss-of-function mutations underscore the involvement of matrix alteration in the pathogenesis of familial thoracic aortic aneurysms and dissections. Am J Hum Genet. 2014;95(6):736–43. https://doi.org/10.1016/j.ajhg.2014.10.018.
Li Q, Zhang Y, Jiang Q. MFAP5 suppression inhibits migration/invasion, regulates cell cycle and induces apoptosis via promoting ROS production in cervical cancer. Biochem Biophys Res Commun. 2018;507(1–4):51–8. https://doi.org/10.1016/j.bbrc.2018.10.146.
Dawoud MM, Abouelfadl D, Abdou AG, Elkhouly E. Immunohistochemical expression of microfibrillar-associated protein 5 (MFAP5) in invasive breast carcinoma of no special type. Appl Immunohistochem Mol Morphol. 2018. https://doi.org/10.1097/PAI.0000000000000686.
Saikawa S, Kaji K, Nishimura N, Seki K, Sato S, Nakanishi K, et al. Angiotensin receptor blockade attenuates cholangiocarcinoma cell growth by inhibiting the oncogenic activity of Yes-associated protein. Cancer Lett. 2018;434:120–9. https://doi.org/10.1016/j.canlet.2018.07.021.
Leung CS, Yeung TL, Yip KP, Pradeep S, Balasubramanian L, Liu J, et al. Calcium-dependent FAK/CREB/TNNC1 signalling mediates the effect of stromal MFAP5 on ovarian cancer metastatic potential. Nat Commun. 2014;5:5092. https://doi.org/10.1038/ncomms6092.
Principe S, Mejia-Guerrero S, Ignatchenko V, Sinha A, Ignatchenko A, Shi W, et al. Proteomic analysis of cancer-associated fibroblasts reveals a paracrine role for MFAP5 in human oral tongue squamous cell carcinoma. J Proteome Res. 2018;17(6):2045–59. https://doi.org/10.1021/acs.jproteome.7b00925.
Wu Z, Wang T, Fang M, Huang W, Sun Z, Xiao J, et al. MFAP5 promotes tumor progression and bone metastasis by regulating ERK/MMP signaling pathways in breast cancer. Biochem Biophys Res Commun. 2018;498(3):495–501. https://doi.org/10.1016/j.bbrc.2018.03.007.
Wang B, Xi C, Liu M, Sun H, Liu S, Song L, et al. Breast fibroblasts in both cancer and normal tissues induce phenotypic transformation of breast cancer stem cells: a preliminary study. PeerJ. 2018;6:e4805. https://doi.org/10.7717/peerj.4805.
Robertson C. The extracellular matrix in breast cancer predicts prognosis through composition, splicing, and crosslinking. Exp Cell Res. 2016;343(1):73–81. https://doi.org/10.1016/j.yexcr.2015.11.009.
Insua-Rodriguez J, Oskarsson T. The extracellular matrix in breast cancer. Adv Drug Deliv Rev. 2016;97:41–55. https://doi.org/10.1016/j.addr.2015.12.017.
Gibson MA, Leavesley DI, Ashman LK. Microfibril-associated glycoprotein-2 specifically interacts with a range of bovine and human cell types via alphaVbeta3 integrin. J Biol Chem. 1999;274(19):13060–5.
Yeung KT, Yang J. Epithelial–mesenchymal transition in tumor metastasis. Mol Oncol. 2017;11(1):28–39. https://doi.org/10.1002/1878-0261.12017.
Yu Y, Xiao CH, Tan LD, Wang QS, Li XQ, Feng YM. Cancer-associated fibroblasts induce epithelial–mesenchymal transition of breast cancer cells through paracrine TGF-beta signalling. Br J Cancer. 2014;110(3):724–32. https://doi.org/10.1038/bjc.2013.768.
Wen S, Hou Y, Fu L, Xi L, Yang D, Zhao M, et al. Cancer-associated fibroblast (CAF)-derived IL32 promotes breast cancer cell invasion and metastasis via integrin beta3-p38 MAPK signalling. Cancer Lett. 2019;442:320–32. https://doi.org/10.1016/j.canlet.2018.10.015.
Shan S, Lv Q, Zhao Y, Liu C, Sun Y, Xi K, et al. Wnt/beta-catenin pathway is required for epithelial to mesenchymal transition in CXCL12 over expressed breast cancer cells. Int J Clin Exp Pathol. 2015;8(10):12357–67.
Wu Y, Wu P, Zhang Q, Chen W, Liu X, Zheng W. MFAP5 promotes basal-like breast cancer progression by activating the EMT program. Cell Biosci. 2019;9:24. https://doi.org/10.1186/s13578-019-0284-0.
Miyamoto A, Lau R, Hein PW, Shipley JM, Weinmaster G. Microfibrillar proteins MAGP-1 and MAGP-2 induce Notch1 extracellular domain dissociation and receptor activation. J Biol Chem. 2006;281(15):10089–97. https://doi.org/10.1074/jbc.M600298200.
Nehring LC, Miyamoto A, Hein PW, Weinmaster G, Shipley JM. The extracellular matrix protein MAGP-2 interacts with Jagged1 and induces its shedding from the cell surface. J Biol Chem. 2005;280(21):20349–55. https://doi.org/10.1074/jbc.M500273200.
Albig AR, Becenti DJ, Roy TG, Schiemann WP. Microfibril-associate glycoprotein-2 (MAGP-2) promotes angiogenic cell sprouting by blocking notch signaling in endothelial cells. Microvasc Res. 2008;76(1):7–14. https://doi.org/10.1016/j.mvr.2008.01.001.
Deford P, Brown K, Richards RL, King A, Newburn K, Westover K, et al. MAGP2 controls Notch via interactions with RGD binding integrins: identification of a novel ECM-integrin-Notch signaling axis. Exp Cell Res. 2016;341(1):84–91. https://doi.org/10.1016/j.yexcr.2016.01.011.
Carey I, Williams CL, Ways DK, Noti JD. Overexpression of protein kinase C-alpha in MCF-7 breast cancer cells results in differential regulation and expression of alphavbeta3 and alphavbeta5. Int J Oncol. 1999;15(1):127–36.
Wong NC, Mueller BM, Barbas CF, Ruminski P, Quaranta V, Lin EC, et al. Alpha V integrins mediate adhesion and migration of breast carcinoma cell lines. Clin Exp Metastasis. 1998;16(1):50–61.
Taherian A, Li X, Liu Y, Haas TA. Differences in integrin expression and signaling within human breast cancer cells. BMC Cancer. 2011;11:293. https://doi.org/10.1186/1471-2407-11-293.
Shao S, Zhao X, Zhang X, Luo M, Zuo X, Huang S, et al. Notch1 signaling regulates the epithelial–mesenchymal transition and invasion of breast cancer in a Slug-dependent manner. Mol Cancer. 2015;28(14):28. https://doi.org/10.1186/s12943-015-0295-3.
Acknowledgements
This work was supported by the National Natural Science Foundation of China (No. 81172517) and Beijing Breast Prevention Institute of Breast Cancer Prevention and Treatment Research Fund and the Beijing Municipal Health System Academic Leaders of High-Level Health Personnel Program (No. 2011-2-28).
Author information
Authors and Affiliations
Corresponding author
Ethics declarations
Conflict of interest
The authors declare that there are no competing interests.
Ethical statement
Ethics approval has been obtained from the Institutional Review Board and the Human Ethics Committee of Xuanwu Hospital. Capital Medical University.
Informed consent
Informed consent was obtained from all individual participants included in the study.
Additional information
Publisher's Note
Springer Nature remains neutral with regard to jurisdictional claims in published maps and institutional affiliations.
Rights and permissions
About this article
Cite this article
Chen, Z., Yan, X., Li, K. et al. Stromal fibroblast-derived MFAP5 promotes the invasion and migration of breast cancer cells via Notch1/slug signaling. Clin Transl Oncol 22, 522–531 (2020). https://doi.org/10.1007/s12094-019-02156-1
Received:
Accepted:
Published:
Issue Date:
DOI: https://doi.org/10.1007/s12094-019-02156-1