Abstract
The coarse perlite 40–80 mesh was selected as an immobilizing material and put into a packed bed reactor (PBR) to continuously convert maltose to isomalto-oligosaccharides (IMOs). The PBR was prepared by mixing the thermo-inactivated cells (TIC) from Aspergillus niger J2 strain with the coarse perlite, then the mixture was put into an overpressure-resistant column. Compared with diatomite 40–80 mesh and thin perlite 80–120 mesh in PBR, coarse perlite was chosen as the best filtration aid, when the ratio of coarse perlite versus TIC was 1:1. The thermal and pH stability of the free and immobilized TIC and the optimum conditions for the transglycosylation reactions were determined. The results show that approximately 75 and 82% and 87 and 91% of α-glucosidase activity were reserved for free and immobilized TIC at temperatures from 30 to 60 °C and pH from 3.00 to 7.00 for 12 h, respectively. With 30% malt syrup under the conditions of 50 °C and pH 4.00, a mini-scale packed bed reactor (Mi-PBR) and medium-scale packed bed reactor (Me-PBR) could continuously produce IMO over 25 and 34 days with the yield of effective IMO (eIMO) ≥ 35% and total IMO (tIMO) ≥ 50%, respectively. The strategy of mixing the coarse perlite with TIC in PBR is a novel approach to continuously produce IMO and has great application potential in industry.
Similar content being viewed by others
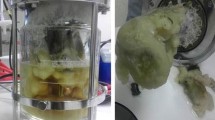
Avoid common mistakes on your manuscript.
Introduction
Isomalto-oligosaccharides (IMOs) are functional oligosaccharides [1], which contain two to ten glucose units with more than one α-(1, 6) linkages [2]. An abundant number of research have shown that IMOs play a significant role in mammalian metabolic processes [3]. The bifidus-stimulating activity of IMO helps to balance the amount of bacteria colonizing the intestinal tract [4]. Moreover, IMOs have the potential to be used as anticariogenic sweeteners because they produce low levels of insoluble dextran forming tartar [5]. Due to these beneficial physiological properties, IMOs were widely utilized for prebiotics, food additives, and feed ingredients [6,7,8].
Generally, IMOs are produced by α-glucosidase (EC 3.2.1.20) which converts hydrolysates of starch during traditional industrial processes [9, 10]. However, α-glucosidase should be isolated after the transformation, and it cannot be used repetitively. The consumption of α-glucosidase increases the cost of producing IMO. Thus, there is a need to develop an immobilized reactor for enzyme immobilization, which can reuse the enzyme as well as the apartment of the enzyme after conversion. Using immobilized enzyme reactors (IMER) for production of IMO has been previously reported [11,12,13]. A membrane reactor utilizing dextransucrase (EC 2.4.1.5) and glucanase (EC 3.2.1.11) was developed to continuously convert maltose into IMO [14]. In other work, endodextranase (EC 3.2.1.11) was immobilized on an epoxy-activated monolithic convective interaction media disk to produce IMO [15]. A novel enzyme membrane reactor with a sandwich structure was developed by confining glucosidase (EC 3.2.1.20) between two sheets of ultrafiltration membranes [16]. Synthesis of IMO by using the whole-cell biotransformation approach has also been reported [17].
Perlite and diatomite [18, 19] are very effective filtration aids and have many advantages. They are porous materials and can increase filler porosity and make the filler “fluffiness.” Fluid can flow through them, so that the substrate and enzyme are in full contact with one another. They also have an excellent adsorption effect and play an important role in immobilizing Aspergillus niger cells. Owing to these characteristics, perlite and diatomite were usually used in beverage industry, beer industry, and petroleum industry [20,21,22]. In those industries, the function of perlite and diatomite is filtration. But in this work, coarse perlite was selected as the immobilizing material and it was simply mixed with TIC to achieve the purpose of immobilization.
The main goal of this paper is to investigate the ability of a packed bed reactor (PBR) to continuously convert maltose to IMO. Then, the type and quantity of filtration aid, the optimum operational parameters, the recycling stability, and production capacity of the PBR were studied.
Materials and Methods
Materials
Malt syrup (80%, w/v), coarse perlite 40–80 mesh, thin perlite 80–120 mesh, and diatomite 40–80 mesh were provided by Bailong Chuangyuan Biotechnology Company (Shandong, China). A small-medium-sized pressure chromatography column (Φ1.60 × L20.00 cm, Φ2.63 × L29.00 cm) was obtained from Shanghai Chu Ding Analytical Instrument Company (Shanghai, China). All other reagents used were of analytical grade and purchased from Sigma-Aldrich.
Preparation of the Thermo-Inactivated Cells
Thermo-inactivated cells from A. niger J2 strain were preserved in China Center For Type Culture Collection with a number CCTCC M2014421. α-Glucosidase mainly distributes on the cell membrane and seldom in the extracellular. Cells of A. niger J2 strain were cultivated in bran medium for 60 h and extracted in 30 mL sterile water. Then, the cell suspension was inoculated in 1 L fermentation medium in order to obtain the primary seeds. The bran medium was 2 g bran and 1 mL water putting into a 50-mL conical flask. The fermentation medium was liquefied corn starch 60 g/L, corn syrup powder 30 g/L, pH 4.60.Thereafter, all the primary seeds were inoculated in a 50-L fermenter containing 30 L fermentation medium to cultivate for 36 h under the conditions at 34 °C and 400 rpm. The fermented mash was heated to 55 °C and kept warm for 1.5 h to inactivate the cells. The α-glucosidase was harvested from the TIC using a centrifuge at 12000 rpm. In order to verify whether the cells were thermo-inactivated, the TIC were spread on the plate. No cells grew on the plate.
Type and Quantity of Filtration Aid
During the development of the PBR, one of the most important parameters was the choice of filtration aid. Filtration aids, including coarse perlite, thin perlite, and diatomite, were investigated as the immobilizing material. TIC and different quantity of filtration aids were mixed after adding water, respectively. All mixtures were put into a medium-sized pressure chromatography column (Φ2.63 × L29.00 cm). The maximum pressure of the medium-sized pressure chromatography column was 2.0–2.5 MPa. A maltose solution (30%, w/v) was passed through the medium pressure chromatography column at a flow rate of 1 mL/min. The volume of filtration aid was measured after 5 h.
Preparation of PBR
The bed of Mi-PBR was a small-medium pressure chromatography column. The filler in the packed bed was the mixture of 3.5 g coarse perlite and 3.5 g TIC. Before loading, a layer of 300 mesh filter cloth was put on the lower section of the chromatography column. In order to maintain the temperature (40, 45, 50, 55, and 60 °C), the packed bed and substrate were placed in an incubator. The constant flow pump could not tolerate the high temperature outside of the incubator because the operating temperature of the constant flow pump was 0–40 °C. The fluid of whole PBR was in a silicone tube (Φ1.20 × 3.00 mm) and flowed through the packed bed using a constant flow pump. The operation of the PBR was up in and down out.
In order to transfer the PBR to an industrial scale process, we magnified the PBR, using a water pipe with characteristic pressure resistance to prepare a Me-PBR with a volume of 8.8 L (Fig. 1b). The bed of Me-PBR was a pressure water pipe (Φ15.0 × L50.00 cm). In order to maintain the temperature (50 °C) for conversion, a jacket was covered around the pressure water pipe, and hot water from the thermostat water bath continuously flowed past the jacket to keep it warm. Before loading filtration aid, a layer of filter screen was also put on the lower section of the water pipe. One hundred gram coarse perlite was placed on the filter screen, and the substrate was placed in an incubator. The fluid from the whole PBR in the silicone tube (Φ6.00 × 9.00 mm) flowed through the packed bed by means of a constant flow pump. The operation of the PBR was up in and down out.
a Schematic diagram of Mi-PBR (1, small-medium pressure chromatography column (Φ1.60 × L20.00 cm); 2, malt syrup; 3, constant flow pump; 4, incubator; 5, filtration aid.). b Schematic diagram of Me-PBR (1, pressure water pipe (Φ15.0 × L50.00 cm); 2, filtration aid; 3 and 4, constant flow pump; 5, thermostat water bath; 6, malt syrup; 7, incubator)
Thermal and pH Stability of α-Glucosidase of TIC
The thermal stability of α-glucosidase activities of free and immobilized TIC was conducted at various temperatures from 30 to 65 °C in 5 °C of increment. Free and immobilized TIC were added to a 0.1-mol/L acetate saline buffer (pH 4.50) at different temperatures for 12 h.
The stability of α-glucosidase under different pH values was also studied. Free and immobilized TIC were added to a 0.1-mol/L acetate saline buffer solution, with pH values ranging from 2.00 to 7.00 for 12 h.
Determination of Kinetic Constants
To determine Km and Vmax, the activity assay was applied for different maltose concentrations. Free and immobilized TIC were added to five different concentrations of malt syrup which was dissolved in acetate buffer (0.1 M, pH 4.00), and the system was incubated for 1 h in a water bath at 50 °C. Km and Vmax values were calculated from Lineweaver–Burk plot.
Optimization Conditions for Transglycosylation Reaction Efficiency
Free TIC were added to the malt syrup (pH 4.50) for 4 h to allow transglycosylation reactions to occur with continuous shaking at 100 rpm under different temperatures (from 40 to 60 °C in 5 °C of increment).
Malt syrup (30% w/v, pH 4.50) was passed through the Mi-PBR for transglycosylation reactions to occur at a flow rate of 1 mL/min at different temperatures (45, 50, and 55 °C). Malt syrup was changed every 24 h. The volume of malt syrup was adjusted based on the results of the last batch to guarantee effective IMO (eIMO) ≥ 35% and total IMO (tIMO) ≥ 50% (IMO-50). The eIMO and tIMO were determined using Eq. (1) and Eq. (2):
where m1, glucose; m2, maltose; m3, isomaltose; m4, maltotriose; m5, panose; m6, isomaltotriose; DCW, dry cell weight.
Malt syrup (30% w/v, pH 3.00, 3.50, 4.00, 4.50, 5.00, 6.00, 6.50, 7.00) was passed through the Mi-PBR at a flow rate of 1 mL/min, respectively. The treatment was continued for 48 h. After 48 h, malt syrup continued to pass through the Mi-PBR to allow transglycosylation for 24 h.
Time Course of Transglycosylation Reaction in PBR
Time course of transglycosylation reaction in Mi-PBR was tested to produce IMO-50 under the optimal conditions. Malt syrup (30% w/v, pH 4.00) was changed every 24 h. The volume of malt syrup was adjusted based on the results of the last batch to guarantee eIMO ≥ 35% and tIMO ≥ 50%.
Time course of transglycosylation reaction in Me-PBR was tested for producing IMO-50 under the optimal conditions, using a flow rate of 90 mL/min (instead of 1 mL/min). The mass of malt syrup (30% w/v, pH 4.00) in each batch was 13 kg, and the time of transglycosylation reaction was recorded when the eIMO ≥ 35% and tIMO ≥ 50%.
Analytical Methods
Activity Assays of α-Glucosidase
To characterize the thermal, pH stability, and kinetic parameters of α-glucosidase, TIC (0.1 g) were added to 10 mL malt syrup (30%, w/v) which was dissolved in acetate buffer (0.1 M, pH 4.00) and the system was incubated for 1 h in a water bath at 50 °C. The reaction was stopped by boiling at 100 °C for 10 min [23, 24]. The control group (without α-glucosidase activity) was performed under the same conditions. Non-fermentable reducing sugars equating the amount of IMO were quantified using the yeast-dinitrosalicylic acid (DNS) method [25]. An enzyme activity unit (U) was defined as the amount of enzyme producing 1 μmol non-fermentable reducing sugar per hour under the assay conditions.
Quantitative Estimation of IMO
The yield of IMO was determined by high-performance liquid chromatography (HPLC) on an analytical column (Tsk–gel Amide 80, 5 μm, 250 × 4.6 mm) with a refractive index detector. The mobile phase was composed of acetonitrile and water (v/v, 68:32) at a flow rate of 1 mL/min. The column temperature was maintained at 40 °C. The reaction mixture was adequately diluted with water and then passed through a syringe filter with a pore size of 0.45 μm. Five microliters of sample was injected for analysis.
Statistical Analyses
All the experiments were done in triplicate. The data were analyzed by SPSS version 22.0 for Windows (SPSS Inc., Chicago, IL, USA). Differences were considered significant at p < 0.05 as determined by one-way analysis of variance (ANOVA) using PASW Statistics 18.0 (SPSS Inc., USA).
Results and Discussion
Determination of Type and Quantity of Filtration Aid
Perlite and diatomite are non-metallic raw material [21], and they have many useful advantages such as high porosity, large specific surface area, and high adsorption capacity [26]. The ratio of the volume versus mass of the filler reflects the bulkiness of the filtration aid [20, 27]. As shown in Fig. 2, the ratio of the volume versus mass of 20 g TIC was 2 mL/g without any filtration aid. When 10 g TIC was mixed with 10 g diatomite, 10 g thin perlite, and 10 g coarse perlite, the ratios of volume versus mass were 2.43, 3.2, and 4 mL/g, respectively. The volume of the mixture was increased due to the adding filtration aid combined with TIC, which was bigger than adding only TIC or only filtration aid. Coarse perlite had the greatest effect on “fluffiness” of the filler structure when the ratio of coarse perlite versus TIC was 1:1. Different mass ratios (1.5:1, 2:1) of coarse perlite and TIC were also measured. However, the filler volume was not significantly increased (about 2.44%). Based on these results, coarse perlite was chosen as best filtration aid compared with diatomite and thin perlite in PBR, using the equal quantity of TIC.
Determination of the type and quantity of filtration aid. (V/M ratio, the ratio of the volume and mass of the fille; hypha, pure hypha (water content was 65%); D-1, pure diatomite (20 g); D-2, the ratio of diatomite to TIC was 1:1 (10 g diatomite and 10 g TIC); D-3, the ratio of diatomite to TIC was 2:1 (20 g diatomite and 10 g TIC); P1-1, pure thin perlite (20 g); P1-2, the ratio of thin perlite to TIC was 1:1 (10 g thin perlite and 10 g TIC); P1–3, the ratio of thin perlite to TIC was 2:1 (20 g thin perlite and 10 g TIC); P2-1, pure coarse perlite (20 g); P2-2, the ratio of coarse perlite to TIC was 0.5:1 (5 g coarse perlite and 10 g TIC); P2-3, the ratio of coarse perlite to TIC was 1:1 (10 g coarse perlite and 10 g TIC); P2-4, the ratio of coarse perlite to TIC was 1.5:1 (15 g coarse perlite and 10 g TIC); P2-5, the ratio of coarse perlite to TIC was 2:1 (20 g coarse perlite and 10 g TIC))
Thermal and pH Stability of the α-Glucosidase of TIC
The thermal stability of α-glucosidase activities of free and immobilized TIC was investigated (Fig. 3a). The cells immobilized on coarse perlite were shown to be more stable than free cells at temperature from 30 to 65 °C for 12 h. From 30 to 60 °C, approximately 75 and 82% of its activity were retained for free and immobilized TIC, implying that the α-glucosidase was moderately thermostable.
The pH stability of α-glucosidase activities of free and immobilized TIC was also studied (Fig. 3b). Results showed that the cells immobilized on coarse perlite were shown to be more stable than free cells over the same pH range, and approximately 87 and 91% of α-glucosidase activity were reserved for free and immobilized TIC at pH from 3.00 to 7.00 for 12 h. Compared to other enzyme for producing IMO, such as transglucosidase [3], the α-glucosidase showed the tolerance to a broad pH range. Thus, in this study, α-glucosidase from TIC would be a suitable enzyme for producing IMO in industry, because it holds good properties of pH stability and thermostability.
Kinetic Constants
Kinetic parameters of free and immobilized cells were also determined using Lineweaver–Burk plot. The Km values for free and immobilized cells were 0.11 and 0.16 g mL−1, respectively. The Vmax values for free and immobilized cells were 1.73 and 1.47 mM min−1 g−1, respectively (Fig. 4).
Optimization Conditions for Transglycosylation Reaction Efficiency
Optimal Temperature for Transglycosylation Reaction Efficiency
As shown in Fig. 5a, a higher temperature can promote the transglycosylation efficiency with the temperature from 40 to 60 °C for 4 h, at the same time, the yield of eIMO and tIMO was increased from 25.13 to 28.89% and 55.02 to 66.29%, respectively. In fact, the time of transglycosylation reaction is much longer than our experiment condition in industry. Therefore, the temperature of 60 °C is not a best choice for transglycosylation reaction due to its negative effect on transglycosylation activity of glucosidase (Fig. 3a). Besides, a lower yield of eIMO and tIMO was produced under the temperature of 40 °C (Fig. 5a), although the transglycosylation activity of glucosidase was high. Consequently, a longer time of transglycosylation reaction was conducted under the temperature of 45, 50, and 55 °C in order to meet the requirements of industry (Fig. 5b–d). A transglycosylation product, up to the standard of IMO-50, was considered as qualified in each batch, and if the product was not up to the standard, it was considered that the enzyme had lost 50% activity, reaching the half-life period. Thirty-five batches were carried out at 45 °C, and for the first 11 batches, initial malt syrup volume was 200 mL. After 11 batches, the volume of maltose syrup was adjusted to achieve the highest transglycosylation efficiency. The decrease volume of malt syrup was optimized from 10 to 60 mL in 10 mL of increment. When 50 or 60 mL of malt syrup was decided to decrease, then, eIMO ≥ 35% and tIMO ≥ 50%. But the more volume of malt syrup could be converted when 50 mL of malt syrup was decreased compared to 60 mL. Figure 5b shows from the 32nd batch, eIMO < 35%, and the half-life period was reached and was 32 days at 45 °C for Mi-PBR. Thirty-three batches were carried out at 50 °C. Figure 5c shows from the 25th batch, eIMO < 35%, and the half-life period of Mi-PBR was 25 days at 50 °C. Thirteen batches were carried out at 55 °C, and for the first two batches, initial malt syrup volume was 200 mL. As Fig. 5d shows, from the eighth batch, eIMO < 35%, and the half-life period of Mi-PBR was 8 days at 55 °C.
The half-life period of Mi-PBR was short at 55 °C. There was little difference in the half-life period between 45 and 50 °C. However, the transglycosylation efficiency of α-glucosidase was higher at 50 °C than at 45 °C, and furthermore, the higher temperature was conducive to inhibiting growth of other microorganisms.
Optimal pH for Transglycosylation Reaction Efficiency
The effect of pH on transglycosylation reaction efficiency was conducted ranging from pH 3.00 to pH 7.00 at 50 °C. It showed that optimal pH for transglycosylation reaction efficiency was pH 4.0 (Fig. 6). With the pH from 3.00 to 4.00, the yield of eIMO and tIMO was increased from 26.37 to 38.81% and 53.74 to 65.45%, respectively. With the pH from 4.00 to 7.00, the yield of eIMO and tIMO was decreased from 38.81 to 27.81% and 65.45 to 55.45%, respectively.
Time Course of Transglycosylation Reaction in PBR
Results of time course of transglycosylation reaction in PBR under optimal conditions (pH 4.00, 50 °C) are shown in Fig. 7. Twenty-nine batches (Fig. 7a) were carried out with Mi-PBR, and the initial malt syrup volume was 150 mL. For the first 21 batches, the volume of maltose syrup was kept constant, but eIMO and tIMO of syrup after transglycosylation all met the set requirements. From the 27th batch, eIMO < 35%, and therefore, the half-life period of Mi-PBR was 26 days under optimal conditions. Twenty-nine batches (Fig. 7b) were carried out in Me-PBR continuous production, and the first 28 batches of eIMO and tIMO reached the quality requirements. To convert 13 kg malt syrup into IMO (eIMO ≥ 35%, tIMO ≥ 50%), the time of the transglycosylation reaction was 20 h in the first batch, but the time of subsequent batches was increased. Therefore, the half-life of Me-PBR was approximately 34 days after calculation.
Conclusion
The Mi-PBR utilized a small-medium pressure chromatography column as the bed and a mixture of TIC and filtration aid as the filler. Coarse perlite 40–80 mesh was chosen as filtration aid over diatomite and thin perlite. When coarse perlite and TIC were mixed at a ratio of 1:1, the mass of coarse perlite used was less, but there was still a good “fluffiness” effect. In the optimum process, the best transglycosylation reaction efficiency of Mi-PBR was reached at 50 °C and pH 4.00. Mi-PBR could continuously produce IMO in approximately 25 days and the yield of eIMO and tIMO reached 35 and 50%, respectively. In order to achieve industrial scale production of IMOs using J2 strain, a Me-PBR was constructed, where the volume of the reactor was approximately 8.8 L. Me-PBR could continuously produce IMO in approximately 34 days. In summary, this study provided an attractive way for production of IMOs by PBR.
References
Bharti, S. K., Krishnan, S., Kumar, A., Gupta, A. K., Ghosh, A. K., & Kumar, A. (2015). Mechanism-based antidiabetic activity of fructo- and isomalto-oligosaccharides: validation by in vivo, in silico and in vitro interaction potential. Process Biochemistry, 50(2), 317–327. https://doi.org/10.1016/j.procbio.2014.10.014
Lee, B. H., Koh, D. W., Territo, P. R., Park, C. S., Hamaker, B. R., & Yoo, S. H. (2015). Enzymatic synthesis of 2-deoxyglucose-containing maltooligosaccharides for tracing the location of glucose absorption from starch digestion. Carbohydrate Polymers, 132, 41–49. https://doi.org/10.1016/j.carbpol.2015.06.012
Mendis, M., Mendoza, B. R., & Simsek, S. (2012). Covalent immobilization of transglucosidase onto polymer beads for production of isomaltooligosaccharides. Catalysis Letters, 142(9), 1107–1113. https://doi.org/10.1007/s10562-012-0866-5
Cho, S. K., Shin, S. Y., Lee, S. J., Li, L., Moon, J. S., Kim, D. J., Im, W. T., & Han, N. S. (2015). Simple synthesis of isomaltooligosaccharides during Sauerkraut fermentation by addition of Leuconostoc starter and sugars. Food Science and Biotechnology, 24(4), 1443–1446. https://doi.org/10.1007/s10068-015-0185-x
Kaneko, T., Yokoyama, A., & Suzuki, M. (2014). Digestibility characteristics of isomaltooligosaccharides in comparison with several saccharides using the rat jejunum loop method. Bioscience, Biotechnology, and Biochemistry, 59, 1190–1194.
Madsen, L. R., Stanley, S., Swann, P., & Oswald, J. (2017). A survey of commercially available isomaltooligosaccharide-based food ingredients. Journal of Food Science, 82(2), 401–408. https://doi.org/10.1111/1750-3841.13623
Sorndech, W., Sagnelli, D., Blennow, A., & Tongta, S. (2017). Combination of amylase and transferase catalysis to improve IMO compositions and productivity. LWT - Food Science and Technology, 79, 479–486.
Rudeekulthamrong, P., Sawasdee, K., & Kaulpiboon, J. (2013). Production of long-chain isomaltooligosaccharides from maltotriose using the thermostable amylomaltase and transglucosidase enzymes. Biotechnology and Bioprocess Engineering, 18(4), 778–786. https://doi.org/10.1007/s12257-012-0777-8
Kuriki, T., Yanase, M., Takata, H., Takesada, Y., Imanaka, T., & Okada, S. (1993). A new way of producing iomalto-oligosaccharide syrup by using the transglycosylation reaction of neopullulanase. Applied and Environmental Microbiology, 59(4), 953–959.
Kawamoto, H., Oguma, T., Sekine, H., & Kobayashi, M. (2001). Immobilization of cycloisomaltooligosaccharide glucanotransferase for the production of cycloisomaltooligosaccharides from dextran. Enzyme and Microbial Technology, 28(6), 515–521. https://doi.org/10.1016/S0141-0229(01)00304-0
Zhang, L., Jiang, Y. J., Jiang, Z. Y., Sun, X. H., Shi, J. F., Cheng, W., & Sun, Q. Y. (2009). Immobilized transglucosidase in biomimetic polymer–inorganic hybrid capsules for efficient conversion of maltose to isomaltooligosaccharides. Biochemical Engineering Journal, 46(2), 186–192. https://doi.org/10.1016/j.bej.2009.05.008
Aslan, Y., & Tanriseven, A. (2007). Immobilization of Penicillium lilacinum dextranase to produce isomaltooligosaccharides from dextran. Biochemical Engineering Journal, 34(1), 8–12. https://doi.org/10.1016/j.bej.2006.11.008
Bertrand, E., Pierre, G., Delattre, C., Gardarin, C., Bridiau, N., Maugard, T., Strancar, A., & Michaud, P. (2014). Dextranase immobilization on epoxy CIM® disk for the production of isomaltooligosaccharides from dextran. Carbohydrate Polymers, 111, 707–713. https://doi.org/10.1016/j.carbpol.2014.04.100
Goulas, A. K., Fisher, D. A., Grimble, G. K., Grandison, A. S., & Rastall, R. A. (2004). Synthesis of isomaltooligosaccharides and oligodextrans by the combined use of dextransucrase and dextranase. Enzyme and Microbial Technology, 35(4), 327–338. https://doi.org/10.1016/j.enzmictec.2004.05.008
Chalane, S., Delattre, C., Michaud, P., Lebert, A., Gardarin, C., Kothari, D., Creuly, C., Goyal, A., Štrancar, A., & Pierre, G. (2017). Optimized endodextranase-epoxy CIM® disk reactor for the continuous production of molecular weight-controlled prebiotic isomaltooligosaccharides. Process Biochemistry, 58, 105–113. https://doi.org/10.1016/j.procbio.2017.04.017
Zhang, L., Su, Y. L., Zheng, Y., Jiang, Z. Y., Shi, J. F., Zhu, Y. Y., & Jiang, Y. J. (2010). Sandwich-structured enzyme membrane reactor for efficient conversion of maltose into isomaltooligosaccharides. Bioresource Technology, 101(23), 9144–9149. https://doi.org/10.1016/j.biortech.2010.07.001
Ojha, S., Mishra, S., & Chand, S. (2015). Production of isomalto-oligosaccharides by cell bound α-glucosidase of Microbacterium sp. LWT - Food Science and Technology, 60(1), 486–494. https://doi.org/10.1016/j.lwt.2014.08.009
Maxime, D., & Lameloise, M. L. (2003). Filterability and filtration experiences of viscous complex food salting solutions. Chemical Engineering Research and Design, 81(9), 1150–1157. https://doi.org/10.1205/026387603770866317
Laoot, P., Rangsriwatananon, K., & Chaisena, A. (2011). Synthesis of sodium-type fluorophlogopite mica from perlite and diatomite. Journal of Ceramic Processing Research, 12, 273–278.
Youn, K. S., Hong, J. H., Bae, D. H., Kim, S. J., & Kim, S. D. (2004). Effective clarifying process of reconstituted apple juice using membrane filtration with filter-aid pretreatment. Journal of Membrane Science, 228(2), 179–186. https://doi.org/10.1016/j.memsci.2003.10.006
Martinovic, S., Vlahovic, M., Boljanac, T., & Pavlovic, L. (2006). Preparation of filter aids based on diatomites. International Journal of Mineral Processing, 80(2-4), 255–260. https://doi.org/10.1016/j.minpro.2006.05.006
Yates, R. A., & Caldwell, J. D. (1993). Regeneration of oils used for deep frying: a comparison of active filter aids. Journal of the American Oil Chemists' Society, 70(5), 507–511. https://doi.org/10.1007/BF02542585
Basu, A., Mutturi, S., & Prapulla, S. G. (2016). Production of isomaltooligosaccharides (IMO) using simultaneous saccharification and transglucosylation from starch and sustainable sources. Process Biochemistry, 51(10), 1464–1471. https://doi.org/10.1016/j.procbio.2016.06.008
Wang, J., Li, W., Niu, D. D., Singh, S., Lu, F. P., & Liu, X. G. (2017). Improved synthesis of isomaltooligosaccharides using immobilized α-glucosidase in organic–aqueous media. Food Science and Biotechnology, 26(3), 731–738. https://doi.org/10.1007/s10068-017-0092-4
Chen, G. G., Li, W., Zhang, Y. K., Qin, Y. L., Wu, K. Y., & Liang, Z. Q. (2011). A high-throughput method for screening of Aspergillus niger mutants with high transglycosylation activity by detecting non-fermentable reducing sugar. World Journal of Microbiology and Biotechnology, 27(6), 1519–1523. https://doi.org/10.1007/s11274-010-0595-0
Ediz, N., Bentli, İ., & Tatar, İ. (2010). Improvement in filtration characteristics of diatomite by calcination. International Journal of Mineral Processing, 94(3-4), 129–134. https://doi.org/10.1016/j.minpro.2010.02.004
Smythe, M. C., & Wakeman, R. J. (2000). The use of acoustic fields as a filtration and dewatering aid. Ultrasonics, 38(1-8), 657–661. https://doi.org/10.1016/S0041-624X(99)00147-X
Funding
This work was financially supported by the Natural Science Foundation of Guangxi Province (2016GXNSFAA380130) and the National Natural Science Foundation of China (31560448).
Author information
Authors and Affiliations
Corresponding authors
Ethics declarations
Conflict of Interest
The authors declare that they have no conflict of interest.
Rights and permissions
About this article
Cite this article
Huang, Z., Li, Z., Su, Y. et al. Continuous Production of Isomalto-oligosaccharides by Thermo-inactivated Cells of Aspergillus niger J2 with Coarse Perlite as an Immobilizing Material. Appl Biochem Biotechnol 185, 1088–1099 (2018). https://doi.org/10.1007/s12010-018-2706-6
Received:
Accepted:
Published:
Issue Date:
DOI: https://doi.org/10.1007/s12010-018-2706-6