Abstract
Nanoparticles (NPs) have been widely used in agriculture, and lanthanum oxide nanoparticles (La2O3) NPs can regulate plant growth. La2O3 NPs treatment was hypothesized to affect the accumulation and distribution of substances in rice seedlings under wet and dry nursery conditions. The objective of the present study was to ascertain the effects of La2O3 NPs foliar spray on the morphology and physiology of fragrant rice seedlings under wet and dry nursery conditions. Seedlings of two fragrant rice cultivars, namely ‘Xiangyaxiangzhan’ and ‘Yuxiangyouzhan,’ were grown under wet and dry nursery conditions with La2O3 NPs treatments at three concentrations (CK, La2O3 NPs 0 mg L−1; T1, La2O3 NPs 20 mg L−1; and T2, La2O3 NPs 40 mg L−1). The results showed that the seedling-raising method was significantly associated with La2O3 NPs application (P < 0.05), affecting the leaf area of both cultivars. Changes in plant morphological parameters, such as dry weight and root–shoot ratio, were the reasons for the differences in cultivars in response to La2O3 NPs application. Changes were also observed in the plant morphological and physiological parameters of leaf area, specific leaf area, chlorophyll contents, antioxidant properties, and activities of nitrogen metabolism enzymes. The relationship between morphological and physiological processes in fragrant rice was investigated to test the hypothesis. In both wet and dry nursery methods, the T2 concentration of La2O3 NPs was beneficial for rice seedlings and significantly increased their leaf area due to changes in morphological and physiological parameters. Therefore, the results of this study provide a theoretical basis for expanding the research on La2O3 NPs application in rice, as well as relevant references for strengthening rice seedlings in the nursery, which has a positive effect on the grain yield improvement in fragrant rice.
Similar content being viewed by others
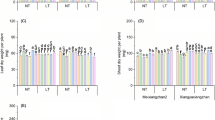
Explore related subjects
Discover the latest articles, news and stories from top researchers in related subjects.Avoid common mistakes on your manuscript.
Introduction
Rice, a globally important nutritious crop, heavily relies on proper seedling development for its production (Qing et al. 2022). China, where fragrant rice is prized for its delectable flavor, produces approximately 30% of rice in the world (Deng et al. 2022). Mechanical transplanting is popular in rice production because it is a simplified cultivation technique with low labor costs (Dou et al. 2021). In mechanical transplanting, different seedling-raising methods greatly influence seedling growth and rice yield (Cheng et al. 2018).
Nano-fertilizer application is a brand-new approach to crop management. Nanoparticles (NPs) are frequently used in agriculture to increase crop growth and productivity (Li et al. 2021). Valojai et al. (2021) demonstrated that nano-fertilizer application improved the yield of milled rice grains. Ahanger et al. (2021) also showed that using nano-vermicompost as organic fertilizer promoted plant growth. Therefore, NPs application during seedling raising can be used to improve rice growth and production.
Technical requirements for rice seedling establishment are crucial when mechanically transplanting rice seedlings (Dou et al. 2021). The establishment of strong and uniform rice seedlings is a prerequisite and key for the consistent development of field transplants to achieve a high rice yield (Li et al. 2020). The water level is undeniably essential to rice seedling quality (Lampayan et al. 2015). High-quality rice mechanical transplanting in paddy fields depends on the high quality of rice seedlings raised using an optimized seedling-raising method (Zhang and Gong 2014). The dry nursery (DN) method had higher seedling establishment rates than the wet nursery (WN) method (Cheng et al. 2018). As both are common rice-raising methods, comparative studies on fragrant rice seedlings grown using these nursery methods may draw more attention to the mechanized production of fragrant rice.
NPs show promise in agriculture, especially in the optimal use of chemical fertilizers and pesticides. Typically, at least one of their dimensions is smaller than 100 nm (Chen et al. 2021). The use of NPs aids in our understanding of plant mechanisms against abiotic stress (Mohapatra et al. 2023). Rare earth oxide (REO) NPs are emerging materials with unique properties (Xu and Qu 2014), which have led to their increasing use in optics, agronomic screening, thermoelectric materials, and so forth (Kumar and Seth 2021). Lanthanum oxide (La2O3) NPs are REO NPs famed for their strong interaction, significant specific surface area, and quick absorption (Yue et al. 2017). Because of extensive use, La2O3 NPs are inevitably released into agricultural soils (Garner et al. 2017). This may result in environmental damage and a serious threat to the health of living organisms (Liu et al. 2020a). Researchers in the scientific community are divided on whether NPs are beneficial or detrimental to crops. For example, Liu et al. (2020a) demonstrated a decrease in the biomass of maize and soybean exposed to 50 mg L−1 La2O3 NPs. In contrast, Zhang et al. (2022) showed that Mo3 NPs promoted plant growth and N3− utilization in rice. Xiao et al. (2022) found that La2O3 NPs exposure significantly increased leaf sucrose content and plant biomass in radish. A recent study confirmed that La2O3 NPs affected plant growth and physiological metabolism (Liu et al. 2020a). La2O3 NPs may change plant growth and maturation. However, the effects of La2O3 NPs application on fragrant rice seedlings cultivated using WN and DN methods remain unclear.
Different nursery methods are critical to the quality of rice seedlings, and the application of La2O3 NPs used as nano-fertilizer promotes the growth of fragrant rice seedlings. However, the effects of both seedling raising methods and La2O3 NPs concentration applied to rice seedlings are not known, so the aim of this study was to assess the effects of La2O3 NPs foliar spray on the morphological and physiological characteristics of fragrant rice seedlings using WN and DN methods. La2O3 NPs treatment was hypothesized to affect the accumulation and distribution of organic substances in rice seedlings in both nursery methods. As there could be plant physiological changes, this study also evaluated the biomass, growth, antioxidant properties, and nitrogen metabolism of fragrant rice seedlings under La2O3 NPs application using the two seedling-raising methods. Furthermore, the relationship between morphological and physiological parameters of fragrant rice was investigated to test the hypothesis.
Materials and methods
Experimental description
The experiments were performed in September 2021 at the South China Agricultural University (SCAU), Guangzhou, Guangdong Province, China. Fragrant rice cultivars ‘Xiangyaxiangzhan’ and ‘Yuxiangyouzhan,’ both of which are popular in South China, were used in this study. ‘Xiangyaxiangzhan’ is a conventional rice variety, whereas ‘Yuxiangyouzhan’ is a super rice variety with great potential for high productivity and wide adaptability (Liu et al. 2020b). Seeds of both cultivars were obtained from the College of Agriculture, SCAU. La2O3 NPs that were 99.99% pure and of 50 nm particle size were obtained from Macklin Biochemical Co., Ltd., Shanghai, China.
Experimental treatments
The experimental design was completely randomized. Approximately 500 g seeds of each cultivar was used. Under room temperature, the seeds were disinfected with 75% alcohol for 10 min, followed by three washes with sterile water, and then soaked in distilled water for 24 h. Two approaches were used to sow and raise seeds, i.e., WN and DN (Cheng et al. 2018). Until the three-leaf stage, rice seedlings were treated with three La2O3 NPs concentrations of 0, 20, and 40 mg L−1. La2O3 NPs solutions were agitated for 5 min before being subjected to ultrasonic treatment (HZS-H, China) (25 °C, 800 W, 40 kHz) for 30 min to facilitate their dispersion (Li et al. 2021). Each treatment solution was prepared just before use. There were 12 treatments, with each treatment repeated four times. During the experiment, the average temperature was 24.9 °C, the average humidity was 71.8%, the average sunshine hours were 173.9 h, and the average light intensity was 263.7 µmolm-2 s-1.
Measurement indicators and methods
Determination of biomass and morphological traits
Three days after treatment, 40 randomly selected rice seedlings were collected from each treatment, i.e., four replicates for each treatment and ten rice seedlings for each replicate. Leaf sheath length, plant height, and leaf area of these seedlings were determined with a digimatic caliper. An electronic balance was used to measure the fresh and dry biomass. Dry biomass was obtained by drying the fresh biomass in an oven at 60 °C for 3 days until constant weight. Formulae based on Awan et al. (2014) were used to determine the shoot dry weight to plant height ratio, root–shoot ratio, specific leaf area, and dry weight ratio of distinct plant tissues.
Determination of chlorophyll contents
Fresh leaf samples (0.1 g) were extracted with 7.5 mL of 95% ethanol and placed in a dark room to determine their chlorophyll levels. After 24 h, samples were centrifuged at 5000 rpm for 5 min. Supernatant chlorophyll and carotenoid contents were evaluated by sequentially measuring the absorbance at 665, 649, and 470 nm (Li et al. 2021).
Determination of physiological characteristics
The plant samples were collected 3 days after treatment, divided into stems, leaves, and roots, flash-frozen in liquid nitrogen for 5 min, and stored at − 80 °C for biochemical analysis.
Determination of antioxidant enzyme activities and malondialdehyde (MDA) content
Superoxide dismutase (SOD), peroxidase (POD), and catalase (CAT) activities, as well as MDA content, were measured as previously reported (Li et al. 2021). Spectrophotometer UV-2550 (Shimadzu Corporation, Kyoto, Japan) was used to measure the absorbance. Fresh samples were homogenized (0.15 g) with 1.5 mL of 0.05 mol L−1 phosphate-buffered saline (PBS) solution in an ice bath and then centrifuged at 14,000 rpm for 15 min at 4 °C, and the supernatant was used to determine the parameters. Each treatment was repeated three times for physiological measurements.
SOD activity was measured as follows. Exactly 5 μL of the crude enzyme solution was added to a reaction system, which consisted of 150 μL of 50 mM sodium phosphate buffer, 30 μL of 130 mM methionine, 30 μL of 750 μM nitrotetrazolium blue chloride (NBT), and 30 μL of 100 μM ethylenediaminetetraacetic acid disodium salt. After mixing them, 30 μL of 20 μM riboflavin was added, the light was set to 4000 lx, and the mixture was allowed to react for 20 min. The amount of enzyme required to inhibit 50% of the NBT photoreduction reaction was taken as one enzyme activity unit (U). To detect absorbance at 560 nm, SOD activity was measured in units of U gFW−1.
POD activity was measured as follows. Exactly 100 μL of PBS (pH 7.0) and 95 μL of 0.2% guaiacol were mixed, 5 μL of crude enzyme solution and 100 μL of 0.3% hydrogen peroxide solution were added. To detect absorbance at 470 nm, the data were recorded every 30 s for 2.5 min. The absorbance increase of one unit per minute (U gFW−1) was chosen as the standard unit of POD activity.
CAT activity was measured as follows. First, 1.95 mL of ultrapure water and 1 mL of 0.3% hydrogen peroxide solution were added to the reaction system. Then, 0.05 mL of the crude enzyme solution was added, and the absorbance of the reaction system was measured at 240 nm for 2 min every 30 s. The walls of the quartz cuvette prevent light absorption in the ultraviolet region. CAT activity was calculated as U gFW−1, and one CAT activity unit (U) was the quantity of enzyme that catalyzed the reaction to cause a decrease in 0.01 absorbance per minute.
MDA content was determined by reacting 0.4 mL of 0.5% thiobarbituric acid solution and 0.2 mL of crude enzyme solution in a boiling water bath for 30 min and measuring the absorbance of the supernatant at 532, 600, and 450 nm. It was expressed as µmol gFW−1.
Determination of nitrogen metabolism enzyme activities
The method developed by Liao et al. (2022) was used to measure nitrate reductase (NR) activity in rice seedlings. Fresh samples (0.1 g) were homogenized in 2 mL of ice-cold PBS before being centrifuged at 4000 rpm and 4 °C for 15 min. The original enzyme extract was collected in the supernatant. Absorbance reduction was used to define one NR activity unit (U), which was then quantified as U gFW−1.
Glutamine synthetase (GS) and glutamate synthase (GOGAT) activities were calculated using the methods of Qing et al. (2022). Extraction buffer was used to homogenize fresh samples (0.2 g), and the samples were then centrifuged at 13,000 rpm for 25 min at 4 °C. GS and GOGAT activities were measured using the supernatants. The absorbance for GS was measured at 540 nm using the spectrophotometer. GS activity was calculated as U gFW−1, and one GS activity unit (U) was the amount of the enzyme required to catalyze the formation of 1 μmol of γ-glutamyl isohydroxamic acid in 15 min of reaction time. Total activity was the number of μmol of γ-glutamyl isohydroxamic acid catalyzed per gram of fresh crude enzyme in 15 min of reaction time. To determine GOGAT activity, the absorbance at 340 nm was measured every 30 s for 11 consecutive times. U gFW−1 is the unit of measure for the absorbance reduction in one micromole of NADH per min.
Statistical analyses
Statistix v8 (Analytical Software, Tallahassee, FL, USA) was used for data analysis, analysis of variance (ANOVA), and correlation analysis, and the least significant difference (LSD) test was used to compare sample means at the 0.05 level of significance.
Correlations between variables were analyzed using the Pearson correlation coefficient. Correlations with an absolute value of less than 0.3 were considered weak, those between 0.3 and 0.7 were considered moderate, and those greater than 0.7 were considered strong. Significance was set at P < 0.05.
Results
Biomass
Method (M) and M × La2O3 NPs (La) significantly (P < 0.05) impacted dry weight. Statistically significant differences (P < 0.05) were observed in the dry weight of stem, leaf, shoot, and total plant after La2O3 NPs application. Under T1 treatment, DN increased the root dry weight of ‘Xiangyaxiangzhan’ and ‘Yuxiangyouzhan’ by 18.65% and 31.88%, respectively, compared with WN. Under T2 treatment, WN increased the stem dry weight of ‘Xiangyaxiangzhan’ and ‘Yuxiangyouzhan’ by 24.58% and 20.77%, respectively, compared with DN. Moreover, T1 and T2 treatments decreased the shoot dry weight of ‘Yuxiangyouzhan’ by 11.49% and 7.99%, respectively, compared with CK (Table 1). Statistically significant differences (P < 0.05) were observed in the fresh weight of stem, leaf, shoot, and total plant for M and La. WN increased the root fresh weight of ‘Xiangyaxiangzhan’ by 20.47% under T2 treatment, compared with DN. It also increased the stem fresh weight of ‘Yuxiangyouzhan’ by 34.88%, 64.28%, and 55.15% under CK, T1, and T2 treatments, respectively, compared with DN. Nonetheless, on average, compared with CK, T1 treatment decreased the leaf fresh weight of ‘Yuxiangyouzhan’ by 7.96% (Table 2).
Distribution of growth parameters
Under T1 treatment, DN increased the root weight ratio of ‘Yuxiangyouzhan’ by 36.50%, compared with WN. Under T2 treatment, WN increased the leaf weight ratio of ‘Xiangyaxiangzhan’ by 13.25%, compared with DN (Table 3). Cultivar (C) and M × La significantly affected the shoot dry weight to plant height ratio. Compared with ‘Xiangyaxiangzhan,’ the shoot dry weight to plant height ratio of ‘Yuxiangyouzhan’ under CK-WN, CK-DN, T1-WN, and T2-WN treatments increased by 26.09%, 18.82%, 24.54%, and 19.43%, respectively. Under T1 treatment, DN increased the root–shoot ratio of ‘Yuxiangyouzhan’ by 44.35%, compared with WN (Table 4).
Morphological traits
M significantly affected plant height. WN increased the plant height of ‘Xiangyaxiangzhan’ by 25.14%, 29.15%, and 22.85% under CK, T1, and T2 treatments, respectively, compared with DN. Compared with ‘Yuxiangyouzhan,’ the leaf sheath length of ‘Xiangyaxiangzhan’ under CK-WN treatment increased by 27.35%. C, M, La, and C × M significantly affected the leaf area. T2 treatment increased the mean value of the leaf area of ‘Xiangyaxiangzhan’ in the two seedling nursery methods by 16.66%, compared with CK. In contrast, the T1 treatment reduced the mean value of the leaf area of ‘Yuxiangyouzhan’ in WN and DN by 15.44%, compared with CK. Moreover, WN increased the leaf area of ‘Yuxiangyouzhan’ by 52.69%, 27.90%, and 49.80% under CK, T1, and T2 treatments, respectively, compared with DN. C, C × M, and M × La significantly affected the specific leaf area. DN increased the specific leaf area of ‘Xiangyaxiangzhan’ by 24.14% and 42.87% under CK and T2 treatments, compared with WN. While DN increased the specific leaf area of ‘Yuxiangyouzhan’ by 4.52% under T2 treatment, compared with WN (Table 5).
Chlorophyll contents
La2O3 NPs treatment significantly affected leaf chlorophyll a (Chl a), chlorophyll b (Chl b), carotenoid, and total chlorophyll (Chl) contents, and the chlorophyll a/b (Chl a/b) ratio, C × La, and M × La. T2 treatment increased the mean value of Chl a, Chl b, carotenoid, and total Chl contents of ‘Xiangyaxiangzhan’ in the two seedling nursery methods by 20.61%, 11.39%, 46.12%, and 17.47%, respectively, compared with CK (Fig. 1). On average, T1 treatment increased the Chl a, Chl b, carotenoid, and total chlorophyll contents of ‘Yuxiangyouzhan’ by 26.39%, 17.42%, 39.57%, and 23.25%, respectively, compared with CK (Fig. 1). Compared with ‘Xiangyaxiangzhan,’ the carotenoid contents of ‘Yuxiangyouzhan’ under CK-WN, T1-WN, and T1-DN treatments increased by 40.15%, 88.49%, and 18.89%, respectively (Fig. 1d). For ‘Xiangyaxiangzhan,’ compared with CK-WN treatment, the Chl a, Chl b, carotenoid, and total Chl contents, and the Chl a/b ratio showed no significant change under CK-DN treatment but increased under T1- and T2-DN treatments (Fig. 1).
The chlorophyll a content (a), the chlorophyll b content (b), the chlorophyll a/b ratio (c), the carotenoid content (d), and the total chlorophyll content (e) of the two fragrant rice cultivars in wet and dry nurseries with foliar spray of La2O3 nanoparticles. C, cultivar; M, method; La, La2O3 NPs. Lowercase letters represent significant differences between treatments (LSD test, P < 0.05)
Antioxidant enzyme activities and MDA content
C, M, and La all had a considerable impact on SOD activity. C × M, C × La, and M × La had an impact as well. Compared with CK, the mean value of SOD activity in leaves of ‘Xiangyaxiangzhan’ substantially improved by 15.28% under T2 treatment (Fig. 2a). Much improvement was observed in the mean value of SOD activity in leaves of ‘Yuxiangyouzhan,’ by 39.93% and 36.69% under T1 and T2 treatments, respectively (Fig. 2a). T2 treatment marginally increased the mean value of SOD activity in roots of ‘Yuxiangyouzhan’ by 50.52% in the two seedling nursery methods (Fig. 2b). Compared with CK, T2 treatment in DN and WN led to a dramatic increase in POD activity in roots of ‘Xiangyaxiangzhan’ by 78.11% and 26.73%, respectively, and a wild surge in POD activity in roots of ‘Yuxiangyouzhan’ by 45.50% and 22.04%, respectively (Fig. 2d). CAT activity was significantly affected by M, La, C × M, and M × La. Compared with CK, T2 treatment led to a significant increase in the mean value of CAT activity in leaves of ‘Xiangyaxiangzhan’ by 15.53% in the two seedling nursery methods (Fig. 2e). T1 and T2 treatments significantly enhanced the mean value of CAT activity in leaves of ‘Yuxiangyouzhan’ by 47.43% and 75.10%, respectively (Fig. 2e). However, CAT activity in roots of ‘Yuxiangyouzhan’ was decreased by 28.42% in T1 treatment, compared with CK (Fig. 2f). On average, T1 and T2 treatments led to a significant decrease in MDA content in leaves of ‘Xiangyaxiangzhan by 46.51% and 49.56%, respectively, compared with CK (Fig. 2g). MDA content was adversely impacted by M, La, C × M, C × La, and M × La. MDA content in leaves of ‘Yuxiangyouzhan’ in DN was reduced by 35.77%, 49.66%, and 36.98% under CK, T1, and T2 treatments, respectively, compared with WN (Fig. 2h). Moreover, MDA content in roots of ‘Yuxiangyouzhan’ in DN was reduced by 64.19% and 66.67% under CK and T1 treatments, respectively, compared with WN (Fig. 2h).
Superoxide dismutase (SOD) activity in leaves (a) and roots (b), peroxidase (POD) activity in leaves (c) and roots (d), catalase (CAT) activity in leaves (e) and roots (f), malondialdehyde (MDA) content in leaves (g) and roots (h) of the two fragrant rice cultivars in wet and dry nurseries with foliar spray of La2O3 nanoparticles. C, cultivar; M, method; La, La2O3 NPs. Lowercase letters represent significant differences between treatments (LSD test, P < 0.05)
Nitrogen metabolism enzyme activities
On average, T1 and T2 treatments increased GS activity in leaves of ‘Xiangyaxiangzhan’ by 8.03% and 11.68%, respectively, and ‘Yuxiangyouzhan’ by 5.00% and 8.57%, respectively, compared with CK (Fig. 3a). T2 treatment marginally improved the mean value of GS activity in roots in the two seedling nursery methods for ‘Yuxiangyouzhan,’ whereas no discernible changes were found for ‘Xiangyaxiangzhan’ after La application (Fig. 3b). T1 treatment decreased the mean value of NR activity in leaves of ‘Xiangyaxiangzhan’ and ‘Yuxiangyouzhan’ by 19.14% and 15.16%, respectively, compared with CK (Fig. 3c). T2 treatment decreased the mean value of NR activity in leaves of ‘Xiangyaxiangzhan’ and ‘Yuxiangyouzhan’ by 10.54% and 19.16%, respectively, compared with CK (Fig. 3c). T1 and T2 treatments increased the mean value of GOGAT activity in leaves of ‘Xiangyaxiangzhan’ by 20.03% and 18.35%, respectively, compared with CK (Fig. 3e). T1 and T2 treatments increased the mean value of GOGAT activity in leaves of ‘Yuxiangyouzhan’ by 24.36% and 36.79%, respectively, compared with CK (Fig. 3e).
Glutamine synthetase (GS) activity in leaves (a) and roots (b), nitrate reductase (NR) activity in leaves (c) and roots (d), and glutamate synthase (GOGAT) activity in leaves (e) and roots (f) of the two fragrant rice cultivars in wet and dry nurseries with foliar spray of La2O3 nanoparticles. C, cultivar; M, method; La, La2O3 NPs. Lowercase letters represent significant differences between treatments (LSD test, P < 0.05). *P ≤ 0.05
Correlation analysis
The root–shoot ratio was significantly negatively correlated with the fresh weight of leaf and shoot; the dry weight of stem, leaf, shoot, and total plant; and GOGAT activity in roots. Moreover, the root–shoot ratio revealed negative and obvious correlations with root dry weight, root dry weight ratio, and CAT activity in leaves. Leaf fresh weight was significantly positively correlated with leaf area, stem fresh weight, shoot fresh weight, total fresh weight, stem dry weight, leaf dry weight, shoot dry weight, total dry weight, plant height, leaf weight ratio, POD activity in roots, and GOGAT activity in roots. POD activity in roots was significantly positively correlated with the fresh weight of root, leaf, shoot, and total plant; the dry weight of leaf, shoot, and total plant; the leaf weight ratio; and the GOGAT activity in leaves (Fig. 4).
Correlation analysis between the investigated parameters. V01, root–shoot ratio; V02, leaf sheath length; V03, leaf area; V04, specific leaf area; V05, root fresh weight; V06, stem fresh weight; V07, leaf fresh weight; V08, shoot fresh weight; V09, total fresh weight; V10, root dry weight; V11, stem dry weight; V12, leaf dry weight; V13, shoot dry weight; V14, total dry weight; V15, plant height; V16, shoot dry weight/plant height; V17, root weight ratio; V18, stem weight ratio; V19, leaf weight ratio; V20, the Chl a content; V21, the Chl b content; V22, carotenoid content; V23, total Chl content; V24, the Chl a/b ratio; V25, SOD activity in leaves; V26, SOD activity in roots; V27, POD activity in leaves; V28, POD activity in roots; V29, CAT activity in leaves; V30, CAT activity in roots; V31, MDA content in leaves; V32, MDA content in roots; V33, GS activity in leaves; V34, GS activity in roots; V35, NR activity in leaves; V36, NR activity in roots; V37, GOGAT activity in leaves; V38, GOGAT activity in roots
Discussion
WN has been widely promoted since the 1960s, while DN, a water-saving seedling-raising method, has been recently adopted in many places. These seedling-raising methods with their own characteristics are the two main ways in China (Lampayan et al. 2015). Water is a key factor among various seedling-raising techniques, which strongly impacts the morphological traits of both rice cultivars. Coleoptile elongation and seedling emergence were brought about by hypoxic conditions in flooded soil (Cheng et al. 2018). An earlier study also indicated that dry soil led to a reduced number of tillers (Tao et al. 2014). The results were consistent with previous research. Plant height was significantly higher and root dry weight was significantly lower in WN-raised rice seedlings than in DN-raised rice seedlings (Tables 1 and 5). Overall, WN-raised rice seedlings were morphologically better than DN-raised rice seedlings. WN-raised rice seedlings had improved fresh weight, dry weight, plant height, leaf sheath length, and leaf area (Tables 1, 2, and 5).
Lanthanum (La) has been used as a plant growth regulator in agricultural production for nearly 50 years (Dai et al. 2017). La application at certain concentrations can reduce growth inhibition and promote growth in wheat and rice plants (Yang et al. 2021; Luo et al. 2021). NPs application plays an important role in food security and green agricultural development (Kumar and Seth 2021). However, nano-agriculture in China is still in the nascent stage (Li et al. 2021). NPs concentrations as low as 50 mg L−1 can stunt plant growth (Yue et al. 2017), whereas concentrations of more than 50 mg L−1 have a more pronounced effect on physiological and biochemical processes in plants (Li et al. 2018; Nhan et al. 2015). Other studies indicated that plant growth may benefit from exposure to NPs at levels as low as 0–50 mg L−1 (Rastogi et al. 2017). Thus, exposure dosages of 0, 20, and 40 mg L−1 were chosen. Studies on La2O3 NPs application have been increasing, and a recent study found that La2O3 NPs treatment affected root development, aerial part growth, and pigment concentration in Pfaffia glomerata plantlets (Neves et al. 2022). Thus, we assessed La2O3 NPs application in regulating growth by using different rice seedling-raising methods. Xiao et al. (2021) showed that the storage root biomass of radish decreased by 38% and 60% on exposure to 100 and 300 mg L−1 La2O3 NPs, respectively. However, the present study found that La2O3 NPs treatment significantly reduced shoot fresh weight but increased root dry weight of WN- and DN-raised rice seedlings (Tables 1 and 2). The difference may be because the La2O3 NPs concentrations used in our study were lower than those used in previous studies; thus, it was demonstrated that low concentrations of La2O3 NPs treatment can promote the root growth of plants.
The effects of drought pressure markedly reduced leaf area (Timmusk et al. 2014). Karimi et al. (2018) also established that the loss of leaves or the development of smaller leaves is one of the ecological responses to drought. Similarly, our study illustrated that the leaf area was smaller in DN-raised rice seedlings than in WN-raised rice seedlings (Table 5). Moreover, La2O3 NPs application under T2 treatment improved the leaf area and specific leaf area compared with plants under T1 and CK treatments for ‘Xiangyaxiangzhan’ (Table 5). Specifically, the increase in the leaf area led to an increase in the photosynthetic area, thereby La2O3 NPs under DN treatment significantly improved plant growth parameters. Positive effects of other NPs on leaf area have been reported in plants under drought stress. In feverfew plants, increased biomass accumulation after treatment with nano-silicon complexes has been linked to enhanced nutrient uptake as a result of improved root growth, and may also be associated with enhanced photosynthetic efficiency under adverse conditions (Esmaili et al. 2022). Therefore, the T2 treatment was able to mitigate the reduction in leaf area under DN.
Chlorophyll (Chl) plays an active role in photosynthesis, a process by which plants assimilate, distribute, and transform solar energy (Agnihotri and Seth 2016). The physiological characteristics of rice seedlings are influenced by the rice cultivation method. Enhanced photosynthesis from more Chl contents in leaves resulted in healthier, stronger seedlings (Kumar et al. 2023). Under drought conditions, the decrease in photochemical efficiency of photosystem II may be associated with the decrease in photosynthetic pigment contents of leaves (Song et al. 2014). Yadav et al. (2022) also showed that under cadmium stress, the Chl a, Chl b, total Chl, and carotenoid contents significantly decreased. Carotenoids are important antioxidants, which can dissipate the excess excitation energy in the photosystem II light-harvesting antenna, especially under stress (Li et al. 2021). Azim et al. (2022) also showed that ZnO NPs enhanced the Chl and carotenoid contents in tomato seedlings. Similarly, in this study, La2O3 NPs application significantly alleviated the reduction in Chl a, Chl b, and carotenoid contents of DN seedlings (Fig. 1). This improves phytochrome content and ensures photosynthesis in rice seedling leaves. Thus, better photosynthesis led to the rapid growth of fragrant rice seedlings. Considering that La2O3 NPs can significantly regulate light utilization and electron transport in photosynthesis (Liu et al. 2020a), we deduced that fragrant rice seedlings uptake La2O3 NPs and consequently enhance photosynthesis.
In addition, increased activities of SOD, POD, and CAT and decreased MDA contents were observed in the fragrant rice seedlings growing in the presence of La2O3 NPs under T1 treatment (Fig. 2). Sun et al. (2020) suggested that stress causes an increase in reactive oxygen species (ROS), resulting in lipid peroxidation in the cell membrane system and MDA formation. MDA is an essential measure of oxidative stress. Gupta and Seth (2020) illustrated that the activation of the cellular antioxidant system is an essential method for safeguarding against diverse environmental stresses by scavenging ROS generated therein. SOD, POD, and CAT play significant roles in the antioxidative defense mechanisms of plants to suppress ROS (Ashraf et al. 2018). Some substances can help plants to resist adversity. For example, 24-Epibrassinolide could modulate the antioxidant defense mechanism of Brassica juncea L., inhibit oxidative damage to cell membranes by salt stress, and reduce the accumulation of oxides, improving plant growth (Gupta and Seth 2022). Rice under stress experienced less oxidative damage because of ZnO NPs application (Li et al. 2021). This might be because these NPs helped to neutralize ROS by triggering antioxidant enzyme systems (Khan et al. 2017). Similarly, in this study, to reduce ROS accumulation under DN conditions, La2O3 NPs treatment greatly increased SOD, POD, and CAT activities (Fig. 2). ROS and MDA levels in rice leaves decreased due to higher antioxidant enzyme activities in plants after La2O3 NPs application, which also decreased the induction of lipid peroxidation. Thus, when applied in proper concentrations, La2O3 NPs could enhance seedling resistance and increase the contents of carbon and nitrogen compounds, thus laying a good foundation for field transplanting.
Nitrogen absorbed by crop roots must be converted by nitrogen metabolism-related enzymes, including GS, NR, and GOGAT, into organic substances that can be used by plants. These three enzymes are necessary and play significant roles in nitrogen metabolism (Hu et al. 2021). However, Gupta et al. (2017) showed that salt stress led to a reduction in NR, GS, and GOGAT activities, and total nitrogen content, thus impeding nitrogen metabolism. GS has multiple functions and is associated with various nitrogen metabolic pathways (Sanz-Luque et al. 2015). Enhanced GS and GOGAT activities facilitate the generation of organic nitrogen (Hu et al. 2021). Increasing irrigation can increase both GOGAT and GS activities (Zhang et al. 2022). Wang et al. (2018) also claimed that endogenous stress-resistant compounds that can withstand stress increase because of the increase in GOGAT activity. Similarly, in this study, GS activity in leaves in both cultivars was found to increase with the increase in La2O3 NPs concentration (Fig. 3a). GOGAT activity in leaves of ‘Xiangyaxiangzhan’ under La2O3 NPs treatment was dramatically increased compared with CK (Fig. 3e). NR is the main nitrogen source entering plants and is considered the primary signaling enzyme in plants (Chamizo-Ampudia et al. 2017). This study revealed that NR activities in leaves and roots were lower under La2O3 NPs treatment than under CK treatment and significantly varied with La2O3 NPs concentration and rice cultivars (Fig. 3 a and b). These findings agree with those of Ahanger et al. (2021), which demonstrated that applying nano-compost dramatically reduced NR activity due to drought treatment. Gupta and Seth (2020) also suggested that the reduction in NR activity during salt stress was because of the oxidative modification of the NR protein caused by an excessive amount of ROS generated by stress. Therefore, this study found that La2O3 NPs application increased the activity of GS and GOGAT in rice seedling leaves and decreased the activity of NR in leaves and roots compared to CK.
Overall, La2O3 NPs treatment at different concentrations could affect chlorophyll contents, antioxidant enzyme activities, MDA content, and nitrogen metabolism in the studied rice cultivars under different raising nurseries. The potential cause of the observed results was the slight difference in the morphological index under La2O3 NPs treatment but a significant change in the physiological indexes (Fig. 5).
Seedling raising methods and their interaction with La2O3 NPs regulated the dry weight of fragrant rice. Changes in plant morphological parameters (e.g., shoot dry weight/plant height and root–shoot ratio) were the reason for the differences in fragrant rice cultivars in response to La2O3 NPs application. These changes resulted from the changes in the leaf area, specific leaf area, the chlorophyll contents, antioxidant properties, and activities of nitrogen metabolism enzymes
Conclusion
Our study indicated that the La2O3 NPs concentration of 40 mg L−1 was beneficial, as it significantly increased the leaf area of seedlings grown by both WN and DN methods due to changes in morphological and physiological parameters, thereby providing a theoretical basis for La2O3 NPs application in agriculture. However, this study lacks molecular biology analysis of rice seedlings after La2O3 NPs application using different seedling-raising methods and electron microscopy studies after La2O3 NPs enter rice seedlings. The results of this study provide a theoretical basis for expanding the research on La2O3 NPs application in rice, as well as relevant references for strengthening rice seedlings in the nursery, which has a positive outcome of grain yield increase.
Data availability
All data generated or analyzed during this study are included in this article.
Abbreviations
- WN:
-
Wet nursery
- DN:
-
Dry nursery
- CK:
-
La2O3 NPs concentration of 0 mg L−1
- T1:
-
La2O3 NPs concentration of 20 mg L−1
- T2:
-
La2O3 NPs concentration of 40 mg L−1
References
Agnihotri A, Seth CS (2016) Exogenously applied nitrate improves the photosynthetic performance and nitrogen metabolism in tomato (Solanum lycopersicum L. cv Pusa Rohini) under arsenic (V) toxicity. Physiol Mol Biol Plants 22:341–349. https://doi.org/10.1007/s12298-016-0370-2
Ahanger MA, Qi MD, Huang ZG, Xu XD, Begum N, Qin C, Zhang CX, Ahmad N, Mustafa NS, Ashraf M, Zhang LX (2021) Improving growth and photosynthetic performance of drought stressed tomato by application of nano-organic fertilizer involves up-regulation of nitrogen, antioxidant and osmolyte metabolism. Ecotoxicol Environ Saf 216. https://doi.org/10.1016/j.ecoenv.2021.112195
Ashraf U, Hussain S, Akbar N, Anjum SA, Hassan W, Tang XR (2018) Water management regimes alter Pb uptake and translocation in fragrant rice. Ecotoxicol Environ Saf 149:128–134. https://doi.org/10.1016/j.ecoenv.2017.11.033
Awan TH, Chauhan BS, Cruz PCS (2014) Physiological and mor phological responses of Ischaemumrugosum salisb. (Wrinkled Grass) to different nitrogen rates and rice seeding rates. Plos One 9(6):e98255. https://doi.org/10.1371/journal.pone.0098255
Azim Z, Singh NB, Khare S, Singh A, Amist N, Niharika Yadav RK, Hussain I (2022) Potential role of biosynthesized zinc oxide nanoparticles in counteracting lead toxicity in Solanum lycopersicum L. Plant Nano Biology 2:100012. https://doi.org/10.1016/j.plana.2022.100012
Chamizo-Ampudia A, Sanz-Luque E, Llamas A, Galvan A, Fernandez E (2017) Nitrate reductase regulates plant nitric oxide homeostasis. Trends Plant Sci 22:163–174. https://doi.org/10.1016/j.tplants.2016.12.001
Chen JL, Zeng XY, Yang WJ, Xie HJ, Ashraf U, Mo ZW, Liu JH, Li GK, Li W (2021) Seed priming with multiwall carbon nanotubes (MWCNTs) modulates seed germination and early growth of maize under cadmium (Cd) toxicity. J Soil Sci Plant Nutr 21:1793–1805. https://doi.org/10.1007/s42729-021-00480-6
Cheng SR, Ashraf U, Zhang TT, Mo ZW, Kong LL, Mai YX, Huang HL, Tang XR (2018) Different seedling raising methods affect characteristics of machine-transplanted rice seedling. Appl Ecol Environ Res 16:1399–1412. https://doi.org/10.15666/aeer/1602_13991412
Dai H, Shan C, Zhao H, Jia G, Chen D (2017) Lanthanum improves the cadmium tolerance of Zea mays seedlings by the regulation of ascorbate and glutathione metabolism. Biol Plant 61(3):551–556. https://doi.org/10.1007/s10535-016-0669-4
Deng SY, Ashraf U, Nawaz M, Abbas G, Tang XR, Mo ZW (2022) Water and nitrogen management at the booting stage affects yield, grain quality, nutrient uptake, and use efficiency of fragrant rice under the agro-climatic conditions of south china. Front Plant Sci 13:907231. https://doi.org/10.3389/fpls.2022.907231
Dou Z, Li YY, Guo HL, Chen LR, Jiang JL, Zhou YC, Xu Q, Xing ZP, Gao H, Zhang HC (2021) Effects of mechanically transplanting methods and planting densities on yield and quality of Nanjing 2728 under rice-crayfish continuous production system. Agronomy 11. https://doi.org/10.3390/agronomy11030488
Esmaili S, Tavallali V, Amiri B, Bazrafshan F, Sharafzadeh S (2022) Foliar application of nano-silicon complexes on growth, oxidative damage and bioactive compounds of feverfew under drought stress. SILICON. https://doi.org/10.1007/s12633-022-01754-z
Garner KL, Suh S, Keller AA (2017) Assessing the risk of engineered nanomaterials in the environment: development and application of the nanoFate model. Environ Sci Technol 51:5541–5551. https://doi.org/10.1021/acs.est.6b05279
Gupta P, Seth CS (2020) Interactive role of exogenous 24 epibrassinolide and endogenous NO in Brassica juncea L. under salinity stress: Evidence for NR-dependent NO biosynthesis. Nitric Oxide 97:33–47. https://doi.org/10.1016/j.niox.2020.01.014
Gupta P, Seth CS (2022) 24-epibrassinolide regulates functional components of nitric oxide signalling and antioxidant defense pathways to alleviate salinity stress in Brassica juncea L. cv. Varuna. J Plant Growth Reg 1–16. https://doi.org/10.1007/s00344-022-10884-y
Gupta P, Srivastava S, Seth CS (2017) 24-epibrassinolide and sodium nitroprusside alleviate the salinity stress in Brassica juncea L. cv. Varuna through cross talk among proline, nitrogen metabolism and abscisic acid. Plant Soil 411:483–498. https://doi.org/10.1007/s11104-016-3043-6
Hu YM, Zhang P, Zhang X, Liu YQ, Feng SS, Guo DW, Nadezhda T, Song ZJ, Dang XL (2021) Multi-wall carbon nanotubes promote the growth of maize (Zea mays) by regulating carbon and nitrogen metabolism in leaves. J Agric Food Chem 69:4981–4991. https://doi.org/10.1021/acs.jafc.1c00733
Karimi S, Rahemi M, Rostami AA, Sedaghat S (2018) Drought effects on growth, water content and osmoprotectants in four olive cultivars with different drought tolerance. Int J Fruit Sci 18:254–267. https://doi.org/10.1080/15538362.2018.1438328
Khan MN, Mobin M, Abbas ZK, Almutairi KA, Siddiqui ZH (2017) Role of nanomaterials in plants under challenging environments. Plant Physiol Biochem 110:194–209. https://doi.org/10.1016/j.plaphy.2016.05.038
Kumar D, Dhankher OP, Tripathi RD, Seth CS (2023) Titanium dioxide nanoparticles potentially regulate the mechanism(s) for photosynthetic attributes, genotoxicity, antioxidants defense machinery, and phytochelatins synthesis in relation to hexavalent chromium toxicity in Helianthus annuus L. J Hazard Mater 454. https://doi.org/10.1016/j.jhazmat.2023.131418
Kumar D, Seth CS (2021) Green-synthesis, characterization, and applications of nanoparticles (NPs): a mini review. Int J Plant Environ 7(01):91–95. https://doi.org/10.18811/ijpen.v7i01.11
Lampayan RM, Samoy-Pascual KC, Sibayan EB, Ella VB, Jayag OP, Cabangon RJ, Bouman BAM (2015) Effects of alternate wetting and drying (AWD) threshold level and plant seedling age on crop performance, water input, and water productivity of transplanted rice in Central Luzon, Philippines. Paddy Water Environ 13:215–227. https://doi.org/10.1007/s10333-014-0423-5
Liao GX, Yang, YH. Xiao, WM, Mo, ZW (2022) Nitrogen modulates grain yield, nitrogen metabolism, and antioxidant response in different rice genotypes. J Plant Growth Reg 0721–7595. https://doi.org/10.1007/s00344-022-10684-4
Li JX, Song YC, Wu KR, Tao Q, Liang YC, Li TQ (2018) Effects of Cr23 nanoparticles on the chlorophyll fluorescence and chloroplast ultrastructure of soybean (Glycine max). Environ Sci Pollut Res 25:19446–19457. https://doi.org/10.1007/s11356-018-2132-x
Li YX, Liu Y, Wang YH, Ding YF, Wang SH, Liu ZH, Li GH (2020) Effects of seedling age on the growth stage and yield formation of hydroponically grown long-mat rice seedlings. J Integr Agric 19(7):1755–1767. https://doi.org/10.1016/S2095-3119(19)62756-5
Li YZ, Liang LX, Li W, Ashraf U, Ma L, Tang XR, Pan SG, Tian H, Mo ZW (2021) ZnO nanoparticle-based seed priming modulates early growth and enhances physio-biochemical and metabolic profiles of fragrant rice against cadmium toxicity. J Nanobiotechnol 19. https://doi.org/10.1186/s12951-021-00820-9
Liu YL, Yue L, Wang CX, Zhu XS, Wang ZY, Xing BS (2020a) Photosynthetic response mechanisms in typical C3 and C4 plants upon La2O3 nanoparticle exposure. Environ Sci Nano 7:81–92. https://doi.org/10.1039/C9EN00992B
Liu XW, Huang ZL, Li YZ, Xie WJ, Li W, Tang XR, Ashraf U, Kong LL, Wu LM, Wang SL, Mo ZW (2020b) Selenium-silicon (Se-Si) induced modulations in physio-biochemical responses, grain yield, quality, aroma formation and lodging in fragrant rice. Ecotoxicol Environ Saf 196. https://doi.org/10.1016/j.ecoenv.2020.110525
Luo HW, Chen YL, He LX, Tang XR (2021) Lanthanum (La) improves growth, yield formation and 2-acetyl-1-pyrroline biosynthesis in aromatic rice (Oryza sativa L). BMC Plant Biol 21:233. https://doi.org/10.1186/s12870-021-03006-5
Mohapatra B, Chamoli S, Salvi P, Saxena SC (2023) Fostering nanoscience’s strategies: a new frontier in sustainable crop improvement for abiotic stress tolerance. Plant Nano Biol 3:100026. https://doi.org/10.1016/j.plana.2023.100026
Neves VM, Heidrich GM, da Costa CC, Farias JG, Nicoloso FT, Pozebon D, Dressler VL (2022) Effects of La2O3 nanoparticles and bulk - La2O3 on the development of Pfaffiaglomerata (Spreng.) Pedersen and respective nutrient element concentration. Environ Sci Pollut Res 29:60084–60097. https://doi.org/10.1007/s11356-022-20117-0
Nhan LV, Ma CX, Rui YK, Liu ST, Li XG, Xing BS, Liu LM (2015) Phytotoxic mechanism of nanoparticles: destruction of chloroplasts and vascular bundles and alteration of nutrient absorption. Sci Rep 5. https://doi.org/10.1038/srep11618
Qing BW, Jiang Y, Chen YJ, Chen JL, Xie HJ, Mo ZW (2022) Nitrogen modulates early growth and physio-biochemical attributes in fragrant rice grown under cadmium and multiwall carbon nanotubes stresses. Environ Sci Pollut Res 29:67837–67855. https://doi.org/10.1007/s11356-022-20432-6
Rastogi A, Zivcak M, Sytar O, Kalaji HM, He XL, Mbarki S, Brestic M (2017) Impact of metal and metal oxide nanoparticles on plant: a critical review. Front Chem 5. https://doi.org/10.3389/fchem.2017.00078
Sanz-Luque E, Chamizo-Ampudia A, Llamas A, Galvan A, Fernandez E (2015) Understanding nitrate assimilation and its regulation in microalgae. Front Plant Sci 6. https://doi.org/10.3389/fpls.2015.00899
Song A, Li P, Fan FL, Li ZJ, Liang YC (2014) The effect of silicon on photosynthesis and expression of its relevant genes in rice (Oryza sativa L.) under high-zinc stress. Plos One 9. https://doi.org/10.1371/journal.pone.0113782
Sun LY, Song FB, Guo JH, Zhu XC, Liu SQ, Liu FL, Li XN (2020) Nano-ZnO-induced drought tolerance is associated with melatonin synthesis and metabolism in maize. Int J Mol Sci 21. https://doi.org/10.3390/ijms21030782
Tao YY, Qu H, Li QJ, Gu XH, Zhang YN, Liu MJ, Guo L, Liu J, Wei JJ, Wei GJ, Shen KR, Dittert K, Lin S (2014) Potential to improve N uptake and grain yield in water saving ground cover rice production system. Field Crop Res 168:101–108. https://doi.org/10.1016/j.fcr.2014.08.014
Timmusk S, Abd El-Daim IA, Copolovici L, Tanilas T, Kannaste A, Behers L, Nevo E, Seisenbaeva G, Stenstrom E, Niinemets U (2014) Drought-tolerance of wheat improved by rhizosphere bacteria from harsh environments: enhanced biomass production and reduced emissions of stress volatiles. Plos One 9. https://doi.org/10.1371/journal.pone.0096086
Valojai STS, Niknejad Y, Amoli HF, Tari DB (2021) Response of rice yield and quality to nano-fertilizers in comparison with conventional fertilizers. J Plant Nutr 44:1971–1981. https://doi.org/10.1080/01904167.2021.1884701
Wang JL, Fu ZS, Chen GF, Zou GY, Song XF, Liu FX (2018) Runoff nitrogen (N) losses and related metabolism enzyme activities in paddy field under different nitrogen fertilizer levels. Environ Sci Pollut Res 25:27583–27593. https://doi.org/10.1007/s11356-018-2823-3
Xiao ZG, Fan NK, Wang X, Ji HH, Yue L, He F, Wang ZY (2022) Earthworms drive the effect of La2O3 nanoparticles on radish taproot metabolite profiles and rhizosphere microbial communities. Environ Sci Technol 56(23):17385–17395. https://doi.org/10.1021/acs.est.2c05828
Xiao ZG, Yue L, Wang CX, Chen FR, Ding Y, Liu YL, Cao XS, Chen Z, Rasmann S, Wang ZY (2021) Downregulation of the photosynthetic machinery and carbon storage signaling pathways mediate La2O3 nanoparticle toxicity on radish taproot formation. J Hazard Mater 411(5):124971. https://doi.org/10.1016/j.jhazmat.2020.124971
Xu C, Qu XG (2014) Cerium oxide nanoparticle: a remarkably versatile rare earth nanomaterial for biological applications. NPG Asia Mater 6. https://doi.org/10.1038/am.2013.88
Yadav M, Gupta P, Seth CS (2022) Foliar application of α-lipoic acid attenuates cadmium toxicity on photosynthetic pigments and nitrogen metabolism in Solanum lycopersicum L. Acta Physiol Plant 44:112. https://doi.org/10.1007/s11738-022-03445-z
Yang H, Xiong ZT, Xu ZR, Liu RX (2021) Interactive effects of lanthanum and calcium on cadmium accumulation in wheat with special reference to TaNramp5 expression regulated by calmodulin. J Agric Food Chem 69(24):6870–6878. https://doi.org/10.1021/acs.jafc.1c00365
Yue L, Ma CX, Zhan XH, White JC, Xing BS (2017) Molecular mechanisms of maize seedling response to La2O3 NP exposure: water uptake, aquaporin gene expression and signal transduction. Environ Sci Nano 4:843–855. https://doi.org/10.1039/C6EN00487C
Zhang HC, Gong JL (2014) Research status and development discussion on high-yielding agronomy of mechanized planting rice in China. Scientia Agricultura Sinica 47(7):1273–1289 (in Chinese)
Zhang HP, Wang R, Chen ZQ, Pu JL, Wang JJ, Zhang HC, Yang YJ (2022) Nanoscale molybdenum oxide improves plant growth and increases nitrate utilisation in rice (Oryza sativa L). Food and Energy Secur 11:e383. https://doi.org/10.1002/fes3.383
Funding
Our research is funded by the Shanwei City provincial science and technology innovation strategy special project in 2022 (Shankezi [2022] No. 277, Project Number: 2022B002) and the Guangdong provincial key field research and development plan project (2019B020221003).
Author information
Authors and Affiliations
Contributions
Zhaowen Mo designed the experiments; Weifen Chen, Gaoxin Liao, and Feiyang Sun examined the characteristics; Zhaowen Mo, Weifen Chen, Zhilong Chen, Feiyang Sun, and Haoming Chen did the data analysis and paper writing; Weifen Chen, Yixian Ma, Gaoxin Liao, Zhilong Chen, Haoming Chen, Xiangru Tang, and Zhaowen Mo revised and edited the manuscript. The final manuscript was read and approved by all authors.
Corresponding author
Ethics declarations
Ethical approval
Not relevant.
Consent to participate
Not relevant.
Consent for publication
Not relevant.
Competing interests
The authors declare no competing interests.
Additional information
Responsible Editor: Gangrong Shi
Publisher's note
Springer Nature remains neutral with regard to jurisdictional claims in published maps and institutional affiliations.
Rights and permissions
Springer Nature or its licensor (e.g. a society or other partner) holds exclusive rights to this article under a publishing agreement with the author(s) or other rightsholder(s); author self-archiving of the accepted manuscript version of this article is solely governed by the terms of such publishing agreement and applicable law.
About this article
Cite this article
Chen, W., Liao, G., Sun, F. et al. Foliar spray of La2O3 nanoparticles regulates the growth, antioxidant parameters, and nitrogen metabolism of fragrant rice seedlings in wet and dry nurseries. Environ Sci Pollut Res 30, 80349–80363 (2023). https://doi.org/10.1007/s11356-023-27892-4
Received:
Accepted:
Published:
Issue Date:
DOI: https://doi.org/10.1007/s11356-023-27892-4