Abstract
The chronic toxicity of diclofenac (DCF) and carbamazepine (CBZ) as separate substances and in conjunction with their mixture on Daphnia magna was assessed in the parental (F0) and first filial (F1) generations. The second (F1–B2) and fifth (F1–B5) broods of F1 offspring were investigated and compared. Both drugs and their mixture were exposed to each generation of Daphnia magna for 21 days with life history, behavioural and gene expressions as measured endpoints. After the parental exposure, offspring from these two broods were transferred to a clean medium for a 21-day recovery. Exposure to diclofenac, carbamazepine and their mixture significantly inhibited growth, reproduction, swimming activities, heart rate, thoracic limb activities, reproductive and antioxidant-related genes in the parental as well as the first filial generations. These effects were relatively greater in the F1 generation. This indicates that Daphnia magna’s sensitivity improved while its fitness declined over the two generations, which is an indicator of greater energy requirements for maintenance. Besides, the significant inhibition in the antioxidant-related genes implies that oxidative stress occurred in Daphnia magna under the exposure to these drugs. The significant reduction in the reproductive output, moulting frequency and cyp314 gene expression as a result of exposure to CBZ simultaneously obtained herein may indicate that this drug could act as an endocrine disruptor. Most of these significant effects were not recoverable after the 21-day recovery period. The findings reported herein highlight the necessity to include maternal effects in environmental risk assessment processes, considering that pollutant effects are underestimated during single-generational exposure.
Similar content being viewed by others
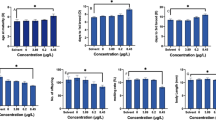
Explore related subjects
Discover the latest articles, news and stories from top researchers in related subjects.Avoid common mistakes on your manuscript.
Introduction
In recent years, many researchers have knuckled down on pharmaceutically active compounds including contrast media, β-blockers, controls for blood lipids, antibiotics, neuroactive, anti-inflammatory and antiepileptic drugs which belong to the group of emerging contaminants. These pharmaceuticals consist of a variety of organic compounds intended to treat and prevent diseases in order to enhance wellbeing (Liu and Wong, 2013). As a result of their large amounts of production and their widespread use worldwide, pharmaceuticals are continually released into the aquatic environment. Besides, researchers who have focused on pharmaceutical degradation have reported that most of the classical treatments are not effective in eliminating these compounds in wastewater treatment plants, making these contaminants a public health threat (Rasheed et al., 2019).
The antiepileptic drug carbamazepine and the non-steroidal anti-inflammatory drug diclofenac were chosen for this study because they are prevalent drugs used throughout the world. Intensive studies undertaken in the last few decades have confirmed that both drugs are ubiquitously present in the various environmental compartments. Although these drugs are frequently used by humans, previous investigators have reported that they can also affect the physiological, behavioural and biochemical processes in nontarget aquatic organisms (Liu et al., 2017; Nkoom et al., 2019a; Oliveira et al., 2017; Rivetti et al., 2016). However, these toxicological investigations were conducted for a short period of time, as particularly short-term toxicity experiments were carried out. Notwithstanding, because pharmaceuticals are continuously discharged into the environment, species are exposed to these contaminants for many generations during their entire lives. According to Kim et al. (2014), researchers did not pay much attention to the multigenerational impacts of pharmaceuticals.
In order to have a more ecologically relevant insight into the effects of these drugs on the ecosystem, investigations are needed to evaluate their transgenerational effects by multigenerational exposure. Furthermore, our understanding of the mixture effect of pharmaceuticals in the aquatic environment is unclear. Given that not just individual pharmaceuticals but their mixture typically pollute the aquatic environment, it is imperative to know the potential joint impacts of such compounds (Dietrich et al., 2010). Previous studies have demonstrated that mixtures of the non-steroidal anti-inflammatory drugs, antiepileptic drugs and other therapeutic classes of pharmaceuticals and personal care products can produce effects that are different from those expected from the individual compounds (Silva et al., 2019). Besides, most of the available research on the ecotoxicological effects of pharmaceuticals are centred on much higher concentrations than those present in the aquatic ecosystems. Therefore, studies are required to understand the effects of pharmaceuticals at environmentally realistic concentrations.
Daphnia magna is a good biological specimen for conducting multigenerational investigations by virtue of its distinctive features such as short life span, high fertility, parthenogenetic reproduction and uncomplicated handling in the laboratory (Harris et al., 2012). Due to these features, Daphnia magna is widely used to assess the effects of contaminants in ecological risk assessment procedures (Tkaczyk et al., 2021). The chronic toxicity tests for Daphnia magna that are described in the OECD 211 guideline (OECD, 2012) last over only one generation. All information on environmental disorders that are passed on from mothers to their offspring (maternal effects) and the offspring’s health status (LaMontagne and McCauley, 2001) are not taken into account. Changes in populations resulting from long-term exposure to pollutants are diverse and may result in reduced sensitivity or tolerance or enhanced sensitivity (Scherer et al., 2013). Both results have significant management implications, but toxicity tests of short period cannot indicate either. Daphnia magna acts as an intermediary between primary producers and fish in aquatic food chains. Therefore, any change in their life history, as well as morphological parameters throughout multiple generations, may bring about repercussions at the next level of the community or ecosystem. Recognising these issues, multigenerational studies may be of great importance as they could provide estimates of population effects and support the process of chemical risk assessment in the aquatic ecosystem.
Bearing in mind the above, and bridging the gap in knowledge on long-term exposure studies, the objective of this study was to assess the chronic toxicity of diclofenac and carbamazepine alone and in combination under 21-day exposure to Daphnia magna in the parental generation (F0) as well as the first filial generation offspring (F1) birthed in the conditions of exposure. The second (F1–B2) and fifth (F1–B5) broods of F1 offspring were observed and compared. Therefore, by investigating two consecutive generations, assessment can be made (1) whether the chronic toxicity may vary between the generation of parents and offspring; (2) whether the chronic toxicity of the drugs might differ between different broods; and (3) whether offspring transferred after parental exposure to a clean medium may recover from maternal effects within 21 days. The chronic toxicity parameters evaluated herein encompasses reproductive indicators (age at first brood [the age at which the first offspring was released or born], number of first brood and total number of offspring per female), growth and development indicators (body length, intrinsic growth rate and moulting frequency). In addition, swimming activities (velocity, accelerated velocity, distance covered), heart rate, thoracic limb activity, antioxidant-related genes (superoxide dismutase [sod], catalase [cat] and glutathione-S-transferase [gst]) and reproduction and development-related genes (vitellogenin [Vtg] and cytochrome P450 314 family [cyp314]) were also determined to thoroughly assess the chronic toxic effects of these pharmaceuticals on Daphnia magna.
Materials and methods
Chemicals and reagents
Aladdin (Shanghai, China) Industrial Company supplied diclofenac (C14H11Cl2NO2; CAS number 15307–86-5; ≥ 98% purity) as well as carbamazepine (C16H12N2O; CAS number 298–46-4; ≥ 97% purity) used in this study. Methanol (HPLC grade) was purchased from Merck Serono Co., Ltd. (Darmstadt, Germany). The Milli-Q integral water purification method (Millipore, Milford, MA, USA) was used to generate deionised water.
Daphnia culture and multigenerational exposure
Daphnia magna was purchased from the Institute of Hydrobiology, Chinese Academy of Sciences (Wuhan, China). The daphnids were cultivated continuously in artificial freshwater (AFW) according to the conditions explicated in the OECD standard procedure for the testing of chemicals as well as that outlined in our previous studies (Nkoom et al., 2019b, 2019a; OECD, 2004). The media used in culturing Daphnia magna were renewed three times every week. Each day Daphnia magna was nourished with 1 × 106 cells/mL of green algae (Scenedesmus obliquus; Institute of Hydrobiology, Chinese academy of sciences). An intelligent incubator of artificial climate (RQX-400H, China) was used in keeping the Daphnia magna culture with a light–dark interval of 16:8 at 20 ± 1 °C.
The two-generational Daphnia magna experiments were performed according to the OECD guideline 211 (OECD, 2012). The stock solutions of diclofenac and carbamazepine were prepared using methanol with not more than 0.01% in the final media that were exposed to the daphnids. In the individual drug treatments, Daphnia magna was exposed to nominal concentrations of 2 μg/L (DCF2 and CBZ2) and 10 μg/L (DCF10 and CBZ10) diclofenac and carbamazepine. In surface water, diclofenac has been detected up to 15.013 μg/L in Erft river, Germany (Jux et al., 2002) and carbamazepine up to 67.715 μg/L in Jarama river, Spain (Valcárcel et al., 2011). The exposure concentrations were chosen based on these environmental concentrations. For easy comparison with the results of the individual exposures, the concentration ratio for the mixtures was 1:1, as 1 + 1 (M1) and 5 + 5 (M2) μg/L, respectively. The experiments included a culture medium and 0.01% methanol that served as the blank and solvent control groups. For parental generation (F0) exposure, one neonate (< 24 h) was placed in a glass beaker of 100 mL containing 50 mL of the test solution. Daphnia magna was provided with 1 × 106 cells/mL green algae every day. The exposure media were freshened daily. Exposure to filial generation (F1) was compared with two groups of neonates not more than 24 h of age, chosen from the 2nd (F1–B2) or 5th (F1–B5) broods of the parent generation. In order to investigate whether the toxicity of the drugs could vary between different broods, the 2nd (F1–B2) and 5th (F1–B5) broods were chosen because the time period between them is comparatively longer over the 21-day life cycle (Minguez et al., 2015).
Daphnids of the F1 generation (F1–B2 and F1–B5) were either exposed to the same drug concentration as exposed to the F0 generation or retreated to a media without drugs to evaluate the recovery capability of the F1 offspring from exposure in the F0 generation. Ten replicates were assessed throughout the 21-day period for the treatment group per generation, with one Daphnia magna neonate in every replicate treatment. The experimental design is shown in Fig. S1. At every 24 h during the exposure period, Daphnia magna was monitored with the removal of newborn neonates and a record was kept on the time of first brood, neonate numbers and moulting frequency. Using a stereomicroscope, body length was determined at the end of the experiment. The intrinsic growth rate (r) values were determined using the Euler-Lotka equation (Lotka, 1913):
where x is time of experiment in days; \({l}_{x}\) is the ratio of survival at day x; and \({m}_{x}\) is the number of neonates produced per surviving adult (fecundity) at day x.
Swimming behaviour and physiological indicator analysis
The heart rate, swimming and thoracic limb activities were measured based on a methodology outlined in a previous study (Yang et al., 2018). Concisely, five individuals were removed from the glass beakers after the 21-day exposure and placed in culture plates. The wells of the plates were filled with exposure media to approximately 2 cm and a daphnid to allow for lateral movements with negligible vertical motion. The swimming behaviour was recorded for 1 min after 5 min of adaptation with a digital camera (EOS 1500D, Canon, Japan) set to 30 frames/s. With the Tracker ® software, Daphnia swimming tracks were digitally analysed using a frame-by-frame process. For ocular determination of heart rate and thoracic limb movement, one Daphnia magna was mounted on a slide of a microscope containing 50 μL of exposure media. The movements of the daphnids were regulated with cotton wool fibres. A digital camera (Canon, Japan, EOS 1500D) was used to capture the microscopic view of the exposed daphnids for more than a minute. In a frame-by-frame process, the heart rates and thoracic limb movements were analysed using the Tracker ® software at a speed of 30 frames/s.
Gene transcription analysis
Total RNA was extracted from the daphnids (five individuals) using a TRIzol reagent (Invitrogen Corp., Carlsbad, CA, USA) relying on guidelines from the producer. Two microliters of the original cDNA (diluted tenfold) was added to a reaction tube that contains 0.1 μM primer and 0.25 × FastStart Universal SYBR GREEN Master (Roche, Germany), resulting in a total volume of 20 μL. An Eppendorf main ring EP real-time PCR detection system (Eppendorf, Germany) was used to quantify the expression of the target genes (Vtg, cyp314, sod, cat and gst). The quantitative RT-PCR amplification procedures were as follows: the first was a pre-denaturation at 95 °C for 3 min, then 40 cycles of denaturation at 95 °C for 10 s and annealing and extension at 60 °C for 1 min. Samples were determined in triplicate. The gene actin was selected as an internal control. Primers for the genes were chosen based on previous studies (S. Liu et al., 2019a, b; Liu et al., 2017) as shown in Table S1. The outcomes of the transcription of the genes were computed following the 2−△△CT method (Livak and Schmittgen, 2001).
Determination of diclofenac and carbamazepine concentration in exposure water
Diclofenac and carbamazepine were extracted and analysed as described in our previous study (Nkoom et al., 2020). In brief, water samples (10 mL) were extracted and cleaned using Oasis HLB cartridges eluted with 6 mL of methanol. Finally, the extracts were evaporated to dryness under a stream of nitrogen and reconstituted in 1 mL methanol. It was then analysed by ultra-high-performance liquid chromatography-tandem mass spectrometry (Agilent, Waldbronn, Germany). Further details are in the supplementary material.
Statistical analysis
The mean and standard deviations were calculated using Microsoft excel. Statistical analyses were performed with SPSS Statistics 24.0 software. Data were evaluated for normality (Shapiro–Wilk test) and homogeneity of variances (Levene test). One-way analysis of variance (ANOVA) and Tukey’s post hoc tests were used to evaluate the significant differences between the exposure groups and control. Experimental data were reported as the mean ± standard deviation of ten replicates. Pearson test was used to evaluate the relationship between the parameters. At p < 0.05, all differences were considered significant.
Results and discussions
Significant differences were not observed in the blank control as well as the solvent control groups; therefore, results of the tested endpoints for both drugs including the mixture were compared with the solvent control.
Reproductive toxicity parameters
Fecundity has been considered as an appropriate indicator endpoint to detect and evaluate disruption in organisms after exposure to environmental contaminants (Zhu et al., 2015). Concerning reproductive parameters, exposure of daphnids to DCF, CBZ and their mixture considerably delayed the age at first reproduction in a dose–response relationship. The average age at first reproduction of Daphnia magna following exposure to DCF ranged from 7.30 to 8.40, 8.95 to 9.70 and 10.6 to 11.4 days in the F0, F1–B2 and F1–B5 generations, respectively. These values are significantly different from that obtained in the control group (Fig. 1A). This implies that the age at which the daphnids produced their first offspring was delayed because of their exposure to DCF. The rate at which the ages at first reproduction were delayed in the daphnids that were exposed to DCF was greater in the F1–B5 generation, followed by the F1–B2 generation. In contrast, the F0 generation recorded the least delay. These results are consistent with those obtained in a previous study when daphnids were exposed to 50 μg/L DCF for a 21-day life history assessment (Liu et al., 2017).
(A) Age at first brood, (C) number of first brood per female and (E) total number of offspring per female daphnia after 21-day exposure to DCF, CBZ and their mixture; (B) age at first brood, (D) number of first brood per female and (F) total number of offspring per female after the transition to a clean medium for 21-day recovery. Data are delineated as mean ± standard deviation (n = 10). Significant differences (p < 0.05) between treatments from an exposure scenario are indicated by different letters
In addition, in the F0, F1–B2 and F1–B5 generations, the average age at first reproduction for Daphnia magna following exposure to CBZ was in the range of 7.60–9.40, 8.90–10.8 and 10.2–12.4 days, respectively. These results mean that the age at first reproduction for daphnids exposed to CBZ was significantly delayed in a concentration-dependent manner (Fig. 1A). The rate of delay was greater in the F1–B5 generation, followed by the F1–B2 generation, while the F0 generation recorded the least delay. Results obtained herein are consistent with a recent study that documented significantly delayed age at first reproduction when daphnids were exposed to 1, 500 and 5000 μg/L CBZ for 21 days (Tian et al., 2019). Comparing the results of the two drugs, CBZ delayed the age at first reproduction for daphnids in the F0, F1–B2 and F1–B5 generations more than DCF most especially at 10 μg/L.
In the mixture treatment, 8.0–9.0, 9.50–10.5 and 11–12 days are the ranges obtained for the average age at first reproduction for daphnids in the F0, F1–B2 and F1–B5 generations, respectively (Fig. 1A). These findings suggest that for Daphnia magna treated with the mixture, the age at first reproduction was significantly delayed in a concentration-dependent manner. The rate was greater in the F1–B5 generation, followed by the F1–B2 generation, while the F0 generation recorded the least delay. Since this study is the first of its kind to examine the combined chronic effects of both drugs, there are no studies to compare the results of the mixture with.
In the recovery, the average age at first reproduction for daphnids in the F1–B2R and F1–B5R generations for DCF, CBZ and their mixture are significantly different from the control group, but no obvious difference was observed between the second brood (F1–B2R) and the fifth brood (F1–B5R) (Fig. 1B). This implies that the daphnids could not recover from the delay in reproduction following exposure to DCF, CBZ and their mixture. The delay was more difficult to recover after exposure to a higher concentration of DCF, CBZ and their mixture. There are limited studies evaluating the recovery effect of pharmaceuticals in multigenerational exposure.
The number of first-brood offspring per female in the F0, F1–B2 and F1–B5 generations for DCF and CBZ treatments were significantly reduced in relation to the control treatment. The mixture also reduced the number of offspring at first brood per female (Fig. 1C). The percentage reductions in the number of offspring at first brood per female for daphnids treated with DCF are 14–23%, 20–23% and 16–24% in the F0, F1–B2 and F1–B5 generations, respectively. The number of offspring at first brood per female in CBZ treatments were reduced by 13–23%, 12–24% and 17–36%, respectively, in the F0, F1–B2 and F1–B5 generations. The number of neonates produced at first reproduction in the mixture was lessened by 16–23%, 27–33% and 32–36% in the F0, F1–B2 and F1–B5 generations, respectively. Although no obvious difference in the number of offspring at first brood per female was observed among different treatments in F0 generation, the mixture induced a stronger inhibitive effect on the F1–B2 and F1–B5 generations than DCF and CBZ alone. The decrease in the number of offspring at first brood per female resulted in a significant reduction in the total number of offspring produced per female during the 21-day exposure for all the treatments and in all the generations as compared to the control (Fig. 1E). Percentage reductions of up to 36, 31 and 42% were obtained for the total number of offspring produced during the 21-day exposure to DCF, CBZ and their mixture, respectively, in all the generations.
These results suggest that exposure to DCF, CBZ and their mixture has significantly reduced the generation of Daphnia magna offspring, both in terms of reproduction time and quantity. In addition, these results mean that both drugs might have acted on cell division, inhibiting the reproductive ability of the daphnids (Canton, 1976) and increased the number of aborted eggs, which, in turn, is likely to be associated with mitosis inhibition in the brood pouch during egg division (Ribeiro et al., 2011). The results obtained herein for CBZ are consistent with previous studies. For instance, the overall number of offspring produced per female throughout a 21-day exposure showed that all the treatments of CBZ ( 10, 100 and 200 μg/L) induced a significant decline in the reproduction rate, with a 55% reduction in offspring production at the highest concentration in comparison with the control (Oropesa et al., 2016).
Conversely, results obtained for DCF are not consistent with previous studies. For example, daphnids that were exposed to 5, 50, 500 and 5000 μg/L DCF for 21 days showed no significant decrease in the number of offspring produced at first reproduction as well as the total number of offspring produced during the entire exposure (Liu et al., 2017).
Similar effects obtained for Daphnia magna herein may happen to wild daphnia populations in the aquatic environment and may have a ripple effect on populations of other aquatic organisms. Comparing the effects of the individual and mixture treatments, CBZ and their mixture had a relatively stronger effect on reproduction than DCF, especially at the higher concentration treatments in all the generations. Regarding different generations, the toxic effect on reproduction obtained in this study was higher on offspring in the F1–B5 generation, followed by the F1–B2 generation with the F0 generation having the least effect.
Results for the number of first brood and the total number of offspring produced per female during the 21-day recovery in the F1 generations (F1–B2R and F1–B5R) are shown in Fig. 1D and 1F. Reproduction rates in the F1–B2R and F1–B5R generations were significantly reduced by 23–31%, 18–32% and 29–36% in the DCF, CBZ and mixture treatments, respectively. Although different treatments did not produce obvious differences for the number of first brood per female in both F1–B2R and F1–B5R, the overall number of offspring produced per female throughout the 21-day recovery felled even more when exposed to the mixture and higher concentrations of DCF and CBZ alone. These observations point to the fact that Daphnia magna could not recover from the reproductive effects induced as a result of exposure to DCF, CBZ and their mixture.
Growth and development
With regards to the body length of Daphnia magna, no significant differences between the treatment and control groups were seen in the F0 generation. In contrast, a significant reduction in the body length was found among the treatment in the F1 generation (F1–B2 and F1–B5) as presented in Fig. 2A. In the F1 generation (F1–B2 and F1–B5), the body length of daphnids was significantly reduced by a maximum of 18, 17 and 20% in the DCF, CBZ and mixture treatments, respectively. The reduction in the body length of Daphnia magna might be associated with the decline in the intake of food. We observed in our previous studies that the filtration and ingestion rates of Daphnia magna were significantly decreased following exposure to DCF and CBZ at different concentrations (5, 15, 50 and 100 μg/L). The percentage decrease in the filtration and ingestion rates were between 16–61% and 9.5–49% for DCF, 16–78% and 11–70% for CBZ, respectively (Nkoom et al., 2019b, 2019a). This indicates that due to the dramatic decrease in the rate of filtration and ingestion, the material required for Daphnia magna’s growth was inadequate, resulting in a reduction in the length of the individual body. The body length of Daphnia magna may also have a significant impact on reproduction. Previous researchers have shown that as the length of Daphnia magna decreases, the size of the brood chambers is reduced, leading to a decline in the total number of eggs (Leblanc and Mclachlan, 1999). These explanations may partly account for the significant reduction in the number of offspring and the total number of offspring per female observed in this study.
(A) Body length, (C) intrinsic growth rate and (E) moulting frequency of daphnia after 21-day exposure to DCF, CBZ and their mixture; (B) body length, (D) intrinsic growth rate and (F) moulting frequency of the daphnids after the transition to a clean medium for 21-day recovery. Data are delineated as mean ± standard deviation (n = 10). Significant differences (p < 0.05) between treatments from an exposure scenario are indicated by different letters
In a study conducted earlier, the authors observed similar results in the body length of Ceriodaphnia dubia in the F0 generation following treatment with CBZ as compared to the control (Lamichhane et al., 2013). All the same, Lürling et al. (2006) found a significant 10 and 32% decrease in the body length of D. pulex throughout a 21-day exposure to 100 and 200 μg/L of CBZ, respectively. Researchers in another study observed a significant decrease in the body length of Daphnia magna during a 21-day exposure to 1–5000 μg/L CBZ (Tian et al., 2019). Liu et al. (2017) observed no significant differences in the body length among the treatment and control groups when Daphnia magna was exposed to 5–5000 μg/L DCF for 21 days consistent with results obtained for the F0 generation herein.
In the recovery, the body lengths of daphnids previously exposed to DCF, CBZ and their mixture were not significantly different from the control in the F1 generations (F1–B2R and F1–B5R) as shown in Fig. 2B. This means that after keeping them in a clean medium for 21 days, Daphnia magna recovered from the significant decline in the body length observed in the filial generations. Body length might not be a sensitive indicator as fecundity to be used in assessing the chronic toxicity of these drugs based on the results obtained for body length herein.
Various researchers have reported that intrinsic growth rate is a dependable and thorough means of evaluating the long-term toxicity of contaminants. The intrinsic growth rate is heavily impacted by the magnitude of both reproduction and mortality throughout the commencing and multiple broods for organisms like Daphnia magna, which undergo numerous overlapping generations. The intrinsic growth rate is, therefore, most immediately linked to size, continuity and breakdown of a population (Borgatta et al., 2015). Results for the intrinsic growth rate of Daphnia magna after exposure to DCF, CBZ and their mixture in the F0 and F1 generations are shown in Fig. 2C. The intrinsic growth rates were significantly reduced up to 11, 12 and 15% in Daphnia magna treated with DCF, CBZ and their mixture, respectively, in all the generations. Although differences among different treatments were not obvious, the intrinsic growth rate clearly dropped in the DCF, CBZ and their mixture treatments as compared to the control. This point to the fact that the survival and reproduction of the population of Daphnia magna may be compromised by the environmentally relevant concentrations of these drugs. Invertebrates function as a central link in the aquatic environment among primary producers and higher organisms (such as freshwater shrimp and fish) that are undoubtedly essential for aquatic food webs (Minguez et al., 2015). As exposure to DCF, CBZ and their mixture has resulted in damage to aquatic invertebrate populations. Responses would, therefore, be initiated at various ecosystem trophic levels. Hence, predictive growth of population ought to be regarded as part of the ecological risk assessment to prevent irreversible population or species extinction.
Similarly, the intrinsic growth rate of Daphnia magna decreased (6 to 17%) as compared to the control after 21-day exposure to CBZ in a previous study (Tian et al., 2019). On the contrary, a previous study found no significant difference in the intrinsic growth rate when daphnids exposed to DCF for 21 days were compared to the control (Liu et al., 2017). With increasing exposure levels of other toxicants such as tetracycline and bisphenol F, the intrinsic growth rates of Daphnia magna were clearly reduced in multigenerational studies (Kim et al., 2012; Liu et al., 2020), which are similar to the results obtained herein.
In the recovery, the intrinsic growth rates were significantly reduced up to 11, 12 and 13% in Daphnia magna previously treated with DCF, CBZ and their mixture, respectively, in the two generations, as shown in Fig. 2D. This means that Daphnia magna could not recover from these effects as a result of exposure to these drugs as well as their mixture.
The results for the frequency at which Daphnia magna moulted during the exposure and the recovery are presented in Fig. 2E and F. There were no significant differences in the moulting frequency for daphnids treated with DCF in all the generations as compared to the control during the entire exposure and after the 21-day recovery. Likewise, previous researchers did not find any significant difference in the moulting frequency in daphnids treated with DCF and the control (Liu et al., 2017). In addition, there were no significant differences in the moulting frequency for daphnids treated with CBZ and their mixture in the F0 generation in relation to the control during the exposure. This outcome is in line with a study conducted earlier in which Daphnia magna that were exposed to 10 and 100 μg/L CBZ for 21 days did not exhibit any significant difference in the moulting frequency (Oropesa et al., 2016).
Contrastingly, exposure to CBZ and their mixture significantly reduced the frequency of moulting for the daphnids in the F1 generation relative to the control after the 21-day exposure. The percentage reductions in moulting frequency by CBZ in the F1–B2 and F1–B5 generations were up to 17 and 20%, respectively. In the mixture treatment, 13–21% and 18–23% were the range obtained for percentage reductions in moulting frequency in the F1–B2 and F1–B5 generations, respectively. Since no significant effects in the moulting frequency were observed in daphnids treated with DCF, the significant reduction observed in the mixture may be attributed to the presence of CBZ. As observed above, the number of offspring produced per female were reduced by exposure to CBZ. The negative effects of this drug on both the rate of reproduction and the frequency of moulting for each daphnid obtained herein may reveal that CBZ has an impact on the ecdysteroid endocrine system in this crustacean. The ecdysteroid endocrine system is responsible for controlling the process of moulting in Daphnia magna. According to Sumiya et al. (2014), whenever these two physiological processes simultaneously occur in an organism, it could imply that the same hormonal system regulates them. This association between the reproductive output and the moulting frequency was observed in a previous study when daphnids were exposed to 200 μg/L CBZ for 21 days. The authors inferred that CBZ could be a potential endocrine disruptor (Oropesa et al., 2016). Similarly, previous researchers have reported the association of moulting frequency with the reproductive cycle in crustaceans, including shrimps, isopods, amphipods, sand crabs and prawns (Gunamalai et al., 2004; Shyama, 1987).
In addition, after the 21-day recovery, daphnids previously treated with CBZ and their mixture reduced moulting frequency by a maximum of 17 and 16% in the F1–B2R and F1–B5R generations, respectively. This implies that Daphnia magna could not recover from the significant decrease in moulting frequency observed in the F1–B2 and F1–B5 subsequent to keeping them in a media without drugs for 21 days.
Heart rate, thoracic limb activity and swimming behaviour
Behaviour has important implications for individual fitness parameters and biotic interactions. Therefore, organisms do not remain motionless in one setting but switch to improve opportunities for foraging and coupling or escape predators and severe abiotic conditions. A major objective in behavioural ecology is to understand how behaviour affects fitness parameters and ecological interactions as well as to determine the degree to which behavioural responses are used to cope with daily threats. In addition, durability in behaviour is often extremely important for individual performance and fitness in a continuously variable environment (Gabriel et al., 2005).
The heart rate and thoracic limb activity of Daphnia magna have often been utilised as physiological indicators that respond to biologically active compounds since both play a vital role in respiration, feeding and metabolism (Smirnov, 2016). Results obtained for the heart rate show a significant decrease. At the same time, that of the thoracic limb activity was significantly increased after exposure to DCF, CBZ and their mixture in the F0 and F1 generations, as shown in Fig. 3. The heart rate was significantly reduced up to 21.4, 24.1 and 22.5% in Daphnia magna treated with DCF, CBZ and their mixture, respectively, in all the generations. The thoracic limb activity was significantly induced up to 25, 28 and 30% in Daphnia magna treated with DCF, CBZ and their mixture, respectively, in all the generations. The increase in the thoracic limbs beating rate observed herein may be attributed to the reduction in the capacity of daphnids to feed (Lari et al., 2017). The enhancement in the rate at which the thoracic limbs thumped is indeed a self-defence strategy for Daphnia magna to the presence of stress in the environment, which may facilitate Daphnia magna in order to fulfil the aims of accelerating the intake of food. On the other hand, the significant decrease in the heart rate may mean that daphnids could not feed well due to exposure to DCF, CBZ and their mixture as a decrease in feeding capability will further reduce metabolism and heart rate (Lari et al., 2017). Also, the significant decrease in the heart rate suggests that daphnids were stressed as a result of exposure to these pharmaceuticals since heart rate is a direct indicator of stress level in Daphnia magna (Smirnov, 2013). The significant increase in the thoracic limb activity, as well as the decrease in the heart rate observed herein, is consistent with those obtained in previous studies when Daphnia magna was exposed to bisphenol F and oil sands process-affected water (Lari et al., 2017; Liu et al., 2020).
(A) Heart rate and (C) thoracic limb activity of daphnia after 21-day exposure to DCF, CBZ and their mixture; (B) heart rate and (D) thoracic limb activity of the daphnids after the transition to a clean medium for 21-day recovery. Data are delineated as mean ± standard deviation (n = 3). Significant differences (p < 0.05) between treatments from an exposure scenario are indicated by different letters
After the 21-day recovery, the heart rate was significantly reduced up to 17, 19 and 18%. At the same time, the thoracic limb activity was increased by 19, 21 and 20% in Daphnia magna previously treated with DCF, CBZ and their mixture, respectively, in the two generations, as shown in Fig. 3B and D. This means that Daphnia magna could not recover from these effects following exposure to these drugs as well as their mixture.
The swimming behaviour of Daphnia magna is complex, involves multiple parameters and is regarded as one of the highly sensitive biological markers used in assessing the toxicity of contaminants (Duquesne and Küster, 2010). It can be defined by many variables that represent changes induced on sensitive systems (nervous and endocrine) by different compounds. Distance moved by daphnids calculated repeatedly or continuously over time can be a valuable swimming parameter that indicates locomotor activity. The trajectory of swimming is a path left by a moving organism and can be defined by length and shape (Bownik, 2017). Previous researchers have used swimming velocity and accelerated velocity to assess the toxicity of environmental contaminants such as bisphenol F and benzoylecgonine when exposed to Daphnia magna and reported a decreasing trend for both parameters (Liu et al., 2020; Parolini et al., 2018).
Herein, swimming behaviours, such as swimming activity and trajectories, were utilised to determine the toxic impacts of DCF, CBZ and their mixture. As shown in Fig. 4A and C, swimming and accelerated velocities were significantly decreased as a result of exposure to DCF, CBZ and their mixture in the F0 and F1 generations. In addition, higher exposure concentrations of both drugs alone and in combination induced a stronger effect on swimming activities, although the differences were not significant in most cases.
(A) Velocity and (C) accelerated velocity of daphnia after 21-day exposure to DCF, CBZ and their mixture; (B) velocity and (D) accelerated velocity of the daphnids after the transition to a clean medium for 21-day recovery. Data are delineated as mean ± standard deviation (n = 3). Significant differences (p < 0.05) between treatments from an exposure scenario are indicated by different letters
After the 21-day recovery, the swimming and accelerated velocities were significantly reduced in Daphnia magna previously treated with DCF, CBZ and their mixture, respectively, in the two generations, as shown in Fig. 4B and D. This means that Daphnia magna could not recover from these effects following exposure to these drugs as well as their mixture.
The trajectories of motion and cumulative distance moved by the daphnids after the 21-day exposure to DCF, CBZ and their mixture are presented in Fig. 5. Daphnia magna exposed to DCF, CBZ and their mixture in the F0 and F1 generations showed fewer trajectories and decreased scope of activities. Daphnia magna’s trajectories of motion and cumulative distance were steadily decreased in all the generations. The percentage reductions in the cumulative distance moved by the daphnids treated with DCF were up to 16, 29 and 33% in the F0, F1–B2 and F1–B5 generations. Daphnia magna treated with CBZ had their cumulative distance moved reduced by 12–18%, 24–30% and 29–36%, respectively, in the F0, F1–B2 and F1–B5 generations. In the mixture, the cumulative distance moved by daphnids were reduced by 15–21%, 26–30% and 31–36% in the F0, F1–B2 and F1–B5 generations. The reductions in the cumulative distance moved by the daphnids that were exposed to DCF, CBZ and their mixture were greater in the F1–B5 generation, followed by the F1–B2 generation, while the F0 generation recorded the least reduction. Comparing the effects of the individual and mixture treatments, CBZ and the mixture had a relatively stronger effect on the trajectories of movement and total distance covered by the exposed daphnids than DCF, especially at the higher concentration treatments in all the generations. Because Daphnia magna’s locomotion is based on a constant, high-energy consuming, muscular operation, the decrease in swimming activity under the exposure to DCF, CBZ and their mixture may be attributable to the organism’s high-energy demand to support critical physiological processes to overcome the toxicity of the drugs. Thus, as swimming activity arises from the incorporation of physiological, sensory, muscular and nervous systems (Charoy et al., 1995), these findings may suggest a general deterioration of the health status in Daphnia magna after exposure to these drugs and may lead to adverse effects on the organism’s fitness and survival.
The reduced swimming output observed herein could adversely affect the filtering operation and, subsequently, the food intake of the treated individuals, which could result in a significant decrease in growth and reproduction, as observed earlier under the “Reproductive toxicity parameters” and “Growth and development” sections in this study. Furthermore, variations in locomotive activity can alter predator–prey relationships, resulting in possible irregularities in trophic interactions between phyto- and zooplankton, and even between zooplankton and fish (Uttieri et al., 2014).
Reproduction, growth and development-related genes
It has been well established that vitellogenin (Vtg) is a four-subunit lipoprotein and the precursor of egg yolk protein that supplies nourishments for the upgrowth of embryos in oviparous organisms. It is required for endocrine control, especially reproduction and development. Vtg gene products in Daphnia magna provide developing embryos with nutrients, such as storage proteins (Jeong et al., 2013). On the other hand, cytochrome P450s (cyps) are a generally distributed and wide superfamily of heme proteins encoded by receptor-dependent transcriptional activation genes and are a class of proteins that react to the toxic chemicals’ hazardous effects (Snyder, 2000). The cyp314 gene is a gene involved in principal moulting hormone biosynthesis, subcategorised into mitochondrial cyps and is essential for transforming ecdysone into its active form (Le et al., 2010). It is, therefore, predicted that changes in reproduction and growth could be addressed partially through the patterns of Vtg and cyp314 gene expressions.
Vtg and cyp314 genes were significantly downregulated following exposure to DCF, CBZ and their mixture in the F0 and F1 generations, and higher concentrations induced more downregulation in most cases, as shown in Fig. 6A and C. The percentage reductions in the Vtg mRNA expression by the daphnids treated with DCF are 38–68%, 28–50% and 39–42% in the F0, F1–B2 and F1–B5 generations. Daphnia magna treated with CBZ reduced the Vtg mRNA expression by 40–60%, 35–39% and 45–48%, respectively, in the F0, F1–B2 and F1–B5 generations. In the mixture, the Vtg mRNA expressions of the daphnids were reduced by 50–59%, 40–42% and 52–57% in the F0, F1–B2 and F1–B5 generations, respectively. No obvious differences were observed in the Vtg mRNA expressions of daphnids in different treatments (DCF, CBZ and their mixture) as well as in the different generations (F0, F1–B2 and F1–B5).
(A) Vtg and (C) cyp314 gene expressions of daphnia after 21-day exposure to DCF, CBZ and their mixture; (B) Vtg and (D) cyp314 gene expressions of the daphnids after the transition to a clean medium for 21-day recovery. Data are delineated as mean ± standard deviation (n = 3). Significant differences (p < 0.05) between treatments from an exposure scenario are indicated by different letters
The cyp314 mRNA expressions were significantly reduced up to 45, 58 and 60% in Daphnia magna treated with DCF, CBZ and their mixture, respectively, in all the generations. Comparing the effects of the individual and the mixture, CBZ and the mixture had a relatively greater effect on the cyp314 mRNA expression than DCF, especially at the higher concentration treatments in all the generations. The inhibition in the cyp314 mRNA expression in daphnids that were exposed to DCF, CBZ and their mixture was relatively greater in the F1–B5 generation, followed by the F1–B2 generation, while the F0 generation had the least reduction.
The significant inhibition in the mRNA expressions of Vtg and cyp314 obtained herein are consistent with changes at the physiological level such as longer days to first reproduction, fewer number of first brood and fewer total number of offspring produced per female obtained herein at the “Reproductive toxicity parameters” section. In earlier investigations, the expression of Vtg mRNA was utilised to determine the impact of toxic contaminants on Daphnia magna reproductive system (Jeong et al., 2013; Liu et al., 2017). Also, the cyp314 gene as a biomarker was utilised by previous researchers to determine the impact of toxic compounds in Daphnia magna (Blewett et al., 2017; Liu et al., 2017; Y. Liu et al., 2019a, b).
The results obtained herein for cyp314 gene expression after exposure to DCF are not consistent with those obtained at the physiological level, such as the moulting frequency. This discrepancy may be attributed to the transient and dynamic nature of gene expression in comparison with the physiological changes (Liu et al., 2017). Previous researchers also observed a contradiction between gene upregulation (cyp314) and undifferentiated moulting frequency performances in Daphnia magna after exposure to DCF (Liu et al., 2017).
After the 21-day recovery, Vtg and cyp314 genes were significantly downregulated in Daphnia magna previously treated with DCF, CBZ and their mixture, respectively, in the two generations, as shown in Fig. 6B and D. Although the differences between treatments, the control and among different treatments became smaller, the downregulation of Vtg and cyp314 genes seems irreversible following exposure to these drugs as well as their mixture.
Antioxidant-related genes
Reactive oxygen species (ROS) are formed when a number of toxic chemicals are introduced to organisms, and ROS play a major role in the occurrence of oxidative stress. Accumulation of ROS in cells could damage macromolecules, like proteins, nucleic acids and lipids. An antioxidant defence mechanism has been formed by organisms to prevent oxidative damage from toxic substances and preserve the body’s homeostasis. Enzymes and non-enzymatic antioxidant scavengers are essential parts of the antioxidant protection complex, and it is predicted that genes associated with this mechanism would respond to toxic substance exposure (S. Liu et al., 2019a, 2019b).
The results for the mRNA expressions of sod, cat and gst are displayed in Fig. 7. The sod, cat and gst transcriptional level exhibited a significant downregulation in daphnids exposed to DCF, CBZ and their mixture in the F0 and F1 generations with an exception of gst in the F0 generation at lower concentration of DCF, as shown in Fig. 7 A, C and E. The percentage reductions in the sod mRNA expression by the daphnids treated with DCF are 30–35%, 27–39% and 33–40%, respectively, in the F0, F1–B2 and F1–B5 generations. Daphnia magna treated with CBZ reduced the sod mRNA expression by 29–37%, 37–42% and 36–47%, respectively, in the F0, F1–B2 and F1–B5 generations. In the mixture, the sod mRNA expressions of the daphnids were reduced by 29–38%, 30–46% and 35–49% in the F0, F1–B2 and F1–B5 generations, respectively. The cat mRNA expressions were significantly reduced up to 50, 58 and 49% in Daphnia magna treated with DCF, CBZ and their mixture, respectively, in all the generations. Also, the gst mRNA expressions were significantly reduced up to 40, 44 and 47% in Daphnia magna treated with DCF, CBZ and their mixture, respectively, in all the generations.
(A) sod, (C) cat and (E) gst gene expressions of daphnia after 21-day exposure to DCF, CBZ and their mixture; (B) sod, (D) cat and (F) gst gene expressions of the daphnids after the transition to a clean medium for 21-day recovery. Data are delineated as mean ± standard deviation (n = 3). Significant differences (p < 0.05) between treatments from an exposure scenario are indicated by different letters
Taken together, detoxification systems can be turned on by increasing levels of toxic substances such as DCF and CBZ; however, if toxins and ROS surpass an organism’s detoxification capability, cellular stress and damage may occur and the physiological metabolism may alter afterwards (Winston and Di Giulio, 1991). The decrease in mRNA levels of sod, cat and gst obtained herein could be described as a sign of overpowered antioxidant capability induced by these pharmaceuticals. In addition, the reduction in sod, cat and gst mRNA expression levels may be associated with increased ROS production. As is known, the overproduction of ROS under severe oxidative stress can exceed the antioxidant ability and lead to reduced activity of the antioxidant defence system (Aksakal, 2020).
However, after the 21-day recovery, the sod, cat and gst mRNA expressions were not significantly different from those obtained in the control in Daphnia magna previously treated with DCF, CBZ and their mixture, respectively, in the two generations, as shown in Fig. 7 B, D and E. This means that Daphnia magna recovered from the oxidative stress following exposure to the tested pharmaceuticals as well as their mixture.
In this study, more often than not, the chronic toxic effects of CBZ on daphnids were profoundly higher than that of DCF, especially at the high concentration (10 μg/L) treatments. This reflected in the mixture, and so toxic effects to daphnids in the mixture treatments were similar to CBZ alone treatments but significantly higher than DCF alone treatments. The bioaccumulation potentials of these drugs in Daphnia magna could partly explain this observation. Because the more a compound accumulates in an organism, the better its chances of eliciting toxic effects to that organism. In our previous studies, it was observed that CBZ accumulated much more with a BCF of 202.56 L/kg than DCF, which had a BCF of 70.94 L/kg in Daphnia magna following exposure to the same concentration of 5 μg/L (Nkoom et al., 2019a, 2019b). This may partly account for the difference in the toxic effects obtained for DCF and CBZ herein.
Also, the general trends in the toxic effects observed for all the measured parameters in this study were not acclimation but enhanced effects in later generations or broods. This implies that the responses of the F1 generation were stronger than those in the F0 generation. Herein, Daphnia magna in the F0 generation were born in a fresh medium without the drugs, while Daphnia magna in the F1 generation were birthed in an exposure media containing DCF, CBZ and their mixture. An instant early encounter with the drugs was thus obtained by the F1 neonates. According to Liu et al. (2018), Daphnia magna neonates are, in most cases, vulnerable during or soon after birth to toxic exposure. It may therefore be that during this extremely precarious developmental period, the initial interaction with DCF, CBZ and their mixture may have resulted in increased sensitivity along with a decline in fitness.
In addition, it is possible that some invertebrates in the aquatic environment are unable to establish tolerance over generations to permanent exposure of harmful chemicals, potentially due to high-energy costs. Reproduction, development and maintenance or ability to survive constitute conflicting needs for an organism’s limited supply of resources. The theory of the allocation of energy states that if an organism apportions energy to one function, for instance, reproduction, the quantity of energy in stock for other functions, like maintenance, is decreased (Cody, 1966). In accordance with this principle, previous researchers theorised that the evolution of tolerance to toxins could be constrained by high fitness costs (Xie and Klerks, 2004). The recurrence of drug impacts found in this study in later generations for all life history, morphological, behavioural and gene expression parameters may therefore suggest that over the two generations, the daphnids were unable to sustain expensive drug resistance. No acclimation but enhanced effects of pollutants in later generations have also been documented in earlier multigenerational investigations. For example, in the second generation, male amphipods (Hyalella azteca) treated with 0.1 and 0.32 μg/L EE2 produced significantly smaller second gnathopods than in the generation of the parent, and a slightly higher incidence of females was observed in subsequent generations (Vandenbergh et al., 2003). Additionally, Brennan et al. (2006) found that in the second generation of Daphnia magna, 200–500 μg/L of oestrogen diethylstilbestrol decreased the number of offspring, while no effects were noticed in the generation of the parent. The authors of the above studies explained that the first-generation offspring were weakened, resulting in greater vulnerability in the second generation to the toxic effects of estrogenic compounds as obtained herein.
Relationships between the various parameters
Analyses of the correlation between the various parameters evaluated are presented in Table S2. The heart rate of Daphnia magna had significant positive correlations with the distance covered, swimming velocity and accelerated velocity and significant negative correlation with thoracic limb activity during the 21-day exposure. The expression of Vtg mRNA was significantly correlated positively with moulting frequency of Daphnia magna during the 21-day exposure. The cyp314 mRNA expression of Daphnia magna had positive significant correlations with the heart rate, distance covered, swimming velocity and accelerated velocity and significant negative correlation with thoracic limb activity during the 21-day exposure. These positive significant correlations may mean that these effects were caused by a similar mode of action. The antioxidant-related (sod, cat, and gst) mRNA expressions of Daphnia magna were significantly positively correlated with the heart rate, cyp314, distance covered, swimming velocity and accelerated velocity and significantly negatively correlated with thoracic limb activity during the 21-day exposure. The significant positive correlations of the antioxidant-related genes obtained herein are consistent with what was obtained for the antioxidant enzyme activities (SOD, CAT and GST) in previous studies when Daphnia magna was exposed to bisphenol F (Liu et al., 2020).
The body length of Daphnia magna had significant positive correlations with sod and cat mRNA expressions, while the intrinsic growth rate significantly positively correlated with the total number of offspring per female during the 21-day exposure. These results affirmed the fact that the use of physiological, behavioural and gene transcription parameters to surmise the potential mechanisms of action in order to elucidate the looming ecological hazard of emerging pollutants is reliable. The heart rate may serve as a more sensitive ecological predictor due to its significant positive correlations with most of the measured behavioural parameters (distance covered, swimming velocity and accelerated velocity) and mRNA expressions (sod, cat, gst and cyp314) obtained herein as well as in a previous study (Liu et al., 2020).
Conclusions
This study juxtaposes the chronic toxicological effects of DCF, CBZ and their mixture to Daphnia magna over two generations. Physiological changes (survival, growth rate, development and reproduction), behavioural changes (heart rate, swimming and thoracic limb activities) and the expression of five selected genes (Vtg, cyp314, sod, cat and gst) were measured. Exposure to DCF, CBZ and their mixture induced chronic toxic effects in Daphnia magna and may present possible risks in the environment due to the induction of significant growth and reproduction inhibitions in the parental as well as the first filial generation. Besides, both drugs affected swimming behaviour, downregulated the expression of reproductive and antioxidant-related genes and disrupted Daphnia magna’s heartbeat and thoracic limb activity. These effects were relatively higher in the first filial generation suggesting that the sensitivity of Daphnia magna increased while their fitness decreased over the two generations, which is evidence of higher energy need for maintenance. CBZ and the mixture induced stronger effects for most endpoints measured in this study. Offspring of the first filial generation could not recover from most of these significant chronic toxicological effects following exposure to these drugs when kept in a clean medium for 21 days. The outcomes reported in this study highlight the need to consider maternal effects in environmental risk assessment procedures, as the influence of pollutants during single-generation exposure may be underestimated.
Data Availability
All data generated or analysed during this study are included in this published article and its supplementary information files.
References
Aksakal, F.I., 2020. Evaluation of boscalid toxicity on Daphnia magna by using antioxidant enzyme activities, the expression of genes related to antioxidant and detoxification systems, and life-history parameters. Comp. Biochem. Physiol. Part C Toxicol. Pharmacol. 237, 108830.
Blewett TA, Delompré PLM, He Y, Folkerts EJ, Flynn SL, Alessi DS, Goss GG (2017) Sublethal and reproductive effects of acute and chronic exposure to flowback and produced water from hydraulic fracturing on the water flea Daphnia magna. Environ Sci Technol 51:3032–3039
Borgatta M, Decosterd L-A, Waridel P, Buclin T, Chèvre N (2015) The anticancer drug metabolites endoxifen and 4-hydroxy-tamoxifen induce toxic effects on Daphnia pulex in a two-generation study. Sci Total Environ 520:232–240
Bownik A (2017) Daphnia swimming behaviour as a biomarker in toxicity assessment: a review. Sci Total Environ 601:194–205
Brennan SJ, Brougham CA, Roche JJ, Fogarty AM (2006) Multi-generational effects of four selected environmental oestrogens on Daphnia magna. Chemosphere 64:49–55
Canton JH (1976) The toxicity of benomyl, thiophanate-methyl, and BCM to four freshwater organisms. Bull Environ Contam Toxicol 16:214–218
Charoy CP, Janssen CR, Persoone G, Clément P (1995) The swimming behaviour of Brachionus calyciflorus (rotifer) under toxic stress. I. The use of automated trajectometry for determining sublethal effects of chemicals. Aquat Toxicol 32:271–282. https://doi.org/10.1016/0166-445X(94)00098-B
Cleuvers M (2003) Aquatic ecotoxicity of pharmaceuticals including the assessment of combination effects. Toxicol Lett 142:185–194
Cody, M.L., 1966. A general theory of clutch size. Evolution (N. Y). 20, 174–184.
Dietrich S, Ploessl F, Bracher F, Laforsch C (2010) Single and combined toxicity of pharmaceuticals at environmentally relevant concentrations in Daphnia magna–A multigenerational study. Chemosphere 79:60–66
Duquesne S, Küster E (2010) Biochemical, metabolic, and behavioural responses and recovery of Daphnia magna after exposure to an organophosphate. Ecotoxicol Environ Saf 73:353–359. https://doi.org/10.1016/j.ecoenv.2009.11.008
Gabriel W, Luttbeg B, Sih A, Tollrian R (2005) Environmental tolerance, heterogeneity, and the evolution of reversible plastic responses. Am Nat 166:339–353. https://doi.org/10.1086/432558
Gunamalai V, Kirubagaran R, Subramoniam T (2004) Hormonal coordination of molting and female reproduction by ecdysteroids in the mole crab Emerita asiatica (Milne Edwards). Gen Comp Endocrinol 138:128–138
Harris, K.D.M., Bartlett, N.J., Lloyd, V.K., 2012. Daphnia as an emerging epigenetic model organism. Genet. Res. Int. 2012.
Jeong SW, Lee SM, Yum SS, Iguchi T, Seo YR (2013) Genomic expression responses toward bisphenol-A toxicity in Daphnia magna in terms of reproductive activity. Mol Cell Toxicol 9:149–158
Jux U, Baginski RM, Arnold H-G, Krönke M, Seng PN (2002) Detection of pharmaceutical contaminations of river, pond, and tap water from Cologne (Germany) and surroundings. Int J Hyg Environ Health 205:393–398. https://doi.org/10.1078/1438-4639-00166
Kim HY, Lee MJ, Yu SH, Kim SD (2012) The individual and population effects of tetracycline on Daphnia magna in multigenerational exposure. Ecotoxicology 21:993–1002
Kim HY, Yu S, Jeong T, Kim SD (2014) Relationship between trans-generational effects of tetracycline on Daphnia magna at the physiological and whole organism level. Environ Pollut 191:111–118
Lamichhane K, Garcia SN, Huggett DB, DeAngelis DL, La Point TW (2013) Chronic effects of carbamazepine on life-history strategies of Ceriodaphnia dubia in three successive generations. Arch Environ Contam Toxicol 64:427–438
LaMontagne JM, McCauley E (2001) Maternal effects in Daphnia: what mothers are telling their offspring and do they listen? Ecol Lett 4:64–71
Lari E, Steinkey D, Morandi G, Rasmussen JB, Giesy JP, Pyle GG (2017) Oil sands process-affected water impairs feeding by Daphnia magna. Chemosphere 175:465–472. https://doi.org/10.1016/j.chemosphere.2017.02.088
Le T-H, Lim E-S, Lee SK, Choi Y-W, Kim Y-H, Min J (2010) Effects of glyphosate and methidathion on the expression of the Dhb, Vtg, Arnt, CYP4 and CYP314 in Daphnia magna. Chemosphere 79:67–71
Leblanc GA, Mclachlan JB (1999) Molt-independent growth inhibition of Daphnia magna by a vertebrate antiandrogen. Environ Toxicol Chem 18:1450–1455. https://doi.org/10.1002/etc.5620180715
Liu J-L, Wong M-H (2013) Pharmaceuticals and personal care products (PPCPs): a review on environmental contamination in China. Environ Int 59:208–224. https://doi.org/10.1016/j.envint.2013.06.012
Liu, J., Shen, J., Lu, G., Xu, X., Yang, H., Yan, Z., Chen, W., 2020. Multilevel ecotoxicity assessment of environmentally relevant bisphenol F concentrations in Daphnia magna. Chemosphere 240, 124917.
Liu S, Ding R, Nie X (2019a) Assessment of oxidative stress of paracetamol to Daphnia magna via determination of Nrf1 and genes related to antioxidant system. Aquat Toxicol 211:73–80. https://doi.org/10.1016/j.aquatox.2019.03.014
Liu, Y., Ding, R., Pan, B., Wang, L., Liu, S., Nie, X., 2019b. Simvastatin affect the expression of detoxification-related genes and enzymes in Daphnia magna and alter its life history parameters. Ecotoxicol. Environ. Saf. 182, 109389.
Liu Y, Han W, Xu Z, Fan W, Peng W, Luo S (2018) Comparative toxicity of pristine graphene oxide and its carboxyl, imidazole or polyethylene glycol functionalized products to Daphnia magna: a two generation study. Environ Pollut 237:218–227
Liu Y, Wang L, Pan B, Wang C, Bao S, Nie X (2017) Toxic effects of diclofenac on life history parameters and the expression of detoxification-related genes in Daphnia magna. Aquat Toxicol 183:104–113
Livak KJ, Schmittgen TD (2001) Analysis of relative gene expression data using real-time quantitative PCR and the 2 (-Delta Delta C (T)) method. Methods 25:402–408
Lotka AJ (1913) A natural population norm. J Wash Acad Sci 3:241–248
Lürling M, Sargant E, Roessink I (2006) Life-history consequences for Daphnia pulex exposed to pharmaceutical carbamazepine. Environ Toxicol an Int J 21:172–180
Minguez L, Ballandonne C, Rakotomalala C, Dubreule C, Kientz-Bouchart V, Halm-Lemeille M-P (2015) Transgenerational effects of two antidepressants (sertraline and venlafaxine) on Daphnia magna life history traits. Environ Sci Technol 49:1148–1155
Nkoom M, Lu G, Liu J, Dong H (2020) Biological uptake, depuration and biochemical effects of diclofenac and carbamazepine in Carassius carassius. Ecotoxicol Environ Saf 205:111106. https://doi.org/10.1016/j.ecoenv.2020.111106
Nkoom M, Lu G, Liu J, Dong H, Yang H (2019a) Bioconcentration, behavioral, and biochemical effects of the non-steroidal anti-inflammatory drug diclofenac in Daphnia magna. Environ Sci Pollut Res 26:5704–5712
Nkoom M, Lu G, Liu J, Yang H, Dong H (2019b) Bioconcentration of the antiepileptic drug carbamazepine and its physiological and biochemical effects on Daphnia magna. Ecotoxicol Environ Saf 172:11–18
OECD, 2012. Test No. 211: Daphnia magna reproduction test. https://doi.org/10.1787/9789264185203-en
OECD, 2004. Test No. 202: Daphnia sp. acute immobilisation test. https://doi.org/10.1787/9789264069947-en
Oliveira P, Almeida Â, Calisto V, Esteves VI, Schneider RJ, Wrona FJ, Soares AMVM, Figueira E, Freitas R (2017) Physiological and biochemical alterations induced in the mussel Mytilus galloprovincialis after short and long-term exposure to carbamazepine. Water Res 117:102–114. https://doi.org/10.1016/j.watres.2017.03.052
Oropesa AL, Floro AM, Palma P (2016) Assessment of the effects of the carbamazepine on the endogenous endocrine system of Daphnia magna. Environ Sci Pollut Res 23:17311–17321
Parolini M, De Felice B, Ferrario C, Salgueiro-González N, Castiglioni S, Finizio A, Tremolada P (2018) Benzoylecgonine exposure induced oxidative stress and altered swimming behavior and reproduction in Daphnia magna. Environ Pollut 232:236–244. https://doi.org/10.1016/j.envpol.2017.09.038
Rasheed T, Bilal M, Nabeel F, Adeel M, Iqbal HMN (2019) Environmentally-related contaminants of high concern: potential sources and analytical modalities for detection, quantification, and treatment. Environ Int 122:52–66. https://doi.org/10.1016/j.envint.2018.11.038
Ribeiro F, Ferreira NCG, Ferreira A, Soares AMVM, Loureiro S (2011) Is ultraviolet radiation a synergistic stressor in combined exposures? The case study of Daphnia magna exposure to UV and carbendazim. Aquat Toxicol 102:114–122
Rivetti C, Campos B, Barata C (2016) Low environmental levels of neuro-active pharmaceuticals alter phototactic behaviour and reproduction in Daphnia magna. Aquat Toxicol 170:289–296. https://doi.org/10.1016/j.aquatox.2015.07.019
Scherer C, Seeland A, Oehlmann J, Müller R (2013) Interactive effects of xenobiotic, abiotic and biotic stressors on Daphnia pulex—results from a multiple stressor experiment with a fractional multifactorial design. Aquat Toxicol 138–139:105–115. https://doi.org/10.1016/j.aquatox.2013.04.014
Shyama SK (1987) Studies on moulting and reproduction in the prawn Macrobrachium idella (Heller). Mahasagar 20:15–21
Silva ARR, Cardoso DN, Cruz A, Mendo S, Soares AMVM, Loureiro S (2019) Long-term exposure of Daphnia magna to carbendazim: how it affects toxicity to another chemical or mixture. Environ Sci Pollut Res 26:16289–16302
Smirnov N (2013) Physiology of the Cladocera. Academic Press, San Diego, CA
Smirnov NN (2016) The recent state and prospects of studying the cladocera (Crustacea) physiology. Zool ZHURNAL 95:788–804
Snyder MJ (2000) Cytochrome P450 enzymes in aquatic invertebrates: recent advances and future directions. Aquat Toxicol 48:529–547
Sumiya E, Ogino Y, Miyakawa H, Hiruta C, Toyota K, Miyagawa S, Iguchi T (2014) Roles of ecdysteroids for progression of reproductive cycle in the fresh water crustacean Daphnia magna. Front Zool 11:60
Tian, Y., Xia, X., Wang, Jinhua, Zhu, L., Wang, Jun, Zhang, F., Ahmad, Z., 2019. Chronic toxicological effects of carbamazepine on Daphnia magna Straus: effects on reproduction traits, body length, and intrinsic growth. bull. Environ. Contam. Toxicol. 1–6.
Tkaczyk A, Bownik A, Dudka J, Kowal K, Ślaska B (2021) Daphnia magna model in the toxicity assessment of pharmaceuticals: a review. Sci Total Environ 763:143038. https://doi.org/10.1016/j.scitotenv.2020.143038
Uttieri, M., Sandulli, R., Spezie, G., Zambianchi, E., 2014. From small to large scale: a review of the swimming behaviour of Daphnia. Daphnia Biol. Math. Perspect. Nov. Sci. Publ. New York 309–312.
Valcárcel Y, González Alonso S, Rodríguez-Gil JL, Gil A, Catalá M (2011) Detection of pharmaceutically active compounds in the rivers and tap water of the Madrid Region (Spain) and potential ecotoxicological risk. Chemosphere 84:1336–1348. https://doi.org/10.1016/j.chemosphere.2011.05.014
Vandenbergh GF, Adriaens D, Verslycke T, Janssen CR (2003) Effects of 17α-ethinylestradiol on sexual development of the amphipod Hyalella azteca. Ecotoxicol Environ Saf 54:216–222
Winston GW, Di Giulio RT (1991) Prooxidant and antioxidant mechanisms in aquatic organisms. Aquat Toxicol 19:137–161. https://doi.org/10.1016/0166-445X(91)90033-6
Xie L, Klerks PL (2004) Fitness cost of resistance to cadmium in the least killifish (Heterandria formosa). Environ Toxicol Chem an Int J 23:1499–1503
Yang H, Lu G, Yan Z, Liu J, Dong H (2018) Influence of suspended sediment characteristics on the bioaccumulation and biological effects of citalopram in Daphnia magna. Chemosphere 207:293–302. https://doi.org/10.1016/j.chemosphere.2018.05.091
Zhu X, Wang Q, Zhang L, Liu J, Zhu C, Yang Z (2015) Offspring performance of Daphnia magna after short-term maternal exposure to mixtures of microcystin and ammonia. Environ Sci Pollut Res 22:2800–2807. https://doi.org/10.1007/s11356-014-3520-5
Acknowledgements
This research was funded by the National Natural Science Foundation of China (No. 51879228), the National Science Funds for Creative Research Groups of China (No. 51421006) and the Priority Academic Programme Development of Jiangsu Higher Education Institutions.
Author information
Authors and Affiliations
Contributions
MN: Conceptualisation, methodology, investigation, formal analysis, writing—original draft, writing—review and editing. GL: conceptualisation, methodology, formal analysis, writing—original draft, writing—review and editing, funding acquisition, supervision. JL: methodology, formal analysis, writing—review and editing, resources. All authors read and approved the final manuscript.
Corresponding author
Ethics declarations
Ethics approval
Not applicable.
Consent to participate
Not applicable.
Consent for publication
Not applicable.
Conflict of interest
The authors declare no competing interests.
Additional information
Communicated by Bruno Nunes.
Publisher’s note
Springer Nature remains neutral with regard to jurisdictional claims in published maps and institutional affiliations.
Supplementary Information
Below is the link to the electronic supplementary material.
Rights and permissions
About this article
Cite this article
Nkoom, M., Lu, G. & Liu, J. Chronic toxicity of diclofenac, carbamazepine and their mixture to Daphnia magna: a comparative two-generational study. Environ Sci Pollut Res 29, 58963–58979 (2022). https://doi.org/10.1007/s11356-022-19463-w
Received:
Accepted:
Published:
Issue Date:
DOI: https://doi.org/10.1007/s11356-022-19463-w