Abstract
Aims
Moso bamboo (Phyllostachys pubescens) invasions into native forest cause a series of ecological problems, including the alteration of soil microorganism community composition. Additionally, it has been established that variation changes in leaf litter types can drive changes in soil microorganism communities. We have previously demonstrated distinct differences in nutrient composition between bamboo leaf litter and leaf litter of native plants. Yet to date, we lack a comprehensive understanding of how Moso bamboo leaf litter inputs change soil microorganism community composition in native forests.
Methods
We conducted an in-situ decomposition experiment to examine the response of soil bacterial and fungi phyla to Moso bamboo leaf litter addition in three native forest soils.
Results
Bamboo leaf litter had higher quality (low lignin content and low C:N) and higher decomposition rates compared to native forest plants. Bamboo leaf litter additions did not change the richness and diversity of soil bacterial and fungi in the Broadleaf forest and Chinese fir forest. Additionally, bamboo leaf litter additions only affected the soil microorganisms in the Cryptomeria japonica var. Sinensis forest with low-quality litter; Principal Component Analysis (PCA) further supported this result.
Conclusions
Our results demonstrate that the soil microbial community has resilience to chemical and biotic inputs from foreign leaf litter. This study helps soil ecologists better understand the impacts of Moso bamboo invasions into native forests, including the weak effects of its litter input on soil microorganisms.
Similar content being viewed by others
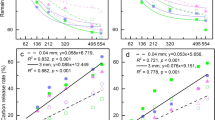
Explore related subjects
Discover the latest articles, news and stories from top researchers in related subjects.Avoid common mistakes on your manuscript.
Introduction
Moso bamboo (Phyllostachys pubescens) is the most widely distributed bamboo species in China, covering an area of ~ 3 million ha (Fu 2001). Moso bamboo is considered a “tree grass,” with large woody stems that can exceed 20 m (Xu et al. 2020). P. pubescens belongs to a group of running bamboo plants that have the capacity for rapid invasion into neighboring forests. Invasions by Moso bamboo trigger a series of ecological problems, including decreasing plant biodiversity (Bai et al. 2016), degradation of soil conservation function (Xu et al. 2020), and altering soil nutrients and microbial communities (Li et al. 2017; Liu et al. 2019).
Microorganisms are one of the most important components of soil, which brings soil to life. For forest ecosystems, soil microorganisms transfer the organic matter into inorganic substances (e.g. nitrogen) and provide nutrients for plants, which are the driving force of biogeochemical nutrient cycling and the bridge linking above-ground and below-ground ecosystem interactions (Chu and Zhu 2019; Fry et al. 2019). Soil microorganisms contribute to major soil respiration processes and are therefore recognized as strong determinants of soil carbon storage and cycling (Guo et al. 2016; Whitaker et al. 2014). Consequently, there has been considerable attention about how soil microorganisms are influenced by plant invasion and vegetation change (Collins et al. 2020; Inderjit and van der Putten 2010). Previous studies showed that there is a significant difference of soil microbial community composition between Moso bamboo forest and invaded forest (e.g., broadleaf forest) (Fang et al. 2022; Liu et al. 2019; Wang et al. 2016). However, we still lack a mechanistic understanding of how this process occurs; decomposition differences associated with leaf litter subsidies from these different forest types may be one of the driving factors affecting the microbial community (Prescott and Zukswert 2016).
Leaf litter quality is a key factor controlling litter decomposition. In general, leaf litter with low concentrations of recalcitrant compounds (e.g., lignin, tannins) represents high-quality litter, but other factors are also important to consider, such as C:N ratios and nutrient concentrations (Bradford et al. 2016). Usually, the litter quality of invasive plants is higher than that of native plants (Lee et al. 2017), leading to faster decay rates compared to native plants (Prescott and Zukswert 2016). Previous studies found that leaf litter lignin content of Moso bamboo was usually lower than that of neighboring native dominant species in subtropical broadleaf and artificial coniferous forests (Song et al. 2016; Tian et al. 2020), suggesting that leaf litter of Moso bamboo is of higher quality than litter from native plants. Therefore, we hypothesized that after Moso bamboo invasion its leaf litter decay would occur faster than litter decay of native plant species (Hypothesis 1).
Findings from a meta-analysis suggested that plant invasion significantly increased the biomass and abundance of soil microorganisms (Zhang et al. 2019). Litter species with different chemical compositions often show distinct quality and decomposability properties, namely species-specific decomposition (Gartner and Cardon 2004; Liu et al. 2020). Litter decomposition provides the energy and food sources for soil microorganisms (Moore et al. 2004); thus, litter species compositional changes should cause variation in soil microorganism abundances and assemblages. The expansion of Moso bamboo to the surrounding forest is a gradual process. New bamboo shoots constantly encroach upon native forests, bringing new bamboo leaf litter in the process. New bamboo leaf litter is relatively high in quality (low lignin) compared to native plants (Song et al. 2016). If Hypothesis 1 is supported, new bamboo leaf litter will bring more nutrients (e.g., nitrogen) via decomposition. Therefore, we hypothesized that new bamboo leaf litter inputs would increase the species richness and abundance of soil microorganisms (i.e., bacteria and fungi) in invaded native forests (Hypothesis 2).
Forest soil provides a good habitat for bacteria and fungi. For most soils, phyla Proteobacteria, Actinobacteria, and Acidobacteria are the most common bacteria (Lladó et al. 2017), and phyla Ascomycota and Basidiomycota are the most common fungi (Li et al. 2022). Soil microorganisms have some preference in terms of carbon sources. For example, phyla Proteobacteria prefer soluble carbon and utilize the easily decomposed compounds in litter (Schroeter et al. 2022), while phyla Basidiomycota tend to use recalcitrant litter compounds (Dong et al. 2021). Generally, high quality litter of invasive plants generate more available C and other resources for soil biota than native plant species (Prescott and Zukswert 2016), which can lead to more abundant decomposers and support a more biodiverse soil community (Zhang et al. 2019). Compared to native plants, high quality, rapidly decomposing Moso bamboo leaf litter will supply a high-quality carbon source to microbes, which vary in their usage of carbon sources, as indicated above. Therefore, we hypothesized that Moso bamboo leaf litter inputs into invaded native forests would favor the growth of bacteria, changing the associated soil microbial community composition (Hypothesis 3).
In the Jinggangshan Nature Reserve in southern China, due to the exclusion of bamboo management, Moso bamboo expansion is more rampant than in other protected areas, and this invasion seriously disturbs the development of native forests (Fang et al. 2022). In this study, we selected three frequently invaded native forests—evergreen broad-leaf forest, Chinese fir (Cunninghamia lanceolata) forest, and Cryptomeria japonica var. sinensis forest—as study areas in the Jinggangshan Nature Reserve in the Jiangxi province. We tested Hypothesis 1 through the comparison of leaf litter decomposition between Moso bamboo and the three native forest types; we tested Hypothesis 2 by investigating bacterial and fungal richness and abundance of three native forest soil types after bamboo leaf litter input; we tested Hypothesis 3 via analysis of the composition of the soil bacterial and fungal assemblages of three native forest soils after bamboo leaf litter inputs.
Materials and methods
Study site
Our study area is located on the Jinggangshan Nature Reserve in Jiangxi province, which is characterized by a subtropical, humid climate with monsoonal activity (26°22’∼26°48’N, 114°05’∼114°23’E). Mean annual temperature and precipitation are 14.2 ºC and 1,865.2 mm, respectively (Deng et al. 2003; Zhu and Shangguan 2009). The climax community in this area is subtropical, evergreen and broad-leaf forest. Because of years of development, much of the subtropical, evergreen and broad-leaf forests have been replaced by bamboo forest, forming a large area of bamboo-broad-leaf mixed forest and pure bamboo forest. Based on data from a secondary forest resources inventory, Moso bamboo composes ~ 20% of total forests on the preserve with an area of 3849.3 hm2, which is increasing by ~ 1–3% yearly (Zhu and Shangguan 2009). Soil types in this area are similar across forest types and are composed of yellowish-red soil (J. Liu, personal observation) made up of soil types with high iron content (e.g., ultisols, alfisols, oxisols).
Experimental design
We collected fresh mixed broad-leaf litter (Machilus thunbergii, Daphniphyllum macropodum, and Alniphyllum fortune, BR), Moso bamboo leaf litter (MO), Chinese fir leaf litter (Cunninghamia lanceolata, CU), and Cryptomeria japonica var. sinensis (CR) leaf litter in evergreen-broad-leaf forest. Fresh litter was oven-dried to constant weight at 60 ℃. We placed 20 g dry litter into litter bags (0.125 mm mesh size, 15 cm × 15 cm) for conducting litter decomposition assays. After removing the surface litter, litter bags were fixed to the soil surface using steel spikes (sensu Hoyos-Santillan et al. 2018). Seven experimental treatments were established (Table 1). MO litter bags were placed in the BR, MO, CU, and CR forest stands, and BR, CU, and CR litter bags were placed in BR, CU, and CR forest stands, respectively.
Sampling design
The experiment started on March 2016 and lasted for 3 years. We collected five litter bags of each treatment for mass loss, assessed at 120, 240, 360, 480, 600, 720, and 840 days of decomposition. The decayed litter was cleaned by rinsing with deionized water and oven-drying to constant weight at 60 ℃ prior to weighing final litter mass. We also collected fresh surface soil (0–10 cm) under litter bags at 120, 480, and 840 days. These soils were preserved at -80 ℃ and subsequently used for high-throughput sequencing analysis.
Litter chemical analyses
Prior to chemical analyses, initial litter from each species was ground into a fine powder using a ball mill (JX-2010, Shanghai, China). C and N concentrations of litter were measured using an elemental analyzer (Flash HT 2000, Thermo Fisher Scientific). The molybdenum antimony method was used to analyze litter P concentrations (Lu 2000). The concentration of lignin was assayed using HPLC (Sluiter et al. 2008). Briefly, 200 mg samples were extracted with ferrous ammonium sulfate hexahydrate and CuO, and then analyzed on a HPLC (Sciex).
DNA extraction and high-throughput sequencing analysis
Soil samples (n = 91) were used for sequencing analysis, including 21 soil samples (three replicates of each treatment) from days 120, 35 soil samples (five replicates of each treatment) from day 480, and 35 soil samples (five replicates of each treatment) from day 840. We used QIAGEN DNeasy PowerSoil kits (Qiagen, Germantown, MD, USA) to extract DNA from soil samples following the manufacturer’s protocol. All DNA extracts were dissolved in 100 ul TE buffer. NanoDrop was used to determine DNA concentrations, and then all the extracts were stored at -80 ℃.
The ITS1F (5’-CTTGGTCATTTAGAGGAAGTAA-3’) (Gardes and Bruns 1993) and ITS2 (5’-GCTGCGTTCTTCATCGATGC-3’) (White et al. 1990) primers were used to amplify fungi for day-120 samples for the ITS1 region, while ITS33-KYO2 (5’-GATGAAGAACGYAGYRAA-3’) (Toju et al. 2012) and ITS4 (5’-TCCTCCGCTTATTGATATGC-3’) (White et al. 1990) primer sets were used to amplify fungi for day-480 and day-840 samples for the ITS2 region. Similarly, the 515F (5’-GTGCCAGCMGCCGCGG-3’) and 907R (5’-CCGTCAATTCMTTTRAGTTT-3’) (Biddle et al. 2008) primers were used to amplify the V4–V5 region of bacteria for day-120 samples, while 341F (5’-CCTAYGGGRBGCASCAG-3’) and 806R (5’-GGACTACNNGGGTATCTAAT-3’) (Callahan et al. 2016) primers were selected for the V3-V4 region of bacteria for day-480 and day-840 days. It is important to note that different primer pairs between 2016 and 2017/2018 were used in this study; we annotated all OTUs initially, and then analyzed all data using relative abundances.
The primers we used target the Internal Transcribed Spacer (ITS) region, albeit at different sites within ITS. The ITS2 region is recognized for its higher level of genomic conservation compared to ITS1, making it capable of providing enhanced resolution and robustness. According to Toju et al. (2012), ITS4_KYO3 exhibited low coverage across non-Dikarya fungi (77.8%), whereas the remaining primers covered more than 90% of non-Dikarya taxa. Consequently, we adjusted the primer pair for subsequent sequencing to enhance the taxonomic coverage of the findings; specifically, we substituted the original primers, ITS1F / ITS2, with ITS3_KYO2 / ITS4 for the final two harvest dates because we wanted higher taxonomic resolution for these later time points, when we expected higher variability in our microbial patterns. Importantly, the aim of our study was to determine the impact of bamboo leaf litter additions on the microbial communities of three native forest soil types, and so we focused our quantitative comparisons on the treatment effects within a time point and only qualitatively compared temporal patterns.
The PCR products were purified using the GeneJET RNA Purification Kit (Thermo Scientific) and quantified with a QuantiFluorTM-ST Fluorometer Instrument (Promega, Madison, WI, USA). Sequencing libraries were then prepared using the PCR products with Illumina TruSeq DNA sample Prep kits (Illumina, San Diego, CA, United States). DNA was sequenced by the Gene Denovo Biotechnology Co., Ltd. (Guangzhou, China) using the Illumina HiSeq2500 (PE250) platform.
Bioinformatic analysis
Clean paired-end reads were merged into raw tags using FLSAH v1.2.11 (Magoč and Salzberg 2011). The merging process required a minimum overlap of 10 bp and allowed for a mismatch error rate of 2%. Subsequently, the QIIME V1.9.1 (Caporaso et al. 2010) pipeline was employed to filter out noisy sequences from raw tags, following specific filtering conditions (Bokulich et al. 2013), resulting in the acquisition of high-quality clean tags. Then, the UCHIME algorithm (http://www.drive5.com/usearch/manual/uchime_algo.html) was employed to remove all chimeric tags, resulting in the acquisition of effective tags for subsequent analysis. The effective tags were clustered into operational taxonomic units (OTUs) with a similarity threshold of ≥ 97% using the UPARSE pipeline (Edgar 2013). Within each cluster, the tag sequence with the highest abundance was selected as the representative sequence. The representative sequences of bacteria were taxonomically classified using a naive Bayesian model implemented in RDP classifier Version 2.2 (Wang et al. 2007), which utilizes the SILVA Database (Pruesse et al. 2007) as the reference. In contrast, the representative sequences of fungi were identified and assigned using a BLAST search against the RDP Classifier. This process employed the Naïve Bayesian assignment method (Wang et al. 2007), with the UNITE Database (version 2016_11_20_ver7) serving as the reference (confidence threshold > 0.8).
Calculations and statistics
For all litterbags, we calculated mass remaining as follows:
where M0 and M1 represent the dry mass of initial and remaining litter, respectively.
Litter decomposition rates (k) were calculated by fitting the observed data to the exponential decay model as described by Olson (1963):
where Mt represented the litter mass remaining at collection day t. The coefficient k was the decomposition rate (expressed as month−1).
The microbial richness index was used to evaluate the number of OTUs contained in the assemblage, represented by Chao 1. Microbial evenness (represented by Simpson’s E) and diversity (Shannon’s H) were used to evaluate different aspects of the diversity of bacteria and fungi (Dong et al. 2021).
For each sample, OTUs < 5 reads were excluded from analyses. OTUs that had zero abundance after bioinformatic filtering were removed from the dataset. After filtering out invalid OTUs, soil bacterial and fungal richness (Chao 1), Shannon’s diversity (H), and Simpson’s evenness (E) were calculated for each sample using the R package vegan (Otsing et al. 2018). Bacterial and fungal assemblages were visualized using principal component analysis (PCA), and non-parametric permutational multivariate analysis of variance (PerMANOVA) was used to determine the significance of overall community differences. All graphs were created using Origin 2019 for Windows 10.
Results
Litter initial chemical composition and decomposition dynamics
The initial chemical composition of leaf litter is given in Table 2. Moso bamboo had the lowest C:N ratio and lignin concentration, as well as the highest nitrogen and phosphorus concentrations. The lowest concentrations of nitrogen and phosphorus were found in C. japonica, which were also significantly lower than these nutrient concentrations in bamboo leaf litter.
After day 840, the decomposition rate of Moso bamboo leaf litter in the Moso bamboo forest stand (mo-MO) was faster than its rate in the other three forest stands (Table 3). Compared to broadleaf, C. japonica and C. lanceolata leaf litter, the decomposition rate of Moso bamboo leaf litter was highest. C. japonica showed the lowest decomposition rate among all seven treatments (Table 3).
Response of soil microorganisms’ richness and diversity to Moso bamboo leaf litter input
Soil bacterial and fungal richness were very consistent across litter treatments, as indicated by the low variation in Chao 1 values (Fig. 1). At the early decay stage (day 120), the bacterial richness of mo-MO was higher than mo-BR (P > 0.05) and mo-CR (P > 0.05), and lower than mo-CU (P < 0.05). For BR and CU forest stands, there was no significant change in bacterial richness between before and after mo leaf litter input; however, mo leaf litter input decreased the bacterial richness of CR soils, especially at the middle decay stage (day 480s, P < 0.05). Results also indicate that mo-BR and mo-CR treatments were lower than mo-mo and mo-CU treatments at 480 and 840 days. The cu-CU treatment was always higher than the br-BR and cr-CR treatments, especially at day 840 (P < 0.05). Soil bacterial richness increased in all treatments as decomposition progressed (Fig. 1A, B, C).
A-C Bacterial and D-F fungal Chao 1 indexes of surface soils (0–10 cm) under litter bags in different treatments: Moso bamboo leaf litter decomposition in Moso bamboo (mo-MO), broad-leaf (mo-BR), Cryptomeria japonica var. sinensis (mo-CR), and Chinese fir (mo-CU) forest stands, and broad-leaf litter decomposition in broad-leaf forest stands (br-BR), C. japonica var. sinensis leaf litter decomposition in C. japonica var. sinensis forest stands (cr-CR), and Cunninghamia lanceolata leaf litter decomposition in Chinese fir forest stands (cu-CU). Lower case letters above bars denote significant differences among treatments
Soil fungal richness was low relative to bacterial richness at the early decay stage (day 120), and the mo leaf litter input did not change the richness in BR, CR, and CU forest stands (P > 0.05, Fig. 1D). Soil fungal richness in the cr-CR treatment was the lowest among all treatments and significantly lower than in the mo-MO, mo-CU, and cu-CU treatments. Soil fungal richness in the mo-CR treatment was significantly lower than in the mo-MO, mo-BR, and mo-CU treatments at day 840 (Fig. 1F).
Soil bacterial and fungal evenness (Simpson’s E) results showed no statistical differences among treatments, except for small differences in bacterial evenness on day 840 (Fig. 2). During the entire decomposition experiment, mo leaf litter input did not result in significant changes in diversity (Shannon’s H) or evenness (Simpson’s E) for soil bacteria or fungi. Shannon’s H for bacteria in the cr-CR treatment was the lowest (Fig. 2A, B, C). At day 480, Shannon’s H of bacteria in the mo-MO treatment was significantly higher than that of bacteria in the mo-BR and mo-CR treatments (Fig. 2B). At day 120, Shannon’s H of fungal diversity in the cu-CU treatment exhibited the highest performance, which was significantly higher than that of fungi in the mo-BR, br-BR, and cr-CR treatments (Fig. 2D).
Bacterial and fungal diversity (Shannon’s H) and evenness (Simpson’s E) in surface soil (0–10 cm) under litter bags in different treatments: Moso bamboo leaf litter decomposition in Moso bamboo (mo-MO), broad-leaf (mo-BR), Cryptomeria japonica var. sinensis (mo-CR), and Chinese fir (mo-CU) forest stands, and broad-leaf litter decomposition in broad-leaf forest stands (br-BR), C. japonica var. sinensis leaf litter decomposition in C. japonica var. sinensis forest stands (cr-CR), and Cunninghamia lanceolata leaf litter decomposition in Chinese fir forest stands (cu-CU). Lower case letters above bars denote significant differences among treatments. Differing lower-case letters above bars denote significant differences of Shannon’s H or Simpson’s E among treatments
At the early decomposition stage (day 120), the dominant phyla were Protebacteria and Actinobacteria, which accounted for ~ 15% of total soil bacteria (Fig. 3). The soil bacterial composition of Moso bamboo was similar to the three native forest plots. For soil fungi, the dominant phyla were Ascomycota, Basidiomycota, and Mortierellomycota at day 120. Moso bamboo leaf litter input changed the fungal composition at the early decay stages (day 120), reducing Ascomycota relative abundance, but increasing Mortierellomycota relative abundance. As decomposition progressed, these changes disappeared, and the relative abundances of taxa mostly returned to their original proportions.
Bacterial and fungal relative abundances in surface soil (0–10 cm) under litter bags of different treatments. Moso bamboo leaf litter decomposition in Moso bamboo (mo-MO), broad-leaf (mo-BR), Cryptomeria japonica var. sinensis (mo-CR), and Chinese fir (mo-CU) forest stands, and broad-leaf litter decomposition in broad-leaf forest stands (br-BR), C. japonica var. sinensis leaf litter decomposition in C. japonica var. sinensis forest stands (cr-CR), and Cunninghamia lanceolata leaf litter decomposition in Chinese fir forest stands (cu-CU). Lower case letters above bars denote significant differences among treatments
Both soil bacterial and fungal communities demonstrated a clustering relationship in each decomposition treatment (Fig. 4)., The soil bacterial community remained close to the original soil community after bamboo leaf litter addition, except for the mo-CR vs. cr-CR treatments at days 480 and 840 (both P = 0.01). community Similarly, the fungal soil community remained close to the original soil community after bamboo leaf litter addition, except for the mo-CR vs. cr-CR treatments at 480 days and 840 days (both P = 0.01) and the mo-CU vs. cu-CU treatments at 480 days (P = 0.03).
Principal component analysis (PCA) of the soil bacterial and fungal assemblage structures based on the relative abundances of each OTU during litter decomposition. Plots depict A the soil bacterial assemblage at day 120, B the soil bacterial assemblage at day 480, C the soil bacterial assemblage at day 840, D the soil fungal assemblage at day 120, E the soil fungal assemblage at day 480, and F the soil fungal assemblage at day 840
Discussion
Leaf litter decomposition
In the same environment, leaf litter quality determines its decomposition rate (Bradford et al. 2016). Lignin and nitrogen content are key indicators for litter quality: high quality litter typically contains low lignin and high nitrogen concentrations, which make it easy to decay (Bradford et al. 2016). In this study, we showed that lignin concentrations of Moso bamboo leaf litter were significantly lower than the other three litters, whereas, nitrogen content showed the opposite trend (Table 2). That is, leaf litter quality of Moso bamboo (based on lignin content and C:N, Table 2) was higher than that of the other three litters, which was also reflected in the litter decomposition patterns. These results support Hypothesis 1, which was also consistent with recent research on Moso bamboo litter decomposition (Luan et al. 2021). High bamboo leaf litter decomposition rates meant that more litter nutrients were made available to participate in biogeochemical cycles, which could accelerate cycling rates and affect the stability of ecosystem function (Lee et al. 2017).
We also compared decomposition rates of bamboo leaf litter in these four study forests (Fig. 1A), which demonstrated a distinct ‘home-field advantage’ (HFA), since the fastest bamboo decay rates arose in bamboo forest plots. Recent studies suggest that low quality litter can produce strong HFA with its low decomposability (Hoyos-Santillan et al. 2018; Palozzi and Lindo 2018). We speculate that bamboo leaf litter produces an HFA effect with high quality litter because of its specificity with microbial decomposers. Moso bamboo belongs to Gramineous plants, which have a high silicon content, such that a specific decomposer community may be formed in the decomposition process (Schaller et al. 2016). Thus, when bamboo leaf litter enters novel forest plots, this maladapted soil decomposer community could result in decreased decay rates.
Soil microorganism richness and diversity
Nutrients from litter decomposition are important resources for the growth and reproduction of soil microorganisms (Tamura and Tharayil 2014). Previous studies have found that invasive plants generally have high quality leaf litter (i.e., low lignin content) compared with native plants, allowing invasive plants to supply more soluble carbon and mineralized nutrients to maintain a richer soil microbial community (Prescott and Zukswert 2016). In this study, the addition of bamboo litter did not alter the microbial richness and diversity in the BR and CU forest soils, but it did affect the microbial richness of CR forest soils with lower litter quality (Figs. 2 and 3). These results partially support Hypothesis 2. A previous study demonstrated that fast-decomposing litter can provide more labile carbon to microorganisms, thus enhancing the microbial carbon use efficiency in soils (Ridgeway et al. 2022). This phenomenon was also evident in the mo-CR treatment in our study, where the fast decomposition of bamboo litter likely brought more labile carbon to the CR forest soils, promoting microbial growth and reproduction and leading to changes in microbial richness and diversity. In the mo-BR and mo-CU treatments, the changes in soil microbial richness and diversity were not significant. This may be because bamboo leaf litter is high inquality (i.e., low lignin) and decomposes rapidly, so the addition of bamboo litter did not generate a noticeable priming effect (Fanina et al. 2020). Another possible reason for the lack of microbial richness and diversity differences in the mo-BR and mo-CU treatments was that we did not continuously add fresh leaf litter in the process of our experiment. A previous study demonstrated that repeated litter additions would increase carbon use efficiency and change soil microbial community structure compared to a single litter addition (Wei et al. 2022). Our results showed that the greater the initial difference in litter quality, the more pronounced the impact was on soil microorganisms.
Soil bacterial phyla
In this study, Proteobacteria, Acidobacteria, Verrucomicrobia, and Planctomycetales were the main bacterial phyla in soils across all seven treatments. During early decomposition, the combined relative abundances of Proteobacteria and Acidobacteria reached up to 75% (Fig. 3), which is consistent with previous research (Jia et al. 2022). Some researchers have speculated that Proteobacteria belongs to early decomposers that can break down recalcitrant substances, and that they are especially effective decomposers when their relative abundances in soils are high (Jia et al. 2022; Zhang et al. 2017). We found that Verrucomicrobia abundances increased during the middle stage of decomposition (day 480), especially in mo-MO and mo-CU treatments, where their increases exceeded 10% (Fig. 3). High abundances of Verrucomicrobia in soils of Moso bamboo (MO) and Chinese fir (CU) forest stands might be related to low nitrogen and phosphorus concentrations in these two soils (Table 2). Verrucomicrobia can participate in nitrogen fixation and polysaccharide degradation (Shen et al. 2017), and they seem to favor barren soils (i.e., with low nitrogen availability) where they appear to compete with other bacteria (Isanapong et al. 2013). During later stages of decomposition in our study, Planctomycetales became a dominant soil bacterial phyla in all treatments. Planctomycetales is unique among microbes in that it can break down chitin in soils (Dedysh and Ivanova 2018; Wang et al. 2015). We speculate that the increase of Planctomycetales abundance in the late stage of decomposition (day 840) was due to chitin accumulation from fungal necromass (Adamczyk et al. 2019).
After the addition of bamboo litter, the relative proportions of soil bacterial phyla in the BR forest soils did not show significant changes at the three sampling time points; however, the PCA analysis results indicate that, at specific time points, the addition of bamboo litter altered the microbial composition of CR and CU forest soils. These findings partially supported Hypothesis 3. Different chemical properties of litter (e.g., N content, lignin content, lignin:N ratio) can lead to variation in soil properties (e.g., pH, C and N pools, N mineralization rates), which may result in changes in the soil bacterial composition (Rivest et al. 2015). The chemical properties of bamboo litter differed significantly from the other three forest litter types (Table 2); however, bamboo litter did not alter the bacterial composition in the soils of these three forest types. This may be related to the resistance and resilience of soil bacterial communities. Many studies have indicated that soil bacterial communities exhibit some resistance (in the short term) and resilience (in the long term) to mild external disturbances (Steven et al. 2021). In this study, we did not observe changes in bacterial composition due to bamboo litter addition, possibly because the first sampling event was after a relatively long time (120 days). Consequently, we could not determine if these forests exhibited bacterial resistance to bamboo litter additions. However, our results do support the notion that soil bacteria are resilient to bamboo litter additions.
Soil fungal phyla
Soil fungi are key players during litter decay because of their ability to degrade recalcitrant compounds such as lignin (Schneider et al. 2012). In our study, Mortierellomycotina was dominant during the early decomposition stage (day 120, Fig. 3). Mortierellomycotina belongs to fast-growing saprotrophs that prefer labile C compounds. Thus, much of the labile C compounds from initial decomposition input can support Mortierellomycotina growth and reproduction (Taylor and Sinsabaugh 2015).
Ascomycota is also a very common fungi in soil (Prober et al. 2015), which is thought to have the ability to degrade cellulose and lignin (Sanaullah et al. 2016). In our study, during the middle- and late-stages of decomposition, soil fungi were dominated by Ascomycota (Fig. 4). Ascomycota may be better able to cope with environmental stressors by utilizing a variety of resources, leading to more generalist strategies that maintain their dominant position in soils (Egidi et al. 2019). In our study, bamboo leaf litter input decreased soil Ascomycota abundances in the other three forest plots. That phenomenon was likely because high quality bamboo litter is conducive to supporting other fungi, which could limit the available ecological niche space of Ascomycota. In this regard, these findings support Hypothesis 3.
Our study found that the relative abundances of Basidiomycota gradually increased throughout the decomposition process. This could be relevant given the accumulation of refractory substances during litter decay (Ma et al. 2013). Our study results also demonstrated that the relative abundances of Basidiomycota were highest in Chinese fir forest soils (i.e., both in mo-CU and cu-CU treatments).
In the later stages of decomposition, the fungal communities in the soils of the three native forests reverted to their original states. Studies suggest that soil fungal communities also possess a certain degree of resilience in response to environmental changes (Alvarez-Manjarrez and Garibay-Orijel 2021), and our results corroborated this idea. Compared to bacteria, the recovery of fungal communities to their original states was relatively slow, which can be attributed to the slower growth rates of fungi (Rivest et al. 2015).
Soil microorganism community composition
Change of soil microbial communities are thought to act on a very local scale when available substrates are altered (Li et al. 2020). After the same litter type is consistently input, specialized microbial decomposer communities can develop in soils (Keiser et al. 2011). For example, previous studies have demonstrated that the diversity of soil microorganisms was influenced by litter incorporation (Bamminge et al. 2014; Müller et al. 2017; Jin et al. 2022). In our study, the soil microbial community of BR forest with high litter quality showed no distinct changes after bamboo litter addition, but we observed significant changes in CR and CU forest soil communities with low litter quality (see PCA results, Fig. 4). A recent study suggested that aboveground litter additions significantly increased the gene copy number of both bacterial and fungal communities in early decomposition (i.e., the first 72 days), which was stimulated by labile C released from litter (e.g., sugars and polysaccharides), and had no effect after 72 days (Xing et al. 2019). In our study, we measured bacterial and fungal communities after 120 days of litter decomposition, which means we may have missed the opportunity to observe differences in BR forest soil microbial communities associated with bamboo litter addition. Our results also suggest that soil microbial communities could return to their original, pre-litter-addition compositions without continuous input of fresh leaf litter. Ultimately, our findings partially support Hypothesis 3, and further research is needed to confirm this phenomenon.
While our study did not directly assess the mycorrhizal symbionts associated with the plant species in our experiment, the identity of these partners likely influenced our decomposition and microbial community patterns, as this has been documented in other systems (Keller and Phillips 2019; Deng et al. 2023). Existing published information suggests that these symbiotic partners were likely different between bamboo and the plant species we examined in this study. For example, for Chinese fir and broad-leaf trees, the primary mycorrhizae associates have been documented to be arbuscular mycorrhizae (AM), but for bamboo the symbiont is ectomycorrhizae (EM) (e.g., Koele et al. 2012; Kong et al. 2014; Pan et al. 2015; Tedersoo et al. 2014; Wang and Qui 2006). Additionally, while we couldn’t find information comparing the different types of mycorrhizae in Daphniphyllum macropodum, spore density and species richness of AM fungi can be high in these shrubs (Yang 2018). Further, while we found no direct evidence documenting the mycorrhizae associated in Machilus thunbergii, indirect evidence suggests that this species is associated with AM fungi as well (Ishikawa and Nara 2023; Kamijo et al. 2002). Collectively, this evidence suggests that the type and composition of mycorrizae associated with the different species we examined influences litter decomposition and associated free-living fungal communities of decomposing leaf litter, and we believe this is an important area of future research.
Conclusions
Our results demonstrate that Moso bamboo leaf litter is of high quality and has rapid litter decomposition rates compared to litter from the three native forest plants. Furthermore, within the decomposition time points we sampled, we only observed distinct differences in certain forest soil microorganisms in response to the addition of bamboo leaf litter. Therefore, we suggest that soil microorganism community responses to changes in litter inputs likely only occur on short time scales, and the soil microorganism communities we studied were resilient to bamboo litter addition.
References
Adamczyk B, Sietiö OM, Biasi C, Heinonsalo J (2019) Interaction between tannins and fungal necromass stabilizes fungal residues in boreal forest soils. New Phytol 223:16–21
Alvarez-Manjarrez J, Garibay-Orijel R (2021) Resilience of soil fungal community to hurricane Patricia (category 4). For Ecol Manag 498:119550. https://doi.org/10.1016/j.foreco.2021.119550
Bai S, Wang Y, Conant RT, Zhou G, Xu Y, Wang N, Fang F, Chen J (2016) Can native clonal moso bamboo encroach on adjacent natural forest without human intervention? Sci Rep 6:31504. https://doi.org/10.1038/srep31504
Bamminge C, Zaiser N, Zinsser P, Lamers M, Marhan S (2014) Effects of biochar, earthworms, and litter addition on soil microbial activity and abundance in a temperate agricultural soil. Biol Fertil Soils 50:1189–1200. https://doi.org/10.1007/s00374-014-0968-x
Biddle JF, Fitz-Gibbon S, Schuster SC, Brenchley JE, House CH (2008) Metagenomic signatures of the Peru Margin subseafloor biosphere show a genetically distinct environment. Proc Natl Acad Sci 105:10583–10588. https://doi.org/10.1073/pnas.0709942105
Bokulich NA, Subramanian S, Faith JJ, Gevers D, Gordon JI, Knight R, Mills DA, Caporaso JG (2013) Quality-filtering vastly improves diversity estimates from Illumina amplicon sequencing. Nat Methods 10:57–59. https://doi.org/10.1038/nmeth.2276
Bradford MA, Berg B, Maynard DS, Wieder WR, Wood SA (2016) Future directions: understanding the dominant controls on litter decomposition. J Ecol 104:229–238
Callahan BJ, Sankaran K, Fukuyama JA, McMurdie PJ, Holmes SP (2016) Bioconductor workflow for microbiome data analysis: from raw reads to community analyses. F1000Research 5:1492
Caporaso JG, Kuczynski J, Stombaugh J, Bittinger K, Bushman FD, Costello EK, Fierer N, Peña AG, Goodrich JK, Gordon J, Huttley GA, Kelley ST, Knights D, Koenig JE, Ley RE, Lozupone CA, McDonald D, Muegge BD, Pirrung M, Reeder J, Sevinsky JR, Turnbaugh PJ, Walters WA, Widmann J, Yatsunenko T, Zaneveld J, Knight R (2010) QIIME allows analysis of high-throughput community sequencing data. Nat Methods 7:335–336. https://doi.org/10.1038/nmeth.f.303
Chu H, Zhu YG (2019) Editorial: China Soil Microbiome thematic issue. FEMS Microbiol Ecol 95. https://doi.org/10.1093/femsec/fiz170
Collins CG, Spasojevic MJ, Alados CL, Aronson EL, Benavides JC, Cannone N, Caviezel C, Grau O, Guo H, Kudo G, Kuhn NJ, Müllerová J, Phillips ML, Pombubpa N, Reverchon F, Shulman HB, Stajich JE, Stokes A, Weber SE, Diez JM (2020) Belowground impacts of alpine woody encroachment are determined by plant traits, local climate, and soil conditions. Glob Change Biol 26:7112–7127. https://doi.org/10.1111/gcb.15340
Dedysh SN, Ivanova AA (2018) Planctomycetes in boreal and subarctic wetlands: diversity patterns and potential ecological functions. FEMS Microbiol Ecol 95. https://doi.org/10.1093/femsec/fiy227
Deng M, Hu S, Guo L, Jiang L, Huang Y, Schmid B, Liu C, Chang P, Li S, Liu X, Ma K, Liu L (2023) Tree mycorrhizal association types control biodiversity-productivity relationship in a subtropical forest. Sci Adv 9:eadd4468. https://doi.org/10.1126/sciadv.add4468
Deng X, Liu Y, Wu Y (2003) Interconnection among dominant plant populations of Castanopsis community in Jianggang mountain nature reserve. Acta Phytoecologica Sinica 27:531–536 (In Chinese)
Dong X, Gao P, Zhou R, Li C, Dun X, Niu X (2021) Changing characteristics and influencing factors of the soil microbial community during litter decomposition in a mixed Quercus acutissima Carruth. And Robinia pseudoacacia L. forest in Northern China. CATENA 196:104811. https://doi.org/10.1016/j.catena.2020.104811
Edgar RC (2013) UPARSE: highly accurate OTU sequences from microbial amplicon reads. Nat Methods 10:996–998. https://doi.org/10.1038/nmeth.2604
Egidi E, Delgado-Baquerizo M, Plett JM, Wang J, Eldridge DJ, Bardgett RD, Maestre FT, Singh BK (2019) A few Ascomycota taxa dominate soil fungal communities worldwide. Nat Commun 10:2369. https://doi.org/10.1038/s41467-019-10373-z
Fanina N, Alavoineb G, Bertrand I (2020) Temporal dynamics of litter quality, soil properties and microbial strategies as main drivers of the priming effect. Geoderma 377:114576. https://doi.org/10.1016/j.geoderma.2020.114576
Fang H, Liu Y, Bai J, Li A, Deng W, Bai T, Liu X, Lai M, Feng Y, Zhang J, Zou Q, Wu N, Zhang L (2022) Impact of Moso Bamboo (Phyllostachys edulis) expansion into Japanese Cedar Plantations on soil fungal and bacterial community compositions. Forests 13:1190
Fry EL, De Long JR, Álvarez Garrido L, Alvarez N, Carrillo Y, Castañeda-Gómez L, Chomel M, Dondini M, Drake JE, Hasegawa S, Hortal S, Jackson BG, Jiang M, Lavallee JM, Medlyn BE, Rhymes J, Singh BK, Smith P, Anderson IC, Bardgett RD, Baggs EM, Johnson D (2019) Using plant, microbe, and soil fauna traits to improve the predictive power of biogeochemical models. Methods Ecol Evol 10:146–157. https://doi.org/10.1111/2041-210X.13092
Fu J (2001) Chinese Moso Bamboo: its importance. Bamboo 22:5–7
Gardes M, Bruns TD (1993) ITS primers with enhanced specificity for basidiomycetes - application to the identification of mycorrhizae and rusts. Mol Ecol 2:113–118. https://doi.org/10.1111/j.1365-294X.1993.tb00005.x
Gartner TB, Cardon ZG (2004) Decomposition dynamics in mixed-species leaf litter. Oikos 104:230–246. https://doi.org/10.1111/j.0030-1299.2004.12738.x
Guo J, Yang Z, Lin C, Liu X, Chen G, Yang Y (2016) Conversion of a natural evergreen broadleaved forest into coniferous plantations in a subtropical area: effects on composition of soil microbial communities and soil respiration. Biol Fertil Soils 52:799–809. https://doi.org/10.1007/s00374-016-1120-x
Hoyos-Santillan J, Lomax BH, Turner BL, Sjögersten S (2018) Nutrient limitation or home field advantage: does microbial community adaptation overcome nutrient limitation of litter decomposition in a tropical peatland? J Ecol 106:1558–1569. https://doi.org/10.1111/1365-2745.12923
Inderjit, van der Putten WH (2010) Impacts of soil microbial communities on exotic plant invasions. Trends Ecol Evol 25:512–519. https://doi.org/10.1016/j.tree.2010.06.006
Isanapong J, Sealy Hambright W, Willis AG, Boonmee A, Callister SJ, Burnum KE, Paša-Tolić L, Nicora CD, Wertz JT, Schmidt TM, Rodrigues JLM (2013) Development of an ecophysiological model for Diplosphaera colotermitum TAV2, a termite hindgut Verrucomicrobium. ISME J 7:1803–1813. https://doi.org/10.1038/ismej.2013.74
Ishikawa A, Nara K (2023) Primary succession of ectomycorrhizal fungi associated with Alnus sieboldiana on Izu-Oshima Island, Japan. Mycorrhiza 33:187–197. https://doi.org/10.1007/s00572-023-01112-w
Jia T, Liang X, Guo T, Wu T, Chai B (2022) Bacterial community succession and influencing factors for Imperata cylindrica litter decomposition in a copper tailings area of China. Sci Total Environ 815:152908. https://doi.org/10.1016/j.scitotenv.2021.152908
Jin Y, Wei X, White JF, Chen T, Li X, Chen Z, Li C (2022) Soil fungal and bacterial communities are altered by the incorporation of leaf litter containing a fungal endophyte. Eur J Soil Sci 73:e13240. https://doi.org/10.1111/ejss.13240
Kamijo T, Kitayama K, Sugawara A, Urushimichi S, Sasai K (2002) Primary succession of the warm-temperate broad-leaved forest on a volcanic island, Miyake-jima, Japan. Folia Geobot 37:71–91. https://doi.org/10.1007/BF02803192
Keiser AD, Strickland MS, Fierer N, Bradford MA (2011) The effect of resource history on the functioning of soil microbial communities is maintained across time. Biogeosciences 8:1477–1486. https://doi.org/10.5194/bg-8-1477-2011
Keller AB, Phillips RP (2019) Leaf litter decay rates differ between mycorrhizal groups in temperate, but not tropical, forests. New Phytol 222:556–564. https://doi.org/10.1111/nph.15524
Koele N, Dickie IA, Oleksyn J, Richardson SJ, Reich PB (2012) No globally consistent effect of ectomycorrhizal status on foliar traits. New Phytol 196:845–852. https://doi.org/10.1111/j.1469-8137.2012.04297.x
Kong D, Ma C, Zhang Q, Li L, Chen X, Zeng H, Guo D (2014) Leading dimensions in absorptive root trait variation across 96 subtropical forest species. New Phytol 203:863–872. https://doi.org/10.1111/nph.12842
Lee MR, Bernhardt ES, van Bodegom PM, Cornelissen JHC, Kattge J, Laughlin DC, Niinemets Ü, Peñuelas J, Reich PB, Yguel B, Wright JP (2017) Invasive species’ leaf traits and dissimilarity from natives shape their impact on nitrogen cycling: a meta-analysis. New Phytol 213:128–139. https://doi.org/10.1111/nph.14115
Li Y, Li Y, Chang SX, Xu Q, Guo Z, Gao Q, Qin Z, Yang Y, Chen J, Liang X (2017) Bamboo invasion of broadleaf forests altered soil fungal community closely linked to changes in soil organic C chemical composition and mineral N production. Plant Soil 418:507–521. https://doi.org/10.1007/s11104-017-3313-y
Li X, Qu Z, Zhang Y, Ge Y, Sun H (2022) Soil Fungal Community and potential function in different forest Ecosystems. Diversity 14:520
Li Y, Veen GF, Hol WHG, Vandenbrande S, Hannula SE, ten Hooven FC, Li Q, Liang W, Bezemer TM (2020) Home’ and ‘away’ litter decomposition depends on the size fractions of the soil biotic community. Soil Biol Biochem 144:107783. https://doi.org/10.1016/j.soilbio.2020.107783
Liu J, Liu X, Song Q, Compson ZG, LeRoy CJ, Luan F, Wang H, Hu Y, Yang Q (2020) Synergistic effects: a common theme in mixed-species litter decomposition. New Phytol 227:757–765. https://doi.org/10.1111/nph.16556
Liu X, Siemann E, Cui C, Liu Y, Guo X, Zhang L (2019) Moso bamboo (Phyllostachys edulis) invasion effects on litter, soil and microbial PLFA characteristics depend on sites and invaded forests. Plant Soil 438:85–99. https://doi.org/10.1007/s11104-019-04010-3
Lladó S, López-Mondéjar R, Baldrian P (2017) Forest soil bacteria: diversity, involvement in ecosystem processes, and response to global change. Microbiol Mol Biol Rev 81:e00063–e00016. https://doi.org/10.1128/MMBR.00063-16
Lu R (2000) Methods of soil and agrochemical analysis. China Agricultural Science and Technology Press, Beijing, China (In Chinese)
Luan J, Liu S, Li S, Whalen JK, Wang Y, Wang J, Liu Y, Dong W, Chang SX (2021) Functional diversity of decomposers modulates litter decomposition affected by plant invasion along a climate gradient. J Ecol 109:1236–1249. https://doi.org/10.1111/1365-2745.13548
Ma A, Zhuang X, Wu J, Cui M, Lv D, Liu C, Zhuang G (2013) Ascomycota members dominate fungal communities during straw residue decomposition in Arable Soil. PLoS ONE 8:e66146. https://doi.org/10.1371/journal.pone.0066146
Magoč T, Salzberg SL (2011) FLASH: fast length adjustment of short reads to improve genome assemblies. Bioinform 27:2957–2963. https://doi.org/10.1093/bioinformatics/btr507
Moore JC, Berlow EL, Coleman DC, de Ruiter PC, Dong Q, Hastings A, Johnson NC, McCann KS, Melville K, Morin PJ, Nadelhoffer K, Rosemond AD, Post DM, Sabo JL, Scow KM, Vanni MJ, Wall DH (2004) Detritus, trophic dynamics and biodiversity. Ecol Lett 7:584–600. https://doi.org/10.1111/j.1461-0248.2004.00606.x
Müller K, Marhan S, Kandeler E, Poll C (2017) Carbon flow from litter through soil microorganisms: from incorporation rates to mean residence times in bacteria and fungi. Soil Biol Biochem 115:187–196. https://doi.org/10.1016/j.soilbio.2017.08.017
Olson JS (1963) Energy storage and the balance of producers and decomposers in ecological systems. Ecology 44:322–331. https://doi.org/10.2307/1932179
Otsing E, Barantal S, Anslan S, Koricheva J, Tedersoo L (2018) Litter species richness and composition effects on fungal richness and community structure in decomposing foliar and root litter. Soil Biol Biochem 125:328–339. https://doi.org/10.1016/j.soilbio.2018.08.006
Palozzi JE, Lindo Z (2018) Are leaf litter and microbes team players? Interpreting home-field advantage decomposition dynamics. Soil Biol Biochem 124:189–198. https://doi.org/10.1016/j.soilbio.2018.06.018
Pan L, Mou P, Bai S-B, Gu M (2015) Impact of Phyllostachys heterocycla ‘Pubescens’ expansion on mycorrhizal associations of the adjacent forests. Chin J Plant Ecol 39:371–382. https://doi.org/10.17521/cjpe.2015.0036. (In Chinese)
Prescott CE, Zukswert JM (2016) Invasive plant species and litter decomposition: time to challenge assumptions. New Phytol 209:5–7
Prober SM, Leff JW, Bates ST, Borer ET, Firn J, Harpole WS, Lind EM, Seabloom EW, Adler PB, Bakker JD, Cleland EE, DeCrappeo NM, DeLorenze E, Hagenah N, Hautier Y, Hofmockel KS, Kirkman KP, Knops JMH, La Pierre KJ, MacDougall AS, McCulley RL, Mitchell CE, Risch AC, Schuetz M, Stevens CJ, Williams RJ, Fierer N (2015) Plant diversity predicts beta but not alpha diversity of soil microbes across grasslands worldwide. Ecol Lett 18:85–95. https://doi.org/10.1111/ele.12381
Pruesse E, Quast C, Knittel K, Fuchs BM, Ludwig W, Peplies J, Glöckner FO (2007) SILVA: a comprehensive online resource for quality checked and aligned ribosomal RNA sequence data compatible with ARB. Nucleic Acids Res 35:7188–7196. https://doi.org/10.1093/nar/gkm864
Ridgeway JR, Morrissey EM, Brzostek ER (2022) Plant litter traits control microbial decomposition and drive soil carbon stabilization. Soil Biol Biochem 175:108857. https://doi.org/10.1016/j.soilbio.2022.108857
Rivest D, Paquette A, Shipley B, Reich PB, Messier C (2015) Tree communities rapidly alter soil microbial resistance and resilience to drought. Funct Ecol 29:570–578. https://doi.org/10.1111/1365-2435.12364
Sanaullah M, Chabbi A, Maron P-A, Baumann K, Tardy V, Blagodatskaya E, Kuzyakov Y, Rumpel C (2016) How do microbial communities in top- and subsoil respond to root litter addition under field conditions? Soil Biol Biochem 103:28–38. https://doi.org/10.1016/j.soilbio.2016.07.017
Schaller J, Böttger R, Dudel G, Ruess L (2016) Reed litter Si content affects microbial community structure and the lipid composition of an invertebrate shredder during aquatic decomposition. Limnologica 57:14–22. https://doi.org/10.1016/j.limno.2015.12.002
Schneider T, Keiblinger KM, Schmid E, Sterflinger-Gleixner K, Ellersdorfer G, Roschitzki B, Richter A, Eberl L, Zechmeister-Boltenstern S, Riedel K (2012) Who is who in litter decomposition? Metaproteomics reveals major microbial players and their biogeochemical functions. ISME J 6:1749–1762. https://doi.org/10.1038/ismej.2012.11
Schroeter SA, Eveillard D, Chaffron S, Zoppi J, Kampe B, Lohmann P, Jehmlich N, von Bergen M, Sanchez-Arcos C, Pohnert G, Taubert M, Küsel K, Gleixner G (2022) Microbial community functioning during plant litter decomposition. Sci Rep 12:7451. https://doi.org/10.1038/s41598-022-11485-1
Shen C, Ge Y, Yang T, Chu H (2017) Verrucomicrobial elevational distribution was strongly influenced by soil pH and carbon/nitrogen ratio. J Soils Sediments 17:2449–2456. https://doi.org/10.1007/s11368-017-1680-x
Sluiter A, Hames B, Ruiz R, Scarlata C, Sluiter J, Templeton D, Crocker DLAP (2008) Determination of structural carbohydrates and lignin in biomass. Lab Anal Procedure 1617:1–16
Song Q-n, Ouyang M, Yang Q-p, Lu H, Yang G-y, Chen F-s, Shi J-M (2016) Degradation of litter quality and decline of soil nitrogen mineralization after moso bamboo (Phyllostachys pubscens) expansion to neighboring broadleaved forest in subtropical China. Plant Soil 404:113–124. https://doi.org/10.1007/s11104-016-2835-z
Steven B, Phillips ML, Belnap J, Gallegos-Graves LV, Kuske CR, Reed SC (2021) Resistance, resilience, and recovery of dryland soil bacterial communities across multiple disturbances. Front Microbiol 12:648455. https://doi.org/10.3389/fmicb.2021.648455
Tamura M, Tharayil N (2014) Plant litter chemistry and microbial priming regulate the accrual, composition and stability of soil carbon in invaded ecosystems. New Phytol 203:110–124. https://doi.org/10.1111/nph.12795
Taylor DL, Sinsabaugh RL (2015) The soil fungi: occurrence, phylogeny, and ecology. Soil Microbiol Ecol Biochem 4:77–109
Tedersoo L, Bahram M, Põlme S, Kõljalg U, Yorou NS, Wijesundera R, Ruiz LV, Vasco-Palacios AM, Thu PQ, Suija A, Smith ME, Sharp C, Saluveer E, Saitta A, Rosas M, Riit T, Ratkowsky D, Pritsch K, Põldmaa K, Piepenbring M, Phosri C, Peterson M, Parts K, Pärtel K, Otsing E, Nouhra E, Njouonkou AL, Nilsson RH, Morgado LN, Mayor J, May TW, Majuakim L, Lodge DJ, Lee SS, Larsson K-H, Kohout P, Hosaka K, Hiiesalu I, Henkel TW, Harend H, Guo L-d, Greslebin A, Grelet G, Geml J, Gates G, Dunstan W, Dunk C, Drenkhan R, Dearnaley J, De Kesel A, Dang T, Chen X, Buegger F, Brearley FQ, Bonito G, Anslan S, Abell S, Abarenkov K (2014) Global diversity and geography of soil fungi. Science 346:1256688. https://www.science.org/doi/abs/10.1126/science.1256688
Tian XK, Wang MY, Meng P, Zhang JS, Zhou BZ, Ge XG, Yu FH, Li MH (2020) Native bamboo invasions into subtropical forests alter Microbial Communities in Litter and Soil. Forests 11:314
Toju H, Tanabe AS, Yamamoto S, Sato H (2012) High-Coverage ITS primers for the DNA-Based identification of Ascomycetes and Basidiomycetes in Environmental samples. PLoS ONE 7:e40863. https://doi.org/10.1371/journal.pone.0040863
Wang B, Qiu YL (2006) Phylogenetic distribution and evolution of mycorrhizas in land plants. Mycorrhiza 16:299–363. https://doi.org/10.1007/s00572-005-0033-6
Wang Q, Garrity GM, Tiedje JM, Cole JR (2007) Naive Bayesian classifier for rapid assignment of rRNA sequences into the new bacterial taxonomy. Appl Environ Microb 73:5261–5267. https://doi.org/10.1128/AEM.00062-07
Wang X, Sasaki A, Toda M, Nakatsubo T (2016) Changes in soil microbial community and activity in warm temperate forests invaded by moso bamboo (Phyllostachys pubescens). J for Res 21:235–243. https://doi.org/10.1007/s10310-016-0533-6
Wang X, Sharp CE, Jones GM, Grasby SE, Brady AL, Dunfield PF (2015) Stable-isotope probing identifies uncultured Planctomycetes as primary degraders of a Complex Heteropolysaccharide in Soil. Appl Environ Microbiol 81:4607–4615. https://doi.org/10.1128/AEM.00055-15
Wei Y, Xiong X, Ryo M, Badgery WB, Bi Y, Yang G, Zhang Y, Liu N (2022) Repeated litter inputs promoted stable soil organic carbon formation by increasing fungal dominance and carbon use efficiency. Biol Fertil Soils 58:619–631. https://doi.org/10.1007/s00374-022-01647-8
Whitaker J, Ostle N, Nottingham AT, Ccahuana A, Salinas N, Bardgett RD, Meir P, McNamara NP, Austin A (2014) Microbial community composition explains soil respiration responses to changing carbon inputs along an Andes-to-Amazon elevation gradient. J Ecol 102:1058–1071. https://doi.org/10.1111/1365-2745.12247
White TJ, Bruns T, Lee S, Taylor J (1990) Amplification and direct sequencing of fungal ribosomal RNA genes for phylogenetics. PCR Protocols: A Guide to Methods and Applications 18:315–322
Xing W, Lu X, Xu F, Ying J, Chen D, Bai Y (2019) Linking microbial community structure to carbon substrate chemistry in soils following aboveground and belowground litter additions. Appl Soil Ecol 141:18–25. https://doi.org/10.1016/j.apsoil.2019.05.007
Xu Q-F, Liang C-F, Chen J-H, Li Y-C, Qin H, Fuhrmann JJ (2020) Rapid bamboo invasion (expansion) and its effects on biodiversity and soil processes. Global Ecol Conserv 21:e00787. https://doi.org/10.1016/j.gecco.2019.e00787
Yang P-H (2018) The biological characteristics of Daphniphyllum macropodum and its exploitation. Fujian Agric Sci Technol 12:11–12 (In Chinese)
Zhang P, Li B, Wu J, Hu S (2019) Invasive plants differentially affect soil biota through litter and rhizosphere pathways: a meta-analysis. Ecol Lett 22:200–210. https://doi.org/10.1111/ele.13181
Zhang W, Lu Z, Yang K, Zhu J (2017) Impacts of conversion from secondary forests to larch plantations on the structure and function of microbial communities. Appl Soil Ecol 111:73–83. https://doi.org/10.1016/j.apsoil.2016.11.019
Zhu C-L, Shangguan L-P (2009) Preliminary study on the influence of the expansion edge on biodiversity of moso bamboo forest in Jinggang Mountain. Territory Nat Resour Stud 3:45–46 (In Chinese)
Acknowledgements
This research was supported by JIANGXI “DOUBLE THOUSAND PLAN” (江西省千人计划, jxsq2020101079); the National Natural Science Foundation of China (42067050, 41807028, 32060319, 32260335); Graduate Innovation Fund of Jiangxi Province (YC2022-s425).
Author information
Authors and Affiliations
Corresponding author
Ethics declarations
The authors declare that they have no known competing financial interests or personal relationships that could have appeared to influence the work reported in this paper.
Additional information
Responsible Editor: Feike A. Dijkstra.
Publisher’s Note
Springer Nature remains neutral with regard to jurisdictional claims in published maps and institutional affiliations.
Rights and permissions
Springer Nature or its licensor (e.g. a society or other partner) holds exclusive rights to this article under a publishing agreement with the author(s) or other rightsholder(s); author self-archiving of the accepted manuscript version of this article is solely governed by the terms of such publishing agreement and applicable law.
About this article
Cite this article
Liu, J., Compson, Z.G., Gui, X. et al. Weak responses of soil microorganisms to leaf litter inputs after native Phyllostachys edulis invasion into adjacent native forests. Plant Soil 494, 685–699 (2024). https://doi.org/10.1007/s11104-023-06311-0
Received:
Accepted:
Published:
Issue Date:
DOI: https://doi.org/10.1007/s11104-023-06311-0