Abstract
Cereal cyst nematodes (CCN) are a global economic problem for cereal production. Heterodera filipjevi is one of the most commonly identified and widespread CCN species found in many wheat production regions of the world. Transferring novel genes for resistance to H. filipjevi from wild relatives of wheat is a promising strategy for protection of wheat crops. A set of wheat–Dasypyrum villosum chromosome addition lines, T6V#4S·6AL translocation lines and their donor parental lines were tested for their response to the nematode. D. villosum and wheat–D. villosum disomic addition line DA6V#4 were resistant. As T6V#4S·6AL translocation lines were susceptible, resistance was presumed to be located on chromosome 6V#4L. The objective of this study was to produce and characterize wheat–6V#4L translocations and confirm the chromosome location of the resistance. Introgression lines T6V#4L·6AS, T6V#4L-4BL·4BS and DT6V#4L were developed and subjected to molecular cytogenetic analysis. These and four additional wheat–6V#4 introgression lines were tested for response to H. filipjevi in the greenhouse. The results indicated that introgression lines DA6V#4, T6V#4L·6AS, T6V#4L-4BL·4BS, T6V#4L·6V#4S-7BS and DT6VL#4 had higher levels of H. filipjevi resistance than their recurrent parent. However, Del6V#4L-1 and translocation line T6V#4S·6AL were equally susceptible to wheat cv. Chinese Spring. The CCN resistance gene, temporarily named CreV, was therefore physically mapped to chromosome arm 6V#4L FL 0.80–1.00. Translocation chromosomes T6V#4L·6AS transferred to a modern wheat cv. Aikang 58 with its co-dominant molecular markers could be utilized as a novel germplasm for CCN resistance breeding in wheat.
Similar content being viewed by others
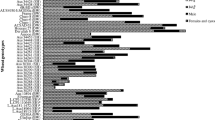
Avoid common mistakes on your manuscript.
Introduction
Cereal cyst nematodes (CCN) are major soilborne parasites that cause serious yield losses in cereal crops in many parts of the world (Nicol and Rivoal 2008). CCN damages the roots of barley, oats and wheat through invasion of root cells and establishment of feeding sites. Symptoms include excessive root branching, reduced plant vigor, small spikes and reduced yield. The Heterodera avenae group parasitizing cereals consists of 12 valid species; the three economically most important in cereal production are H. avenae, H. filipjevi and H. latipons (Nicol and Rivoal 2008). Effective management of this parasite in Australia demonstrated that control can be achieved through host resistance and maintenance of nematode populations below economically damaging thresholds (Ogbonnaya et al. 2009).
Genetic stocks with stable resistance to CCN are necessary for breeding resistant cultivars, but resistance genes are uncommon in Triticum aestivum. Only two CCN resistance genes have been identified from hexaploid wheat, namely Cre1 in AUS10894 (Williams et al. 1994) and Cre8 in cv. Festiguay (Paull et al. 1998; Jayatilake et al. 2015). Otherwise, the wild relatives of wheat are important sources of CCN resistance that can be transferred to cultivated wheat (Barloy et al. 2007). To date, nine Cre genes have been transferred from wild wheat relatives, including Cre2 (Delibes et al. 1993), Cre5 (Jahier et al. 1996, 2001) and Cre6 (Ogbonnaya et al. 2001) from Aegilops ventricosa, Cre3 and Cre4 from Aegilops tauschii (Eastwood et al. 1991, 1994), Cre7 from Aegilops triuncialis (Romero et al. 1998) and CreR from Secale cereale (Taylor et al. 1998). CreR was located on the long arm of chromosome 6R and its transfer to wheat was attempted by crossing a disomic substitution line 6R(6D) to the ph1bph1b Chinese Spring mutant line (Asiedu et al. 1990). Among resistance genes, Cre1 and Cre8 have been the most useful sources used in Australian wheat breeding programs (Murray and Brennan 2009), although their effectiveness depends on the particular race and CCN species (Nicol et al. 2009). For example, the Cre3 gene proved to be highly effective in Australia and moderately effective against H. avenae populations in Hebei province in China, but was not effective in Henan province (Nicol and Rivoal 2008; Peng et al. 2009). The known Cre genes are mostly H. avenae resistance genes, with only CreR and Cre1 being effective against H. avenae pathotype Ha13 in Australia and local populations of H. avenae in Henan, as well as against H. filipjevi in Turkey (Nicol et al. 2009). H. filipjevi is currently the most common and widespread CCN species occurring in dry-land winter wheat production regions of China and Turkey. The distribution of H. filipjevi has been demonstrated to be wider than previously documented, with losses in wheat in Turkey averaging 40 %, and is especially damaging under drought conditions (Nicol et al. 2009). Recent field micro-plot studies in China have also indicated its economic importance (Peng et al. 2009). Global warming and increased retention of maize-straw in farming systems will exacerbate the impact of this nematode in intensive production systems of the middle-east region in China (Peng et al. 2009). Of the published Cre genes, only CreR was found to provide high levels of H. filipjevi resistance in China, while the other genes tested (Cre1, Cre2, Cre3, Cre7 and Cre8) were found to be ineffective (Yuan et al. 2011). Recently, in a collection of 290 winter wheat accessions, 1 % of the wheat accessions were ranked as resistant and 16 % as moderately resistant to H. filipjevi (Pariyar et al. 2016). The restricted number of wheat germplasms with resistance to H. filipjevi therefore makes it necessary to find and transfer novel resistant genes from wild relatives.
Dasypyrum villosum (2n = 14, genomes VV), a cross-pollinated relative of bread wheat, has many agronomically important traits. Seven different D. villosum accessions have been introgressed into bread wheat (De Pace et al. 2011) and the beneficial alien genes located on chromosomes 1V, 2V, 4V, 5V and 6V were transferred into bread wheat by translocations (Chen et al. 1995; Zhang et al. 2005, 2010; Qi et al. 2011; Zhang et al. 2014, 2015). Zhang et al. (2012) tested a set of wheat–D. villosum addition lines for response to H. filipjevi and found that D. villosum and the chromosome 6V disomic addition line DA6V were both highly resistant. This indicated that D. villosum may be a valuable source of resistance to CCN in wheat. The present study describes the production of wheat–D. villosum introgression lines involving chromosome 6V and localization of a CCN resistance gene to the long arm of a T6V#4L·6AS chromosome.
Plant materials
The plant materials used in this study included T. aestivum cv. Chinese Spring and D. villosum accession GP005 (2n = 14, VV, the donor of the V chromatin in the present study). In order to distinguish this accession from others, the chromosomes of GP005 were numbered #4 by De Pace et al. 2011). Details of other lines, including T. durum cv. ZY1286–D. villosum amphiploid (2n = 42, AABBVV), T. aestivum–D. villosum disomic substitution line DS6V#4 (6A), translocation line T6V#4S·6AL, and other wheat–D. villosum genetic stocks, are given in Table 1. These materials were all developed and are maintained at the Cytogenetic Institute, Nanjing Agricultural University (CINAU). Seventy-six T. aestivum–D. villosum translocations involving chromosomes 1V#4 to 7V#4 developed by Bie et al. (2007) and Cao et al. (2009) were used in a search for those translocation lines carrying molecular markers on chromosome 6VL. Wheat cultivar Aikang 58 was used as a recurrent backcross parent for translocated chromosomes and cv. Wenmai 19 was used as a H. filipjevi-susceptible control.
In order to produce compensating Robertsonian translocations (RobTs) involving chromosomes 6A of wheat and 6V#4 of D. villosum, crosses were made between DS6V#4 (6A) and a homozygous ph1bph1b Chinese Spring mutant line. F1 plants with 42 chromosomes that were double monosomic for chromosomes 6A/6V were identified cytologically and self-pollinated. The F2 progeny were genotyped by molecular markers for putative RobTs.
Cytological procedures
Chromosome C-banding was conducted according to Gill et al. (1991). Genomic in situ hybridization (GISH) and fluorescence in situ hybridization (FISH) followed Zhang et al. (2004). The total genomic DNA of D. villosum labeled with fluorescein-12-dUTP was used to detect segments of chromosome 6V. Clone pSc119.2, a 120-bp tandem-repeat sequence from rye inserted in the plasmid pBR322 (Mukai et al. 1993) and labeled with digoxigenin-11-dUTP, was used for identification of the wheat B-genome chromosomes. Images of chromosomes were captured with an Olympus BX60 fluorescence microscope fitted with a SPOT Cooled Color Digital Camera (Diagnostic Instruments; http://www.diaginc.com).
PCR sequence-tagged site (STS) marker analyses
Three previously reported 6V#4 arm-specific expressed sequence tag (EST)-PCR markers, CINAU15 for 6V#4S and 6L-4 and WMC256 for 6V#4L, were used to identify translocations involving chromosome 6V (Table 2). GWM570 for chromosome 6A long arm was used to select homozygous translocations (Qi et al. 2011). A further seven 6VL-specific markers were selected from 53 EST-based primers to amplify different regions of wheat homeologous group-6L chromosomes. The primers were designed using Conserved Primers 2.0 software (Frank et al. 2009). Genomic DNA was isolated from young leaves using a DNA Secure Plant Kit (Tiangen Biotech Co., Ltd, Nanjing, China). The procedures for PCR amplification followed those of Zhang et al. (2015). The PCR products were separated on 8 % polyacrylamide gels in 1× TBE buffer.
CCN response assessment
Mass culture of CCN in a controlled greenhouse was used to screen the plant material over 3 years in order to determine response to a H. filipjevi Xuchang population. The procedures followed those of Zhang et al. (2012). Ten seedlings per line were planted individually in tubes (30 mm × 130 mm) filled with sterile soil and inoculated four times. After 75 days the number of white females of H. filipjevi per tube was counted. Relative resistance index (RRI) was used as the evaluation indicator (Zhang et al. 2012): RRI = [1 – (the mean number of white females per plant on a tested line/the mean number of white females per Wenmai 19 check plant)]. The scale of resistance was classified based on the RRI as: RRI = 1, immune (I); 0.9 ≤ RRI < 1.0, highly resistant (HR); 0.7 ≤ RRI < 0.9, resistant (R); 0.5 ≤ RRI < 0.7, moderately susceptible (MS); 0.3 ≤ RRI < 0.5, susceptible (S); RRI < 0.3, highly susceptible (HS).
Results
Development of chromosome 6VL-specific STS markers
Seven of the 53 STS primer pairs specifically identified the 6V#4 chromosome and polymorphism was detected among cv. Chinese Spring, T. durum cv. ZY1286 (AABB), T. durum cv. ZY1286–D. villosum amphiploid (AABBVV), and 6V chromosome disomic addition line DA6V#4. Further analysis revealed that all seven molecular markers were absent in T6V#4S·6AL; they were therefore assigned to chromosome arm 6V#4L (Table 1). Including two previously reported 6VL-specific markers, a total of nine loci on the long arm of chromosome 6V, including three located in the distal region, were used to detect 6V#4L segments in the 76 T. aestivum–D. villosum translocation lines.
Development and characterization of translocation lines
A total of 153 F2 plants derived from F1 plants double monosomic for 6V and 6A were screened with markers CINAU15 for 6V#4S and 6L-4 for 6V#4L. 6VL- or 6VS-specific polymorphic markers were observed in seven plants. Of them, four plants were positive for the 6VS marker and were highly resistant to powdery mildew, indicating that they were wheat-6VS Robertsonian translocations. The other three plants had only the 6V#4L marker and were susceptible to powdery mildew, suggesting that they involved misdivision products for 6V#4L. Homozygote line NAU423 was identified in F3 plants by GISH (GISH using Dasypyrum villosum genomic DNA labeled with digoxigenin-11-dUTP as probe and the alien chromatin in colour Fig. 1 is yellowish-green color). Mitotic and meiotic GISH patterns showed that NAU423 was a RobT line with 2n = 42, pairing as 21 bivalents at meiotic metaphase I (Fig. 1a, b). Sequential C-banding and GISH revealed that the opposite arm of the translocated chromosome in NAU423 did not have recognizable C-banding patterns, which indicated that the chromosome arm could belong to wheat A genome (Fig. 1g). Molecular markers analysis showed that the diagnostic band of 6V#4S-specific marker CINAU15 was absent in NAU423 (Fig. S1d), but all the diagnostic bands of nine 6V#4L-specific markers were present with simultaneous absence of 6AL-specific bands, indicating that this translocation chromosome could be T6V#4L·6AS (Fig. S1a, b and c).
Cytogenetic analyses of wheat–6V#4L introgression lines. GISH using D. villosum genomic DNA labeled with digoxigenin-11-dUTP as probe. D. villosum chromatin fluoresced with a yellowish–green color. a and b Mitotic and meiotic GISH patterns of NAU423 containing a pair of T6V#4L·6AS Robertsonian translocation chromosomes; c and d mitotic and meiotic GISH patterns of NAU424 containing a pair of T6V#4L-4BL·4BS translocation chromosomes. e Dual-color GISH/FISH patterns of NAU424. GISH used D. villosum DNA as probe (green) and B-genome chromosomes painted by the pSc119.2 probe (red) are marked. f Mitotic GISH patterns of NAU426 containing a pair of 6V#4L chromosome arms. g From left to right: C-banding pattern of 6V#4; sequential C-banding and GISH patterns of T6V#4L·6AS; h sequential C-banding and GISH patterns of T6V#4L-4BL·4BS translocated chromosome; i dual-color pSc119.2 FISH patterns of chromosome 4B and T6V#4L-4BL·4BS translocated chromosome. (Color figure online)
NAU424 (Fig. 1c) and NAU426 (Fig. 1f) were identified among the 76 introgression lines by molecular markers and GISH analysis. NAU424 was a small alien terminal translocation segment with its breakpoint at FL 0.80. The alien segment was the terminal part of 6VL and the diagnostic bands of three 6VL-specific molecular markers were present whereas the other six 6VL-specific molecular markers were not (Fig. 2). The standard FISH patterns for pSc119.2 established for the wheat cv. Chinese Spring by Mukai et al. (1993) were compared with the pSc119.2 pattern of NAU424 (Fig. 1e, i). The combined sequential C-banding and GISH patterns (Fig. 1h) showed that the translocation involved wheat chromosome arm 4BS and segment of chromosome arm 4BL. Chromosome at meiotic meiosis I (MI) configurations in pollen mother cells (PMCs) showed 21 ring or rod bivalents (Fig. 1d), among which one ring bivalent was formed by two wheat–D. villosum translocation chromosomes, indicating that this line was homozygous. Hence, NAU424 was a homozygous 6V#4L-4BL·4BS translocation line. NAU426 was a disomic telosomic addition line because all nine 6VL-specific markers were present whereas the 6VS-specific marker CINAU15 was absent (Fig. 2).
Characterization of resistance in homozygous translocation lines
Previous screening of a set of D. villosum disomic addition stocks from cv. Chinese Spring revealed that the disomic addition line DA6V#4 was resistant to H. filipjevi (Zhang et al. 2012). Homozygous lines T6V#4S·6AL (92R137), T6V#4L·6AS (NAU423), Del6V#4L-1 (92R112), T6V#4L·6VS-7BS (11R082) and DT6V#4L (NAU426) along with resistant DA6V#4 (11R274), susceptible recurrent parent cv. Chinese Spring and the highly susceptible cv. Wenmai 19 were tested for reaction to H. filipjevi (Table 3). T6V#4S·6AL and Del6V#4L-1 exhibited low RRIs similar to the susceptible parent, Chinese Spring. The other lines, including T6V#4L·6AS, DT6V#4L, T6V#4L·6VS-7BS and DA6V#4, showed significantly higher RRIs than their background parent in both years. All of these lines had the same alien whole arm, 6V#4L, suggesting that a CCN resistance gene was located on this chromosome arm. T6V#4L-4BL·4BS (NAU424) also exhibited a higher RRI (0.98) than cv. Chinese Spring (0.52) in the greenhouse experiment carried out in 2013. For further confirmation, the RRI was determined in 2014 for five homozygous T6V#4L·6AS lines and five homozygous T6V#4L-4BL·4BS lines derived from BC2F2 populations of crosses Aikang 58 × NAU423 or Aikang 58 × NAU424. All the translocation selections were resistant whereas Aikang 58 was susceptible. We therefore presumed that a resistance gene, temporarily named CreV was located in the alien segment, which represented FL 0.80–1.00 of 6V#4L. Roots of cv. Aikang 58 (Fig. S2d) infested by CCN showed more CCN eggs and abnormal branching than those of 14zrq7-29-1 (T6V#4L·6AS) (Fig. S2e), confirming the resistance attributable to CreV.
Discussion
Employing alien genes from the wheat secondary gene pool, such as D. villosum, is an effective way of increasing genetic diversity and improving cultivated wheat (Li et al. 2007). CreV is the first nematode resistance gene, discovered in D. villosum, that is effective against H. filipjevi. The integrated use of cytogenetic stocks, molecular markers, GISH, and FISH analyses, allowed the introgression of gene CreV from D. villosum into bread wheat by developing translocated chromosomes T6V#4L·6AS and T6V#4L-4BL·4BS, and this gene was mapped to 6VL-0.80-1.00. Qi et al. (2011) developed a compensating wheat–D. villosum RobT T6V#3L·6AS with a temperature-sensitive gene Sr52 resistant to stem rust race Ug99. In the present study, two wheat–D. villosum translocation lines, T6V#4L·6AS (NAU423) and T6V#4L-4BL·4BS (NAU424) with the H. filipjevi resistance gene, CreV, were developed and transferred into cv. Aikang 58. The chromosome arm 6VL in different accessions of D. villosum may carry different beneficial genes and the presence of Sr52 gene on 6V#3L and CreV on 6V#4L opens up the possibility of pyramiding the two genes into the same line by the crossing T6V#4L·6AS, CreV × T6V#3L·6AS, Sr52.
Host-plant resistance is the most effective method of controlling H. avenae and H. filipjevi. The compensating translocation 6V#4L·6AS present in the stock NAU423 has a H. filipjevi resistance gene, CreV, which may be useful in wheat improvement. After backcrossing twice, the T6V#4L·6AS translocation line and its recurrent parent Aikang 58 are very similar in their major agronomic characteristics, including plant height, spike length, seed weight, flowering and maturity stages (Fig. S2a, b and c). However, the detailed effects of T6V#4L·6AS translocated chromosomes on yield- and quality-related traits are currently unknown. Further research is also needed to confirm the value of this translocated chromosome in different wheat backgrounds against both H. filipjevi and H. avenae under field conditions.
Ionizing radiation has proved to be an efficient method of inducing chromosome translocation (Sears 1993). Seventy-six translocation lines involving 1V–7V chromosomes were developed by irradiating T. durum–D. villosum amphiploid (AABBV) pollen using a 60Co source (Bie et al. 2007; Cao et al. 2009), but most of them were non-compensating translocations because the chromosome breakages and rejoins produced by radiation are random. Two molecular markers (6L-4 and WMC256) allowed for the identification of a 6VL-translocation line NAU424 from the 76 lines of the translocation pool. Sequential C-banding analysis was used to identify the wheat chromosome arms involved in NAU424 translocations. The C-banding patterns preliminarily determined that the translocated chromosomes in NAU424 could belong to the B genome (Fig. 1h). Dual-color pSc119.2 FISH patterns can not only differentiate the wheat and D. villosum chromatin, but also determine the identities of particular chromosomes of the B genome. Referring to the standard FISH pattern for pSc119.2 (Mukai et al. 1993) established for wheat variety Chinese Spring, it could be concluded that the wheat chromosome involved in the translocation with 6V#4L in NAU424 was chromosome 4B (Fig. 1e, i). The terminal and internal pSc119.2 FISH patterns of the long arm of chromosome 4B were absent in the T6V#4L-4BL·4BS translocated chromosome, indicating that approximately 60 % of the long arm of chromosome 4B was substituted by approximately a 20 % segment of the long arm of chromosome 6V#4L in this translocated chromosome (Fig. 1i). The genes located on the missing segment of the long arm of chromosome 4B in NAU424 cannot be compensated by the alien chromatin of D. villosum. Thus, T6V#4L-4BL·4BS translocated chromosomes could have a negative effect on agronomic characteristics and fertility. After transferring T6V#4L-4BL·4BS translocated chromosomes into a modern wheat cultivar Aikang 58, ten lines involving this translocated chromosome were all vigorous but less fertile. The spikelet fertility of nine lines was approximately 70 %, while one line was less than 50 %. Therefore, the T6V#4L-4BL·4BS translocation line may not be used in wheat breeding except for physical mapping of the alien genes.
Due to the high cost and time involved in phenotypic evaluation of CCN resistance, molecular markers will be of great benefit for selection. Nine informative molecular markers which are sufficient to recover the alien chromosome arm 6 V#4L could be used for molecular-assisted selection. When using 6V#4L·6AS lines as resistant donors, co-dominant 6VL-specific EST-PCR markers that distinguish 6VL and 6AL can be used in agarose gel electrophoresis, as a convenient marker-assisted breeding assay. Of the nine 6VL-specific markers evaluated in this study. 6L-4, WMC256, CINAU933 and CINAU954 are easily used and co-dominant. In addition, the molecular markers developed in three bins of chromosome arm 6V#4L in the present study will not only facilitate the selection of T6V#4L·6AS translocated chromosomes, but also will be useful for screening the shortening 6V#4L segment retaining the CreV gene by ph1b-induced recombinants or ionizing radiation on T6V#4L·6AS translocated chromosomes.
In conclusion, the novel H. filipjevi resistance gene CreV was identified and physically mapped to chromosome bin 6VL-0.80–1.00. CreV is present in the translocation lines 6V#4L·6AS and 6V#4L-4BL·4BS, which were transferred to an adapted cv. Aikang 58 line. The co-dominant molecular markers developed are available for marker-assisted selection in breeding programs.
References
Asiedu R, Fisher JM, Driscoll CJ (1990) Resistance to Heterodera avenae in the rye genome of triticale. Theor Appl Genet 79:331–336
Barloy D, Lemoine J, Abelard P, Tanguy AM, Rivoal R, Jahier J (2007) Marker-assisted pyramiding of two cereal cyst nematode resistance genes from Anabaena variabilis in wheat. Mol Breed 20:31–40
Bie TD, Cao YP, Chen PD (2007) Mass production of intergeneric chromosomal translocatons through pollen irradiation of Triticum durum–Haynaldia villosa amphiploid. J Integr Plant Biol 49:1619–1626
Bie TD, Zhao RH, Jiang ZN, Gao DR, Zhang BQ, He HG (2015) Efficient marker-assisted screening of structural changes involving Haynaldia villosa chromosome 6 V using a double-distal-marker strategy. Mol Breed 35:34–43
Cao AZ, Wang XE, Chen YP, Zou XW, Chen PD (2006) A sequence-specific PCR marker linked with Pm21 distinguishes chromosomes 6AS, 6BS, 6DS of Triticum aestivum and 6VS of Haynaldia villosa. Plant Breed 125:201–205
Cao YP, Bie TD, Wang XE, Chen PD (2009) Induction and transmission of wheat–Haynaldia villosa chromosomal translocations. J Genet Genom 36:313–320
Chen PD, Qi LL, Zhou B, Zhang SZ, Liu DJ (1995) Development and molecular cytogenetic analysis of wheat–Haynaldia villosa 6VS/6AL translocation lines specifying resistance to powdery mildew. Theor Appl Genet 91:1125–1128
De Pace C, Vaccino P, Cionini G, Pasquini M, Bizzarri M, Qualset CO (2011) Dasypyrum. In: Kole C (ed) Wild crop relatives: genomic and breeding resources, cereals, vol 1, chapter 4. Springer, Heidelberg, pp 185–292
Delibes A, Romero D, Aguaded S, Duce A, Mena M, López-Braña I, Andrés MF, Martin-Sanchez JA, García-Olmedo F (1993) Resistance to the cereal cyst nematode (Heterodera avenae Woll.) transferred from the wild grass Aegilops ventricosa to hexaploid wheat by a ‘‘stepping-stone’’ procedure. Theor Appl Genet 87:402–408
Eastwood RF, Lagudah ES, Appels R, Hannah M, Kollmorgen J (1991) Triticum tauschii: a novel source of resistance to cereal cyst nematode (Heterodera avenae). Aust J Agric Res 42:69–77
Eastwood RF, Lagudah ES, Appels R (1994) A directed search for DNA sequences tightly linked to cereal cyst nematode resistance genes in Triticum tauschii. Genome 37:311–319
Frank MY, Naxin H, Yong QG, Gerard RL, Dvorak J, Anderson OD (2009) ConservedPrimers 2.0: a high-throughput pipeline for comparative genome referenced intron-flanking PCR primer design and its application in wheat SNP discovery. BMC Bioinform 10:331–341
Gill BS, Friebe B, Endo TR (1991) Standard karyotype and nomenclature system for description of chromosome bands and structural aberrations in wheat (Triticum aestivum). Genome 34:830–839
Jahier J, Tanguy AM, Abelard P, Rivoal R (1996) Utilization of deletions to localize a gene for resistance to the cereal cyst nematode, Heterodera avenae, on an Aegilops ventricosa chromosome. Plant Breed 115:282–284
Jahier J, Abelard P, Tanguy M, Dedryver F, Rivoal R, Khatkar S, Bariana HS, Koebner R (2001) The Aegilops ventricosa segment on chromosome 2AS of the wheat cultivar ‘VPM1’ carries the cereal cyst nematode resistance gene Cre5. Plant Breed 120:125–128
Jayatilake DV, Tucker EJ, Brueggemann J, Lewis J, Garcia M, Dreisigacker S, Hayden MJ, Chalmers K, Mather DE (2015) Genetic mapping of the Cre8 locus for resistance against cereal cyst nematode (Heterodera avenae Woll.) in wheat. Mol Breed 35:66–78
Li GP, Chen PD, Zhang SZ, Wang XE, He ZH, Zhang Y, Zhao H, Huang HY, Zhou XC (2007) Effects of the 6VS.6AL translocation on agronomic traits and dough properties of wheat. Euphytica 155:305–313
Mukai Y, Nakahara Y, Yamamoto M (1993) Simultaneous discrimination of the three genomes in hexaploid wheat by multicolor fluorescence in situ hybridization using total genomic and highly repeated DNA probes. Genome 36:489–494
Murray GM, Brennan JP (2009) The current and potential costs from diseases of wheat in Australia. Grains Research and Development Corporation, Canberra, Australia, https://grdc.com.au/Resources/Publications/2009/10
Nicol JM, Rivoal R (2008) Global knowledge and its application for the integrated control and management of nematodes on wheat. In: Ciancio A, Mukerji KG (eds) Integrated management and biocontrol of vegetable and grain crops nematodes. Springer Academic Publishing, Dordrecht, pp 243–287
Nicol JM, Ogbonnaya F, Singh AK, Bishnoi SP, Kanwar RS, Li HL, Chen SL, Peng DL, Bolat N, Şahin E, Elekçioğlu İH (2009) Current global knowledge of the usability of cereal cyst nematode resistant bread wheat germplasm through international germplasm exchange and evaluation. In: Riley IT, Nicol JM, Dababat AA (eds) Cereal cyst nematodes: status, research and outlook. CIMMYT, Ankara, pp 149–153
Ogbonnaya FC, Seah S, Delibes A, Jahier J, López-Braña I, Eastwood RF, Lagudah ES (2001) Molecular-genetic characterisation of a new nematode resistance gene in wheat. Theor Appl Genet 102:623–629
Ogbonnaya FC, Eastwood RF, Lagudah E (2009) Identification and utilization of genes for cereal cyst nematode resistance (Heterodera avenae) resistance in wheat: the Australian experience. In: Riley IT, Nicol JM, Dababat AA (eds) Cereal cyst nematodes: status, research and outlook. CIMMYT, Ankara, pp 166–171
Pariyar SR, Dababat AA, Siddique S, Erginbas-Orakci G, Elashry A, Morgounov A, Grundler FM (2016) Identification and characterization of resistance to the cereal cyst nematode Heterodera filipjevi in winter wheat. Nematology 18:377–402
Paull JG, Chalmers KJ, Karakousis A, Kretschmer JM, Manning S, Langridge P (1998) Genetic diversity in Australian wheat varieties and breeding material based on RFLP data. Theor Appl Genet 96:435–446
Peng DL, Nicol JM, Li HM, Hou SY, Li HX, Chen SL, Ma P, Li HL, Riley IT (2009) Current knowledge of cereal cyst nematode (Heterodera avenae) on wheat in China. In: Riley IT, Nicol JM, Dababat AA (eds) Cereal cyst nematodes: status, research and outlook. CIMMYT, Ankara, pp 29–34
Qi LL, Wang SL, Chen PD, Liu DJ, Gill BS (1998) Identification and physical mapping of three Haynaldia villosa chromosome-6 V deletion lines. Theor Appl Genet 97:1042–1046
Qi LL, Pumphrey MO, Friebe B, Zhang P, Qian C, Bowden RL, Rouse MN, Jin Y, Gill BS (2011) A novel Robertsonian translocation event leads to transfer of a stem rust resistance gene (Sr52) effective against race Ug99 from Dasypyrum villosum into bread wheat. Theor Appl Genet 123:159–167
Romero MD, Montes MJ, Sin E, López-Braña I, Duce A, Martn-Sanchez JA, Andrés MF, Delibes A (1998) A cereal cyst nematode (Heterodera avenae Woll.) resistance gene transferred from Aegilops triuncialis to hexaploid wheat. Theor Appl Genet 96:1135–1140
Sears ER (1993) Use of radiation to transfer alien chromosome segments to wheat. Crop Sci 33:897–901
Taylor C, Shepherd KW, Langridge P (1998) A molecular genetic map of the long arm of chromosome 6R of rye incorporating the cereal cyst nematode resistance gene, CreR. Theor Appl Genet 97:1000–1012
Williams KJ, Fisher JM, Langridge P (1994) Identification of RFLP markers linked to the cereal cyst nematode resistance gene (Cre) in wheat. Theor Appl Genet 89:927–930
Yuan HX, Zhang FX, Zhang JJ, Hou XS, Li HJ, Li HL (2011) Resistance of CIMMYT wheat germplasm to Heterodera filipjevi Xuchang population from Henan Province. Acta Agron Sin 37:1956–1966
Zhang P, Li WL, Friebe B, Gill BS (2004) Simultaneous painting of three genomes in hexaploid wheat by BAC-FISH. Genome 47:979–987
Zhang QP, Li Q, Wang XE, Wang HY, Lang SP, Wang Y, Wang SL, Chen PD, Liu DJ (2005) Development and characterization of a Triticum aestivum–Haynaldia villosa translocation line T4VS/4DL conferring resistance to wheat spindle streak mosaic virus. Euphytica 145:317–320
Zhang W, Gao AL, Zhou B, Chen PD (2006) Screening and applying wheat microsatellite markers to trace individual Haynaldia villosa chromosome. Acta Genetica Sinica 33:236–241
Zhang RQ, Cao YP, Wang XE, Feng YG, Chen PD (2010) Development and characterization of a Triticum aestivum–Dasypyrum villosum T5VS.5DL translocation line with soft grain texture. J Cereal Sci 51:220–225
Zhang JJ, Yuan HX, Zhang RQ, Xing XP, Dai JL, Niu JS, Li HL, Chen PD (2012) Analysis of resistance to Heterodera filipjevi in Triticum aestivum–Dasypyrum villosum germplasm. Acta Agron Sin 38:1–8
Zhang W, Zhang RQ, Feng YG, Bie TD, Chen PD (2013) Distribution of highly repeated DNA sequences in Haynaldia villosa and its application in the identification of alien chromatin. Chin Sci Bull 58:890–897
Zhang RQ, Zhang MY, Wang XE, Chen PD (2014) Introduction of chromosome segment carrying the seed storage protein genes from chromosome 1V of Dasypyrum villosum showed positive effect on bread-making quality of common wheat. Theor Appl Genet 127:523–533
Zhang RQ, Hou F, Feng YG, Zhang W, Zhang MY, Chen PD (2015) Characterization of a Triticum aestivum–Dasypyrum villosum T2VS·2DL translocation line expressing a longer spike and more kernels traits. Theor Appl Genet 128:2415–2425
Acknowledgments
This study was supported by the National Natural Science Foundation of China (Project no. 30871519) and The 863 High-tech Program (Project no. 2012AA101105). The authors would like to thank Dr. Ian Riley, Australia and Prof. Bob McIntosh, the University of Sydney Plant Breeding Institute Cobbitty, NSW, Australia, for their comments on the manuscript.
Author contributions
Conceived and designed the experiments: Ruiqi Zhang; Developed translocation lines NAU424 and NAU426: Yigao Feng; Developed the translocation line NAU423: Haifeng Li; Identified the CCN resistance: Hongxia Yuan, Junli Dai and Honglian Li; Wrote the paper: Ruiqi Zhang, Aizhong Cao and Liping Xing.
Author information
Authors and Affiliations
Corresponding authors
Ethics declarations
Conflict of interest
All the authors have no conflicts of interest and agree with publication.
Electronic supplementary material
Below is the link to the electronic supplementary material.
Supplemental Figure S1
PCR patterns of markers in cv. Chinese Sprint, D. villosum, Triticum durum cv. ZY1286–D. villosum amphiploid (AABBVV), T. aestivum–D. villosum disomic substitution line DS6V#4 (6A), translocation line T6V#4S·6AL, homozygous translocation lines NAU423 and NAU424 and nullisomic 6A–tetrasomic 6B (N6AT6B). The straight line (right) indicates the fragments that can be assigned to a certain chromosome. a PCR pattern of 6L-4. b PCR pattern of SSR marker WMC256. c PCR pattern of SSR marker GWM570. d PCR pattern of CINAU15. Translocation line NAU423 harbors the 6VL- and 6AS-specific fragments, but is missing the 6VS-specific and 6AL-specific fragments, indicating they are homozygous for T6V#4S·6AL. (TIFF 1082 kb)
Supplemental Figure S2
a Plants of T6V#4S·6AL translocation line 14zrq7-29-1 (left) and Aikang 58 (right). b Spikes of T6V#4S·6AL translocation line 14zrq7-29-1 (left) and Aikang 58 (right. c Seeds of T6V#4S·6AL translocation line 14zrq7-29-1 (left) and Aikang 58 (right). d Root phenotype of Aikang 58 after infected with H. filipjevi; arrow indicates the CCN eggs attached on the root resulting in abnormal branching. e Root phenotype of T6V#4S·6AL translocation line 14zrq7-29-1 after infection with H. filipjevi. (TIFF 2644 kb)
Rights and permissions
About this article
Cite this article
Zhang, R., Feng, Y., Li, H. et al. Cereal cyst nematode resistance gene CreV effective against Heterodera filipjevi transferred from chromosome 6VL of Dasypyrum villosum to bread wheat. Mol Breeding 36, 122 (2016). https://doi.org/10.1007/s11032-016-0549-9
Received:
Accepted:
Published:
DOI: https://doi.org/10.1007/s11032-016-0549-9