Abstract
Few reports are available on the monitoring of regional cerebral oxygen saturation (rSO2) in pediatric patients undergoing non-cardiac surgical procedures. In addition, no study has examined the rSO2 levels in children of a broad age range. In this study, we aimed to assess and compare rSO2 levels in pediatric patients of different age groups undergoing non-cardiac surgery. We used two oximeters, tNIRS-1, which uses time-resolved spectroscopy, and conventional INVOS 5100C. Seventy-eight children—26 infants, 26 toddlers, and 26 schoolchildren—undergoing non-cardiac surgery were included. We investigated the differences in the rSO2 levels among the age groups and the correlation between the models and physiological factors influencing the rSO2 values. rSO2 measured by INVOS 5100C was significantly lower in infants than those in other patients. rSO2 measured by tNIRS-1 was higher in the toddler group than those in the other groups. The rSO2 values of tNIRS-1 and INVOS 5100C were moderately correlated (r = 0.41); however, those of INVOS 5100C were approximately 20% higher, and a ceiling effect was observed. The values in INVOS 5100C and tNIRS-1 were affected by blood pressure and the minimum alveolar concentration of sevoflurane, respectively. In pediatric patients undergoing non-cardiac surgery, rSO2 values differed across the three age groups, and the pattern of these differences varied between the two oximeters employing different algorithms. Further research must be conducted to clarify cerebral oxygenation in children.
Similar content being viewed by others
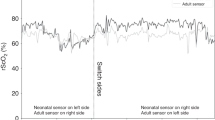
Explore related subjects
Discover the latest articles, news and stories from top researchers in related subjects.Avoid common mistakes on your manuscript.
1 Introduction
Near-infrared spectroscopy (NIRS) is used for monitoring regional cerebral oxygen saturation (rSO2). rSO2 monitoring is a non-invasive continuous measurement method and has been reported to be useful in pediatric cardiac surgery [1]. However, very few reports are available regarding the use of NIRS in pediatric non-cardiac surgery [2].
Some reports have studied the differences in rSO2 values due to children’s growth. Dullenkopf et al. examined rSO2 in 30 pediatric surgery patients using INVOS 5100 (Somanetics, Troy, MI, USA) and reported that children weighing < 40 kg had lower rSO2 than that of those weighing > 40 kg [3]. In another study, the changes in rSO2 during slow induction with sevoflurane were examined in children under 2 years of age using INVOS 5100C. In that study, the increase in rSO2 after induction was approximately 10% lower in children under 6 months of age than in those over 6 months of age [4]. However, no study, as far as we know, has divided children over a wide range of ages into several age groups and compared their rSO2 levels.
The NIRS includes several algorithms, such as the modified Beer–Lambert (MBL) law, spatially resolved spectroscopy (SRS), and time-resolved spectroscopy (TRS) [5]. The TRS method can calculate the optical path length using short pulse light. Thus, this method enables the direct extraction of the absolute oxygenated hemoglobin and deoxygenated hemoglobin values for the brain. In addition, the effect of extracranial blood flow, bone, and cerebrospinal fluid layer (also known as extracranial contamination) is lesser with the TRS method than that with the MBL and SRS methods [6]. Therefore, the TRS method is considered one of the most accurate and reproducible measurement algorithms, as we described in previous reports [7].
Some reports have compared the rSO2 measured with two or more oximeters in newborns undergoing non-cardiac surgery [8, 9]. However, to the best of our knowledge, such studies included only newborns and did not perform comparisons using the TRS method.
Therefore, this study divided children aged 0–12 undergoing non-cardiac surgery into three groups (infants: 0 years old, toddlers: 1–6 years old, schoolchildren: 7–12 years old) and examined the difference in the rSO2 values among the three age groups using two models of oximeters: tNIRS-1 (Hamamatsu Photonics, Hamamatsu, Japan), which uses the TRS method, and INVOS 5100C, which uses the SRS method and is the most widely used model. In addition, as an exploratory study, we examined the correlation between the rSO2 values obtained by tNIRS-1 and INVOS 5100C. Furthermore, we also examined the physiological factors that affect the rSO2 value obtained using each model. Our hypotheses are that the rSO2 values are different among the three age groups and that the pattern of these differences varies between the two oximeters.
2 Materials and methods
2.1 Participants
This prospective observational study was conducted with the approval of the Hokkaido University Hospital Voluntary Clinical Research Review Board (approval number: ji-017-0137). It was registered with the UMIN Clinical Trials Registry (UMIN000030799) prior to the commencement of the study. The study was conducted in accordance with the Declaration of Helsinki and relevant regulations. Written informed consent was obtained from all participants’ representatives.
The participants were pediatric patients aged 12 years or younger with American Society of Anesthesiologists physical status classification (ASA-PS) 1–2 who were scheduled to undergo non-cardiac surgery under general anesthesia at our hospital. The blood hemoglobin (Hb) concentration of the participants had been measured within 1 week before the surgery. Patients with abnormalities in the brain, cardiovascular, or respiratory organs and those with hemorrhage or marked anemia were excluded.
2.2 Measurement procedure
General anesthesia was administered through slow or rapid induction. For slow induction, anesthesia was induced with 3–5% sevoflurane and 1–5 µg/kg of fentanyl. Subsequently, 0–0.9 mg/kg of rocuronium was administered, followed by laryngeal mask insertion or tracheal intubation. For rapid induction, anesthesia was induced with 2–2.5 mg/kg of propofol or 4–6 mg/kg of thiamylal and 1–5 µg/kg of fentanyl. Laryngeal mask insertion or tracheal intubation was performed after the administration of 0–0.9 mg/kg of rocuronium. In most cases, 2–4 min elapsed between the start of anesthetic administration and intubation (insertion). Thereafter, sevoflurane and remifentanil were maintained at 0.5–1.5 minimum alveolar concentration (MAC) and 0.1–0.3 µg/kg/min, respectively, using the vaporizer dials. The MAC level was determined based on a report by Lerman et al. [10]. The fraction of inspired oxygen (FiO2) was set to 45–50% by adding air immediately after intubation.
Immediately after securing the airway with the instrument, INVOS 5100C and tNIRS-1 probes were attached to the frontal region. The INVOS 5100C probe for newborns was used for patients weighing < 5 kg, the probe for children was used for patients weighing 5–40 kg, and the probe for adults was used for patients weighing > 40 kg. In half of the expected participants of each group, the INVOS 5100C probe was attached on the left side, and the tNIRS-1 probe was attached on the right side of the frontal region. In the other half, the position of the respective probes was switched. The end-tidal CO2 (ETCO2) was confirmed to be within 35–45 mmHg and more than 5 min after securing the airway with an instrument (at least 7–9 min after the start of anesthetic administration), rSO2 was continuously monitored using INVOS 5100C for 1 min, while waiting for the measurement values to stabilize, and the final value was recorded. Subsequently, the power to INVOS 5100C was turned off to avoid interference between two oximeters, and rSO2 was measured with tNIRS-1 for 1 min, and the final value was recorded. The power to tNIRS-1 was then turned off, and the measurement by INVOS 5100C and tNIRS-1 was similarly repeated. The average value of the two measurements was considered the respective rSO2 value.
Non-invasive blood pressure (NIBP), heart rate (HR), oxygen saturation of peripheral artery (SpO2), FiO2, ETCO2, and end-tidal sevoflurane concentration (ETsevo) were monitored every minute and recorded using an automated anesthesia recording system (Mirrel version 3.4.1.24728(19), FUKUDA DENSHI, Tokyo). After confirming the absence of fluctuations, the last value of the measurement was extracted. The most recent preoperative blood Hb value was recorded. ETsevo was converted into age-adjusted MAC [11].
2.3 Outcomes and statistical analysis
The rSO2 value was 78.6 ± 6.1% according to a previous study evaluating rSO2 measured by INVOS 5100C on the first day of life in full-term newborns [8]. A 20% reduction in rSO2 from baseline has been suggested to be associated with hypoxia; thus, a 10% difference (7.9%) was hypothesized to be clinically meaningful [12, 13]. Assuming the same dispersion as in other age groups, a sample size of at least 18 cases was required to ensure a significance level of 0.05/3 based on the Bonferroni correction and a power of 90%. The sample size was, at first, determined as 60 cases in total, with 20 cases in each of the three groups: 0 years old (infants), 1–6 years old (toddlers), and 7–12 years old (schoolchildren). However, after measuring 20 cases, the standard deviation of rSO2 was larger than the initial estimate; therefore, six cases were added to each group, resulting in a total of 78 cases.
The primary endpoint was the presence or absence of differences in the rSO2 values for each model among the three age groups (infants, toddlers, and schoolchildren). Considering that the anesthetic induction method (slow or rapid) may influence rSO2 values [14, 15], we also compared the rSO2 values between the two induction methods. If the induction methods influenced the results, we adjusted for them and determined the least squares mean by using multiple regression analysis. As an exploratory study, we also examined the correlation between the rSO2 values measured with INVOS 5100C and tNIRS-1. Furthermore, to determine the influencing factors in each model, we investigated the correlation between the intraoperative parameters (Hb, NIBP, HR, SpO2, FiO2, ETCO2, and MAC) and rSO2 at the end of rSO2 measurement.
During the comparison of rSO2 among the different age groups, multiple comparisons were performed using the Steel–Dwass test, as the measured values obtained using INVOS 5100C had a ceiling effect. Similarly, the rSO2 values between different anesthetic induction methods were analyzed using the Steel–Dwass test. Spearman’s correlation analysis was used for the correlation analysis of continuous variables [16]. We also examined the differences between the two rSO2 measurement instruments using the Bland–Altman plot.
For comparison of the patient data and intraoperative measurement items except for sex and ASA classification, Tukey’s HSD method was used for parametric data and Steel–Dwass test for nonparametric data. Statistical significance was set at p < 0.05. Chi-square test was used to compare the sex distribution and ASA classification among the patients, and significance based on the Bonferroni correction was set at p < 0.05/3. JMP® Pro14 (SAS Institute, North Carolina, USA) was used for statistical analysis.
3 Results
3.1 Patient background and intraoperative parameters
Table 1 shows the background and intraoperative parameters of the 78 patients. The mean NIBP differed significantly among the groups, and multiple comparisons showed that schoolchildren had significantly higher NIBP than those of the other groups. The preoperative Hb levels were significantly higher in schoolchildren than those in infants. MAC did not differ significantly among the age groups. Regarding the anesthetic induction methods, the numbers of patients who underwent rapid induction with intravenous anesthetics varied among the age groups, with the highest number among schoolchildren.
3.2 Changes in rSO2 with age
The rSO2 values differed according to the age group, whereas the pattern of the difference differed according to the model. Table 2 and Fig. 1 show the difference in rSO2 according to the age group in each model. rSO2 measured using INVOS 5100C was significantly lower in infants than those in the other two groups (vs. toddlers: p = 0.0085, vs. schoolchildren: p = 0.0082). In contrast, rSO2 measured using tNIRS-1 was significantly higher in toddlers than those in the other groups (vs. infants: p < 0.0001, vs. schoolchildren: p < 0.0001). No significant difference was observed between infants and schoolchildren (p = 0.8799).
When we evaluated the rSO2 values according to the anesthetic induction method in all the children, the rSO2 value of the rapid-induction group in which intravenous anesthetics were used was approximately 5% lower when measured using tNIRS-1, although this difference was not statistically significant (p = 0.0791, Fig. 2). The values were almost the same when measured using INVOS 5100C (Fig. 2). In response to these results, we adjusted the rSO2 values according to the anesthetic method among different age groups and determined the least squares mean. The results are summarized in Table 3. This tendency was the same as that observed before adjusting.
Comparison of the rSO2 values between anesthetic induction methods in all the children. ♢: Mean, center line of box: median, top and bottom of the box: 25th and 75th percentile values, respectively; top and bottom of the whiskers: 10th and 90th percentile values, respectively. The Steel–Dwass test was used for comparisons among the induction groups. *: p < 0.05, Means ± SD by groups: INVOS 5100C Slow: 88.9% ± 0.9%, INVOS 5100C Rapid: 89.0% ± 5.9%, tNIRS-1 Slow: 71.1% ± 0.9%, tNIRS-1 Rapid: 66.7% ± 1.3%
3.3 Correlation between tNIRS-1 and INVOS 5100C
A moderate correlation was observed between the rSO2 values obtained using INVOS 5100C and tNIRS-1 in all the children (r = 0.41, p = 0.0002, Fig. 3). However, when stratified according to age, no significant correlations were observed among infants and toddlers (Fig. 4). As previously reported, the ceiling effect was observed in the measured values of INVOS 5100C. Bland–Altman analysis revealed that the measurements of rSO2 obtained using INVOS 5100C were higher than those obtained using tNIRS-1, with a mean difference of approximately 20% between the two models (Figs. 3, 4).
Correlations between the rSO2 values (upper) and Bland–Altman plot (lower) measured using tNIRS-1 and INVOS 5100C for each age group. r: Spearman’s rank correlation coefficient. The dotted line in the Bland–Altman plot shows the average values of the differences: infants: 18.9%, toddlers: 15.2%, schoolchildren: 23.3%
3.4 Correlation between rSO2 and physiological indicators in each model
Figure. 5 shows the correlation diagram between the physiological indicators and rSO2. The mean NIBP showed a positive correlation with INVOS 5100C only and no correlation with tNIRS-1 (r = 0.297, p = 0.0082). MAC also showed a correlation only with tNIRS-1. SpO2 was correlated with both INVOS 5100C and tNIRS-1; however, SpO2 values were almost 99–100%, suggesting little physiological significance.
4 Discussion
This study measured the rSO2 values of children undergoing non-cardiac surgery using two models with different measurement algorithms and found that the rSO2 values varied according to the age group, and the pattern of the differences varied according to the model. A moderate correlation was observed between tNIRS-1 and INVOS 5100C. A difference in the physiological indicators that affected the rSO2 levels measured using INVOS 5100C and tNIRS-1 was also observed.
Previous studies that used INVOS 5100C reported lower rSO2 levels in younger children [3, 4]; consistent with these findings, the present study also observed significantly lower rSO2 levels in infants compared with the other age groups using INVOS 5100C. This finding may be attributed to the physiological indicators. rSO2 is affected by the arterial oxygen saturation (SaO2), cerebral blood flow (CBF), Hb, and cerebral metabolic rate for oxygen (CMRO2) [17]. A comparison of the physiological indicators among the three groups showed no significant difference in SpO2, which reflects SaO2, or MAC, which further reflects CBF and CMRO2 [18, 19]. No significant difference was observed in ETCO2, which affects CBF [20]. However, blood pressure and Hb were significantly lower in infants than those in other age groups. CBF might have been influenced when blood pressure fell below the lower limit of cerebral autoregulation. Conversely, if blood pressure was above the lower limit of cerebral autoregulation, rSO2 might have reflected variations in extracranial blood flow due to blood pressure. Two previous reports demonstrated that rSO2 measured using INVOS 5100C decreased by more than 15% after the inflation of the head cuff in adults [6, 21], whereas the decrease after inflation was less than 10% in tNIRS-1 [6]. This suggests that the impact of extracranial contamination might be more pronounced with INVOS 5100C than with tNIRS-1. Regarding Hb, our previous study indicated that changes in rSO2 were more influenced by Hb alterations in INVOS 5100C compared to tNIRS-1 [22]. Consequently, both low blood pressure and Hb levels may be associated with reduced rSO2 measured using INVOS 5100C in infants.
Meanwhile, the rSO2 levels measured using tNIRS-1 were significantly higher in toddlers than those in the other groups. However, this result cannot be attributed to the physiological indicators examined in the present study. There have been no reports of transient high rSO2 in early childhood. However, regarding the relationship between age and CBF, our results may be explained by the following reports. It has been reported that the combined total CBF (total blood flow) of the internal carotid and vertebral arteries increases significantly between the ages of 3 and 6.5 years and then decreases to a certain level [23]. Another study showed that the flow velocity of the basal cerebral arteries measured using transcranial Doppler ultrasonography peaked at the age of 5–6 years and decreased to 70% at the age of 18 years [24]. Recent studies have also shown that the total CBF measured using magnetic resonance imaging increases significantly from 7 months to 6 years of age and then decreases [25]. Our results on tNIRS-1 (transition of rSO2 by age group) are fairly consistent with the results of these studies.
In our study, general anesthesia was administered through slow or rapid induction. For example, when 2 mg/kg of propofol was administered at the induction, the plasma concentration reached a therapeutic range within 8 min [14]. Accordingly, the difference of induction method may affect rSO2 values. Indeed, Kondo et al. reported a lower rSO2 in the 4 min following propofol induction than that following sevoflurane induction in adults [15], possibly due to the decrease in cerebral blood flow caused by propofol. In our study, although we measured rSO2 at least 7–9 min after the start of the anesthetic administration, the rSO2 values in the rapid-induction group were approximately 5% lower when measured using tNIRS-1, similar to the previous report. However, after adjusting the rSO2 values according to the anesthetic method in each age group, the results were similar to those before adjusting.
Thus, INVOS 5100C and tNIRS-1 showed different patterns of rSO2 changes according to age. Further research is needed to define which model is the gold standard (i.e., which model obtained the correct values). Anyway, this study revealed that rSO2 changes with age and that the transition differs with the model, that is, the measurement algorithm.
Our study found a moderate correlation between the two models but not for infants and toddlers. In addition, INVOS 5100C showed a ceiling effect, and several patients showed the 95% maximum value. Past studies have also reported the ceiling effect of INVOS 5100C. However, considering that INVOS 5100C assumes that the ratio of arteries and veins in the regional brain tissue is 25:75, the values obtained by the model are unlikely to be correct [16, 26]. In that respect, tNIRS-1 has no ceiling effect and seems useful even in the high rSO2 range. The correlation remained moderate; however, if this ceiling effect of INVOS 5100C was eliminated, the correlation coefficient may have been higher. The SO2 values differed by approximately 20% between the two models. Previously, similar results have been reported for healthy adults, with tNIRS-1 yielding approximately 10% lower SO2 values than INVOS 5100C [6, 22]. This suggests that the degree of difference is larger in children than in adults. The reason for the difference is unknown, but it may be attributed to differences between the algorithms. In addition, considering that no significant correlations were observed among infants and toddlers, the differences in the algorithms may become more pronounced in the lower age.
The influencing physiological indicators also differed between tNIRS-1 and INVOS 5100C. A correlation with NIBP was only noted for INVOS 5100C, whereas the correlation with MAC was only noted for tNIRS-1. Recent studies on cerebral autoregulation in children have reported that full-term infants have normal cerebral autoregulation, whereas cerebral autoregulation is incomplete in preterm infants [27]. In this study, as almost all babies were full-term, it can be inferred that they had intact cerebral autoregulation. Although the lower limit of cerebral autoregulation in children is not well understood, a possible reason why the rSO2 of INVOS 5100C depended on NIBP was that the blood pressure might have been lower than the lower limit during measurement [28]. However, assuming that the blood pressure was within the capacity of cerebral autoregulation, it might have been affected by extracranial blood flow, which is more dependent on blood pressure [21]. The reason why rSO2 of tNIRS-1 was not dependent on blood pressure but dependent on MAC may be that blood pressure at measurement was within the capacity of cerebral autoregulation and purely reflected the changes in CBF and CMRO2. Sevoflurane increases rSO2 above 0.5 MAC due to the decrease in CMRO2 and the increase in CBF [29, 30]. In the present study, sevoflurane had a MAC of 0.71 ± 0.16 (mean ± standard deviation), which is consistent with a positive correlation. In addition, this study revealed that the rSO2 values measured using tNIRS-1 were affected by the induction method (rapid vs. slow). Therefore, tNIRS-1 may be more sensitive to changes in cerebral circulation caused by anesthetics than INVOS 5100C.
The present study had some limitations. The results were obtained through a single measurement; hence, further studies should ideally investigate changes over time. Secondly, we did not investigate children with brain or heart problems. Furthermore, since it is relatively difficult to conduct measurements at rest while children are awake, the present study was limited to the comparison of rSO2 under general anesthesia. Nonetheless, this study’s novelty lies in the examination of rSO2 of children of varying ages who do not have brain or heart damage according to a wide range of age groups and performed using two oximeters with different algorithms, one of which is TRS.
5 Conclusions
The rSO2 of children aged 0–12 years without serious illness, such as heart disease, was measured using tNIRS-1, which employs TRS, and compared with that measured using INVOS 5100C. The rSO2 value differed according to the age group, whereas the pattern of the difference varied according to the model. The two models showed a moderate correlation; however, no significant correlations were observed in children below school age. The value measured using INVOS 5100C was approximately 20% higher, and a ceiling effect was observed. INVOS 5100C was affected by blood pressure, whereas tNIRS-1 was affected by the MAC of sevoflurane. Although non-invasive and continuous monitoring is a great clinical advantage of NIRS, its usefulness in children remains unclear. Further research should be conducted in the future to clarify the state of cerebral oxygenation in children.
References
Zaleski KL, Kussman BD. Near-infrared spectroscopy in pediatric congenital heart disease. J Cardiothorac Vasc Anesth. 2020;34:489–500. https://doi.org/10.1053/j.jvca.2019.08.048.
Weber F, Scoones GP. A practical approach to cerebral near-infrared spectroscopy (NIRS) directed hemodynamic management in noncardiac pediatric anesthesia. Paediatr Anaesth. 2019;29:993–1001. https://doi.org/10.1111/pan.13726.
Dullenkopf A, Frey B, Baenziger O, Gerber A, Weiss M. Measurement of cerebral oxygenation state in anaesthetized children using the INVOS 5100 cerebral oximeter. Paediatr Anaesth. 2003;13:384–91. https://doi.org/10.1046/j.1460-9592.2003.01111.x.
Rhondali O, Juhel S, Mathews S, Cellier Q, Desgranges FP, Mahr A, De Queiroz M, Pouyau A, Rhzioual-Berrada K, Chassard D. Impact of sevoflurane anesthesia on brain oxygenation in children younger than 2 years. Paediatr Anaesth. 2014;24:734–40. https://doi.org/10.1111/pan.12397.
Yoshitani K, Kawaguchi M, Ishida K, Maekawa K, Miyawaki H, Tanaka S, Uchino H, Kakinohana M, Koide Y, Yokota M, Okamoto H, Nomura M. Guidelines for the use of cerebral oximetry by near-infrared spectroscopy in cardiovascular anesthesia: a report by the Cerebrospinal Division of the Academic Committee of the Japanese Society of Cardiovascular Anesthesiologists (JSCVA). J Anesth. 2019;33:167–96. https://doi.org/10.1007/s00540-019-02610-y.
Kato S, Yoshitani K, Kubota Y, Inatomi Y, Ohnishi Y. Effect of posture and extracranial contamination on results of cerebral oximetry by near-infrared spectroscopy. J Anesth. 2017;31:103–10. https://doi.org/10.1007/s00540-016-2275-1.
Kubo Y, Kubo T, Toki T, Yokota I, Morimoto Y. Effects of ephedrine and phenylephrine on cerebral oxygenation: observational prospective study using near-infrared time-resolved spectroscopy. J Clin Monit Comput. 2023;37:1171–7. https://doi.org/10.1007/s10877-023-01036-y.
Szczapa T, Karpinski L, Moczko J, Weindling M, Kornacka A, Wroblewska K, Adamczak A, Jopek A, Chojnacka K, Gadzinowski J. Comparison of cerebral tissue oxygenation values in full term and preterm newborns by the simultaneous use of two near-infrared spectroscopy devices: an absolute and a relative trending oximeter. J Biomed Opt. 2013;18:87006. https://doi.org/10.1117/1.JBO.18.8.087006.
Ziehenberger E, Urlesberger B, Binder-Heschl C, Schwaberger B, Baik-Schneditz N, Pichler G. Near-infrared spectroscopy monitoring during immediate transition after birth: time to obtain cerebral tissue oxygenation. J Clin Monit Comput. 2018;32:465–9. https://doi.org/10.1007/s10877-017-0052-9.
Lerman J, Sikich N, Kleinman S, Yentis S. The pharmacology of sevoflurane in infants and children. Anesthesiology. 1994;80:814–24. https://doi.org/10.1097/00000542-199404000-00014.
Mapleson WW. Effect of age on MAC in humans: a meta-analysis. Br J Anaesth. 1996;76:179–85. https://doi.org/10.1093/bja/76.2.179.
Hoffman GM, Ghanayem NS, Tweddell JS. Noninvasive assessment of cardiac output. Semin Thorac Cardiovasc Surg Pediatr Card Surg Annu. 2005. https://doi.org/10.1053/j.pcsu.2005.01.005.
Gómez-Pesquera E, Poves-Alvarez R, Martinez-Rafael B, Liu P, Alvarez J, Lorenzo-López M, Fierro I, Gómez-Sánchez E, Heredia-Rodriguez M, Gómez-Herreras JI, Tamayo E. Cerebral oxygen saturation and negative postoperative behavioral changes in pediatric surgery: a prospective observational study. J Pediatr. 2019;208:207–13.e1. https://doi.org/10.1016/j.jpeds.2018.12.047.
Vuyk J, Sitsen E, Reekers M. Intravenous anesthetics. In: Gropper MA, editor. Miller’s anesthesia. 9th ed. Amsterdam: Elsevier; 2019. p. 639–79.
Kondo Y, Hirose N, Maeda T, Suzuki T, Yoshino A, Katayama Y. Changes in cerebral blood flow and oxygenation during induction of general anesthesia with sevoflurane versus propofol. Adv Exp Med Biol. 2016;876:479–84. https://doi.org/10.1007/978-1-4939-3023-4_60.
Hessel TW, Hyttel-Sorensen S, Greisen G. Cerebral oxygenation after birth—a comparison of INVOS((R)) and FORE-SIGHT near-infrared spectroscopy oximeters. Acta Paediatr. 2014;103:488–93. https://doi.org/10.1111/apa.12567.
Yagi Y, Yamamoto M, Saito H, Mori T, Morimoto Y, Oyasu T, Tachibana T, Ito YM. Changes of cerebral oxygenation in sequential glenn and Fontan procedures in the same children. Pediatr Cardiol. 2017;38:1215–9. https://doi.org/10.1007/s00246-017-1647-0.
Summors AC, Gupta AK, Matta BF. Dynamic cerebral autoregulation during sevoflurane anesthesia: a comparison with isoflurane. Anesth Analg. 1999;88:341–5. https://doi.org/10.1097/00000539-199902000-00022.
Gupta S, Heath K, Matta BF. Effect of incremental doses of sevoflurane on cerebral pressure autoregulation in humans. Br J Anaesth. 1997;79:469–72. https://doi.org/10.1093/bja/79.4.469.
Mariappan R, Mehta J, Chui J, Manninen P, Venkatraghavan L. Cerebrovascular reactivity to carbon dioxide under anesthesia: a qualitative systematic review. J Neurosurg Anesthesiol. 2015;27:123–35. https://doi.org/10.1097/ANA.0000000000000092.
Davie SN, Grocott HP. Impact of extracranial contamination on regional cerebral oxygen saturation: a comparison of three cerebral oximetry technologies. Anesthesiology. 1996;116:834–40. https://doi.org/10.1097/ALN.0b013e31824c00d7.
Yagi Y, Kubo Y, Hoshino K, Okada K, Hotta K, Shinohara N, Morimoto Y. Differences of cerebral oxygen saturation in dialysis patients: a comparison of three principals of near infrared spectroscopy. J Anesth. 2023;37:861–7. https://doi.org/10.1007/s00540-023-03245-w.
Schoning M, Hartig B. Age dependence of total cerebral blood flow volume from childhood to adulthood. J Cereb Blood Flow Metab. 1996;16:827–33. https://doi.org/10.1097/00004647-199609000-00007.
Bode H, Wais U. Age dependence of flow velocities in basal cerebral arteries. Arch Dis Child. 1988;63:606–11. https://doi.org/10.1136/adc.63.6.606.
Wu C, Honarmand AR, Schnell S, Kuhn R, Schoeneman SE, Ansari SA, Carr J, Markl M, Shaibani A. Age-related changes of normal cerebral and cardiac blood flow in children and adults aged 7 months to 61 years. J Am Heart Assoc. 2016;5:e002657. https://doi.org/10.1161/JAHA.115.002657.
Bickler PE, Feiner JR, Rollins MD. Factors affecting the performance of 5 cerebral oximeters during hypoxia in healthy volunteers. Anesth Analg. 2013;117:813–23. https://doi.org/10.1213/ANE.0b013e318297d763.
Rhee CJ, da Costa CS, Austin T, Brady KM, Czosnyka M, Lee JK. Neonatal cerebrovascular autoregulation. Pediatr Res. 2018;84:602–10. https://doi.org/10.1038/s41390-018-0141-6.
Armstead WM. Cerebral blood flow autoregulation and dysautoregulation. Anesthesiol Clin. 2016;34:465–77. https://doi.org/10.1016/j.anclin.2016.04.002.
Cooter M, Ni K, Thomas J, Gupta DK, Hopkins TJ, Miller TE, James ML, Kertai MD, Berger M. Age-dependent decrease in minimum alveolar concentration of inhaled anaesthetics: a systematic search of published studies and meta-regression analysis. Br J Anaesth. 2020;124:e4–7. https://doi.org/10.1016/j.bja.2019.09.036.
Patel PM, Drummond JC, Lemkkuil BP. Cerebral physiology and the effects of anesthetic drugs. In: Gropper MA, editor. Miller’s anesthesia. 9th ed. Amsterdam: Elsevier; 2019. p. 295–332.
Funding
This research received no external funding.
Author information
Authors and Affiliations
Contributions
Conceptualization, YM and YK; Methodology, YK, YI and TK; Formal analysis and investigation, YK, KO and YMI; Writing—original draft preparation, YK; Writing—review and editing, HS and YM; Supervision, YM.
Corresponding author
Ethics declarations
Competing interests
The authors have no relevant financial or non-financial interests to disclose.
Ethical approval
This prospective observational study was approved by the Hokkaido University Hospital Voluntary Clinical Research Review Board (Approval Number: ji-017-0137) and registered in the UMIN Clinical Trials Registry (UMIN000030799) prior to the start of the study. The study was conducted in accordance with the Declaration of Helsinki and relevant regulations.
Consent to participate
Written informed consent was obtained from all participants’ representatives.
Additional information
Publisher's Note
Springer Nature remains neutral with regard to jurisdictional claims in published maps and institutional affiliations.
Rights and permissions
Springer Nature or its licensor (e.g. a society or other partner) holds exclusive rights to this article under a publishing agreement with the author(s) or other rightsholder(s); author self-archiving of the accepted manuscript version of this article is solely governed by the terms of such publishing agreement and applicable law.
About this article
Cite this article
Kubo, Y., Itosu, Y., Kubo, T. et al. Cerebral oxygenation saturation in childhood: difference by age and comparison of two cerebral oximetry algorithms. J Clin Monit Comput 38, 639–648 (2024). https://doi.org/10.1007/s10877-023-01124-z
Received:
Accepted:
Published:
Issue Date:
DOI: https://doi.org/10.1007/s10877-023-01124-z