Abstract
Pyrethroid insecticides are one of the most commonly used pesticide groups, but these compounds have brought risks to non-target species, such as amphibians. This study evaluated the toxicological effects (mortality, swimming activity and oral morphology) caused to a South American species of anuran amphibian, Physalaemus gracilis, exposed to the pyrethroids cypermethrin and deltamethrin. Total spawnings of this anuran were collected in the natural environment and transported to the laboratory where they were kept under controlled conditions. Chronic assays were defined between 0.1 and 0.01 mg L−1 of cypermethrin, and 0.009 and 0.001 mg L−1 of deltamethrin. For cypermethrin, a further chronic toxicity test was performed at 0.05 and 2.0 mg L−1, with hatchlings at stages S.20–S.25. Cypermethrin and deltamethrin were lethal enough to kill over 70% of exposed tadpoles in 1 week at concentrations that can be found in nature (0.01–0.1 mg L−1). The exposure effects also influenced swimming activity and caused changes in oral morphology, which would make it difficult for the animals to survive in their natural habitat. Both pyrethroids presented a risk for P. gracilis, so they should be re-evaluated for non-target wild species.
Similar content being viewed by others
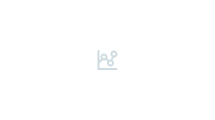
Explore related subjects
Discover the latest articles, news and stories from top researchers in related subjects.Avoid common mistakes on your manuscript.
Introduction
Pyrethroids are insecticides of plant origin, obtained from flowers of the Chrysanthemum genus (Kumar et al. 2005), and are considered one of the most commonly used agrochemical groups (Saillenfait et al. 2015). Pyrethroid insecticides cause immediate paralysis, mortality and shock effects that are called Knock-down in insects (Santos et al. 2007). The use of these insecticides is associated with several negative environmental impacts, mainly concerned with toxicity in non-target species, such as bees (Del Sarto et al. 2014), aquatic arthropods (Grisolia 2005; Gutiérrez et al. 2016), fish (Montanha and Pimpão 2012; Viran et al. 2003), mammals (Kaneko 2011) and amphibians (Aydin-Sinan et al. 2012; Macagnan et al. 2017; Wrubleswski et al. 2018).
Cypermethrin and deltamethrin are among the more toxic synthetic pyrethroids (Smith and Stratton 1986), especially towards aquatic organisms (Sánchez-Bayo 2012). These two pyrethroids are applied mainly in public health to control the vectors of diseases, such as malaria (Galardo et al. 2015), dengue, yellow fever, chikungunya and Zika virus (e.g., Bellinato et al. 2016; Rodríguez et al. 2017), in addition to several insects and mites in cattle (Mendes et al. 2011). Both insecticides are commonly used for pest control, and residues of these insecticides are present in worldwide surface waters of agricultural and urban areas (Jabeen et al. 2015; McKnight et al. 2015).
In Brazil, cypermethrin is the best-selling pyrethroid insecticide (Ribeiro and Pereira 2016), despite being classified as highly toxic and risky to the environment by the National Health Surveillance Agency (ANVISA 2019). In other countries, such as the United States, it has restricted use due to its high toxicity to fish (Bhutia et al. 2015). Cypermethrin is a persistent chemical that can be leached from the soil and transported to nearby waterways, culverts and sewage drains (Duaví et al. 2015).
Deltamethrin is used in agriculture (Belluta et al. 2010) and, mainly, in public health in Brazil (Boelaert et al. 2018; Gentile et al. 2004). This broad-spectrum synthetic insecticide is used intensively as a control method (Clark and Fernandez-Salas 2013) and, consequently, may appear in the water (Belluta et al. 2010; Chicati et al. 2012). In Brazil, the permitted mean value of cypermethrin in water for human consumption is 0.3 mg L−1 (300 µg L−1) (BRASIL 2014), but there is no legislation on deltamethrin in water.
Amphibians are susceptible to chemical contamination due to their skin permeability and the dependence of most species on the aquatic environment for reproduction and development of tadpoles, which makes them excellent models for toxicological tests (Buggren and Warburton 2007; Silva et al. 2013). These animals are present in several aquatic ecosystems with potential for contamination by pesticides. In addition, they are among the vertebrates that suffer most from human activities, resulting in population decline and the threat of extinction (Kouba et al. 2013). Despite their vulnerability, toxicological research on amphibians in Brazil remains rare (e.g., Franca et al. 2015; Leite et al. 2010; Rutkoski et al. 2018; Santana et al. 2015; Wrubleswski et al. 2018).
While the effects of pyrethroids on amphibians have been reported (e.g., Agostini et al. 2010; Aydin-Sinan et al. 2012; Izaguirre et al. 2000, 2006; Macagnan et al. 2017; Salibián 1992; Svartz and Pérez-Coll (2013); Svartz et al. 2016; Wrubleswski et al. 2018), little is known about how these products affect wildlife, especially when associated with aquatic environments. Some studies have shown that pyrethroids are toxic to amphibians, which can cause physiological changes, morphological abnormalities and delayed development, besides presenting neurotoxic and genotoxic potential (Agostini et al. 2010; David et al. 2012; Lajmanovich et al. 2014; Peltzer et al. 2011; Yu et al. 2013). These data emphasise the importance of understanding the effects of these compounds for the preservation of the species and the ecosystems in which they are inserted.
Physalaemus gracilis (crying frog) is a small anuran amphibian species (about 3 cm long) (Borges-Martins et al. 2007) from the Leptodactylidae family, native to southern South America, and occurring in Brazil, Uruguay and Argentina (Lavilla et al. 2010). It inhabits open areas and, during the reproductive period, is found in permanent lentic water bodies (Achaval and Olmos 2007). Physalaemus gracilis reproduce from spring to summer (September/March; Achaval and Olmos 2007), with deposition of 400 to 1300 eggs (Camargo et al. 2008) in foam nests on the water (Borges-Martins et al. 2007; Camargo et al. 2008). The species is widely distributed, tolerant to a wide range of habitats (Lavilla et al. 2010), including locations used for agriculture, and has a rapid tadpole development (Moreira et al. 2016). Consequently, P. gracilis can be considered a non-target vertebrate model for evaluating pesticide effects in studies of wildlife toxicology. In this study, we evaluated the sub-lethal effects of two pyrethroids (cypermethrin and deltamethrin) on the mortality, swimming activity and oral morphology of the anuran amphibian P. gracilis (Leptodactylidae).
Methods
Pesticides
We used two commercial pyrethroid insecticides, cypermethrin (Cipertrin EC [emulsifiable concentrate], 250 g L−1 active ingredient, 723 g L−1 inert ingredient) and deltamethrin (Decis 25 EC, 25 g L−1 active ingredient, 886 g L−1 inert ingredient).
To generate pesticide solutions, we dissolved the commercial-grade solution in distilled water to create a stock solution of 500 mg L−1. The stock solution was added with a micropipette to each glass container to obtain the desired working concentrations. The working concentrations used for tadpoles were 0.01, 0.02, 0.03, 0.05, 0.07 and 0.1 mg L−1 of cypermethrin, and 0.001, 0.0015, 0.003, 0.004, 0.006 and 0.009 mg L−1 of deltamethrin, chosen because they are below the maximum environmental concentration found in streams and rivers, namely 0.11–0.194 mg L−1 for cypermethrin (Belluta et al. 2010; Marino and Ronco 2005) and 0.005–0.05 mg L−1 for deltamethrin (Moraes et al. 2003; Todeschini 2013). For hatchlings, we tested the cypermethrin concentrations 0.05, 0.1, 0.5, 1.0, 1.5 and 2.0 mg L−1. We adopted different concentrations between tadpoles and hatchlings assays since P. gracilis embryos have proven to be more resistant to cypermethrin (Macagnan et al. 2017). The pesticide was added at the beginning of the experiment. The pyrethroid concentrations of the stock solution and experimental units could not be assessed at the Federal University of Fronteira Sul because of the absence of available testing laboratories.
The experiments were carried out during 7 days, within the half-life of the two pesticides. In aqueous solutions and under normal environmental conditions, cypermethrin presents a half-life between 9 and 17 days (Mantzosab et al. 2016), and 21 days for deltamethrin (Lutnicka et al. 1999), respectively.
Spawning collection and acclimatisation
Physalaemus gracilis total spawns were collected in the lake of the Federal University of the Southern Frontier, a reference area, without pesticides contamination influences (latitude −27.728681°; longitude −52.285852°), 24 h prior to oviposition. The collections occurred from October 2015 to March 2016. For the tadpole test, tadpoles in spawning condition were matured in the laboratory for about 1 month until reaching the development stage S.25 (Gosner 1960), in 10-L aquariums with artesian well-water, artificial aeration, a temperature of 24 ± 2 °C, humidity between 60 and 80%, and controlled lighting (12/12 h light/dark). The tadpoles were fed ad libitum with complete feed flakes for fish (Alcon Basic™; flaky vegetable with 45% crude protein) every 24 h. The hatchlings test was performed on the same day of collection. The spawning was placed in aquariums, and the S.20 embryos were separated with the aid of a stereomicroscope and placed in the assays.
The well-water used in the aquaria, controls and treatments had the following characteristics: 20 °C ± 2 °C, pH 7.0 ± 0.5, dissolved oxygen 5.0 ± 1.0 mg L−1, turbidity < 5, conductivity 160 ± 10 μS cm−1, alkalinity 9.74 mg CaCO3.L−1, Ca 6.76 mg L−1, Na 44.1 mg L−1, Mg 1.35 mg L−1, Fe 0.08 mg L−1, Ni < 0.001 mg L−1. The room was acclimated under controlled temperature, humidity and lighting, as described above.
Chronic toxicity assay with tadpoles
The chronic toxicity test (168 h) with tadpoles was performed for cypermethrin and deltamethrin, with tadpoles at S.25 (Gosner 1960) with operculum covering the gills (McDiarmid and Altig 1999). The length (10.61 ± 2.72 mm) and weight (0.09 ± 0.02 g) of tadpoles was measured at the start of the chronic assay, using a digital pachymeter and an analytical balance (to the nearest 0.001 g), respectively.
The chronic assays were carried out in static systems, in vials containing 500 mL of the test solution and a density of 1 tadpole per 100 mL, in six replicates, with 30 tadpoles exposed for each concentration. The animals were fed daily with 15% of the live weight, with the same food used in breeding. The physical–chemical parameters of the acclimation water were monitored. Mortality was checked daily, and dead individuals were removed.
During the chronic study, the swimming activity and oral malformation were analysed. The swimming activity of tadpoles was assayed in the presence of cypermethrin at 0.05, 0.07 and 0.1 mg L−1, and 0.004, 0.006 and 0.009 mg L−1 of deltamethrin, respectively. The swimming activity was measured once a day, by inducing a circular movement in the aquarium three times, with the aid of a glass rod, to stimulate the movement of tadpoles and hatchlings. As a comparison, the swimming activity pattern was based on five endpoints, numbered from 0 to 4 (modified from Rutkoski et al. 2018): (0) swimming movements equal to the control; (1) reduced movement relative to the control; (2) increased movement relative to the control; (3) spasmodic contraction; (4) without swimming activity.
The oral morphology was assessed with cypermethrin at 0.01, 0.02 and 0.03 mg L−1, and 0.001, 0.0015 and 0.003 mg L−1 of deltamethrin. This analysis was performed at the end of the chronic assay, with the aid of a stereomicroscope (Axio Scope.A1, AxioCam ERc 5s, Carl Zeiss, Göttingen, Germany) and Zen 2012 software, and each mouth was photographed for analysis. The first analysis was performed blindly, and the changes were observed and recorded without knowledge of which solution the animal was exposed. Then, the results of those exposed to pesticides were compared with the negative controls.
Chronic assay with hatchlings
For cypermethrin, the chronic toxicity assay (168 h) with hatchlings started in the development stage S.20 and ended when they reached the S.25, which is the earlier stage of development when the amphibian exits the embryonic gel coat, and marks the transition from a relatively immobile embryo to a free-swimming tadpole (McDiarmid and Altig 1999). This phase was chosen for the test because it is the phase in which swimming activity begins, and the mouth appears. The hatchlings were placed in 60-mm glass Petri dishes, with six replicates, and 5 hatchlings per plate, totalling 30 hatchlings per concentration of insecticide, and the negative control. The remainder of the assay design was equal to the chronic test with tadpoles. Dead hatchlings were removed every 24 h.
The swimming activity was evaluated at all the pyrethroid concentrations described, using the same analysis method performed with tadpoles. Oral morphology was assessed only at the highest concentrations of 1.0, 1.5 and 2.0 mg L−1. Due to the small size of the hatchlings, it was not possible to detail changes in the oral morphology, such as the lower and upper jaw and denticles. Hence, only the presence and absence of changes were identified.
Statistical analysis
Data were analysed for homogeneity of variance (Bartlett’s test) and normality of the errors (Shapiro–Wilk test) as a prelude to one-way analysis of variance (ANOVA), and post hoc Tukey’s test when p < 0.05. Comparisons were made between: (a) number of deaths at each pyrethroid concentration and under the various treatments; (b) number of deaths each 24 h and time of exposure (24, 48, 72, 96, 120, 144 and 168 h); (c) tadpoles swimming activity relative to pyrethroid concentration; (d) swimming activity each 24 h and time of exposure to pyrethroid; (e) number of tadpoles with malformation at each pyrethroid concentration and under the various treatments.
Concentration–response curves of mortality of tadpoles and hatchlings were adjusted using non-linear sigmoid models, by observing the significance of ANOVA and that of the coefficients of variation and the normality test (Shapiro–Wilk) for the mean values. The no-observable-effect concentration (NOEC) and lowest-observable-effect concentration (LOEC) were determined by the effect on mortality and swimming activity, using ANOVA, followed by Dunnett’s test to compare the means of each treatment with the control, when p < 0.05. The maximum acceptable toxicant concentration (MATC) was calculated from NOEC and LOEC and expressed mathematically as the geometric mean of NOEC and LOEC.
Ecological risk evaluation
The ecological risk was calculated using the hazard quotient (HQ) approach, where the formula for chronic risk was used: CHQ = EEC/NOEC. The EEC is an estimated (or maximum) environmental contaminant concentration. In this study, we consider the ECC as the maximum concentration of the pesticide registered in surface waters within the geographic range of the studied species. The EEC for cypermethrin (maximum level) reported within the geographical range of P. gracilis is 0.194 mg L−1 (Marino and Ronco 2005), and 0.05 mg L−1 for deltamethrin, respectively (Todeschini 2013). After HQ was calculated, it was compared with the United States Environmental Protection Agency (USEPA 2019) level of concern (LOC), where the risk presumption for aquatic animals is LOC = 1 for chronic risk. If HQ > 1, harmful effects are likely due to the contaminant in question.
For comparison, the toxicity exposure ratio (TER; Damalas and Eleftherohorinos 2011) was also used. TER is the inverse of HQ and is calculated by dividing the indicated toxic concentration by the predicted environmental concentration or EEC (chronic risk = NOEC/EEC). When TER < 10 for chronic risk, assessment is required.
Results
Chronic toxicity
The survival rate of tadpoles was 23.8% during the chronic trial, with higher survival under deltamethrin treatment than cypermethrin treatment (17.7%). There was a significant difference in mortality of P. gracilis tadpoles in relation to the exposure time (Fcyper (6,42) = 5.66, p < 0.001 and Fdelta (6,42) = 3.76, p < 0.001, significant at 96 and 120 h for both pyrethroids, Tukey’s test, p < 0.05), but not regarding the concentrations (Fcyper (6,42) = 1.01, p = 0.43; Fdelta (6,42) = 0.94, p = 0.47).
The survival rate of hatchlings during the chronic trial was 73.3%. There was a significant difference between mortality and time of exposure (F(6,42) = 3.35, p < 0.01, significant from 144 h, Tukey’s test, p< 0.05) but not among the concentrations (F(6,42) = 1.22, p= 0.31). The survival rate of the control was 90% for tadpoles and hatchlings.
Concentrations were not significant, but had a mortality effect, as can be observed in the concentration–response curve of P. gracilis (Fig. 1). The data over time were added as supplementary material (Table SM1).
Swimming activity
The tadpoles presented swimming activity different from the control already in the first 24 h of exposure to the two tested pesticides. In 48 h, tadpoles showed spasmodic contraction.
For cypermethrin, at the two highest tested concentrations, from 96 h, the tadpoles stopped swimming and presented lethargy (Table 1). There was a significant difference in the tadpoles swimming activity relative to pyrethroid concentration (Fcyper(3,161) = 113, p < 0.001; Fdelta(3,164) = 85.91, p < 0.001, significant at all concentrations, Tukey’s test, p < 0.05). The length of exposure was significant for both pyrethroids (Fcyper(6,116) = 5.72, p < 0.001, significant from 96 h; Fdelta(6,119) = 6.10, p < 0.001, significant from 24 h, Tukey’s test, p < 0.05). The NOEC; LOEC and MATC for tadpole swimming activity were respectively, 0.05, 0.07 and 0.06 mg L−1 for cypermethrin, and 0.004, 0.006 and 0.005 mg L−1 for deltamethrin.
Hatchlings also had a change in swimming activity from the first hours of exposure, with spasms in 48 h at the three highest concentrations of cypermethrin. The change in swimming activity was significant in the hatchlings exposed to cypermethrin, regarding the concentration (F(5,246) = 44.28; p < 0.001, significant from 0.5 mg L−1, Tukey’s test, p < 0.05) and exposure time (F(6,245) = 13.43; p < 0.001, significant from 72 h, Tukey’s test, p < 0.05). For hatchling swimming activity, NOEC was 0.1, LOEC was 0.5 mg L−1 and MATC was 0.3 mg L−1.
Oral morphology
A total of 66 tadpoles were analysed for oral morphology (alive at the end of the chronic toxicity assay), 39 tadpoles exposed to cypermethrin (n= 20) and deltamethrin (n = 19) concentrations, and 27 from the negative control (Table 2). Tadpoles were analysed at the end of the chronic assay (168 h). Counting only those exposed to pyrethroids, 15 (75%) of the 20 tadpoles exposed to cypermethrin, and 14 (73.6%) of the 19 tadpoles exposed to deltamethrin, presented alterations. Malformations in oral morphology were significant for cypermethrin (F(3,20) = 3.51, p = 0.03, significant at 0.01 and 0.03 mg L−1, Dunnet’s test, p < 0.05) but not for deltamethrin (F(3,20) = 2.23, p = 0.11).
The abnormalities identified in the tadpoles exposed to cypermethrin included alterations in the upper mandible (n = 7; 25.6%), lower mandible (n = 4; 23%), upper denticles line (n = 3; 7.6%), lower denticles line (n = 8; 33.3%), and upper and lower denticles line (n = 4; 30.7%). Several of these changes occurred together in the same tadpole (Fig. 2).
Changes in the oral morphology of Physalaemus gracilis exposed to cypermethrin and deltamethrin. a Tadpole from the negative control, unchanged. b Absence of denticles and partial absence of lower jaw. c Absence of denticles and absence of upper jaw. d Absence of lower denticles. e Absence of denticles and partial absence of upper jaw. f Absence of denticles and mandibles. (Arrows) Absence of jaws. (Stars) Absence of denticles. Axio Scope.A1, Carl Zeiss. Increased 10×
Regarding the hatchlings, at the concentration of 1.0 mg L−1, from 27 analysed, 16 presented alterations in oral morphology (59.2%). At concentrations of 1.5 and 2.0 mg L−1, all analysed individuals presented changes (100% from 14 hatchlings at 1.5 mg L−1, and 10 hatchlings at 2.0 mg L−1). No change was observed in the control. This malformation was significant in the three analysed concentrations in hatchlings (F(3,20) = 5.27, p < 0.01, Tukey’s test, p < 0.05).
Ecological risk evaluation
It was only possible to calculate the ecological risk evaluation for swimming activity since it was the only analysis where the concentration was significant. In the ecological risk evaluation using HQ, the chronic risk for both pyrethroids was CHQ > 1, with probable harmful effects. In TER calculation, the result for chronic risk for cypermethrin and deltamethrin was below 10, indicating a need for evaluation. The results are presented in Table 3.
Discussion
Cypermethrin and deltamethrin were lethal enough to kill more than 70% of the tadpoles exposed for 1 week to the concentrations examined in this study. These results are consistent with acute toxicity, but at concentrations that may be considered environmentally relevant. Within the geographic distribution of P. gracilis, cypermethrin has already been registered in surface waters at concentrations between 0.11 and 0.194 mg L−1 (Belluta et al. 2010; Marino and Ronco 2005), while deltamethrin concentrations ranged from 0.005 to 0.05 mg L−1 (Moraes et al. 2003; Todeschini 2013), levels which lead to a consistent concern about the toxicity of these insecticides for amphibians. Although laboratory-forced exposure is continuous and mandatory, the pesticide concentrations in amphibian habitats are larger than those tested in this study, as described above. Thus, in this study, we sought to use sub-concentrations relative to found in surface waters in Brazil, in addition to limiting exposure to a short time, inside the half-life of the insecticides. Nonetheless, the studied concentrations were strong enough to kill a significant number of tadpoles in the 7-day continuous exposure period.
The two pyrethroids altered the swimming of tadpoles and hatchlings of P. gracilis at a level that would hinder the survival of the animals in their natural habitat. Recovery of swimming activity was observed in hatchlings exposed to 0.05 mg L−1 cypermethrin, but at this same concentration, the tadpoles presented spasmodic contractions at 48 h of exposure. A large number of tadpoles and hatchlings with spasms and paralysis at the end of the 7-day exposure may be related to the neurotoxic effects of these pyrethroids. The paralysis caused by cypermethrin, and the dramatic reduction of swimming caused by deltamethrin, might be interpreted as a neurological effect (Materna et al. 1995; Narahashi 1992). Other studies with pyrethroids also report an alteration in tadpole swimming (Biga and Blaustein 2013; Edwards et al. 1986; Junges et al. 2017; Macagnan et al. 2017; Wrubleswski et al. 2018), associating these effects with the toxicity of the compounds on the nervous system (Velásquez et al. 2017; Yilmaz et al. 2008). In nature, reducing swimming increases the risk of predation (Pérez-Iglesias et al. 2015), making the food demand unfeasible and generating growth and development delays (Bridges 1997). To compensate for the toxic effects of pesticides, amphibians expend energy trying to restore their physiological balance, which can cause changes in swimming activity and reduction of physiological fitness and physical structure, as well as or, also, death (Greulich and Pflugmacher 2003; Pérez-Iglesias et al. 2015). Together, these factors impact on the life history of the animal and, consequently, on the population exposed to pyrethroids.
Besides causing a reduction in swimming activity, both studied pyrethroids caused mouth malformation, with partial or total absence of the mandible and denticles. The tadpole buccal structure acts to grasp and manipulate food: jaws cut large foods into smaller pieces, and teeth rows act as scrapers to remove food from rocks and plant surfaces (Vitt and Caldwell 2014). Any abnormality in the mouth is a disadvantage in nature, as it affects the individual’s ability to obtain food. Morphological abnormalities were found in other amphibians exposed to cypermethrin (Agostini et al. 2010; David et al. 2012; Greulich and Pflugmacher 2004), including mouth abnormalities in Boana pulchellus (Agostini et al. 2010).
The ecological risk assessment (ERA) aims to evaluate the likelihood and extent of adverse effects that may occur or are occurring as a result of exposure to pollutants (USEPA 2019). The ERA is a tool for decision-making (Hill et al. 2000) and can be used for regulatory purposes, as well as in the development of environmental quality guidelines (Solomon and Takacs 2001). Both pyrethroids tested in this study presented a chronic risk for swimming activity of P. gracilis, showing that they are toxic to amphibians and should be re-evaluated for native non-target species. Brazilian regulation allows 0.3 mg of cypermethrin per litre of water (BRASIL 2014), which is above the maximum acceptable toxicant concentration observed for swimming activity of tadpoles, and equal to the MATC for hatchlings of P. gracilis. For deltamethrin, there are no regulations or other studies showing the risk of this substance to native species.
The species studied here is found in agricultural areas (Moreira et al. 2014) with tadpoles developing at the bottom of temporary puddles, lakes and lagoons (Loebmann 2005), with potential for contamination by pesticides, such as pyrethroids. Commercial formulations of cypermethrin have been shown to be more toxic than the isolated active ingredient of cypermethrin, and it also remained viable in the freshwater aquatic ecosystem for a longer period (Majumder and Kajirav 2015). For deltamethrin, it is known that the commercial formulation may cause ecologically significant effects on aquatic systems (Giddings et al. 2014).
Conclusions
In conclusion, this study showed that the pyrethroids deltamethrin and cypermethrin were significantly toxic to the larval phase of P. gracilis. Thus, tadpoles exposed to cypermethrin and deltamethrin in their natural environment may undergo changes that would lead to the reduction of populations and communities. The chronic concentrations used in this study are very low in relation to those observed/quantified in the environment, which leads to a real concern about the survival of the populations of anurans, mainly of P. gracilis. As demonstrated by the results of this study, exposure to pyrethroids may impair skills required for the species survival.
References
Achaval F, Olmos A (2007) Guía de anfibios y reptiles del Uruguay. 3rd edn. Graphis, Montevideo
Agostini GM, Natale GS, Ronco AE (2010) Lethal and sublethal effects of cypermethrin to Hypsiboas pulchellus tadpoles. Ecotoxicology 19:1545–1550
ANVISA (2019) Agência Nacional de vigilância Sanitária. National Health Surveillance Agency. Ministry of Health, Brazil. http://portal.anvisa.gov.br/registros-e-autorizacoes/agrotoxicos/produtos/monografia-de-agrotoxicos/autorizadas. Acessed 19 Jun 2019
Aydin-Sinan H, Güngördü A, Ozmen M (2012) Toxic effects of deltamethrin and λ-cyhalothrin on Xenopus laevis tadpoles. J Environ Sci Health Part B 47:397–402
Bellinato DF, Viana-Medeiros PF, Araújo SC, Martins AJ, Lima JBP, Valle D (2016) Resistance status to the insecticides temephos, deltamethrin, and diflubenzuron in Brazilian Aedes aegypti populations, BioMed Res Int 2016. https://doi.org/10.1155/2016/8603263
Belluta I, Almeida AA, Coelho IC, Nascimento AB, A. Silva MM (2010) Avaliação temporal e espacial no córrego do Cintra (Botucatu – SP) frente aos defensivos agrícolas e parâmetros físico-químicos de qualidade da água—Um estudo de caso. Energ na Agricultura 25:54–73
Bhutia D, Rai BK, Pal J (2015) Hepatic cytochrome P450 as biomarkers of cypermethrin toxicity in freshwater teleost, Channa punctatus (Bloch). Braz Arch Biol Technol 58:131–136. https://doi.org/10.1590/S1516-8913201400049
Biga LM, Blaustein AR (2013) Variations in lethal and sublethal effects of cypermethrin among aquatic stages and species of anuran amphibians. Environ Toxicol Chem 32:2855–2860. https://doi.org/10.1002/etc.2379
Boelaert M, Burza S, Romero G (2018) Control and public health aspects. In: Bruschi F, Gradoni L (eds) The leishmaniases: old neglected tropical diseases. Springer. https://doi.org/10.1007/978-3-319-72386-0_10
Borges-Martins M, Colombo P, Zank C, Becker FG, Melo MTQ (2007) Anfíbios. In: Becker FG, Ramos RA, Moura LA (eds) Biodiversidade: Regiões da Lagoa do Casamento e dos Butiazais de Tapes, Planície Costeira do Rio Grande do Sul, Ministério do Meio Ambiente, Brasília, p 276–291
BRASIL (2014) Health Department of Rio Grande do Sul State, N° 320. Porto Alegre, RS, Brazil. https://www.legisweb.com.br/legislacao/?id=269539. Acessed 5 Sept 2018
Bridges CM (1997) Tadpole swimming performance and activity affected by acute exposure to sublethal levels of carbaryl. Environ Toxicol Chem 16:1935–1939. https://doi.org/10.1002/etc.5620160924
Buggren WW, Warburton S (2007) Amphibians as animal models for laboratory research in physiology. ILAR J 3:260–269
Camargo A, Sarroca M, Maneyro R (2008) Reproductive effort and the egg number vs. size trade-off in Physalaemus frogs (Anura: Leiuperidae). Acta Oecologica 34:163–171. https://doi.org/10.1016/j.actao.2008.05.003
Chicati ML, Nanni MR, Cézar E (2012) Chemical contamination of water in irrigated rice on Paraná State, Brazil. Semina 33:1455–146. https://doi.org/10.5433/1679-0359.2012v33n4p1455
Clark GG, Fernandez-Salas I (2013) Mosquito vector biology and control in Latin America—a 23rd symposium. J Am Mosq Control Assoc 29:251–269. https://doi.org/10.2987/13-6356.1
Damalas CA, Eleftherohorinos IG (2011) Pesticide exposure, safety issues, and risk assessment indicators. Int J Environ Res Public Health 8:1402–1419 https://doi.org/10.3390/ijerph8051402
David M, Marigoudar RS, Patil KV, Halappa R (2012) Behavioral, morphological deformities and biomarkers of oxidative damage as indicators of sublethal cypermethrin intoxication on the tadpoles of D. melanostictus (Schneider, 1799). Pestic Biochem Physiol 2:127–134
Del Sarto MCL, Oliveira EE, Guedes RNC, Campos LAO (2014) Differential insecticide susceptibility of the Neotropical stingless bee Melipona quadrifasciata and the honey bee Apis mellifera. Apidologie 5:626–636
Duaví WC, Gama AF, Morais PCV, de Oliveira AHB, do Nascimento RF, Cavalcante RM (2015) Contamination of aquatic environments by “urban pesticides”: the case of Cocó and Ceará rivers, fortaleza—Ceará, Brazil. Quimica Nova 38(5):622–630. https://doi.org/10.5935/0100-4042.20150055
Edwards R, Millburn P, Hutson DH (1986) Comparative toxicity of cis-cypermethrin in rainbow trout, frog, mouse and quail. Toxicol Appl Pharmacol 84:512–522
Franca MT, Paiva TCB, Marcantônio AS, Teixeira PC, Ferreira CM (2015) Acute toxicity and ecotoxicological risk assessment of rice pesticides to Lithobates catesbeianus tadpoles. J Environ Sci Health Part B 50:406–410. https://doi.org/10.1080/03601234.2015.1011950
Galardo AKR, Póvoa MM, Sucupira IMC, Galardo CD, dos Santos RLC (2015) Anopheles darlingi and Anopheles marajoara (Diptera: Culicidae) susceptibility to pyrethroids in an endemic area of the Brazilian Amazon. Rev da Soc Brasileira de Med Tropical 48(6):765–769. https://doi.org/10.1590/0037-8682-0082-2015
Gentile AG, Sartini JL, Campos MC, Sánchez JF (2004) Air temperature elevation as an alternative for the control of deltamethrin-resistant Triatoma infestans (Hemiptera, Reduviidae). Cad de Saúde Pública 20(4):1014–1019
Giddings J, Dobbs M, McGee S, Henry K, Mitchell G, McCoole M, Allen R, Whatling P, Freedlander D, Jackson S, Hendley P, Ritter A, Desmarteau D, Holmes C, Wirtz J, Campana D (2014) Higher-tier risk characterization of agricultural uses of synthetic pyrethroids: species sensitivity distributions, species response distributions, risk quotients, joint probability curves, and risk statements. Poster Pirethroid working group. US task force. http://www.complianceservices.com/wp-content/uploads/2014/08/Giddings_IUPAC-2014_Risk-characterization-poster.pdf. Acessed 29 Sept 2019
Gosner KL (1960) A simplified table for staging anuran embryos and tadpoles with notes on identification. Herpetologica 3:183–189
Greulich K, Pflugmacher S (2003) Differences in susceptibility of various life stages of amphibians to pesticide exposure. Aquat Toxicol 3:329–336
Greulich K, Pflugmacher S (2004) Uptake and effects on detoxication enzymes of cypermethrin in embryos and tadpoles of amphibians. Arch Environ Contam Toxicol 47:489–495. https://doi.org/10.1007/s00244-004-2302-3
Grisolia CK (2005) Agrotóxicos: mutações, câncer e reprodução. Universidade de Brasília, Brasília
Gutiérrez Y, Santos HP, Serrão JE, Oliveira EE (2016) Deltamethrin-mediated toxicity and cytomorphological changes in the midgut and nervous system of the mayfly Callibaetis radiates. PLoS One 11. https://doi.org/10.1371/journal.pone.0152383
Hill RA, Chapmann PM, Mann GS, Lawrence GS (2000) Level of detail in ecological risk assessments. Mar Pollut Bull 40(6):471–477. https://doi.org/10.1016/S0025-326X(00)00036-9
Izaguirre MF, Lajmanovich RC, Peltzer PM, Soler AP, Casco VH (2000) Cypermethrin-induced apoptosis in the telencephalon of Physalaemus biligonigerus tadpoles (Anura: Leptodactylidae). Bull Environ Contam Toxicol 4:501–507
Izaguirre MF, Marín L, Vergara MN, Lajmanovich RC, Peltzer P, Casco VH (2006) Modelos experimentales de anuros para estudiar los efectos de piretroides. Cienc, Docencia y Tecnolía 32:181–206
Jabeen F, Chaudhry AS, Manzoor S, Shaheen T (2015) Examining pyrethroids, carbamates and nicotenoids in fish, water and sediments from the Indus river for potential health risks. Environ Monit Assess 187–29. https://doi.org/10.1007/s10661-015-4273-4
Junges CM, Maglianese MI, Lajmanovich RC, Peltzer PM, Atademo AM (2017) Acute toxicity and etho-toxicity of three insecticides used for mosquito control on Amphibian tadpoles Water Air Soil Pollut 228:143. https://ez372.periodicos.capes.gov.br/, https://doi.org/10.1007/s11270-017-3324-6
Kaneko H (2011) Pyrethroids: mammalian metabolism and toxicity. J Agric Food Chem 59:2786–2791. https://doi.org/10.1021/jf102567z
Kouba AJ, Lloyd RE, Houck ML, Silla AJ, Calatayud N, Trudeau VL, Clulow J, Molina F, Langhorne C, Vance C, Arregui L, Germano J, Lermen D, Della Togna G (2013) Emerging trends for bio banking amphibian genetic resources: the hope, reality and challenges for the next decade. Biol Conserv 164:10–21. https://doi.org/10.1016/j.biocon.2013.03.010
Kumar A, Singh SP, Bhakuni RS (2005) Secondary metabolites of chrysanthemum genus and their biological activities. Curr Sci 89(9):1489–1501. http://www.jstor.org/stable/24110912
Lajmanovich RC, Cabagna-Zenkusen MC, Attademo AM, Jungesc M, Peltzer PM, Bassó A, Lorenzatti E (2014) Inducion of micronuclei and nuclear abnormalities in tadpoles of the common toad (Rhinella arenarum) treated with glifosinate –ammonium. Mutat Res 769:7–12. https://doi.org/10.1016/j.mrgentox.2014.04.009
Lavilla E, Kwet A, Segalla MV, Baldo JLD (2010) Physalaemus gracilis. the iUCN red list of threatened species. https://doi.org/10.2305/IUCN.UK.2010-2.RLTS.T57258A11610839.en. Acessed 19 Jun 2019
Leite PZ, Margarido TCS, Lima D, Rossa-Feres DC, Almeida EA (2010) Esterase inhibition in tadpoles of Scinax fuscovarius (Anura, Hylidae) as a biomarker for exposure to organophosphate pesticides. Environ Sci Pollut Res Int 8:1411–1421
Loebmann D (2005) Guia Ilustrado: Os anfíbios da região costeira do extremo sul do Brasil. Coleção manuais de campo. USEB, Pelotas
Lutnicka H, Bogacka T, Wolska L (1999) Degradation of pyrethroids in an aquatic ecosystem model. Water Res 33(16):3441–3446. https://doi.org/10.1016/S0043-1354(99)00054-8
Macagnan N, Rutkoski CF, Kolcenti C, Vanzetto VV, Macagnan L, Sturza P, Hartmann PA, Hartmann MT (2017) Toxicity of cypermethrin and deltamethrin insecticides on embryos and larvae of Physalaemus gracilis (Anura:Leptodactylidae). Environ Sci Pollut Res 24:20699–20704. https://doi.org/10.1007/s11356-017-9727-5
Majumder R, Kajirav A (2015) Variations in acute toxicity between technical grade and commercial formulation of cypermethrin to some non-target freshwater organisms. Int J Curr Res 7(6):16755–16759
Mantzosab N, Karakitsouac A, Helac I, Konstantinoua D (2016) Environmental fate of the insecticide cypermethrin applied as microgranular and emulsifiable concentrate formulations in sunflower cultivated field plots. Sci Total Environ 541:542–550. https://doi.org/10.1016/j.scitotenv.2015.09.11
Marino D, Ronco EA (2005) Cypermethrin and chlorpyrifos concentration levels in surface water bodies of the Pampa Ondulada, Argentina. Bull Environ Contam Toxicol 75(4):820–826
Materna EJ, Rabeni CF, Lapoint TW (1995) Effects of the synthetic pyrethroid insecticide, esfenvalerate, on girinol leopard frogs (Rana spp.). Environ Toxicol Chem 14:613–622. https://doi.org/10.1002/etc.5620140409
McDiarmid RW, Altig R (1999) Tadpoles: the biology of anuran. The University of Chicago Press, Chicago and London
McKnight US, Rasmussen JJ, Kronvang B, Binning PJ, Bjerg PL (2015) Sources, occurrence and predicted aquatic impact of legacy and contemporary pesticides in streams. Environ Pollut 200:64–76. https://doi.org/10.1016/j.envpol.2015.02.015
Mendes MC, Lima CKP, Nogueira AHC, Chiebao DP, Gabriel FHL, Ueno TEH, Namindome A, Klafke GM (2011) Resistance to cypermethrin, deltamethrin and chlorpyriphos in populations of Rhipicephalus (Boophilus) microplus (Acari:Ixodidae) from small farms of the State of São Paulo, Brazil. Vet Parasitol 178(3-4):383–388. https://doi.org/10.1016/j.vetpar.2011.01.006
Montanha FP, Pimpão CT (2012) Efeitos Toxicológicos de Piretróides (Cipermetrina e Deltametrina) em peixes—Revisão. Rev Científica Eletrônica de Med Veterinária 18:58
Moraes R, Elfvendahl S, Kylin H, Molander S (2003) Pesticide residues in rivers of a Brazilian rain forest reserve: assessing the potential concern for effects on freshwater biota and human health. Ambio 4:258–263. https://doi.org/10.1579/0044-7447-32.4.258
Moreira LFB, Knauth DS, Maltchik L (2014) Checklist of amphibians in a rice paddy area in the Uruguayan savanna, southern Brazil. Check List 10(5):1014–1019
Moreira LFB, Moura RG, Maltchik L (2016) Stop and ask for directions? Factors affecting anuran detection and occupancy in Pampa farmland ponds. Ecol Researcher 31:65–74. https://doi.org/10.1007/s11284-015-1316-9
Narahashi T (1992) Nerve membrane Na+ channels as targets of insecticides. Trends Pharmacol Sci 13:236–241
Peltzer PM, Lajmanovich RC, Sanchez LC, Attademo A, Junges C, Bionda CL, Martino A, Bassó A (2011) Morphological abnormalities in amphibian populations from the mid-eastern region of Argentina. Herpetological Conserv Biol 3:432–442
Pérez-Iglesias JM, Soloneski S, Nikoloff N, Natale GS, Larramendy ML (2015) Toxic and genotoxic effects of the imazethapyr-based herbicide formulation Pivot H on montevideo tree frog Hypsiboas pulchellus tadpoles (Anura, Hylidae). Ecotoxicol Environ Saf 119:15–24
Ribeiro DS, Pereira TS (2016) Our every day life pesticide. O agrotóxico nosso de cada dia. Rev de ciências da saúde 28:14–26
Rodríguez MM, Crespo A, Hurtado D, Fuentes I, Rey J, Bisset JA (2017) Diagnostic doses of insecticides for adult Aedes aegypti to assess insecticide resistance in Cuba. J Am Mosq Control Assoc 33:142–144. https://doi.org/10.2987/16-6593.1
Rutkoski CF, Macagnan N, Kolcenti C, Vanzetto VV, Sturza P, Hartmann PA, Hartmann MT (2018) Lethal and sublethal effects of the herbicide atrazine in the early stages of development of Physalaemus gracilis (Anura: Leptodactylidae). Arch Environ Contamination Toxicol 74(4):587–593. https://doi.org/10.1007/s00244-017-0501-y
Saillenfait AM, Ndiaye D, Sabaté JP (2015) Pyrethroids: esposure and health effects—an update. Int J Hyg Environ Health 218:281–292. https://doi.org/10.1016/j.ijheh.2015.01.002
Salibián A (1992) Effects of deltamethrin on the South American toad, Bufo arenarum, Tadpoles. Bull Environ Contam Toxicol 48:616–621
Sánchez-Bayo F (2012) Insecticides mode of action in relation to their toxicity to non-target organisms. J Environ Analytic Toxicol S4(002):1–9. https://doi.org/10.4172/2161-0525.S4-002
Santana JM, Reis A, Teixeira PC, Ferreira FC, Ferreira CM (2015) Median lethal concentration of formolaldehyde and its genotoxic potential in bullfrog tadpoles (Lithobates catesbeianus). J Environ Sci Health Part B 1–5, https://doi.org/10.1080/03601234.2015.1067095
Santos MAT, Areas MA, Reyes FGR (2007) Piretróides—Uma visão geral. Alimentos e Nutrção 3:339–349
Silva HSVP, Santos CL, Ferreira Pereira SR, Luvizotto Santos R, Andrade GV, Nunes GS (2013) Toxicidade aguda e genotoxicidade do agrotóxico comercial folisuper 600BR a girinos de Physalaemus cuvieri (Anura: Leiuperidae). Pesticidas 23:1–10
Smith TM, Stratton GW (1986) Effects of synthetic pyrethroid insecticides on nontarget organisms. In: Gunther FA (ed) Residue reviews, 97. Springer, New York, NY
Solomon KR, Takacs P (2001) Probabilistic risk assessment using species sensitivity distributions In: Postuma L, Traas T, Suter GW (eds), Species sensitivity distributions in risk assessment, CRC Press, Boca Raton, FL
Svartz G, Meijide F, Coll CP (2016) Effects of a fungicide formulation on embryo-larval development, metamorphosis, and gonadogenesis of the South American toad Rhinella arenarum. Environ Toxicol Pharmacol 45:1–7. https://doi.org/10.1016/j.etap.2016.05.008
Svartz GV, Pérez-Coll CS (2013) Comparative toxicity of cypermethrin and a commercial formulation on Rhinella arenarum larval development (Anura: Bufonidae). Int J Environ Health 6. https://doi.org/10.1504/IJENVH.2013.056973
Todeschini BH (2013) Avaliação da presença de agrotóxicos por cromatografia líquida acoplada a espectrometria de massas em águas superficiais e na rede pública de abastecimento de água no Rio Grande do Sul. Dissertation, Universidade Federal de Santa Catarina, Florianópolis
USEPA (2019) United States Environmental Protection Agency. https://www.epa.gov/pesticide-science-and-assessing-pesticide-risks/technical-overview-ecological-risk-assessment-0. Acessed 11 Jun 2019
Velásquez TMT, Muñoz LMH, Bautista MHB (2017) Toxicidad aguda del insecticida cipermetrina (Cypermon® 20 EC) en cuatro especies de anuros colombianos. Acta Biológica Colombiana 22(3):340–347. https://doi.org/10.15446/abc.v22n3.62631
Viran R, Unlu EF, Polat H, Koçak O (2003) Investigation of acute toxicity of deltamethrin on guppies (Poecilia reticulata). Ecotoxicol Environ Saf 1:82–85
Vitt LJ, Caldwell JP (2014) Herpetology: an introductory biology of Amphibians and Reptiles. 4th edn. Elsevier, USA
Wrubleswski J, Reichert Júnior FW, Galon L, Hartmann PA, Hartmann MT (2018) Acute and chronic toxicity of pesticides on tadpoles of Physalaemus cuvieri (Anura, Leptodactylidae). Ecotoxicology 27:360–368. https://doi.org/10.1007/s10646-018-1900-1
Yilmaz Ş, Çömelekoğlu Ü, Coşkun B, Balli E, Özge A (2008) Effects of cypermethrin on isolated frog sciatic nerve: an ultrastructural study. Turkish J Med Sci 38(2):121–125
Yu S, Wages MR, Cai Q, Maul JD, Cobb GP (2013) Lethal and sublethal effects of three insecticides on two developmental stages of Xenopus laevis and comparison whit other amphibians. Environ Toxicol Chem 9:2056–2064
Acknowledgements
We thank Gregori B. Bieniek for technical support. We are grateful to the Federal University of Fronteira Sul—UFFS for providing logistical support. Camila Rutkoski, Natani Macagnan and Cassiane Kolcenti were supported by fellowship from Fundação de Amparo a Pesquisa do Estado do Rio Grande do Sul—FAPERGS. This study is financed in part by the Coordenação de Aperfeiçoamento de Pessoal de Nivel Superior—Brazil (CAPES), finance code 001.
Author information
Authors and Affiliations
Corresponding author
Ethics declarations
Conflict of interest
The authors declare that they have no conflict of interest.
Ethical approval
This study was developed under ethical conditions and in accordance with national, international and institutional guidelines on the use of animals in the research. It was licensed by IBAMA (50398-1) and authorised by the Ethics Committee for Animal Use of the Federal University of Fronteira Sul.
Additional information
Publisher’s note Springer Nature remains neutral with regard to jurisdictional claims in published maps and institutional affiliations.
Supplementary information
Rights and permissions
About this article
Cite this article
Vanzetto, G.V., Slaviero, J.G., Sturza, P.F. et al. Toxic effects of pyrethroids in tadpoles of Physalaemus gracilis (Anura: Leptodactylidae). Ecotoxicology 28, 1105–1114 (2019). https://doi.org/10.1007/s10646-019-02115-0
Accepted:
Published:
Issue Date:
DOI: https://doi.org/10.1007/s10646-019-02115-0